Abstract
Polychlorinated dibenzo-p-dioxins (PCDDs) and polychlorinated dibenzofurans (PCDFs) are a group of dangerous compounds, emitted mostly from anthropogenic sources, that have negative effects on human health. Therefore, it is interesting to analyze the emission patterns of dioxins proceeding from different sources around the world, to observe the actual trend of the transmission of dioxins and furans into the atmosphere.For that reason, the main objective of the present document is to provide a general assessment about the dioxin problematic, analyzing the main parameters that influence the ambient concentration of dioxins worldwide, and describing the most characteristic features of the fingerprint from different sources, while making emphasis in the importance that non-industrial sources are gaining over the last years in front of the decreasing tendency of industrial sources. The description of the most important abatement technologies for dioxins is also included in this review.
Implications: Given the negative effects of dioxins in human health, it is important to depict and locate the main sources of these dangerous compounds. Emissions proceeding from industrial facilities have decreased over the last years; however, other zones where nonindustrial sources used to be relevant contributors do not show the same decreasing tendency because it is more difficult to control this type of emissions. For that reason, future studies should focus on measuring and regulating this highly uncontrolled source of dioxins.
Introduction
Polychlorinated dibenzo-p-dioxins (PCDDs) and polychlorinated dibenzofurans (PCDFs) are a group of aromatic hydrocarbons formed by a triple-ring structure of two benzene rings interconnected by a third oxygenated ring. Depending on their structural configuration, especially depending on the positions where chlorine atoms may be attached, different forms and congeners of these compounds can be formed. Theoretically, 75 PCDD and 135 PCDF congeners are possible, with their physical and chemical properties determined by the number of chlorine atoms and their respective positions regarding the molecular nucleus.
Globally speaking, PCDDs and PCDFs (PCDD/Fs) represent a class of toxic semivolatile aromatic compounds where the toxicology is prominently controlled by the presence of chlorine atoms in the 2, 3, 7, and 8 positions of the different congeners. There are 7 PCDDs and 10 PCDFs with this pattern, with the compound 2,3,7,8-tetrachlorodibenzo-p-dioxin (TCDD) the most important congener of all the dioxin-like class, with a toxic equivalency factor (TEF) of 1.0 (Shields et al., Citation2010), and classified as a group 1 carcinogen by the International Agency for Research on Cancer (IARC), although some authors do not support the same classification (Cole at al., Citation2003; Wielgosinski and Targaszewska, Citation2014), considering that it has a lower toxicity potential. Even though dioxins are emitted in relatively low concentrations, they are very persistent and bioaccumulative compounds and more prevalent in body fat of animals that later can be ingested by humans. The main risk of dioxins for human health is that they can alter the development of many cells and can be the cause of illnesses like cancer, disruption of the endocrine system, or reproductive and development problems (Mandal, Citation2005; Srogi, Citation2008; Booth et al., Citation2013). Their toxicity can be expressed in different forms (N-TEQ, I-TEQ, and WHO-TEQ) depending on the baseline TEF used for their estimation. However, differences between estimations with each unit are not supposed to be higher than 28% (Ren and Zheng, Citation2009).
The main source of dioxin and dioxin-like products formation is anthropogenic. There is also evidence of formation in nature, but the dominant sources proceed from human interaction, especially combustion-related processes. PCDD/Fs formation is driven by reactions at high temperature between organic compounds and chlorine. Therefore, activities such as waste incineration, metal smelting, forest fires, and diesel fuel combustion are the perfect breeding environment for the formation of the different dioxin-like congeners, since chlorine and volatile organic compounds are unavoidable from the feed materials of these processes (Kulkarni et al., Citation2008).
The principal mechanism in the formation of PCDD/Fs is known as “de novo synthesis.” Basically, de novo synthesis reaction is the oxidative breakdown and transformation of macromolecular carbon structures to aromatic compounds (Addink and Olie, Citation1995; Buekens et al., Citation2001). One part of these PCDD/Fs from de novo synthesis will remain in the solid phase and the other part will desorb to the gas phase and be carried by off-gas flow (Buekens et al., Citation2001).
Dioxin concentration levels in the atmosphere have changed within the last decades. Since PCDD/Fs formation is mainly influenced by anthropogenic sources, dioxin concentrations in the environment were not significant until the development of the chemical industry in the 1920s. From that time until the peak in the 1970s (Hites, Citation2010), dioxin emission and concentration levels in ambient air, soils, and water were amply increased, mostly due to a general ignorance of the negative effect of these compounds in human health. During those years and because of the lack of control and emergency procedures in industrial plants of the time, there were also important accidents with catastrophic environmental effects in different parts of the world, as in Seveso, Italy. PCDD/Fs like TCDD were even present during that time in armed conflicts, like Agent Orange gas in the Vietnam War (Hites, Citation2010). But the tendency changed from the 1970s to our days; increased social awareness, more efficient industrial procedures, and stricter legislation have caused a reduction in the emission and contamination levels of PCDD/Fs proceeding from industrial sources (Hites, Citation2010). On the other hand, emissions from nonindustrial sources have remained more or less constant in the last years and nowadays are one of the main focuses in dioxins proliferation (Quass et al., Citation2004; Cao et al., Citation2013).
Different reviews have been published in the last years related to dioxin characterization. Some of them have focused on the definition and explanation of what dioxins exactly are and what their physical and chemical properties are. Proliferation of dioxins and observation of their effects are relatively recent; that is why different approaches have been done in these overview documents. For example, Mandal focused on the effects dioxins have on human health (Mandal, Citation2005); Hays explained what the main routes for human exposure are (Hays and Aylward, Citation2003). Another approach was performed by Hites, who collected the main punctual historic events over the last years (Hites, Citation2010). Finally, there is another observed possibility that consists in focusing on how to determine dioxins and what their typical background levels are in the atmosphere (Shields et al., Citation2010).
Measurements of ambient concentrations have also been reviewed by several authors, performing studies where results are shown in a global scale (Booth et al., Citation2013), or differentiating between sample techniques, rural and urban locations, and ambient transformations in the dioxins that might take place during their dispersion (Lohmann and Jones, Citation1998; Jones and De Voogt, Citation1999). These authors gave a proper representation of dioxin concentrations around the world, but failed to link these levels to the different sources that produce dioxins.
Other authors have represented the typical concentrations and fingerprints from different sources. This type of studies can go from a brief representation of the congener profile from different thermal sources (Buekens et al., Citation2000), to deeper analysis where there is also depiction of the formation mechanisms that take place in these sources and the influencing variables during the composition of the fingerprints (Everaert and Baeyens, Citation2002), or the representation at the same time of typical fingerprints and quantitative values for the emission factors from different sources (Lin et al., Citation2006). These studies have something in common: They are mostly focused on industrial sources and do not have in consideration the ambient concentrations influenced by those emissions.
Other important variables have been taken under consideration by reflecting relevant information, like the most common abatement technologies of dioxins (Kulkarni et al., Citation2008), or dissecting the situation in specific places, focusing in the main sources and ambient concentrations for a particular geographical area, like the air emissions in China (Liu et al., Citation2013), or the releases to land and water in the United Kingdom (Dyke et al., Citation1997), or the ambient concentration in oceans (Morales et al., Citation2014). The principal inconvenience of these studies is that they offer extremely localized observations; thus, it is impossible to obtain a global assessment of the current situation.
One punctual observation is that most of these reviews do not usually offer a general representation of the global picture, and when they do, their agenda is different from the settled objectives for this document. For example, there are papers that offer a complete vision of dioxins concentration and their evolution over time, but focusing on how they behave later in the atmosphere and with little information about the main sources (Jones and De Voogt, Citation1999). In the same line, other studies can be found in the bibliography which main objective is to represent the effects that the main sources of dioxins produce in the environment, but under the perspective of observing the mechanisms of absorption phenomena that might take place and suppose a risk for human health (Srogi, Citation2008), or how economical situations influence in the total production of dioxins around the world and how the lower the gross national income per capita, the higher is the share from non-industrial sources (Cao et al., Citation2013).
As it have been seen, it is odd to find a review that all together, shows a worldwide study that includes ambient concentrations, emission characteristics from the main sources, and description of the minimization technologies employed, in the same document. Besides that, only few studies fall upon the importance that non-industrial sources have nowadays and how they ought to be considered in the immediate future (Fiedler, Citation2007; Shih et al., Citation2008; Wielgosinski, Citation2011; Kaivojosa et al., Citation2012; Cao et al., Citation2013). The “European dioxin air emission inventory project” (Quass et al., Citation2004) is another exception that reached the same conclusion. However, that document differs from the present review in two principal aspects; in the first place, in the European project, the evolution of concentration is given in absolute measures without a description of the distinctive features of the fingerprints from the different sources, which is, in fact, included in the present review. Secondly, the geographical frame for both documents is different.
The next sections of this document give a general assessment of the current situation of dioxins, first analyzing the ambient concentrations around the world, and then focusing on the different fingerprints that are produced by different sources, both industrial and nonindustrial. Finally, the main mechanisms that exist to control and minimize these emissions are described.
State of Dioxins in the World
A global perspective of dioxins concentrations around the world in air, soil, and water is given in the following paragraphs. Second, it goes into detail with more specific values per continents, and some countries are also depicted. It is shown that the most polluted areas in the world are eastern North America, Europe, and South and East Asia. Finally, a global conclusion describes the decreasing tendency of ambient concentrations over the last years.
Worldwide concentration of dioxins
Sources of dioxins are mainly anthropogenic, so industrial processes where combustion activities are an important part of the production are big contributors to dioxin emissions. The observed tendency is that countries with higher industrial and economic activity present higher emissions of dioxins (countries belonging to the G20 account for more than 80% of the estimated annual emissions) (Booth et al., Citation2013). On the other hand, among the most active nonindustrial sources, residential heating and residential waste combustion are the most relevant. Therefore, countries where these practices are more entrenched and more common will also contribute as main sources of the world emission of dioxins (Booth et al., Citation2013).
Quantitatively speaking, the annual global dioxin production is of 17,226 kg, which corresponds to approximately 287 kg-TEQ. From the total annual emission rate of dioxins into the atmosphere, only 9 kg-TEQ (3%) remains in the air. For the rest, 57% is deposited to land areas (163 kg-TEQ), while the remainder (40%), is absorbed by ocean waters (115 kg-TEQ or 354 kg/year) (Booth et al., Citation2013; Morales et al., Citation2014).
Referring to the PCDD/F fraction that is deposited or absorbed in soils and waters, the main sources are fuel combustion, metal production, pesticides production and use, waste incineration, sewage disposal, accidental fires, disposal in landfills, combustion processes, and the runoff of herbicides in agricultural usage (Dyke et al., Citation1997; Yao et al., Citation2002). In these cases, lowest concentration levels are found in the Antarctic and Oceania, while Europe presents the highest concentrations in soils. On the other hand, the north of the Pacific Ocean, the High Seas, and the Mediterranean Sea show the highest levels of dioxins in water (Booth et al., Citation2013).
Monitoring of ambient air concentrations is then an important source of information in order to measure the evolution of these pollutants, depending on the location, meteorology variables (Gunes and Saral, Citation2014), mechanisms of transportation, deposition and dispersion (Castro Jimenez et al., Citation2011), or even seasonal parameters; it has been probed that the concentration of dioxins in ambient air is higher during winter months (Ding et al., Citation2013; Gunes and Saral, Citation2014; Wcgiel et al., Citation2014). Other important meteorological parameters that interact with dioxin concentrations are ultraviolet (UV), solar radiation, and precipitation.
Another reason for the need for these monitoring networks is the possibility of comparing the evolution of expected values, or contrasting ambient concentrations in different sites where similar activities are taken place (Schuhmacher et al., Citation1997; Onofrio et al., Citation2011).
In the following sections, a separation in continents is made, and their characteristic ambient concentrations are depicted depending on the region, sampling sites, and period of measurement.
Dioxins in Europe
The continent of Europe is one of the main producers of dioxins worldwide. The emissions proceed both from industrial activities and nonindustrial burning processes, and the ambient concentration vary depending on the climate, residential activities, and degree of development and social awareness of the habitants from the respective countries. represents some gathered data by different reviews, where different ambient concentrations in Europe are shown (expressed in I-TEQ fg/m3), depending on the source, location type of the source, and time of measurement.
Table 1. Ambient air concentrations in Europe: (A) Lohmann and Jones (Citation1998); (B) Mari et al. (Citation2008); (C) Gunes and Saral (Citation2014)
It can be seen in that industrial locations have the highest peaks of concentrations, followed by urban, rural, and remote sites, although this does not imply that industrial areas have implicit dangerous levels of air concentrations, if proper measures are established (Abad et al., Citation2007; Li et al., Citation2010). Although only refers to air concentrations, the same differences between different location areas can be extended to soils pollution, with higher concentrations for urban samples than for rural ones (Creaser et al., Citation1990; Urban et al., Citation2014).
As a particular example of the evolution of dioxins in Europe, the Baltic Marine Environment Protection Commission (HELCOM) country members have controlled the PCDD/Fs emission to air as totals in tonnes per year for the period 1990–2012. Main conclusions from that study are (Gusev, Citation2014):
Heavily industrialized countries like Germany were the main producers of dioxins until a few years ago (1990s), when stricter legislation and the implementation of more efficient treatment mechanisms of flue gas led to the decrease of emissions to minimal values. Other industrialized countries like Poland, where less strict legislation was applied, have not showed the same behavior.
The most common tendency is that countries have decreased their emission levels over the last years, with exceptions in countries like Lithuania, where the emissions from nonindustrial burning processes are the major contributors, and added to the lack of social awareness of people have as consequence a constant (or even increasing) production rate over the last years.
Countries like Finland represent perfectly the tendency that sooner or later, industrial emissions are severely decreased when legislation and better technologies are implemented, leaving as one of the major pollutants the sources from nonindustrial operations, like residential heating.
The higher is the influence of industrial sources in a particular country, the higher are the levels of contamination achieved. On the other hand, in countries where nonindustrial processes are more extensive, emission levels are lower than in industrialized countries, but they do not show the same reduction over time, due mainly to a lack of social awareness and difficulties in controlling these emissions.
Once industrial emissions have been reduced, the weight of nonindustrial emissions will rapidly increase. An example of that can be seen in a study from Belgium, where the emission of dioxins from combustion of household waste was found to be 36 times higher than the emission limit value (0.1 ng TEQ/m3) for modern municipal waste incinerators (Wevers et al., Citation2004).
Dioxins in Asia
As happens for European countries, combustion activities, metal industries, and waste incineration are the main sources of dioxin emissions in Asia, where concentrations can differ depending on the activity itself, legislation for the area, or social awareness of the population. shows some typical ambient air concentrations (in I-TEQ fg/m3) gathered by different studies in Asia, depending on the location site and the period of measurement.
Table 2. Ambient concentrations in Asia: (A) Gunes and Saral (Citation2014); (B) Ding et al. (Citation2013); (C) Lohmann and Jones (Citation1998); (D) Lee et al. (Citation2004)
Measurements in Taiwan from serve as example of the typical behavior, with industrial locations having the highest ambient concentrations in air, and background or remote sites the lowest.
The main differences between Asian and European emissions are given not only for cultural reasons, but also for natural resources availability. Different raw materials and different compositions of them can be used in combustion processes that differ from the ones employed in Europe; thus, different emissions can be expected from the same industrial processes. For example, in China, paper mills do not usually use wood or recycled waste paper for fiber, as done in Europe. In this particular country, due to geographical and availability reasons, non-wood plant fibers such as cereal, rice, and reeds are extensively used as raw material for paper production. Thus, dioxin emissions from Chinese paper mills have a different congener composition than for other countries (Zheng et al., Citation2001). Another example can be found in the open burning of biomass, where rice straw is one of the most important feeding materials, given the importance of this crop in Asian countries (Shih et al., Citation2008; Zhang et al., Citation2008).
With China being one of the greatest producers of dioxins in the Asian continent, the highest contributor to dioxin emissions in this country are the ferrous and nonferrous metal production (46%), followed by heat and power generation (18%) and waste incineration (17%) (Liu et al., Citation2013). Other industrialized countries like Japan, Korea, and Taiwan have the same characteristic issues. For other countries with a lower degree of technological and industrial development, again there are the nonindustrial sources from uncontrolled events, as the most representative sources of dioxins from those areas (Chang et al., Citation2003; Shih et al., Citation2008; Huang et al., Citation2011; Shin et al., Citation2011).
Finally, concentrations of dioxins in Asia are also influenced by a specific event from the past, whose consequences are still noticeable today: The use of Agent Orange during Vietnam War in the 1960s–1970s caused residual TCDD to be still present in low concentrations in this country, similar to suburban areas in an industrialized country like Japan (Kishida et al., Citation2010).
Dioxins in America
America shows results similar to Europe, where the dioxin emission rates are typical for processes developed in each zone (industrial activities in urban and uncontrolled combustion in rural zones). Measured concentrations in ambient air from remote or urban areas have generally similar fingerprint profiles, but the volumes of concentration levels are lower in remote areas where the only deposition of dioxins take place by transportation and transformation mechanisms from the producer sources in urban areas. summarizes (in I-TEQ pg/m3) the work by different authors of relevant information about ambient air concentrations in America, depending on the period of sampling and location site.
Table 3. Ambient air concentrations in America: (A) Lohmann and Jones (Citation1998); (B) Gunes and Saral (Citation2014); (C) Mari et al. (Citation2008); (D) Wohrnschimmel and Yao (Citation2014)
With the United States being the highest generator of dioxins in America, and according to the study of the National Dioxin Air Monitoring Network (NDAMN), which measured the distribution of atmospheric pollution from 1999 to 2004 in the United States, higher ambient concentrations in air can be seen in the east of the country (Cleverly et al., Citation2007; Zhang et al., Citation2009).
Dioxins in Africa and Oceania
The existence of different dispersion and transportation mechanisms allows dioxin proliferation by secondary emission: emission of dioxins that are present in the environment not as a direct effluent of flue gas from a near industrialized area, but as the result of different transformations and transportation phenomena of dioxins generated from distant activities. Africa is one of the continents more influenced by this type of contamination, which alters the relationship of the ratio of dioxin accumulation with the ratio of dioxin production per country. Evidence of this tendency is given by the fact that 11 countries from Africa are placed in the top 20 most impacted countries when comparing the ambient concentration with the ratio of production (Booth et al., Citation2013).
This behavior can be found in countries from the north of Africa like Egypt, where besides their own attributable production of dioxins, they receive pollutants from Europe according to the different transportation and dispersion mechanisms of PCDD/Fs. Typical ambient air concentrations in African countries can be found between 18 and 532 fg WHO-TEQ/m3 (mean value of 51 fg WHO-TEQ/m3) for urban sites (Bogdal et al., Citation2013). More concrete results for industrial areas have shown concentrations between 210 and 780 fg I-TEQ/m3 in Algeria, although these are expected to be even higher in countries like Egypt (~1000 fg I-TEQ/m3; Moussaoui et al., Citation2012). Africa shows the same behavior where industrial locations show higher ambient concentrations than urban and rural ones (Quinn et al., Citation2009; Ssebugere et al., Citation2013).
On the other hand, the Pacific islands have shown lower concentrations, as could be expected, with a mean value of 3 fg WHO-TEQ/m3 (1-87 WHO-TEQ/m3) (Bogdal et al., Citation2013). The most likely sources of dioxins in these developing countries are nonindustrial activities like open waste burning, combustion of biomass, forest fuel, and open dumping sites (Bogdal et al., Citation2013).
Finally, it is important to mention that ambient air concentrations in Polar regions show the lowest values and are estimated to be one-tenth of the ambient concentration in the United Kingdom (Lohmann and Jones, Citation1998).
Global conclusion
Despite the disparity of data detected in the literature, which other authors have recognized as well (Schatowitz et al., Citation1994; Breivik et al., Citation2006; Urban et al., Citation2014), and the way that different location sites, different units, different periods of monitoring, seasonal and meteorological variables, different monitoring techniques, or even different estimation tools (Pulles et al., Citation2006; Booth et al., Citation2013), cause great difficulties in establishing a uniform comparison of the concentration of dioxins on a worldwide level, a decreasing tendency can be observed over time, after the peak in the 1970s–1980s. First European and American countries and later Asian regions have decreased their emissions, mainly by the application of technological measures in industrial sources, while nonindustrial sources have remained more or less constant over time (Alcock and Jones, Citation1996; Pacyna et al., Citation2003; Quass et al., Citation2004; Breivik et al., Citation2006; Fiedler, Citation2007; Ren and Zheng, Citation2009; Nzihou et al., Citation2012; Cao et al., Citation2013). In consequence, ambient concentrations have also decreased over the last years (Shin et al., Citation2011; Assefa et al., Citation2014), as can be seen in , where the results from different monitoring studies are presented, including Asian countries like Japan (United Nations Environmental Programme [UNEP], Citation2015), South Korea (Shin et al., Citation2011), and Taiwan (Zheng et al., Citation2008), and European countries like Spain (Abad et al., Citation2007) and the United Kingdom (Coleman et al., Citation1997; Katsoyiannis et al., Citation2010).
Figure 1. Evolution of ambient air concentration in different regions, 1991–2012 (Shin et al., Citation2011; Katsoyiannis et al., Citation2010; Abad et al., Citation2007; Zheng et al., Citation2008; Coleman et al., Citation1997; UNEP, Citation2015).
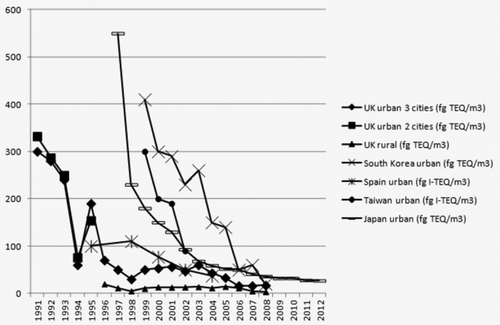
As consequence, nowadays a weak degree of influence from a direct and particular source can be related to a certain level of pollution in ambient air (Onofrio et al., Citation2011; Wcgiel et al., Citation2014), especially in urban environments. Although exceptions of preponderant influence from one source can be found (Shih et al., Citation2008), it is more common that ambient concentrations in air, soils, and water are a mixing result of different sources, where of course some may have more influence than others, but dioxin levels near an efficient industrial plant cannot be entirely related to this activity. Traffic, other processes, and deposition mechanisms from different sources contribute to the final concentration, thus reducing the gap between urban and rural ambient concentrations (Katsoyiannis et al., Citation2010; Huang et al., Citation2011; Vassura et al., Citation2011; Gworek et al., Citation2013; Wohrnschimmel and Yao, Citation2014).
Fingerprint of Dioxins by Industrial and Non-Industrial Sources
Dioxins that proceed from anthropogenic sources have different congener profiles depending on the source and the process itself inside each source. In consequence, these compounds are released to the environment in a variety of ways and in varying quantities, depending upon the influence of each industrial or non-industrial activity.
As was said before, previous authors have tracked the evolution of the emission levels from different sources of dioxin, where it can be seen that the share of nonindustrial producers has gained importance since emissions from industrial sources have been reduced, as shown in , where results from the European inventory of dioxins (expressed in g I-TEQ/year) are shown (Quass et al., Citation2004).
Table 4. Evolution of dioxin sources in Europe in g/ I-TEQ year (Quass et al., Citation2004)
Emission values can be expressed in different units. It is our opinion that inventories that express their releases in the form of emission factors (EF) are more concrete and give better knowledge from particular sources than expressions like grams per /year from other inventories (Pacyna et al., Citation2003; Chen, Citation2004; Quass et al., Citation2004; Pulles et al., Citation2006; Van der Gon et al., Citation2007; Wielgosinski, Citation2011; Cao et al., Citation2013; Relvas et al., Citation2013), where a value of the total raw emission is given, without considering the actual emission rate from each of the estimated sources, which can be influenced by feed materials, abatement technologies, economical reasons, geography, and so on (Chen, Citation2004; Pulles et al., Citation2006; Chiu et al., Citation2011; Trivedi and Majumbdar, Citation2013).
However, the production of dioxins expressed as emission factors (EF) also needs to be complemented with data from the process, like the feed flow from the plant, fuel consumption, or the definition of the raw materials and process stages. Nevertheless, in order to simplify the provided information and ease their comparison and interpretation, this document joins EFs by sector (main industrial and nonindustrial sources), region (continent), and period (decade), presuming that processes in the same regions during the same period of time have the same operational technologies.
Fingerprint of industrial sources
Waste combustion and metal production are the main producers of dioxins in industrial environments. Other relevant processes are paper mills, cement kilns, coal power plants, wastewater treatment facilities, and crematory incinerators. Each one of these processes has its own particular emission factors and fingerprints, where different congeners are emitted in different concentrations.
Waste combustion
It is important to differentiate between operations like municipal solid waste incinerators (MSWI), medical waste incinerators (MWI), industrial waste incinerators (IWI), and hazardous waste incinerators (HWI). Each one of these combustion activities has different raw materials and performs at different conditions; hence the fingerprint of these activities can differ.
For waste incineration, the most relevant concentration occurs in the fly ashes (as high as 858 ng I-TEQ/kg), which makes fundamental the installation of proper abatement and disposal technologies for the dust fraction (Lin et al., Citation2010; Zhang et al., Citation2012; Zhong et al., Citation2014). Considering the air emissions to the atmosphere, represents different emission factors for MSWI, gathered by different authors.
Table 5. Emission factors from MSWI: (A) Huang and Buekens (Citation1995); (B) Eduljee and Dyke (Citation1996); (C) Alcock et al. (Citation1998); (D) Nzihou et al. (Citation2012); (E) Pacyna et al. (Citation2003); (F) Caserini and Monguzzi (Citation2002); (G) Lee et al. (Citation2003); (H) Lin et al. (Citation2007); (I) Wang et al. (Citation2005); (J) Du et al. (Citation2010); (K) Lin et al. (Citation2010); (L) Liu et al. (Citation2013); (M) Zhang et al. (Citation2012); (N) Zhong et al. (Citation2014)
Fingerprints from the same processes under the same operational conditions generally have the same congener distribution. In fact, there are studies that have concluded that there is no clear indication that the waste composition has a significant effect on the air emission factors (Zhang et al., Citation2011).
Typical congener profiles from MSWI show high concentrations of OCDD, 1,2,3,4,6,7,8-HpCDD, and 1,2,3,4,6,7,8-HpCDF (Lee et al., Citation2003; Wang et al., Citation2003a; Wang et al., Citation2005; Du et al., Citation2010; Lin et al., Citation2010). Other important contributors to the resultant emissions are 2,3,4,7,8-PeCDF (Zhang et al., Citation2012; Zhong et al., Citation2014) and 2,3,4,7,8-HxCDF (Everaert and Baeyens, Citation2002; Zhong et al., Citation2014); 2,3,7,8-TCDD is shown to have one of the lowest concentrations of the fingerprint (Zhang et al., Citation2012; Everaert and Baeyens, Citation2002; Wang et al., Citation2003; Lin et al., Citation2010).
However, when a congener profile is depicted, it is important to differ between simple concentration or I-TEQ concentration because congener distribution will differ. For example, considering a study from MSWI in China, 2,3,4,7,8-PeCDF is the second congener with the highest presence in the fingerprint (after OCDD). On the other hand, when considering the toxic equivalence of the congeners, this compound is by far the one with the highest concentration in all the profile, while OCDD is barely noticeable (Zhang et al., Citation2012).
These congeners contribute to the global TEQ to reach emission levels that, added to the higher number of installed and operative MSWI compared with the rest of waste combustors, situate emission from MSWI as the most important ones in absolute values in contrast with the rest of waste combustion processes, even though emission factors from MWI in small combustors are higher than those from MSWI (Lee et al., Citation2003), as it can be seen by comparing emission factors from (MSWI) and (MWI).
Table 6. Emission factors from MWI: (A) Lindner et al. (Citation1990); (B) Eduljee and Dyke (Citation1996); (C) Pacyna et al. (Citation2003); (D) Lee et al. (Citation2003); (E) Lin et al. (Citation2007); (F) Liu et al. (Citation2013); (G) Singh and Prakash (Citation2007); (H) Yan et al. (Citation2011)
The congener profiles from MWI, HWI, and IWI are similar to those seen for MSWI, with greater presence of OCDD, 1,2,3,4,6,7,8-HpCDF (Lindner et al., Citation1990; Lee et al., Citation2003; Wang et al., Citation2003a; Du et al., Citation2010; Wang et al., Citation2014; Wcgiel et al., Citation2014), 2,3,4,7,8-PeCDF (Lee et al., Citation2003; Khumsaeng et al., Citation2013; Wang et al., Citation2014), and 1,2,3,4,7,8-PeCDD (Khumsaeng et al., Citation2013; Wang et al., Citation2014). Finally, shows typical emission factors of IWI and HWI gathered by the literature.
Table 7. Emission factors from HWI and IWI: (A) Huang and Buekens (Citation1995); (B) Schuhmacher et al. (Citation1998); (C) Eduljee and Dyke (Citation1996); (D) Du et al. (Citation2010); (E) Liu et al. (Citation2013); (F) Lin et al. (Citation2006); (G) Wang et al. (Citation2014); (H) Khumsaeng et al. (Citation2013)
Metal production
In this subsection, zinc, lead, copper, aluminum, and steel production emissions are depicted. The first four elements are compared together in the same analysis. Second, and considering that the iron and steel industry is one of the most important sources of dioxins (Quass et al., Citation2004), the analysis of its emissions is included separately to differ between two sensitive stages in the process: coke making and sintering.
The four metals in this group are studied differently depending on the reference; sometimes their emissions are included under the same category of nonferrous metallurgy (Relvas et al., Citation2013; Eduljee and Dyke, Citation1996), and at other times they are studied independently, considering different stages of the process or different conditions (EPA, Citation2006; Caserini and Monguzzi, Citation2002; Srogi, Citation2008; Lin et al., Citation2006; Lin et al., Citation2007; Lee et al., Citation2005a; Chiu et al., Citation2011; Zou et al., Citation2012). represents studies where all four metals were analyzed in the same document, so comparisons can be established.
Table 8. Emission factors from metal industry (A) Pacyna et al. (Citation2003); (B) Holtzer et al. (Citation2007); (C) Ba et al. (Citation2009a,Citationb); (D) Du et al. (Citation2010); (E) Liu et al. (Citation2013)
Observing the previous table, it can be said that zinc emissions are generally the highest in both volume and toxicity, followed by copper, aluminum, and lead. Fingerprint profiles have similar main congeners; for example (Ba et al., Citation2009a; Ba et al., Citation2009b), the most abundant congener in the representative TEQ profile from these sources is generally 2,3,4,7,8-PeCDF for zinc metallurgies (36.1%), copper metallurgies (27.9%) and aluminum metallurgies (29.4%). On the other hand, for lead metallurgies, 2,3,4,7,8-PeCDF presents a concentration in stack gas of 16.3%, with 1,2,3,4,6,7,8-HpCDF being the highest contributor in the TEQ profile (20.2%). In the case of 2,3,7,8-TCDD, one of the most dangerous congeners in the mixture, the highest concentrations in stack gas are found in zinc metallurgies (6.1%), being lower for the other three industries. Similar profiles were observed in other studies, where most relevant congeners were also 2,3,7,8-TeCDF (Lee et al., Citation2005a; Lin et al., Citation2006; Chiu el al., Citation2011; Zou et al., Citation2012; Buekens et al., Citation2000) or OCDF (Buekens et al., Citation2000; Du et al., Citation2010; Zou et al., Citation2012).
As was said before, given the relevance of iron and steel industry, the analysis of its emissions is included now separately to differ between two sensitive stages in the process: coke making and sintering, where sintering is the activity with highest emission of PCDD/Fs (Aries et al., Citation2006; Liu et al., Citation2009). represents some characteristic emission factors from both sources.
Table 9. Emission factors from sinter and coking plants: (A) Anderson and Fisher (Citation2002): (B) Eduljee and Dyke (Citation1996); (C) Pacyna et al. (Citation2003); (D) Alcock et al. (Citation1998); (E) EPA (Citation2006); (F) Aries et al. (Citation2006); (G) Holtzer et al. (Citation2007); (H) Srogi (Citation2008); (I) Esposito et al. (Citation2014); (J) Lin et al. (Citation2007); (K) Wang et al. (Citation2003); (L) Du et al. (Citation2010); (M) Wang et al. (Citation2010); (N) Zou et al. (Citation2012); (O) Liu et al. (Citation2009)
The literature also showed that several congeners, comprising 1,2,3,4,6,7,8-HpCDF, 2,3,4,7,8-PeCDF, 2,3,7,8-TCDF, and OCDF, are generally the dominant species in the fingerprint profile from sinter plants (Anderson and Fisher, Citation2002; Aries et al., Citation2006; Wang et al., Citation2003; Du et al., Citation2010; Zou et al., Citation2012). Taking under consideration the contribution of 2,3,7,8-TCDD, the concentration of this congener is one of the lowest in all the mixture, near the nondetection limit (Aries et al., Citation2006; Liu et al., Citation2009). In the case of coking plants, OCDD, 1,2,3,4,6,7,8-HpCDF, and OCDF are characterized to be the most frequent congeners (Liu et al., Citation2009). However, considering the influence of I-TEQ concentrations, the most relevant congeners are 2,3,4,7,8-PeCDF, 2,3,4,6,7,8-HxCDF, 2,3,7,8-TCDF, and 1,2,3,4,7,8-HxCDF (Anderson and Fisher, Citation2002; Aries et al., Citation2006).
Other industrial sources
Wastewater treatment can be another source of dioxins. Analyzing the fingerprint profile of wastewaters, OCDD/Fs are the most significant congeners, followed by Hp-CDD/F, and with minimal values for the most toxic compound, 2,3,7,8-TCDD (Dai et al., Citation2007). Wastewater from industrial or urban sewage requires the application of certain treatments before their disposal. One of the consequences of this wastewater treatment is the formation of sludge that will also need to be treated before discarding and that shows fingerprint profiles similar to those of the wastewater (with OCDD, Hp-CDD/F, and TeCDF being the most relevant congeners), and typical concentrations between 3.47 and 88.24 ng I-TEQ/kg dry weight (Dai et al., Citation2007; Clarke et al., Citation2008; Wcgiel et al., Citation2014).
Depending on the presence and concentrations of dioxins in the sludge, two treatment possibilities are discussed for the disposal of this sludge: sludge incineration and the use of sludge in agriculture. On the one hand, different analysis of the contents of dioxins and furans in the incineration of sewage sludge samples indicated that the content of dioxins and furans can vary from 9.0 to 930 ng I-TEQ/kg dry matter (Eduljee and Dyke, Citation1996; Alcock et al., Citation1998; Werther and Ogada, Citation1999). On the other hand, when using sludge in agriculture, if the concentration of dioxins is high, given the persistence property of these compounds, an agricultural use would end in the accumulation of dioxins in the soils. Nevertheless, different studies estimate that in most cases, the PCDD/F input to soils through agricultural use is lower than the input that already takes place through atmospheric deposition (McLachlan et al., Citation1996; Clarke et al., Citation2008).
Pulp and paper mills are another relevant source of dioxins. The higher I-TEQ dioxin emissions during the production of paper are produced in the bleaching step, especially in the wastewater from the process, so proper treatment of wastewater is demanded. For a developed country, emission factors for pulp production have a mean value of 0.068 ng I-TEQ/kg (EPA, Citation2006; Relvas et al., Citation2013). On the other hand, the concentrations of dioxins in bleached pulps in China range from 2.77 to 31.47 ng I-TEQ/kg dry pulp (Wang et al., Citation2012). Therefore, this is the critical stage in the formation of dioxins, where changes in the process itself or in the use of different raw materials can produce differences in the characteristic fingerprint of this activity. For example, congener profiles of a pulp mill in China are found with a bigger presence of OCDD and 1,2,3,7,8-PCDD (Zheng et al., Citation2001).
Production of cement is another known source of dioxins, but the amount and pattern of these emissions can vary widely depending on the design and operating conditions of the kiln and the fuels and raw materials fed into it. For example, the consumption of used tires as feeding material produced the highest I-TEQ levels compared with other secondary fuels. In addition, the only quantifiable concentrations of 2,3,7,8-TCDD congener were found for used tires as feeding material. In consequence, from a dioxin proliferation perspective, used tires are the least recommended material to employ as secondary fuel (Abad et al., Citation2004). On the opposite side, distinguishing between the most employed primary fuels (coke or coal), emission factors and fingerprint profiles do not offer great differences in their distribution, which generally shows a more pronounced enrichment in 2,3,7,8-TCDF, with minimal emissions of 2,3,7,8-TCDD (Ames et al., Citation2012; Rivera-Austrui et al., Citation2014). Other common relevant congeners are OCDD, OCDF, 1,2,3,4,6,7,8-HpCDF, 2,3,4,7,8-PeCDF, 1,2,3,4,6,7,8-HpCDF, or 1,2,3,7,8-PeCDF (Abad et al., Citation2004; Lin et al., Citation2006; Ames et al., Citation2012; Rivera-Austrui et al., Citation2014; Li et al., Citation2015). Referring to emission factors, shows the estimations in different studies.
Table 10. Emission factors from cement production: (A) Caserini and Monguizzi (Citation2002); (B) Eduljee and Dyke (Citation1996); (C) Alcock et al. (Citation1998); (D) Pacyna et al. (Citation2003); (E) Relvas et al. (Citation2013); (F) Abad et al. (Citation2004); (G) Rivera-Austrui et al. (Citation2014); (H) Lin et al. (Citation2007); (I) Li et al. (Citation2015)
Coal power and industrial plants must be considered as well. Flue gases from coal power plants are characterized by low levels of dioxin concentrations, as shown in . However, the large volumes of coal consumption and the consequent great dimension of flow gas emission that carries more than 99% of total I-TEQ emitted (Lin et al., Citation2010) make it necessary to take under consideration the emission from these sources, where OCDD, PeCDF, and HpCDF are the dominant congeners (Everaert and Baeyens, Citation2002; Lin et al., Citation2007; Lin et al., Citation2010) in the fingerprint. Also, the fraction of 2,3,7,8-TCDD is lower than 5% (Lin et al., Citation2007).
Table 11. Emission factors from coal plants: (A) Eduljee and Dyke (Citation1996); (B) Pacyna et al. (Citation2003); (C) EPA (Citation2006); (D) Wielgosinski (Citation2011); (E) Lin et al. (Citation2007); (F) Lin et al. (Citation2010)
One last example of relevant industrial sources is crematory incineration. Different studies have observed the dioxin production from a crematory, like the ones shown in . In the fingerprint distribution from these studies, the most abundant congeners in a crematory incinerator are proven to be 1,2,3,4,6,7,8-HpCDD, 1,2,3,4,6,7,8-HpCDF, and OCDD, with a minimal contribution of 2,3,7,8-TCDD, with values almost in the limit of nonquantification (Du et al., Citation2010; Chiu et al., Citation2011).
Table 12. Emission factors from crematories: (A) Eduljee and Dyke (Citation1996); (B) Mari and Domingo (Citation2010); (C) Caserini and Monguzzi (Citation2002); (D) Relvas et al. (Citation2013); (E) Lin et al. (Citation2007); (F) Singh and Prakash (Citation2007); (G) Du et al. (Citation2010); (H) Chiu et al. (Citation2011)
Fingerprint of nonindustrial sources
Dioxin emissions from nonindustrial sources are difficult to quantify and qualify because they can proceed from several diffuse focuses, where the combustion and generation process is generally uncontrolled. Therefore, the estimates of emission from these sources are usually characterized by highly uncertain data. Important contributors are accidental fires, diesel engines, release of PCDD/F from pentachlorophenol-treated wood products, and illegal incineration of household wastes (Quass et al., Citation2004).
Therefore, there is one obvious difference between industrial and nonindustrial sources: While industrial sources can be easily measured, and thus controls and legislation can be applied to impose emission limits, nonindustrial sources have bigger variability (Lee et al., Citation2005a) and the emission levels are mostly influenced by the degree of social awareness of the population. For that reason, the amounts of industrial emissions have decreased in the last years, while nonindustrial ones have remained constant, and in consequence, it is the nonindustrial sources that are gaining high importance nowadays when a global analysis of dioxins released in the atmosphere is done. The next subsections focus on significant nonindustrial sources like domestic wood combustion, diesel engines, forest fires, and domestic waste combustion.
Domestic wood and coal combustion
Domestic wood combustion generally shows higher emission factors than industrial wood combustion because of the presence of different conservation treatments, paints, binders, or fire retardants applied to wood, which act as the main source of PCDD/Fs during wood combustion (Tame et al., Citation2007). They are sources of uncertainty since in most of these combustion processes there is no control of the composition of the raw materials or the levels of dioxin formation and emission. However, certain patterns can be identified during these processes—for example, when the wood fuel exhibits notably higher chlorine content, higher levels of PCDD/F in the emissions can be expected in most cases, in fingerprint profiles dominated by HpCDD, HxCDF, TCDF, and HpCDF (Everaert and Baeyens, Citation2002; Tame et al., Citation2007; Kaivojosa et al., Citation2012). Last, the presence of 2,3,7,8-TCDD in the fingerprint of dioxins from wood combustion is characterized by a concentration that can be as high as 10% of the sum of all PCDD/Fs congeners for a typical wood combustion (Tame et al., Citation2007).
Although the values for PCDD/F emissions from domestic wood fires can span several orders of magnitude, the concentrations are found to be in the range shown in , depending on the wood is contaminated or not.
Table 13. Emission factors from domestics wood combustion: (A) Schatowitz et al. (Citation1994); (B) Huang and Buekens (Citation1995); (C) Eduljee and Dyke (Citation1996); (D) Pacyna et al. (Citation2003); (E) Caserini and Monguzzi (Citation2002); (F) Wielgosinski (Citation2011); (G) Lavric et al. (Citation2004); (H) Tame et al. (Citation2007); (I) Kaivojosa et al. (Citation2012)
On the other hand, coal can be burned as well in domestic environments. shows typical emission factors, depending on the class of coal, gathered by different authors.
Table 14. Emission factors from domestic coal combustion: (A) Caserini and Monguzzi (Citation2002); (B) Relvas et al. (Citation2013); (C) Eduljee and Dyke (Citation1996); (D) Pacyna et al. (Citation2003); (E) Lee et al. (Citation2005a); (F) Paradiz et al. (Citation2015)
Diesel vehicles
Personal and industrial use of diesel vehicles also has certain emissions of dioxins associated. Although they might not be higher, the extensive use of this transportation method around the world causes the need to consider its correspondent emission levels and dioxins fingerprint. There are different studies that have measured the dioxin emissions at the tailpipe, with typical values that are depicted in , considering type of vehicle and fuel. The fingerprint profiles typically show that diesel engines emit a large amount of high-chlorinated (hepta- and octa-)PCDD/Fs congeners, especially OCDD/F, OCDD, and 1,2,3,4,6,7,8-HpCDD/F. However, 2,3,4,7,8-PeCDF is the highest contributor to I-TEQ concentration. Finally, 2,3,7,8-TCDD emissions are not included along the most relevant in concentration value (Gullet and Ryan, Citation2002; Kim et al., Citation2003; Chuang et al., Citation2011; Chang et al., Citation2014).
Table 15. Emission factors from vehicles: (A) Huang et al. (Citation2011); (B) Srogi (Citation2008); (C) Eduljee and Dyke (Citation1996); (D) Pacyna et al. (Citation2003); (E) Gullet and Ryan (Citation2002); (F) Kim et al. (Citation2003); (G) Chuang et al. (Citation2011); (H) Chang et al. (Citation2014)
Open fires
This accidental source of PCDD/Fs can liberate high amounts of dioxins, with the aggravation that it is completely unpredictable and influenced by many variables like the material being burned or the type of fire; thus, there are very limited data to from which to generate emission factors. However, as represents, there are some authors who have dared to give an estimate emission factor for forest fires.
Table 16. Emission factors from forest fires: (A) Caserini and Monguzzi (Citation2002); (B) Eduljee and Dyke (Citation1996); (C) Relvas et al. (Citation2013); (D) Gullet et al. (Citation2008)
Domestic waste combustion
Open waste burning, backyard barrel burning, or biomass waste burning, when performed in a domestic environment, is another nonindustrial source of dioxins that must be considered, since its emission factors are already higher than those from modern industrial waste incinerators (Wevers et al., Citation2004; Wu et al., Citation2014). In fact, this is probably the most important nonindustrial source and one of the most difficult to determine precisely, given the variability in composition of waste and mostly uncontrolled combustion techniques (Lemieux et al., Citation2004; Solorzano-Ochoa et al., Citation2012). However, some authors have tried to unify and review documented emission factors depending on the composition of the waste (Lemieux et al., Citation2004). Some other values, signs of the variability of these sources depending on the raw material, are included in .
Table 17. Emission factors from domestic waste combustion: (A) Lemieux et al. (Citation2000); (B) Zhang et al. (Citation2011); (C) Gullet et al. (Citation2010); (D) Gullet et al. (Citation2001); (E) Lundin et al. (Citation2013); (F) Pacyna et al. (Citation2003); (G) Schatowitz et al. (Citation1994); (H) Relvas et al. (Citation2013); (I) Wevers et al. (Citation2004); (J) Hedman et al. (Citation2005); (K) Lin et al. (Citation2007); (L) Zhang et al. (Citation2008); (M) Black et al. (Citation2012)
Technologies to Control Dioxin Emissions
Different mechanisms can be used to control and reduce the formation of dioxins, and to avoid their release into the environment once they are formed. Two basic approaches can be taken:
The modification of the industrial activity that generates the dioxins toward more efficient mechanisms that minimize PCDD/Fs formation. These are primary methods that prevent the formation of contamination.
The addition of chemical agents that will react with the dioxins, capturing and removing them from the flue gas stream. These are secondary methods that limit the emission of contamination by means of their decomposition or adsorption. Those methods do not prevent the formation of dioxins in the source, but provide measures to limit their emission to the atmosphere.
Primary and secondary methods can be performed separately or in combination to enhance the reduction of dioxin emissions. Some possibilities to minimize this problematic situation are briefly described in the next paragraphs. Most common primary methods include mechanisms that alter operational variables (like temperature or recirculation mass flow), use of inhibitors and suppressors to restrain the formation of dioxins, or the application of changes in the operation design toward higher combustion efficiencies. On the other hand, most common secondary methods include treatments to the flue gas involving absorption/adsorption mechanisms to capture the pollutants, filtration and scrubbing operations to remove the dust particles, selective catalytic reduction to control dioxin concentration, or modern techniques involving ionization devices. In the secondary methods there are also included those treatments that must be performed to the captured fly ashes before their disposal.
Primary methods
PCDD/F formation may be reduced by lowering the O2 level (Buekens et al., Citation2001). This can be achieved by recirculating the off-gas and increasing the depth of the burden. The PCDD:PDCF ratio increases as O2 concentration increases. Obviously, the formation of PCDD, which contains two oxygen atoms, is stimulated by the presence of O2, compared with PCDF, which has only one oxygen atom (Addink and Olie, Citation1995).
Proper selection of raw materials, avoiding as possible the addition of chlorine into the process, is an effective method to reduce the formation of dioxins, although sometimes this operation is not possible (Wielgosinski et al., Citation2011). In relation to that, initial pretreatment of materials before combustion, especially in the case of waste incinerators, may help to minimize the posterior formation of PCDD/Fs (Zeng et al., Citation2011).
Another possibility is the use of active inhibitors as an effective way for dioxin formation reduction. Some inhibitors, such as triethanolamine, may reduce de novo synthesis mechanism by 50%. Other chemical agents, like ammonia, have been tested for possible inhibiting effects on PCDD/F formation but the results so far were not as effective, especially for large plants (Buekens et al., Citation2001; Wielgosinski, Citation2011; Lundin and Jansson, Citation2014; Lechtanska and Wielgosinski, Citation2014). On the other hand, the use of dioxin suppressors like SO2, CaO, or nitrogen compounds has been proven to have a large effect in suppressing the chlorination rate and blocking the metallic active centers in dust particles, which are some of the main mechanisms during dioxin formation (Altarawneh et al., Citation2009; Wielgosinski, Citation2011; Zeng et al., Citation2011; Bajamundi et al., Citation2014). This can be done by the addition of relatively high-sulfur coal as fuel for combustion, or by the introduction of quick lime (CaO) as an economical cohesive element.
Other technical measures that can be applied are the installation of modifications in the design of the furnace to enhance combustion efficiency and lower hydrocarbon and dust emissions (Buekens et al., Citation2001; Wielgosinski, Citation2011; Zeng et al., Citation2011).
One last way to minimize the formation of dioxins is the application, during the cooling stage, of an accelerated cooling of the off-gas in the temperature zone 600–200ºC, to prevent the regeneration of these compounds (UNEP, Citation2005).
Secondary methods
There are different measures that can be included as secondary methods to reduce the emission of dioxins. One of them is the recirculation of the off-gas to recycle a part of the waste gas to the sintering process. Nevertheless, some of the most common abatement mechanisms to treat dioxins from the off-gas stream consist of absorption/adsorption processes, by the injection of adsorbents/absorbents with high take-up capacities for dioxins (Wielgosinski, Citation2011; Zeng et al., Citation2011). There are several methods that can be employed: flow injection of finely grained coke stemming from coal; fluidized bed process with adsorbent recycling; fixed bed or moving bed processes (Kulkarni et al., Citation2008); entrained flow reactor (UNEP, Citation2005); adsorption using polymers like polypropylene, in where active carbon particles are dispersed (Lindgren and Andersson, Citation2008); adsorption on carbon nanotubes (Wielgosinski, Citation2011); and newer adsorption methods using ionic liquids (Pan et al., Citation2013).
Also, different particulate matter collection systems can be used to reduce dioxin emissions that are enriched in the surface of fine dust. Besides fabric filters, other common devices are cyclones, KN filters (Menad et al., Citation2006), and scrubbers or electrostatic precipitators (Kulkarni et al., Citation2008). In the same line of action, it is possible to use technical measures that are the combination of various methods, like the injection of activated carbon and use of fabric filtration in the end-of-pipe control (Buekens et al., Citation2001). Scrubbers can also be used to remove both acid gas and particulate matter from the off-gases. Some examples are spraying dry scrubbers, wet scrubbers, and fine dust absorbers (UNEP, Citation2005).
Moreover, extensive research and field measurements have indicated that commercial NOx control catalysts reduce also PCDD/F emission. Nitrogen oxide is controlled by selective catalytic reduction (SCR), using NH3. V2O5/TiO2-based catalysts are considered state of the art for this application (Wang et al., Citation2003; Wielgosinski, Citation2011).
Again, combination of methods can be performed, like the implementation of catalytic bag filters, where the membrane is impregnated with a catalyst, or contains a powered catalyst directly mixed in the fiber tissue (UNEP, Citation2005), or the application of the filtrating catalytic method Remedia, consisting of a combination of adsorption, dedusting, and catalytic decomposition techniques (Wielgosinski, Citation2011), or the combination of catalysis and activated carbon adsorption (Ji et al., Citation2014).
Finally, in recent years, newer methods are being tested to determine their efficiency in reducing the concentration of dioxins in the flue gas (Wielgosinski, Citation2011). Two of them are the pulse corona-induced plasma chemical process, where the gas passes through a reactor that oxidizes NO to NO2 and decomposes dioxins simultaneously (Yoshida et al., Citation2009); and the electron irradiation processes, where the degradation of gaseous dioxin agents is done by OH radicals formed under the action of ionizing radiation (Kulkarni et al., Citation2008).
In relation to the treatment of fly ashes, principal measures that can be applied before fly ash disposal include thermal treatments for stabilization of dioxins, nonthermal plasma for the destruction of dioxins in the fly ash, UV irradiation for photocatalytic degradation of dioxins, addition of chemical reagents for the decomposition of dioxins, submission to hydrothermal treatments to be recycled in the cement industry, and newer methods for the remediation of dioxins like the supercritical water oxidation (Kulkarni et al., Citation2008).
Conclusion
Dioxin and furans are organic compounds that proceed mostly from anthropogenic sources and can be dangerous to human health, especially the most toxic congeners like 2,3,7,8-TCDD. The effects of dioxins are more dangerous when they deposit and accumulate in fat tissues of animals that later can be ingested, affecting the proper growth of cells that might derive in illness like cancer or development effects, with children and infants being the most sensitive population group.
The annual global dioxin production of 17226 kg is equivalent to approximately 287 kg-TEQ, with most of it being deposited on land areas when compared with the deposition in ocean waters (Booth et al., Citation2013). Industrialized countries in North America, Europe, and South and East Asia are the most polluted but also the biggest producers of dioxins; however, transportation, dispersion and transformation mechanisms, climate and geographical influence, and specific events like accidents or wars, affecting other countries with lower production rates, have high ambient concentration of dioxins.
It is also important to observe the evolution of concentrations over time, and how the trend differs depending on the geographic area. It can be said that after a peak in the 1970s–1980s, industrial areas and sources have decreased their emissions after the implementation of policies of flue gas treatment, social awareness campaigns, and application of strict legislation that have translated to a reduction of dioxin emission levels and a general decline in ambient air concentrations. However, in other zones where nonindustrial sources have been traditionally high contributors, their emissions have remained more or less constant because it is more difficult to control this type of processes. In consequence, nowadays dioxin production from nonindustrial sources is almost as significant as the production from industrial sources (Quass et al., Citation2004). Another issue that supports this conclusion is the resolution from the U.S. Environment Protection Agency inventory of sources of dioxin-like compounds in the United States for the year 2000, where it was concluded that the open burning of residential refuse in backyard burn barrels was the largest source of dioxins (35.1%) in the country for that year (EPA, Citation2006).
Analyzing the fingerprint from several sources, it can be said that although the amounts of emitted levels of 2,3,7,8-TCDD are generally low or even undetectable, combustion processes still have associated significant emissions of dioxins, where industries like waste incinerators or metal manufacturers like steel industries or zinc production have the highest emission rate of PCDD/Fs. Nevertheless, different technologies can be used either to reduce the formation of dioxins by altering the industrial process toward more efficient conditions that do not enhance the formation of these compounds, or to reduce the proliferation of dioxins once they are formed, by different treatments in the flue gas or particles that capture and remove the organic congeners before they are emitted into the atmosphere.
It is more difficult to establish the same resolutions with nonindustrial sources because they depend on different and highly variable factors, like the composition of raw materials or the origin from many small different sources that make the emission be more diffuse and difficult to measure and control.
In conclusion, this document reflects the fact that dioxins are dangerous compounds whose emission must be controlled and reduced when possible. Also, the main features of the fingerprints from the most important sources are depicted, along with the emission levels of dioxins and their evolution over time. From this analysis it can be said that great improvements have been observed into the minimization of dioxins from industrial sources. On the other hand, factors like the lack of social awareness among the population lead to the interpretation that if that tendency is not reversed, the nonindustrial sources might be the main contributors in the formation of dioxins in the immediate future.
Additional information
Notes on contributors
Miguel Dopico
Miguel Dopico is an industrial engineer at the Universidad de Oviedo, Gijón, Asturias, Spain.
Alberto Gómez
Alberto Gómez is a research professor at the Universidad de Oviedo, Gijón, Asturias, Spain.
References
- Abad, E., K. Martinez, J. Caixach, and J. Rivera. 2004. Polychlorinated dibenzo-p-dioxin/polychlorinated dibenzofuran releases into the atmosphere from the use of secondary fuels in cement kilns during clinker formation. Environ. Sci. Technol. 38(18):4734–38. doi:10.1021/es049641a
- Abad E., K. Martinez, L. Gustems, R. Gomez, X. Guinart, I. Hernandez, and J. Rivera. 2007. Ten years measuring PCDDs/PCDFs in ambient air in Catalonia (Spain). Chemosphere 67:1709–14. doi:10.1016/j.chemosphere.2006.05.083
- Addink, R., and K. Olie. 1995. Role of oxygen in formation of polychlorinated dibenzo-p-dioxins/dibenzofurans from carbon on fly ash. Environ. Sci. Technol. 29(6):1586–90. doi:10.1021/es00006a023
- Alcock, R., and K. Jones. 1996. Dioxins in the environment: A review of trend data. Environ. Sci. Technol. 30:3133–43. doi:10.1021/es960306z
- Alcock, R., P. Behnisch, K. Jones, and H. Hagenmaier. 1998. Dioxin-like PCBs in the environment - Human exposure and the significance of sources. Chemosphere 37:1457–1472. doi:10.1016/S0045-6535(98)00136-2
- Altarawneh, M., B.Z. Dlugogorski, E.M. Kennedy, and J.C. Mackie. 2009. Mechanisms for formation, chlorination, dechlorination and destruction of polychlorinated dibenzo-p-dioxins and dibenzofurans (PCDD/Fs). Prog. Energy Combust. Sci. 35(3):245–74. doi:10.1016/j.pecs.2008.12.001
- Ames, M., S. Zemba, L. Green, M. J. Botelho, D. Gossman, I. Linkov, and J. Palma-Oliveira. 2012. Polychlorinated dibenzo p dioxin and furan (PCDD/F) congener profiles in cement kiln emissions and impacts. Sci. Total Environ. 419:37–43. doi:10.1016/j.scitotenv.2011.12.062
- Anderson, D.R., and R. Fisher. 2002. Sources of dioxins in the United Kingdom: The steel industry and other sources. Chemosphere 46(3):371–81. doi:10.1016/S0045-6535(01)00178-3
- Aries, E., D.R. Anderson, R. Fisher, T.A. Fray, and D. Hemfrey. 2006. PCDD/F and “dioxin-like” PCB emissions from iron ore sintering plants in the UK. Chemosphere 65(9):1470–80. doi:10.1016/j.chemosphere.2006.04.020
- Assefa, A., A. Sorbek, K. Sundqvist, I. Cato, P. Jonsson, M. Tysklind, and K. Wiberg. 2014. Temporal trends of PCDD/Fs in Baltic Sea sediment cores covering the 20th century. Environ. Sci. Technol. 48:947–53. doi:10.1021/es404599z
- Ba, T., M. Zheng, B. Zhang, W. Liu, K. Xiao, and L. Zhang. 2009a. Estimation and characterization of PCDD/Fs and dioxin-like PCBs from secondary copper and aluminum metallurgies in China. Chemosphere 75(9):1173–78. doi:10.1016/j.chemosphere.2009.02.052
- Ba, T., M. Zheng, B. Zhang, W. Liu, G. Su, and K. Xiao. 2009b. Estimation and characterization of PCDD/Fs and dioxin-like PCBs from secondary zinc and lead metallurgies in China. J. Environ. Monit. 11(4):867–72. doi:10.1039/b818555g
- Bajamundi, C., P. Vainikka, M. Hedman, I. Hyytiainen, J. Silvennoinen, T. Heinanen, R. Taipale, and J. Konttinen. 2014. Towards controlling PCDD/F production in a multi-fuel fired BFB boiler using two sulfur addition strategies. Part I: Experimental campaign and results. Fuel 134:677–87. doi:10.1016/j.fuel.2014.05.034
- Black, R., C. Meyer, A. Touati, B. Gullet, H. Fiedler, and J. Mueller. 2012. Emission factors for PCDD/PCDF and dl-PCB from open burning of biomass. Environ. Int. 38:62–66. doi:10.1016/j.envint.2011.07.003
- Bogdal, C., M. Scheringer, E. Abad, M. Abalos, B. Van Bavel, J. Hagberg, and H. Fiedler. 2013. Worldwide distribution of persistent organic pollutants in air, including results of air monitoring by passive air sampling in five continents. TrAC Trends Anal. Chem. 46:150–61. doi:10.1016/j.trac.2012.05.011
- Booth, S., J. Hui, Z. Alojado, V. Lam, W. Cheung, D. Zeller, D. Steyn, and D. Pauly. 2013. Global deposition of airborne dioxin. Mar. Pollut. Bull. 75(1):182–86. doi:10.1016/j.marpolbul.2013.07.041
- Breivik, K., V. Vestreng, O. Rozovskaya, and J. Pacyna. 2006. Atmospheric emissions of some POPs in Europe: A discussion of existing inventories and data needs. Environ. Sci. Policy 9:663–74. doi:10.1016/j.envsci.2006.09.001
- Buekens, A., E. Cornelis, H. Huang, and T. Dewettinck. 2000. Fingerprints of dioxin from thermal industrial processes. Chemosphere 40(9):1021–24. doi:10.1016/S0045-6535(99)00348-3
- Buekens, A., L. Stieglitz, K. Hell, H. Huang, and P. Segers. 2001. Dioxins from thermal and metallurgical processes: Recent studies for the iron and steel industry. Chemosphere 42(5):729–35. doi:10.1016/S0045-6535(00)00247-2
- Cao, Z., H. Fiedler, B. Wang, T. Zhang, G. Yu, J. Huang, and S. Deng. 2013. Economic status as a determinant of national PCDD/PCDF releases and implications for PCDD/PCDF reduction. Chemosphere 91:328–35. doi:10.1016/j.chemosphere.2012.11.054
- Caserini, S., and A. Monguzzi. 2002. PCDD/Fs emissions inventory in the Lombardy region: Results and uncertainties. Chemosphere 48:779–86. doi:10.1016/S0045-6535(02)00127-3
- Castro Jimenez, J., G, Mariani, I. Vives, H. Skejo, G. Umlauf, J. Zaldivar, S. Dueri, G. Messiaen, and T. Laugier. 2011. Atmospheric concentrations, occurrence and deposition of persistent organic pollutants (POPs) in a Mediterranean coastal site (Etang de Thau, France). Environ. Pollut. 159(7):1948–56. doi:10.1016/j.envpol.2011.03.012
- Chang, M., Y. Weng, T. Lee, Y. Chen, S. Chang, and K. Chi. 2003. Sampling and analysis of ambient dioxins in northern Taiwan. Chemosphere 51:1103–10. doi:10.1016/S0045-6535(02)00711-7
- Chang, Y., W. Lee, L. Wang, H. Yang, M. Cheng, J. Lu, Y. Tsai, and L. Young. 2014. Effects of waste cooking oil-based biodiesel on the toxic organic pollutant emissions from a diesel engine. Appl. Energy 113:631–38. doi:10.1016/j.apenergy.2013.08.005
- Chen, C.-M. 2004. The emission inventory of PCDD/PCDF in Taiwan. Chemosphere 54(10):1413–20. doi:10.1016/j.chemosphere.2003.10.039
- Chiu, J. C., Y. H. Shen, H. W. Li, L. F. Lin, L. C. Wang, G. P. Chang-Chien, et al. 2011. Emissions of polychlorinated dibenzo-p-dioxins and dibenzofurans from an electric arc furnace, secondary aluminum smelter, crematory and joss paper incinerators. Aerosol Air Qual. Res. 11(1):13–20. doi:10.4209/aaqr.2010.06.0051
- Chuang, S., K. Huang, S. Chen, L. Wang, G. Chang-Chien, and J. Tsai. 2011. PCDD/F emissions from gasoline and diesel fueled vehicles. Sustain. Environ. Res. 21(1):29–36.
- Clarke, B., N. Porter, R. Symons, J. Blackbeard, P. Ades, and P. Marriott. 2008. Dioxin-like compounds in Australian sewage sludge—Review and national survey. Chemosphere 72:1215–28. doi:10.1016/j.chemosphere.2008.01.076
- Cleverly, D., J. Ferrario, C. Byrne, K. Riggs, D. Joseph, and P. Hartford. 2007. A general indication of the contemporary background levels of PCDDs, PCDFs, and coplanar PCBs in the ambient air over rural and remote areas of the United States. Environ. Sci. Technol. 41:1537–44. doi:10.1021/es0616736
- Cole, P., D. Trichopoulos, H. Pastides, T. Starr, and J. Mandel. 2003. Dioxin and cancer: a critical review. Regul. Toxicol. Pharmacol. 38(3):378–88. doi:10.1016/j.yrtph.2003.08.002
- Coleman, P., R. Lee, R. Alcock, and K. Jones. 1997. Observations on PAH, PCB and PCDD/F trends in UK urban air, 1991–1995. Environ. Sci. Technol. 31:2120–24. doi:10.1021/es960953q
- Creaser, C., A. Fernandes, S. Harrad, and E. Cox. 1990. Levels and sources of PCDDs and PCDFs in urban British soils. Chemosphere 21:931–38. doi:10.1016/0045-6535(90)90116-B
- Dai, J., M. Xu, J. Chen, X. Yang, and Z. Ke. 2007. PCDD/F, PAH and heavy metals in the sewage sludge from six wastewater treatment plants in Beijing, China. Chemosphere 66(2):353–61. doi:10.1016/j.chemosphere.2006.04.072
- Ding, L., Y. Li, P. Wang, X. Li, Z. Zhao, T. Ruan, and Q. Zhang. 2013. Spatial concentration, congener profiles and inhalation risk assessment of PCDD/Fs and PCBs in the atmosphere of Tianjin, China. Chinese Sci. Bull. 58:971–78. doi:10.1007/s11434-013-5694-5
- Du, B., M. Zheng, H. Tian, A. Liu, Y. Huang, L. Li, et al. 2010. Occurrence and characteristics of polybrominated dibenzo-p-dioxins and dibenzofurans in stack gas emissions from industrial thermal processes. Chemosphere 80(10):1227–33. doi:10.1016/j.chemosphere.2010.05.044
- Dyke, P., C. Foan, M. Wenborn, and P. Coleman. 1997. A review of dioxin releases to land and water in the UK. Sci. Total Environ. 207(2):119–31. doi:10.1016/S0048-9697(97)00254-4
- Eduljee, G., and P. Dyke. 1996. An updated inventory of potential PCDD and PCDF emission sources in the UK. Sci. Total Environ. 177(1):303–21. doi:10.1016/0048-9697(95)04929-0
- Esposito, V., A. Maffei, D. Bruno, B. Varvaglione, S. Ficocelli, C. Capoccia, et al. 2014. POP emissions from a large sinter plant in Taranto (Italy) over a five-year period following enforcement of new legislation. Sci. Total Environ. 491:118–22. doi:10.1016/j.scitotenv.2014.03.077
- Everaert, K., and J. Baeyens. 2002. The formation and emission of dioxins in large scale thermal processes. Chemosphere 46(3):439–48. doi:10.1016/S0045-6535(01)00143-6
- Fiedler, H. 2007. National PCDD/PCDF release inventories under the Stockholm convention on persistent organic pollutants. Chemosphere 67(9):s96–108. doi:10.1016/j.chemosphere.2006.05.093
- Gullet, B., P, Lemieux, C. Lutes, C. Winterrowd, and D. Winters. 2001. Emissions of PCDD/F from uncontrolled, domestic waste burning. Chemosphere 43:721–25. doi:10.1016/S0045-6535(00)00425-2
- Gullet, B., and J. Ryan. 2002. On-road emissions of PCDDs and PCDFs from heavy duty diesel vehicles. Environ. Sci. Technol. 36:3036–40. doi:10.1021/es011376v
- Gullet, B., A. Touati, and L. Oudejans. 2008. PCDD/F and aromatic emissions from simulated forest and grassland fires. Atmos. Environ. 42:721–25. doi:10.1016/j.atmosenv.2008.06.046
- Gullet, B., B. Wyrzykowska, E. Grandesso, A. Touati, D. Tabor, and G. Ochoa. 2010. PCDD/F, PBDD/F, and PBDE emissions from open burning of a residential waste dump. Environ. Sci. Technol. 44:394–99. doi:10.1021/es902676w
- Gunes, G., and A. Saral. 2014. Seasonal variation of PCDD/Fs in the metropolis of Istanbul, Turkey. Environ. Sci. Pollut. Res. 21:8718–29. doi:10.1007/s11356-014-2798-7
- Gusev, A. 2014. Atmospheric emissions of PCDD/Fs in the Baltic Sea region. October 22, 2014. http://helcom.fi/baltic-sea-trends/environment-fact-sheets/hazardous-substances/atmospheric-deposition-of-pcdd-fs-on-the-baltic-sea ( accessed December 4, 2014).
- Gworek, B., A. Hajduk, E. Koda, A. Grochowalski, and A. Jeske. 2013. Influence of a municipal waste landfill on the spatial distribution of polychlorinated dibenzo-p-dioxins and dibenzofurans (PCDD/Fs) in the natural environment. Chemosphere 92(7):753–59. doi:10.1016/j.chemosphere.2013.03.037
- Hays, S.M., and L.L. Aylward. 2003. Dioxin risks in perspective: Past, present, and future. Regul. Toxicol. Pharmacol. 37(2):202–17. doi:10.1016/S0273-2300(02)00044-2
- Hedman, B., M. Naslund, C. Nilsson, and S. Marklund. 2005. Emissions of polychlorinated dibenzodioxins and dibenzofurans and polychlorinated biphenyls from uncontrolled burning of garden and domestic waste (backyard burning). Environ. Sci. Technol. 39:8790–96. doi:10.1021/es051117w
- Hites, R.A. 2010. Dioxins: An overview and history†. Environ. Sci. Technol. 45(1):16–20. doi:10.1021/es1013664
- Holtzer, M., J. Danko, and R. Danko. 2007. Possibilities of formation of dioxins and furans in metallurgical processes as well as methods of their reduction. Metalurgija 46(4):285–90.
- Huang, C., K. Chen, Y. Lai, L. Wang, and G. Chang-Chien. 2011. Characterization of atmospheric dry deposition of polychlorinated dibenzo-p-dioxins/dibenzofurans in a rural area of Taiwan. Aerosol Air Qual. Res. 11:448–59. doi:10.4209/aaqr.2011.03.0033
- Huang, H., and A. Buekens. 1995. On the mechanisms of dioxin formation in combustion processes. Chemosphere 31(9):4099–117. doi:10.1016/0045-6535(95)80011-9
- Ji, S., Y. Ren, A. Buekens, T. Chen, S. Lu, K. Cen, et al. 2014. Treating PCDD/Fs by combined catalysis and activated carbon adsorption. Chemosphere 102:31–36. doi:10.1016/j.chemosphere.2013.12.008
- Jones, K.C., and P. De Voogt. 1999. Persistent organic pollutants (POPs): State of the science. Environ. Pollut. 100(1):209–21. doi:10.1016/S0269-7491(99)00098-6
- Kaivojosa, T., A. Viren, J. Tissari, J. Ruuskanen, J. Tarhanen, O. Sippula, et al. 2012. Effects of a catalytic converter on PCDD/F, chlorophenol and PAH emissions in residential wood combustion. Chemosphere 88:278–85.
- Katsoyiannis, A., R. Gioia, A. Sweetman, and K. Jones. 2010. Continuous monitoring of PCDD/Fs in the UK atmosphere: 1991–2008. Environ. Sci. Technol. 44:5735–40. doi:10.1021/es1009828
- Khumsaeng, T., N. Oanh, K. Kare, and C. Polprasert. 2013. Emission of dioxins/furans and other U-POPs from test burns of non-POP pesticides in a hazardous waste incinerator. Waste Manage. 33(4):833–41. doi:10.1016/j.wasman.2012.11.012
- Kim, K., S., K. H. Hong, Y. H. Ko, K. D. Yoon, and M. G. Kim. 2003. Emission characteristics of PCDD/Fs in diesel engine with variable load rate. Chemosphere 53(6):601–7. doi:10.1016/S0045-6535(03)00540-X
- Kishida, M., K. Imamura, N. Takenaka, Y. Maeda, P. H. Viet, A. Kondo, and H. Bandow. 2010. Characteristics of the abundance of polychlorinated dibenzo-p-dioxin and dibenzofurans, and dioxin-like polychlorinated biphenyls in sediment samples from selected Asian regions in Can Gio, Southern Vietnam and Osaka, Japan. Chemosphere 78(2):127–33. doi:10.1016/j.chemosphere.2009.10.003
- Kulkarni, P.S., J.G. Crespo, and C.A. Afonso. 2008. Dioxins sources and current remediation technologies a review. Environ. Int. 34(1):139–53. doi:10.1016/j.envint.2007.07.009
- Lavric, E., A. Konnov, and J. Ruyck. 2004. Dioxin levels in wood combustion—A review. Biomass Bioenergy 26(2):115–45. doi:10.1016/S0961-9534(03)00104-1
- Lechtanska, P., and G. Wielgosinski. 2014. The use of ammonium sulfate as an inhibitor of dioxin synthesis in iron ore sintering process. Ecol. Chem. Eng. S 21(1):59–70.
- Lee, W., G. Chang-Chien, L. Wang, W. Lee, P. Tsai, and C. Chen. 2003. Emissions of polychlorinated dibenzo-p-dioxins and dibenzofurans from the incinerations of both medical and municipal solid wastes. Aerosol Air Qual. Res 3(1):1–6. doi:10.4209/aaqr.2003.06.0001
- Lee, W., G. Chang-Chien, L. Wang, W. Lee, P. Tsai, K. Wu, et al. 2004. Source identification of PCDD/Fs for various atmospheric environments in a highly industrialized city. Environ. Sci. Technol. 38:4937–44. doi:10.1021/es0499795
- Lee, W., G. Chang-Chien, L. Wang, W. Lee, K. Wu, and P. Tsai. 2005. Emissions of polychlorinated dibenzo-p-dioxins and dibenzofurans from stack gases of electric arc furnaces and secondary aluminum smelters. J. Air Waste Manage. Assoc. 55:219–26. doi:10.1080/10473289.2005.10464613
- Lee, R., P. Coleman, J. Jones, K. Jones, and R. Lohmann. 2005. Emission factors and importance of PCDD/Fs, PCBs, PCNs, PAHs and PM10 from the domestic burning of coal and wood in the UK. Environ. Sci. Technol. 39:1436–47. doi:10.1021/es048745i
- Lemieux, P., C. Lutes, J. Abbott, and K. Aldous. 2000. Emissions of polychlorinated dibenzo-p-dioxins and polychlorinated dibenzofurans from the open burning of household waste in barrels. Environ. Sci. Technol. 34:377–84. doi:10.1021/es990465t
- Lemieux, P., C. Lutes, and D. Santoianni. 2004. Emission of organic air toxics from open burning: a comprehensive review. Prog. Energy Combust. Sci. 30:1–32. doi:10.1016/j.pecs.2003.08.001
- Li, Y., P. Wang, L. Ding, X. Li, T. Wang, Q. Zhang, et al. 2010. Atmospheric distribution of polychlorinated dibenzo-p-dioxins, dibenzofurans and dioxin-like polychlorinated biphenyls around a steel plant area, Northeast China. Chemosphere 79(3):253–58. doi:10.1016/j.chemosphere.2010.01.061
- Li, Y., T. Chen, J. Zhang, W. Meng, M. Yan, H. Wang, et al. 2015. Mass balance of dioxins over a cement kiln in China. Waste Manage. 36:130–35. doi:10.1016/j.wasman.2014.11.027
- Lin, L.F., W.J. Lee, and G.P. Chang-Chien. 2006. Emissions of polychlorinated dibenzo-p-dioxins and dibenzofurans from various industrial sources. J. Air Waste Manage. Assoc. 56(12):1707–15. doi:10.1080/10473289.2006.10464575
- Lin, L. F., W. J. Lee, H. W. Li, M. S. Wang, and G. P. Chang-Chien. 2007. Characterization and inventory of PCDD/F emissions from coal-fired power plants and other sources in Taiwan. Chemosphere 68(9):1642–49. doi:10.1016/j.chemosphere.2007.04.002
- Lin, W., Y. Wu, L. Tu, L. Wang, and X. Lu. 2010. The emission and distribution of PCDD/Fs in municipal solid waste incinerators and coal fired power plant. Aerosol Air Qual. Res. 10:519–32. doi:10.4209/aaqr.2010.03.0017
- Lindgren, P., and S. Andersson. 2008. Adiox (R) for dioxin removal in wet scrubbers and semi-wet or dry absorbers. Waste management and the environment IV, pp. 569–77. doi:10.2495/WM080581
- Lindner, G., A. Jenkins, J. McCormack, and R. Adrian. 1990. Dioxins and furans in emissions from medical waste incinerators. Chemosphere 20:1793–800. doi:10.1016/0045-6535(90)90344-S
- Liu, G., M. Zheng, W. Liu, C. Wang, B. Zhang, L. Gao, G. Su, K. Xiao, and P. Lv. 2009. Atmospheric emission of PCDD/Fs, PCBs, hexachlorobenzene, and pentachlorobenzene from the coking industry. Environ. Sci. Technol. 43(24):9196–201. doi:10.1021/es902429m
- Liu, G., M. Zheng, G. Jiang, Z. Cai, and Y. Wu. 2013. Dioxin analysis in China. TrAC Trends Anal. Chem. 46:178–88. doi:10.1016/j.trac.2012.05.012
- Lohmann, R., and K.C. Jones. 1998. Dioxins and furans in air and deposition: a review of levels, behavior and processes. Sci. Total Environ. 219(1):53–81. doi:10.1016/S0048-9697(98)00237-X
- Lundin, L., B. Gullet, W. Carroll, A. Touati, S. Marklund, and H. Fiedler. 2013. The effect of developing nations’ municipal waste composition on PCDD/PCDF emissions from open burning. Atmos. Environ. 79:433–41. doi:10.1016/j.atmosenv.2013.06.040
- Lundin, L., and S. Jansson. 2014. The effects of fuel composition and ammonium sulfate addition on PCDD, PCDF, PCN and PCB concentrations during the combustion of biomass and paper production residuals. Chemosphere 94:20–26. doi:10.1016/j.atmosenv.2013.06.040
- Mandal, P.K. 2005. Dioxin: a review of its environmental effects and its aryl hydrocarbon receptor biology. J. Comp. Physiol. B 175(4):221–30. doi:10.1007/s00360-005-0483-3
- Mari, M., M, Nadal, M. Schuhmacher, and J. Domingo. 2008. Monitoring PCDD/Fs, PCBs and metals in the ambient air of an industrial area of Catalonia, Spain. Chemosphere 73:990–98. doi:10.1016/j.chemosphere.2008.06.025
- Mari, M., and J. Domingo. 2010. Toxic emissions from crematories: A review. Environ. Int. 36:131–37. doi:10.1016/j.envint.2009.09.006
- McLachlan, M. D., M. Horstmann, and M. Hinkel. 1996. Polychlorinated dibenzo-p-dioxins and dibenzofurans in sewage sludge: Sources and fate following sludge application to land. Sci. Total Environ. 185(1):109–23. doi:10.1016/0048-9697(96)05046-2
- Menad, N., H. Tayibi, F.G. Carcedo, and A. Hernandez. 2006. Minimization methods for emissions generated from sinter strands: A review. J. Cleaner Product. 14(8):740–47. doi:10.1016/j.jclepro.2004.03.005
- Morales, L., J. Dachs, B. Gonzalez-Gaya, G. Hernan, M. Abalos, and E. Abad. 2014. Background concentrations of polychlorinated dibenzo-p-dioxins, dibenzofurans, and biphenyls in the global oceanic atmosphere. Environ. Sci. Technol. 48:10198–207. doi:10.1021/es5023619
- Moussaoui, Y., L. Tuduri, Y. Kerchich, B. Meklati, and G. Eppe. 2012. Atmospheric concentrations of PCDD/Fs, dl-PCBs and some pesticides in northern Algeria using passive air sampling. Chemosphere 88:270–77. doi:10.1016/j.chemosphere.2012.02.025
- Nzihou, A., N. Themelis, M. Kemiha, and Y. Benhamou. 2012. Dioxin emissions from municipal solid waste incinerators (MSWIs) in France. Waste Manage. 32:2273–77. doi:10.1016/j.wasman.2012.06.016
- Onofrio, M., R. Spataro, and S. Botta. 2011. The role of a steel plant in north-west Italy to the local air concentrations of PCDD/Fs. Chemosphere 82:708–17. doi:10.1016/j.chemosphere.2010.10.095
- Pacyna, J., K. Breivik, J. Munch, and J. Fudala. 2003. European atmospheric emissions of selected persistent organic pollutants, 1970–1995. Atmos. Environ. 37:s119–31. doi:10.1016/S1352-2310(03)00240-1
- Pan, W., Y. Qi, R. Wang, Z. Han, D. Zhang, and J. Zhan. 2013. Adsorption of TCDD with 1-butyl-3-methylimidazolium dicyanamide ionic liquid: a combined molecular dynamics simulation and quantum chemistry study. Chemosphere 91(2):157–64. doi:10.1016/j.chemosphere.2012.12.021
- Paradiz, B., P. Dilara, G. Umlauf, I. Bajsic, and V. Butala. 2015. Dioxin emissions from coal combustion in domestic stove: Formation in the chimney and coal chlorine content influence. Thermal Sci. 19:295–304. doi:10.2298/TSCI140113079P
- Pulles, T., H. Kok, and U. Quass. 2006. Application of the emission inventory model TEAM: Uncertainties in dioxin emission estimates for central Europe. Atmos. Environ. 40:2321–32. doi:10.1016/j.atmosenv.2005.12.015
- Quass, U., M.W. Fermann, and G. Broker. 2004. The European dioxin air emission inventory project – Final results. Chemosphere 54(9):1319–27. doi:10.1016/S0045-6535(03)00251-0
- Quinn, L., R. Pieters, C. Nieuwoudt, A. Borgen, H. Kylin, and H. Bouwman. 2009. Distribution profiles of selected organic pollutants in soils and sediments of industrial, residential and agricultural areas of South Africa. J. Environ. Monit. 11:1647–57. doi:10.1039/b905585a
- Relvas, H., M. Lopes, and M. Coutinho. 2013. Portuguese inventory of dioxins and furans atmospheric emissions. Chemosphere 93:1569–77. doi:10.1016/j.chemosphere.2013.08.005
- Ren, Z., and M. Zheng. 2009. Impacts of human activities on dioxins emissions at national scale. Chemosphere 76:853–59. doi:10.1016/j.chemosphere.2009.03.070
- Rivera-Austrui, J., K. Martinez, L. Marco-Almagro, M. Abalos, and E. Abad. 2014. Long-term sampling of dioxin-like substances from a clinker kiln stack using alternative fuels. Sci. Total Environ. 485:528–33. doi:10.1016/j.scitotenv.2014.03.021
- Schatowitz, B., G. Brandt, F. Gafner, E. Schlumpf, R. Buhler, P. Hasler, et al. 1994. Dioxin emissions from wood combustion. Chemosphere 29:2005–13. doi:10.1016/0045-6535(94)90367-0
- Schuhmacher, M., S. Granero, J. Llobet, H. deKok, and J. Domingo. 1997. Assessment of baseline levels of PCDD/F in soils in the neighborhood of a new hazardous waste incinerator in Catalonia, Spain. Chemosphere 35:1947–58. doi:10.1016/S0045-6535(97)00269-5
- Schuhmacher, M., J. Domingo, J. Llobet, L. Muller, W. Sunderhauf, and J. Jager. 1998. Baseline levels of PCDD/Fs in vegetation samples collected in the vicinity of a new hazardous waste incinerator in Catalonia, Spain. Chemosphere 36:2581–91. doi:10.1016/S0045-6535(97)10220-X
- Shields, W. J., Y. Tondeur, L. Benton, and M. R. Edwards. 2010. 14 Dioxins and furans. In Environmental forensics: Contaminant specific guide, ed. R.D. Morrison and B.L. Murphy, 293. New York: Academic Press.
- Shih, S., W. Lee, L. Lin, J. Huang, J. Su, and G. Chang-Chien. 2008. Significance of biomass open burning on the levels of polychlorinated dibenzo-p-dioxins and dibenzofurans in the ambient air. J. Hazard. Mater. 153:276–84. doi:10.1016/j.jhazmat.2007.08.048
- Shin, S., G. Jin, W. Kim, B. Kim, S. Hwang, J. Hong, et al. 2011. Nationwide monitoring of atmospheric PCDD/Fs and dioxin-like PCBs in South Korea. Chemosphere 83:1339–44. doi:10.1016/j.chemosphere.2011.03.024
- Singh, S., and V. Prakash. 2007. Toxic environmental releases from medical waste incineration: A review. Environ. Monit. Assess. 132:67–81. doi:10.1007/s10661-006-9503-3
- Solorzano-Ochoa, G., D. de la Rosa, P. Maiz-Garralde, B. Gullet, D. Tabor, A. Touati, et al. 2012. Open burning of household waste: Effect of experimental condition on combustion quality and emission of PCDD, PCDF and PCB. Chemosphere 87:1003–8. doi:10.1016/j.chemosphere.2011.11.038
- Srogi, K. 2008. Levels and congener distributions of PCDDs, PCDFs and dioxin-like PCBs in environmental and human samples: A review. Environ. Chem. Lett. 6(1):1–28. doi:10.1007/s10311-007-0105-2
- Ssebugere, P., B. Kiremire, B. Henkelmann, S. Bernhooft, J. Wasswa, G. Kasozi, et al. 2013. PCDD/Fs and dioxin-like PCBs in surface sediments from Lake Victoria, East Africa. Sci. Total Environ. 454:528–33. doi:10.1016/j.scitotenv.2013.03.046
- Tame, N. W., B. Z. Dlugogorski, and E. M. Kennedy. 2007. Formation of dioxins and furans during combustion of treated wood. Prog. Energy Combust. Sci. 33(4):384–408. doi:10.1016/j.pecs.2007.01.001
- Trivedi, J., and D. Majumdar. 2013. Memory effect driven emissions of persistent organic pollutants from industrial thermal processes, their implications and management: A review. J. Environ. Manage. 119:111–20. doi:10.1016/j.jenvman.2013.01.026
- United Nations Environment Programme. 2005. Draft guidelines on best available techniques and provisional guidance on best environmental practices relevant to Article 5 and Annex C**. Paper presented at the conference of the parties of the Stockholm Convention on Persistent Organic Pollutants, first meeting, Punta del Este, Uruguay, May 2–6.
- United Nations Environment Programme. 2015. The 2nd POPs monitoring report Asia-Pacific region. chm.pops.int/implementation/globalmonitoringplan/monitoringreports
- U.S. Environmental Protection Agency. 2006. An inventory of sources and environmental releases of dioxin-like compounds in the United States for the years 1987, 1995, and 2000. Washington, DC: National Center for Environmental Assessment, Office of Research and Development, U.S. Environmental Protection Agency.
- Urban, J., D. Wikoff, A. Bunch, M. Harris, and L. Haws. 2014. A review of background dioxin concentrations in urban/suburban and rural soils across the United States: Implications for site assessments and the establishment of soil cleanup levels. Sci. Total Environ. 466:586–97. doi:10.1016/j.scitotenv.2013.07.065
- Van der Gon, H., M. van het Bolscher, A. Visschedijk, and P. Zandveld. 2007. Emissions of persistent organic pollutants and eight candidate POPs from UNECE—Europe in 2000, 2010 and 2020 and the emission reduction resulting from the implementation of the UNECE POP protocol. Atmos. Environ. 41(40):9245–61. doi:10.1016/j.atmosenv.2007.06.055
- Vassura, I., F. Passarini, L. Ferroni, E. Bernardi, and L. Morselli. 2011. PCDD/Fs atmospheric deposition fluxes and soil contamination close to a municipal solid waste incinerator. Chemosphere 83:1366–73. doi:10.1016/j.chemosphere.2011.02.072
- Wang, L. C., W. J. Lee, P. J. Tsai, W. S. Lee, and G. P. Chang-Chien. 2003. Emissions of polychlorinated dibenzo-p-dioxins and dibenzofurans from stack flue gases of sinter plants. Chemosphere 50(9):1123–29. doi:10.1016/S0045-6535(02)00702-6
- Wang, L., W.J. Lee, W.S. Lee, G. Chang-Chien, and P. Tsai. 2003. Effect of chlorine content in feeding waste of incineration on the emission of polychlorinated dibenzo-p-dioxins/dibenzofurans. Sci. Total Environ. 302(1):185–98. doi:10.1016/S0048-9697(02)00306-6
- Wang, L., Y. Wang, H. Hsi, and G. Chang-Chien. 2010. Characterizing the emissions of polybrominated diphenylethers (PBDEs) and polybrominated dibenzo-p-dioxins and dibenzofurans (PBDD/Fs) from metallurgical processes. Environ. Sci. Technol. 44(4):1240–46. doi:10.1021/es903128e
- Wang, M., L. Wang, G. Chang-Chien, and L. Lin. 2005. Characterization of polychlorinated dibenzo-p-dioxins and dibenzofurans in the stack flue gas of a municipal solid waste incinerator, in the ambient air, and in the Banyan leaf. Aerosol Air Qual. Res. 5(2):171–84.
- Wang, Q., Y. Jin, X. Li, J. Chen, S. Lu, T. Chen, et al. 2014. PCDD/F emissions from hazardous waste incinerators in China. Aerosol Air Qual. Res. 14:1152–59. doi:10.4209/aaqr.2013.03.0069
- Wang, X., Y. Ni, H. Zhang, X. Zhang, and J. Chen. 2012. Formation and emission of PCDD/Fs in Chinese non-wood pulp and paper mills. Environ. Sci. Technol. 46:12234–40. doi:10.1021/es303373b
- Wcgiel, M., R. Chrzaszcz, A. Maslanka, and A. Grochowalski. 2014. Study on the impact of industrial flue gases on the PCDD/Fs congener profile in ambient air. Chemosphere 114:76–83. doi:10.1016/j.chemosphere.2014.03.104
- Werther, J., and T. Ogada. 1999. Sewage sludge combustion. Progress in Energy and Combustion Science 25(1):55–116. doi:10.1016/S0360-1285(98)00020-3
- Wevers, M., R. De Fre, and M. Desmedt. 2004. Effect of backyard burning on dioxins deposition and air concentrations. Chemosphere 54:1351–56. doi:10.1016/S0045-6535(03)00253-4
- Wielgosinski, G. 2011. The reduction of dioxin emissions from the processes of heat and power generation. J. Air Waste Manage. Assoc. 61(5):511–26. doi:10.3155/1047-3289.61.5.511
- Wielgosinski, G., and A. Targaszewska. 2014. The impact of waste incineration on human beings and the environment. Ecol. Chem. Eng. S 21(2):353–63. doi:10.2478/eces-2014-0027
- Wohrnschimmel, H., and Y. Yao. 2014. Assessing comparability of atmospheric PCDD, PCDF and coplanar PCB data from North American ambient air monitoring networks. Canada: Commission for Environmental Cooperation. http://www3.cec.org/islandora/en/item/11537-assessing-comparability-atmospheric-pcdd-pcdf-and-coplanar-pcb-data-from-north
- Wu, J., T. Lin, Y. Wang, J. Wang, C. Wang, and Y. Kuo. 2014. Polychlorinated dibenzo-p-dioxin and dibenzofuran (PCDD/F) emission behavior during incineration of laboratory waste. Part 1: Emission profiles obtained using chemical assay and bioassay. Aerosol Air Qual. Res. 14(4):1199–205. doi:10.4209/aaqr.2013.05.0140
- Yan, M., X. D. Li, S. Y. Lu, T. Chen, Y. Chi, and J. H. Yan. 2011. Persistent organic pollutant emissions from medical waste incinerators in China. J. Mater. Cycles Waste Manage. 32(3):213–18. doi:10.1007/s10163-011-0020-2
- Yao, Y., S. Masunaga, H. Takada, and J. Nakanishi. 2002. Identification of polychlorinated dibenzo-p-dioxin, dibenzofuran, and coplanar polychlorinated biphenyl sources in Tokyo Bay, Japan. Environmental Toxicol. Chem. 21(5):991–98. doi:10.1002/etc.5620210514
- Yoshida, K., T. Yamamoto, T. Kuroki, and M. Okubo. 2009. Pilot-scale experiment for simultaneous dioxin and NOx removal from garbage incinerator emissions using the pulse corona induced plasma chemical process. Plasma Chem. Plasma Process. 29(5):373–86. doi:10.1007/s11090-009-9184-0
- Zeng, X., J. Ren, W. Pan, T. Zong, and Y. Li. 2011. Research on the formation and control measures of dioxin emissions from municipal solid waste incinerator. 2011 International Conference on Electrical and Control Engineering (ICECE), Yichang, China, September 2011, pp. 1707–10.
- Zhang, B., F. Meng, C. Shi, F. Yang, D. Wen, J. Aronsson, et al. 2009. Modeling the atmospheric transport and deposition of polychlorinated dibenzo-p-dioxins and dibenzofurans in North America. Atmos. Environ. 43:2204–12. doi:10.1016/j.atmosenv.2009.01.004
- Zhang, G., J. Hai, and J. Cheng. 2012. Characterization and mass balance of dioxin from a large-scale municipal solid waste incinerator in China. Waste Manage. 32(6):1156–62. doi:10.1016/j.wasman.2012.01.024
- Zhang, Q., J. Huang, and G. Yu. 2008. Polychlorinated dibenzo-p-dioxins and dibenzofurans emissions from open burning of crop residues in China between 1997 and 2004. Environ. Pollut. 151:39–46. doi:10.1016/j.envpol.2007.03.011
- Zhang, T., H. Fiedler, G. Yu, G. Ochoa, W. Carroll, B. Gullet, et al. 2011. Emissions of unintentional persistent organic pollutants from open burning of municipal solid waste from developing countries. Chemosphere 84:994–1001. doi:10.1016/j.chemosphere.2011.04.070
- Zheng, G., A. Leung, L. Jiao, and M. Wong. 2008. Polychlorinated dibenzo-p-dioxins and dibenzofurans pollution in China: Sources, environmental levels and potential human health impacts. Environ. Int. 34(7):1050–61. doi:10.1016/j.envint.2008.02.011
- Zheng, M.H., Z.C. Bao, B. Zhang, and X.B. Xu. 2001. Polychlorinated dibenzo-p-dioxins and dibenzofurans in paper making from a pulp mill in China. Chemosphere 44(6):1335–37. doi:10.1016/S0045-6535(00)00488-4
- Zhong, J., G. Zhang, J. Hai, J. Lv, J. Zhang, S. Zhang, et al. 2014. Polychlorinated dibenzo-p-dioxins and dibenzofurans from a grate-type municipal solid waste incinerator in China. Adv. Mater. Res. 878:616–21. doi:10.4028/www.scientific.net/AMR.878
- Zou, C., J. Han, and H. Fu. 2012. Emissions of PCDD/Fs from steel and secondary nonferrous productions. Procedia Environ. Sci. 16:279–88. doi:10.1016/j.proenv.2012.10.039