Abstract
We compare the performance of an alternative method based on portable Fourier-transform infrared (FTIR) spectroscopy described in TGN M22, “Measuring Stack Gas Emissions Using FTIR Instruments,” to the Standard Reference Methods (SRMs) for CO (EN 15058), NOx (EN 14792), SO2 (EN 14791), HCl (EN 1911), and H2O (EN 14790). Testing was carried out using a Stack Simulator facility generating complex gas matrices of the measurands across concentration ranges of 0–75 mg m−3 and 0–100 mg m−3 CO, 0–200 mg m−3 and 0–300 mg m−3 NO, 0–75 mg m−3 and 0–200 mg m−3 SO2, 0–15 mg m−3 and 0–60 mg m−3 HCl, and 0–14 vol% H2O. The former values are the required monitoring range for each measurand as described in the European Union (EU) Industrial Emissions Directive (2010/75/EU) for waste incineration processes, and the latter are supplementary ranges representative of emissions from some large combustion plant processes. Test data were treated in accordance with CEN/TS 14793, and it was found that equivalency test criteria could be met across all concentration ranges with the exception of the NO supplementary range. The results demonstrated in principle where TGN M22/FTIR could be used in place of the existing SRMs to provide, as required under the Industrial Emissions Directive, annual validation/calibration of automated measuring systems (AMSs being permanently installed on industrial stacks to provide continuous monitoring of emissions to air). These data take a step toward the wider regulatory acceptance of portable FTIR providing the advantages of real-time calibration and quantification of all measurands on a single technique.
Implications: Portable FTIR offers significant advantages for the calibration (as is required by the EU’s Industrial Emissions Directive, 2010/75/EU) of process plant operators instrumentation installed for continuous monitoring of emissions to air. All key gaseous emission species regulated under the directive can be calibrated using a single technique, and the real-time calibration data allows issues with plant instrumentation to be identified sooner, reducing the amount of time where unreliable emissions data might be reported from the plant. This work takes an important step toward the regulatory acceptance of portable FTIR for the validation/calibration of in situ emissions monitoring systems.
Introduction
Standardized measurement methods are key to ensuring comparability of emissions monitoring data between nations and to ensuring that observed trends are real, providing confidence that there is compliance with international commitments to reduce pollution. The United Nations Economic Commission for Europe (UNECE) Convention on Long-range Transboundary Air Pollution (CLRTAP) has been in place since 1983 and requires all signatories to develop policies and strategies to limit and reduce air pollution (UNECE, Citation1979). Within the industrial sector such commitments were until recently fulfilled within Europe across seven different directives, including Large Combustion Plant (European Commission [EC], Citation2001), Waste Incineration (EC, Citation2000), and others, before being consolidated into the Industrial Emission Directive (IED) in 2011 (European Union [EU], Citation2010), which also brought in decreased emission limits for many industrial processes. Member states are required to collate emissions data for key species (NOx, SO2, etc.) from a range of processes and report these annually for compilation into the European Pollutant Release and Transfer Register (E-PRTR: EC, Citation2006). Consistent with the Kiev Protocol (United Nations [UN], Citation2003) the E-PRTR is publically available, ensuring there is transparency in terms of progress of attempts to reduce air pollution.
Historically, there are various national standards providing measurement methods for monitoring emissions to air; this was until the European Commission mandated the Comité Européen de Normalisation (CEN) to produce a series European Standard Reference Methods (SRMs) for key emission species including CO, EN 15058 (CEN, Citation2006); NOx, EN 14792 (CEN, Citation2007c); SO2, EN 14791 (CEN, Citation2005a); and HCl, EN 1911 (CEN, Citation2010). The SRMs ensure traceability of emission measurements to the international system of units (SI—Système International de’Unités; Bureau International des Poids et Measures [BIPM], Citation2006) and comparability of data across Europe. One of the most important roles of the SRMs is to calibrate in situ automated measuring systems (AMSs—in the United States and United Kingdom often referred to as continuous emission monitors [CEMs]), which have become commonplace (due to licencing) on many stacks.
Within Europe, EN 14181 (CEN, Citation2004) provides a framework for the quality assurance and control of AMSs, describing three quality assurance levels in addition to an annual surveillance test (AST). The first assurance level requires demonstration that the AMS uncertainty (derived from performance testing data) conforms to the uncertainty requirements laid out in applicable directives/regulation. The second quality assurance level (QAL2) prescribes a procedure for determining a calibration function for the AMS via parallel measurements of the stack emissions against the applicable SRM(s) carried out by a stack testing organization accredited to ISO 17025 (International Organization for Standardization [ISO], Citation2005), or directly approved by the relevant competent authority. The third assurance level involves the plant operator carrying out a procedure to check the AMS for drift and precision in order to demonstrate that the system continues to function within the uncertainty requirements. These checks are sometimes carried out weekly, although the frequency is dependent upon the method selected. The final requirement of EN 14181 is the annual surveillance test, which is carried out in intervening years between the QAL2, which itself is carried out once every 3 or 5 years, depending on the type of process plant. The AST again involves parallel measurements between the AMS and applicable SRM(s); however, fewer measurements are required than for determining a calibration function under QAL2. If the agreement between the AMS and SRM(s) remains within a given tolerance, then the AST is passed; if not, the requirement is to repeat QAL2 and determine a new calibration function. This framework helps support the intercomparability of European emissions data, ensuring that defined uncertainty limits are maintained, and provides traceability to the SI.
While the regulatory framework just described works well, there are regulatory and commercial drivers requiring continuous development of the measurement science. The SRMs for SO2 and HCl are based on a wet chemistry approach and hence unable to provide real-time data, and they require a significant amount of labor to carry out. For example, for SO2, EN 14791 describes using an H2O2(aq)-filled glass impinger train, heated probe, filter, and sample line in order to pump sample out of the stack, dissolving the measurand as sulfate ions. Captured solutions are then decanted into sample bottles and despatched under controlled conditions to a chemistry laboratory for sulfate quantification by ion chromatography or thorin titration. Under EN 14181 there are certain criteria designed to test whether there is an underlying issue with the AMS; for example, the stack testing team is required to report a fail if the AMS shows nonlinear deviation from the applicable SRM beyond a prescribed tolerance.
With real-time data it usually becomes clear within the first few hours if there is an issue with the AMS and if the calibration is going to be failed, enabling the process plant operator to take immediate action (i.e., bring in the manufacturer,) allowing the stack testing team to resume the EN 14181 testing with a minimal delay. Alternatively, with a wet chemistry approach there are several days of sampling (EN 14181 requires a minimum of 15 samples to be collected across 3 days to determine a calibration function under QAL2) and then some weeks pass before the sulfate analyses by the chemistry laboratory are returned, at which point it can sometimes be found that the AMS has failed the calibration and the entire process must be repeated. Furthermore, from the perspective of the regulator this represents increased time where the AMS has been reporting potentially unreliable data. Hence, there are clear incentives to carry out calibration using methods and techniques capable of producing real-time data. Although data for CO and NOx are produced in real-time (as these SRMs are based on instrumental techniques), it would be desirable to measure all measurands not only in real time but also using a single technique.
In principle, Fourier-transform infrared (FTIR) spectroscopy is a technique that can measure all the pollutants of interest, addressing many of the issues just outlined. Moreover, there are already some portable FTIR systems commercially available (MCERTS, Citation2014) that have been certified in accordance with EN 15267-3 (CEN, Citation2007a) (the standard that harmonised TUV and MCERTS type approval testing in 2007) for measurement of such pollutants. However, while certification under EN 15267-3 determines the system performance characteristics and from those data demonstrates that it is capable of operating within the required uncertainty stipulated in the IED, a method is still required in order to quality assure and control the technique. This ensures stack testing organizations are following common operational steps, have tests to detect if the portable system has fallen out of specification, and importantly establish an uncertainty budget, key to understanding the comparability of data and providing an unbroken link back to the SI. To address this need the Environment Agency for England (EA) has published the document TGN M22 (EA, Citation2012b), which describes an alternative method (AM) under which certified portable FTIR systems are operated ensuring quality assurance (QA)/quality control (QC) of the produced emissions monitoring data and therefore also of EN 14181-calibrated AMS installations.
There is a risk that if an alternative method and technique is used in place of the analogous SRM, comparability of data could be lost. However, the trade-off against this is that if new methods and techniques are not permitted then the measurement science in emissions monitoring may not progress. Recognizing this, CEN has provided a document describing procedure to determine whether one method and technique can be considered to produce equivalent data to another, namely, CEN/TS 14793 “Stationary Source Emission—Intralaboratory Validation Procedure for an Alternative Method Compared to a Reference Method” (CEN, Citation2005c). We report results comparing portable FTIR systems operated in accordance with TGN M22 to the SRMs for CO, NOx, SO2, HCl, and H2O (CEN, Citation2005b) via measurements using the National Physical Laboratory’s Stack Simulator facility. A range of test conditions is simulated and all collected data are treated in accordance with CEN/TS 14793 in order to determine whether the specified equivalency test criteria are satisfied.
Experimental
The NPL Stack Simulator facility consists of a recirculating stack equipped with 127-mm BSP (British Standard Piping) ports able to accommodate all standardized gas sampling apparatus. The simulator was run at 180°C with a recirculatory gas velocity of 12 m s−1, while water was vaporized into the gas phase using the incumbent liquid injection system. Further details of the facility can be found elsewhere (Coleman et al., Citation2013, Citation2010), along with data demonstrating homogeneous mixing within the simulator (Williams, Citation2011) via testing carried out in accordance with EN 15259 (CEN, Citation2007b).
Fifty-four different test matrices were generated within the Stack Simulator facility, covering the following concentration ranges for the measurands of interest: CO 0–100 mg m−3; NO 0–300 mg m−3; SO2 0–200 mg m−3; HCl 0–60 mg m−3; H2O 0–14 vol%. Concentrations were varied uncorrelated across these ranges for the 54 tests; also, additional compounds were included in some of the test mixtures as potential interferences, specifically, 15 mg m−3 NH3; 30 mg m−3 NO2; volatile organic compounds (VOC) mixture (9 mg m−3 CH4/8 mg m−3 C2H6/8.5 mg m−3 C3H8); 10 vol% CO2. The interferents were selected based on compounds typically emitted from many process types and also on the operating principle of the AM; for example, NH3 absorbs radiation in a region of the mid-infrared spectrum similar to that of SO2. The diluent gas within the simulator was dry 10 vol% O2/N2, created by blending the gas flow from the facility’s dry air and dry nitrogen generators in approximately equal ratio, and with the exception of water vapor all other gases were acquired from BOC Specialist Gases, UK.
In accordance with the requirements of CEN/TS 14793, two SRMs were run for each measurand. Two Horiba PG250 analysers were interfaced with two sampling ports via M & C PSS-5 gas conditioning and pumping systems. The PG-250 analysers contained both NDIR and chemiluminescence cells and hence were able to simultaneously monitor CO and NO, respectively. In addition to this, two borosilicate glass impinger trains were interfaced with two facility ports using Apex heated probes with titanium liner, insulated polytetrafluoroethylene (PTFE) lines, pump, and calibrated flowmeter. An alternating approach was used in that the two impinger trains were filled with solution to absorb SO2 (H2O2(aq)) and a run was completed before being exchanged with a second set of impinger trains filled with solution to absorb HCl (deionized water). Hence, fewer data were collected for these measurands compared to CO and NO. Lastly, as the SRM for H2O is based on gravimetry, the concentration of this measurand was determined by weight change in the SO2 and HCl impinger trains. Such an approach is in accordance with EN 14790 and is the most common application of this SRM, as rarely do stack testing organizations have a requirement to measure water vapor alone.
A sample run of the Stack Simulator was defined as averaging for 30 min. SO2 and HCl wet chemistry samples were analyzed for sulfate and chloride at the National Physical Laboratory (NPL) laboratories using ion chromatography. NPL is ISO 17025 accredited for the implementation of the described SRMs, including carrying out sulfate and chloride analyses. Also, all staff members involved in the testing were certified to MCERTS Level 2 under the Environment Agency for England Personnel Competency Standard (EA, Citation2012a).
Two ProtIR 204m portable FTIR systems were interfaced with the Stack Simulator using titanium probes, M & C PS4000 heated filters with ceramic membranes, and M & C heated lines (pumping apparatus being integral to the FTIR instruments). Both systems were operated by NPL staff certified to MCERTS Level 2 in accordance with TGN M22, the implementation of which NPL is ISO 17025 accredited. The ProtIR 204m FTIR system was certified under EN 15267-3 across the following ranges: 0–75 mg m−3 CO; 0–200 mg m−3 NO, 0 - 75 mg m−3 SO2; 0–15 mg m−3 HCl; 0–40 vol% H2O.
All acquired measurements were processed in accordance with the “in-field” testing element of CEN/TS 14793 and collated in tabular format as described in the document (), where r is the correlation coefficient for an orthogonal regression between the mean AM and mean SRM measurements; is the slope of the regression;
is the intercept of the regression;
is the standard deviation of reproducibility of the SRM;
is the mean of the SRM measurements;
is the repeatability variance of the SRM measurements;
is the repeatability variance of the AM measurements; and
is the maximum allowable standard deviation of repeatability for the SRM.
Table 1. CEN/TS 14793 pro forma for determining and summarising test data in comparing if an alternative method is equivalent to an SRM.
In accordance with CEN/TS 14793, values of and
were found for each measurand using formulas specific to each SRM. For example, for SO2, EN 14791 stipulates that the requirements for repeatability and reproducibility are found from
Results and Discussion
In terms of successfully demonstrating equivalence under CEN/TS 14793 there are two requirements that must be satisfied:
The expanded uncertainty associated with the AM must be determined in accordance with ISO 14956 (ISO, Citation2002) or ENV 13005 (CEN, Citation1999), and it shall not exceed that for the associated SRM at the emission limit value.
The in-field testing criteria of systematic deviation and repeatability must be conformed to.
These data provide evidence for the local competent authority to either accept or reject that the AM has been demonstrated as equivalent to the SRM and permit its use. However, it should be noted that compliance with these two criteria does not oblige the authority to accept equivalence and there are various legal and political rationales, meaning acceptance is more readily achieved in some member states than others (such rationales being beyond the scope of this paper). Hence, it is usual for the test laboratory to consult with the local competent authority before beginning such equivalency testing.
With regard to the first criterion just given, then, the performance characteristics of the measuring system must be determined. The simplest mechanism for doing this, as is the case for the data reported here, is to use a system that has been certified under EN 15267-3. The EN 15267-3 certificate, as mentioned previously, provides information regarding characteristics including drift, linearity, and others; hence from this it is possible to compile an uncertainty budget for each applicable measurand and determine an expanded uncertainty for comparison to the SRM. While some of the uncertainty sources associated with the measuring system may remain fixed, others, such as quality of calibration gas, losses in sampling systems, and so on are likely to vary between stack testing organizations (an area where providers can make improvements in the measurement services they offer), depending on the procedures they have written for implementation of the method (in this case, TGN M22). The procedures used in the testing described here have associated uncertainties for all five measurands (values not shown) that are not in excess of the applicable SRM, as evidenced in terms of NPL’s ISO 17025 accreditation. Given that measurement uncertainties vary between organizations and that acceptance of equivalence by a local competent authority is a case-specific topic, the testing described in the following focuses on the second criterion, namely, the in-field testing of systematic deviation and repeatability between the AM and SRM.
The in-field testing element of CEN/TS 14793 requires that two SRMs are operated in parallel with two AMs. An orthogonal regression is calculated between the mean SRM and mean AM measurements and criteria of correlation, slope, and intercept are applied. An orthogonal regression is used, as it is recognized that the SRM itself is not without uncertainty; hence it is appropriate to regress a straight line minimizing least squares along the x-axis in addition to the y-axis to take some account of this. In addition, to provide confidence that the two SRMs have been performed correctly and that therefore comparison of the AM is valid, the repeatability of the SRM is determined and compared to that required in the applicable CEN standard (e.g., eq 2 from EN 14791 in the case of SO2). The repeatability of the AMs must also meet this same requirement.
Under the IED it is a requirement to report emissions data corrected to dry conditions and at a reference oxygen concentration (with the reference value depending on the process type). In the case of the latter, O2 has no dipole change upon vibration and therefore does not satisfy the selection rule for FTIR. However, while O2 is irrelevant to FTIR and the discussions presented here, it should be noted that many FTIR system now possess O2 sensors embedded within the instrument’s gas cell. Hence, simultaneous monitoring of this measurand is often possible using a single measuring system. In contrast, FTIR is able to detect water vapor, and hence in terms of demonstrating (or otherwise) equivalence this is the first measurand considered.
It can be seen () that the repeatability requirement of EN 14790 is met by the SRM confirming validity of the data for comparison against the AM, which also conforms to the requirement showing an identical level of repeatability. The orthogonal regressions () shows compliance with all the systematic deviation test criteria (), and also shows no significant bias when tested at zero, that is, when water vapor is removed from the Stack Simulator. This last test demonstrates the advantage of using simulating facilities, as the same level of control is not possible on real process plants. Overall, the results evidence that across the concentration range tested the AM is able to produce data equivalent to the SRM and therefore can be used to correct the remaining measurand channels to dry conditions, which is found from
Figure 1. Orthogonal regression of mean AM (TGN M22/FTIR) vs. mean SRM (EN 14790/gravimetry) measurements of water vapor in the NPL Stack Simulator facility in a changing gas matrix of CO/NO/SO2/HCl at 180°C and 12 m s−1 velocity.
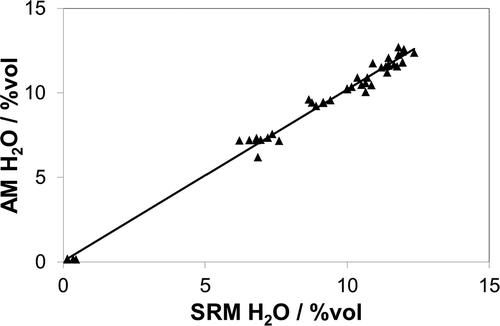
Table 2. CEN/TS 14793 test of equivalence of TGN M22/FTIR to EN 14790/gravimetry for measurements of water vapour across a concentration range of 0–14 vol%.
where is the concentration of the ith measurand under dry conditions,
is the concentration of the ith measurand under wet conditions, and
is the concentration of water vapor (vol%).
Correcting measurements of CO, NO, SO2, and HCl to dry conditions using eq 3 allows equivalency to be tested against each applicable SRM ( to ). The appropriate test range for a measuring system is linked to the emission limit value (ELV) of the measurand as stipulated in the IED. It is a requirement that the measuring system conforms to the performance characteristic criteria found in EN 15267-3 and when operated under the AM demonstrates equivalence in accordance with CEN/TS 14793 across the same range. For waste incineration processes the applicable range is defined as 1.5 × ELV, and under the IED the following ELVs can be found for the measurands of interest: 50 mg m−3 CO; 133 mg m−3 NO; 50 mg m−3 SO2; 10 mg m−3 HCl. It is seen from earlier (Experimental section) that the ProtIR 204m is certified across the waste incineration process range, as each certified range is 1.5 × ELV. This is a common approach in certification of measuring systems, as the performance criteria stipulated in EN 15267-3 are expressed as a percentage of concentration range; hence, in principle as the range increases the performance requirements become broader and therefore less stringent. While the required range on the waste incineration process is 1.5 × ELV, it is 2.5 × ELV on all other process types; hence if a measuring system conforms to the performance criteria across a waste incineration range, then it would also be expected to meet requirements for other process types. On waste incinerators it is typical to find a mixture of CO/NO/SO2/HCl being emitted; hence this is the type of process that is simulated in the testing.
For SO2 it is seen that the test criteria are met when equivalency is tested over the waste incineration range of 75 mg m−3 (). It is also seen that the AM appears to possess a better repeatability than the SRM. While the majority of the 54 test matrices generated in the Stack Simulator contain concentrations of SO2 spanning 0–75 mg m−3, a smaller number of the matrices include increased concentrations of up to 200 mg m−3. Concatenating the data allows equivalency to be tested over this supplementary range, where again it is found that test criteria are met and that the AM demonstrates better repeatability than the SRM (). The supplementary test range isn’t directly linked to a specific process in terms of multiples of a particular ELV as in the case of waste incineration. However, instead the range is representative of typical emission levels in that on large combustion plant processes emissions of 200 mg m−3 are sometimes found. Also, the VOC mix is deliberately included in some of the test matrices to simulate unburned hydrocarbons, which is something that is typically found in large combustion plants. In contrast, HCl is generally not found on such plants; however, the absence of a compound is unlikely to affect performance. The IED sets an emission limit of 200 mg m−3 for burning coal, biomass, peat, and other solid and liquid fuels on large combustion plants of >300 MW thermal input, so the test range is applicable to these type of processes.
Table 3. CEN/TS 14793 test of equivalence of TGN M22/FTIR to EN 14791/impinger train and ion chromatography for measurements of SO2 across a concentration range of 0–75 mg m−3.
Table 4. CEN/TS 14793 test of equivalence of TGN M22/FTIR to EN 14791/impinger train and ion chromatography for measurements of SO2 across a concentration range of 0–200 mg m−3.
Similar to SO2, NO is tested for equivalency across both a waste incineration and supplementary range of 200 mg m−3 () and 300 mg m−3 (), respectively. However, for this measurand the criteria are only met across the former range, while for the latter the intercept requirement for the orthogonal regression is failed. This is perhaps unexpected, as the values for slope and validation of the test () show a very close correlation between the methods. However, ultimately the cause of the noncompliance is due to the AM providing readings slightly greater than those of the SRM (not shown). However, it is interesting to note that without further work it is not possible to determine which method is actually closest to the “true value,” leading to the observation that if an SRM produces biased data, equivalency can only be demonstrated if the AM is equally biased.
Table 5. CEN/TS 14793 test of equivalence of TGN M22/FTIR to EN 14792/chemiluminescence for measurements of NO across a concentration range of 0–200 mg m−3.
Table 6. CEN/TS 14793 test of equivalence of TGN M22/FTIR to EN 14792/chemiluminescence for measurements of NO across a concentration range of 0–300 mg m−3.
With regard to CO, equivalency is successfully demonstrated over a waste incineration range of 75 mg m−3 (). In terms of other processes there are very few limits set for CO in the IED with the exception of large combustion plants burning gas, where the limit is 100 mg m−3. Including data up to this limit value, it is found that equivalency is also successfully demonstrated over this increased range ().
Table 7. CEN/TS 14793 test of equivalence of TGN M22/FTIR to EN 15058/NDIR for measurements of CO across a concentration range of 0–75 mg m−3.
Table 8. CEN/TS 14793 test of equivalence of TGN M22/FTIR to EN 15058/NDIR for measurements of CO across a concentration range of 0–100 mg m−3.
With respect to HCl, testing equivalence is less straightforward in that equations to determine test criteria of repeatability () and reproducibility (
) are not provided in EN 1911. Consequently, repeatability and reproducibility requirements are determined with reference to EN 15267-3. This standard as described earlier provides test requirements for certification of measuring systems; hence, it is reasonable to propose that an AM used with the FTIR technique should be able to meet these same requirements. The requirements for repeatability and reproducibility under EN 15267-3 are 2% and 3.3% of test range, respectively. Calculating the test criterion on this basis for
and
it is seen that across both a waste incineration range of 15 mg m−3 () and a supplementary range of 60 mg m−3 () equivalency is demonstrated. It should be noted that the equivalency testing across the former range is based only on 11 pairs of data; hence while this is still valid, it would be desirable to have further testing across this range in the future to build further confidence. With respect to the supplementary range, under the IED emission limits are only stipulated for HCl for waste incineration processes; however, apart from academic interest, testing across this supplementary range is relevant as there are some processes in the United Kingdom that are actually licenced as large combustion plants despite incinerating waste. This may be an abnormality unique to the United Kingdom; however, the result is that due to this licensing the required measuring system range is 2.5 × ELV rather than 1.5 × ELV. Consequently, even for the same ELV a greater measurement range is required, which is taken into account here.
Table 9. CEN/TS 14793 test of equivalence of TGN M22/FTIR to EN 1911/impinger train and ion chromatography for measurements of HCl across a concentration range of 0–15 mg m−3.
Table 10. CEN/TS 14793 test of equivalence of TGN M22/FTIR to EN 1911/impinger train and ion chromatography for measurements of HCl across a concentration range of 0–60 mg m−3.
Lastly, it was mentioned earlier with respect to NO that if the SRM is biased then equivalency can only be demonstrated if the AM is equally biased. An SRM both in terms of regulation and convention is considered to give the “accepted” answer; that is, for a given measurand, regulators, plant operators, and stack testing organizations all operate using the SRM as the accepted point of reference. Furthermore, when ELVs are set by regulators, some of the information that informs such decisions often comes from previous SRM measurements. Hence, in principle if the SRM has a bias, this same bias potentially influences the setting of ELVs and is also intrinsic to the AMS calibration function determined via parallel measurements against the applicable SRM(s) under EN 14181. Consequently, the measurement framework is internally consistent and assumes the SRM as the accepted answer, even if there is a systematic bias from the “true” answer. But when an SRM is validated under mandate from CEN prior to publication, would any bias not have been found?
The requirement is that validation work must be applicable, and in the case of the SRMs, that involves contracting several stack testing organizations to simultaneously carry out the draft SRM across a series of field trails at different process types. This provides both a data set for examination of variance and confidence that the SRM will work on different industrial processes. ISO 5725-2 (ISO, Citation1994a) and ISO 5725-6 (ISO, Citation1994b) are applied to determine the SRM repeatability and reproducibility from the collected data ( and
). However, the calculations are based on differences between the testing organizations as opposed to the differences between organizations and the “true” in-stack concentration (since it is impossible to know this). However, in addition to determining variance between stack testing organizations as a measure of random uncertainty, there is also consideration given to bias sources, although these are not validated via the fieldwork. Hence, while it is not possible to say that no SRM is without bias, it is likely that if a significant bias existed it would have been identified either in the validation work or through use, as the SRMs discussed here have been in use for many years.
Conclusions
It has been shown that an alternative method described in Technical Guidance Note M22 based on portable FTIR can produce emissions monitoring data for CO, NO, SO2, HCl, and H2O equivalent to the applicable Standard Reference Methods described in CEN publications EN 15058, EN 14792, EN 14791, EN 1911, and EN 14790, respectively (). Equivalency test criteria described in CEN/TS 14793 were met across a concentration range of 0–14 vol% for H2O and for the pollutants across the range required by the EU’s Industrial Emissions Directive (2010/75/EU) for waste incineration plants, specifically, 0–75 mg m−3 CO, 0–200 mg m−3 NO, 0–75 mg m−3 SO2, and 0–15 mg m−3 HCl. It was also shown that equivalency test criteria were met across supplementary ranges of 0–100 mg m−3 CO, 0–200 mg m−3 SO2, and 0–60 mg m−3 HCl. However, the equivalence test criteria were not met for NO across a supplementary range of 0–300 mg m−3, due to increased readings of the AM compared to the SRM. These data demonstrated where TGN M22/FTIR could in principle be used (in place of the existing suite of SRMs), providing real-time validation/calibration of automated measuring systems (permanently installed on industrial stacks to provide continuous emissions data) by a single technique.
Table 11. Summary of CEN/TS 14793 test of equivalence of TGN M22/FTIR to the European Standard Reference Methods for the Monitoring of Emissions from Stacks and Flues of H2O, SO2, NO, CO, and HCl.
Funding
We gratefully acknowledge joint funding from the Environment Agency for England, the European Metrology Research Programme (which is jointly funded by the EMRP participating countries within EURAMET and the European Union) and the United Kingdom’s Department for Business, Innovation and Skills (BIS) National Measurement System under the Chemistry and Biology Metrology Programme. We also thank Protea Ltd. for provision of some of the instrumentation used in the testing and Sonya Beccaceci for assistance in carrying out analyses of sulfate and chloride in collected samples.
Additional information
Funding
Notes on contributors
Simon Render
Marc D. Coleman is a Senior Research Scientist in the Environmental Measurements Group at the National Physical laboratory, UK, where his research interests revolve around the application of spectrometric measurement techniques to the measurement of emission sources and atmospheric pollution.Rod A. Robinson leads emissions monitoring research within the same institution supported by Chris Dimopoulos, Adam Lilley and Thomas O.M. Smith, all of whom possess expertise in different aspects of stack emissions monitoring techniques and the associated regulation. Simon Render is currently employed at E.ON, UK, as a Senior Environmental Advisor within the Generation Environment Team. Richard Camm is Managing Director of Protea Ltd. who manufactures the ProtIR 204m FTIR and Rupert Standring is a Senior Advisor within the Environment Agency for England.
References
- Bureau International des Poids et Measures. 2006. Le Système International de’Unités, 8th ed. www.bipm.org/
- Coleman, M.D., R.A. Robinson, M. Williams, R. Elliott, M. Clack, and A. Curtis. 2010. Demonstrating equivalence of an alternative method for SO2 emissions monitoring using NPL’s Stack Simulator facility. PPChem. 12(4):218.
- Coleman, M.D., R.A. Robinson, M.B. Williams, M.J. Clack, and D.M. Butterfield. 2013. State of UK emissions monitoring of stacks and flues: An evaluation of proficiency testing data for SO2, NO and particulates. Accred. Qual. Assur. 18:517–24. doi:10.1007/s00769-013-1011-x
- Comité Européen de Normalisation. 1999. Guide to the Expression of Uncertainty in Measurement. ENV 13005. https://www.cen.eu/
- Comité Européen de Normalisation. 2004. Stationary Source Emissions—Quality Assurance of Automated Measuring Systems. EN 14181. https://www.cen.eu/
- Comité Européen de Normalisation. 2005a. Stationary Source Emissions—Determination of Mass Concentration of Sulphur Dioxide—Reference Method. EN 14791. https://www.cen.eu/
- Comité Européen de Normalisation. 2005b. Stationary Source Emissions—Determination of the Water Vapour in Ducts. EN 14790. https://www.cen.eu/
- Comité Européen de Normalisation. 2005c. Stationary Source Emission—Intralaboratory Validation Procedure for an Alternative Method Compared to a Reference Method. CEN/TS 14793. https://www.cen.eu/
- Comité Européen de Normalisation. 2006. Stationary Source Emissions—Determination of the Mass Concentration of Carbon Monoxide (CO). Reference Method: Non-Dispersive Infrared Spectrometry. EN 15058. https://www.cen.eu/
- Comité Européen de Normalisation. 2007a. Air Quality—Certification of Automated Measuring Systems. EN 15267-3. https://www.cen.eu/
- Comité Européen de Normalisation. 2007b. Air Quality—Measurement of Stationary Source Emissions—Requirements for Measurement Sections and Sites and for the Measurement Objective, Plan and Report. EN 15259. https://www.cen.eu/
- Comité Européen de Normalisation. 2007c. Stationary Source Emissions—Determination of Mass Concentration of Nitrogen Oxides (NOx)—Reference Method: Chemiluminescence. EN 14792. https://www.cen.eu/
- Comité Européen de Normalisation. 2010. Stationary Source Emissions—Determination of Mass Concentration of Gaseous Chlorides Expressed as HCl. Standard Reference Method. EN 1911. https://www.cen.eu/
- Environment Agency for England. 2012a. Personnel Competency Standard for Manual Stack Emission Monitoring, version 8.1. https://www.gov.uk/
- Environment Agency for England. 2012b. Technical Guidance Note M22, Measuring Stack Gas Emissions using FTIR Instruments. version 3. https://www.gov.uk/
- European Commission. 2000. Directive 2000/76/EC of the European Parliament and of the Council of 4 December 2000 on the Incineration of Waste. Off. J. Eur. Commun. L332:91–111.
- European Commission. 2001. Directive 2001/80/EC of the European Parliament and of the Council of 23 October 2001 on the Limitation of Emissions of Certain Pollutants into the air from Large Combustion Plants. Off. J. Eur. Commun. L309:1–21.
- European Commission. 2010. Directive 2010/75/EU of the European Parliament and of the Council of 24 November 2010 on Industrial Emissions (Integrated Pollution Prevention and Control). Off. J. Eur. Commun. L334:17–119.
- European Commission. 2006. Regulation (EC) No 166/2006 of the European Parliament and of the Council of 18th January 2006 Concerning the Establishment of a European Pollutant Release and Transfer Register and Amending Council Directives 91/689/EEC and 96/61/EC. Off. J. Eur. Union L33:1–17.
- International Organization for Standardization. 1994a. Accuracy (Trueness and Precision) of Measurement Methods and Results—Part 2: Basic Method for the Determination of Repeatability and Reproducibility of a Standard Measurement Method. ISO 5725-2. http://www.iso.org
- International Organization for Standardization. 1994b. Accuracy (Trueness and Precision) of Measurement Methods and Results—Part 6: Use in Practice of Accuracy Values. ISO 5725-6. http://www.iso.org
- International Organization for Standardization. 2002. Air Quality—Evaluation of the Suitability of a Measurement Procedure by Comparison with a Required Measurement Uncertainty. ISO 14956. http://www.iso.org
- International Organization for Standardization. 2005. General Requirements for the Competence of Testing and Calibration Laboratories. ISO 17025. http://www.iso.org
- MCERTS. 2014. MCERTS Certified Products. http://www.siraenvironmental.com/UserDocs/mcerts/MCERTSCertifiedProductsCEMS.pdf (accessed October 2014).
- United Nations Economic Commission for Europe. 1979. Convention on Long-range Transboundary Air Pollution. Geneva, Switzerland: UNECE.
- United Nations. 2003. Protocol on Pollutant Release and Transfer Registers. Kiev, Ukraine, May 21.
- Williams, M.B. 2011. Gas Measurement Proficiency Testing Scheme Round 2. NPL Report AS57. http://www.npl.co.uk/publications