Abstract
The purpose of this research is to investigate the feasibility of utilizing the slag collected after gasification of organic fuel combined with sewage sludge. The residue left after gasification process is likely usable as raw material for production of supercondensers. The sewage sludge neutralization system consists of a dosing system (fuel tank), gasifier, plasma reactor, electrostatic filter, and heat exchangers. For the gasification process, dried solid sewage is supplied in proportion of 70% to biomass 30% by weight. The slag is collected in a specially designed chamber beneath the gasifier. A scanning electron microscope (SEM) was used to evaluate surface morphology of the samples. Elemental analysis of the sewage sludge slag was performed using the energy-dispersive spectroscopy (EDS) method, which showed different solid-state elements contained in the porous structure of the solid phase: carbon 29%, aluminum 26%, potassium 20%, chlorine 1%, and others. The specific surface area of the sewage sludge slag is 6.15 m2/g as the BET analysis shows. In order to use the slag as a secondary raw material, detailed analysis of the structure and properties is necessary for a decision on whether the slag left after gasification of sewage sludge is suitable for any further usages. Initial results indicate that the slag may be used for production of electrodes for supercapacitors.
Implications: Every year thousands of tons of sewage sludge are formed in Lithuania. Sewage sludge consists of organic and inorganic compounds. Partial combustion, plasma decomposition, and other methods are used to neutralize the sewage sludge. The incineration of sewage sludge results in generation of solid-phase slag. In this paper the material structure and composition of a solid slag (formed during neutralization of sewage sludge) is considered. Also, the impact the ambient temperature on structure and composition of solid slag is analyzed.
Introduction
The disposal of organic waste is a key topic worldwide, and in Lithuania as well. Huge amounts of organic waste, such as pesticides, used medicine chemicals, and chemical fertilizers, are accumulated each year. Many of these organic substances contain Cl, F, P, and Hg, to name few chemical elements. High concentrations of these elements in the waste may harm the environment when disposed of directly (Tang et al., Citation2013; Jiang et al., Citation2013). Most of these organic compounds are disposed of by thermal treatment technologies: low-temperature oxidation, distillation, desorption, and so on (Zhang et al., Citation2013b; Preis et al., Citation2013). The main disadvantage of these methods is the low temperature (sewage sludge gasification process temperature ranges from 850 to 900°C), which limits the possibility of complete destruction of organic substances in order to obtain complete combustion products, such as CO2 and H2O (Zhang et al., Citation2013a; Striugas et al., Citation2012). Using thermal destruction methods, viscous and solid-phase waste are transformed into the gaseous phase, where toxic substances such as furans, dioxins, and others are present. As is known, these substances are completely destroyed at temperatures higher than 1200°C and residence time at least 2 sec. These conditions guarantee nonhazardous emissions to environmental air.
Sewage sludge contains chemical elements such as C at 39.8%, H at 5.11%, N at 5.83%, S at 1.53%, O at 17.50%, and 51.02% volatile organic compounds. Also, some metal elements are present, such as Cl (0.14% detected). When combusted, sewage sludge produces solid slag adjacent to gaseous emissions. The main constituent of the slag is soot: carbon of various forms, and calcite, calcium oxalate, and oxygen, trapped in pores of residual carbon. Applying proper treatment of the residual slag and removal of unwanted substances makes it a valuable product for industry. After purification and activation of residual carbon it is feasible to utilize this as a raw material for high-capacity supercondenser production technology. Nevertheless, utilizing the slag from gasification as suitable product for raw material requires more detailed investigation into its physical characteristics, such as surface morphology, porosity, specific surface area, and so on. These characteristics greatly influence the usage of slag as a raw material for industry. It is worth mentioning that a huge amount of slag is produced during combustion of sewage sludge, and it is a valuable residue. In such a way it is possible to increase the economy of industrial processes by saving and employing the chemical compounds contained in the slag (Wang et al., Citation2009; Wang et al., Citation2014).
The slag can be properly processed to be suitable for production of supercapacitor electrodes, as well as for formation of different coatings. Coatings can be formed from the slag in powder form using a plasma spray coating technology. Since the slag contains carbon, formed coating layers can be used for friction reduction and life extension of various rapidly wearing mechanical structures.
Whether thermally treated slag is more promising for utilization than untreated, or not, depends on intended further usage. For example, the composition and properties of thermally treated slag suggest its further usage possibilities for production of supercondenser electrodes, while untreated slag is more suitable for coatings.
In order to use the slag, resulting after gasification of sewage sludge, as a secondary or tertiary raw material, initial analysis studies are necessary as structure and composition of the material are unknown. Moreover, there properties depend at least on the origin of sewage sludge and gasification process conditions.
The objective of this study is to find out utilization possibilities of the resulting sewage sludge slag, left after the gasification process, as a secondary raw material. Initial studies indicate that the slag contains considerable amounts of carbon and metals. This suggests the possibility of using it for production of electrodes for supercapacitors in the future. Heating of the slag is required in order to activate the carbon and remove some unwanted products and components, such as calcite and others.
Experimental Setup
The sewage sludge neutralization system consists of a dosing system (fuel tank), gasifier (which is responsible for solid material transformation into the gaseous phase), plasma reactor, electrostatic filter, and heat exchangers (). Dried solid sewage is supplied in proportion by weight of 70% to biomass 30% for the gasification process.
Feeding rate of the mixed fuel was 57 kg/hr, the gasification process temperature varied in-between 800 ºC to 850 ºC, and airflow was about 30 N-m3/hr. The gasification of 1 kg palletized mixture of 70wt% of dried sewage sludge and 30wt% of biomass produces 7.8–14.3wt% of slag. The analysis of the solid state slag results in C at 12%, H2 at 5.11%, N2 at 5.83%, S at 1.53%, O2 at 17.50%, and Cl at 0.14%. The slag of such composition is fed into the gasifier, where thermal decomposition takes place. The evolved gases from the gasification process are directed to the plasma reactor for thermal destruction of hazardous chemicals. The slag is collected in specially designed chamber beneath the gasifier. Solid particles, after the gasification of slag and plasma treatment of gases, are collected at electrostatic filters.
An electron scanning microscope (SEM) was used for evaluation of surface morphology of the slag. The chemical elements and crystallinity properties were identified using x-ray spectroscopy (XRD). This method allows determining amorphous or crystalline properties of the material. Porosity and specific surface area of the material were determined by the Brunauer–Emmet–Teller (BET) method. Volatile fraction was determined by a thermal analysis method. Thermal analysis was performed by the system coupled to a Fourier-transform infrared spectrometer (FTIR) for evolved gas analysis.
Results and Discussion
The gasification residue of the sewage sludge was obtained from the gasification reactor (). Volumetric content of oxygen during gasification was about 0.1% and the ambient temperature of the process was around 1000ºC.
A scanning electron microscope (SEM) was used to evaluate surface morphology of the samples (). This picture reveals the differences between thermally untreated slag and slag treated under 1000ºC. From it can be seen that slag composition is uneven, formed of microgranules that resemble microtubes with diameter around 50 µm. As can be seen from the SEM picture in , crude slag powder presents as irregular-shaped, sharp-edged derivatives, and the surface structure is irregular. It can be assumed that this structure is influenced by the amorphous carbon that is present in the slag. After thermal treatment, average diameter of microgranules decreases, while the surface becomes smoother. Solid particles of the slag are split into smaller ones of irregular shape (). This process is caused by the volatile part of the sample being released from the microsurface. Mesh-like structure can be noticed from the SEM image (). Such structure may develop due to melting of the slag components at high temperatures, thus resulting in a mesh surface.
Figure 2. SEM images of the sewage sludge slag: (a, b) before thermal treatment; (c, d) after treatment under 1000ºC.
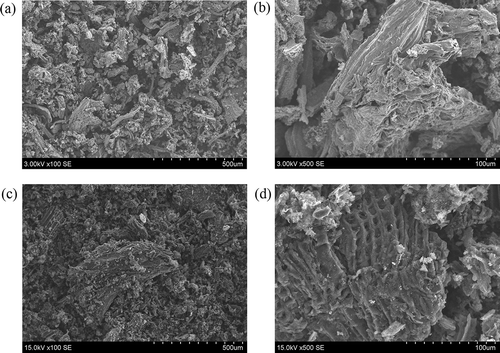
The elemental analysis of the sewage sludge slag made by the energy-dispersive spectroscopy (EDS) method showed different elements contained in the porous structure of the solid phase before and after thermal treatment of the slag. The results of analyses are presented in .
Table 1. EDS elemental analysis of sewage sludge slag.
As it can be noticed, EDS analysis shows a considerable decrease of chlorine, phosphorus, iron, and silicon after thermal treatment. After the treatment, Al, As, and Na elements were not discovered by the EDS method. This can be explained by chemical reactions at high temperatures, where these elements form other compounds, undetectable by the EDS method. The elements are not detected also by XRD analysis, most probably due to low concentrations or because of amorphous structure. Since the EDS method characterizes the sample only in elemental analysis, the extended analysis of the samples was performed by the x-ray diffraction (XRD) method.
The results of the XRD analysis of thermally treated and untreated sewage sludge slag are presented in . As can be seen, mainly three chemical compounds of crystallinity form were identified: calcite, calcium oxide, and magnesium oxide (Wang et al., Citation2014; Kong et al., Citation2013). The XRD results of thermally untreated slag shows the specific peaks of calcite, while these are negligible in the case of thermally treated sample. This may be explained by the calcite decomposition to calcium oxide at a temperature of about 800ºC and release of volatiles and carbon dioxide. The peak of thermally treated calcium oxide on the XRD profile is relatively lower than in the untreated sample due to probable partial decomposition of crystallites to the amorphous structure. Since calcite is a nonconductor, electrical conductivity of thermally treated slag should increase. This suggests usage of thermally treated slag for supercondensers, rather than untreated slag.
BET analysis shows 6.15 m2/g specific surface area of the sewage sludge slag. Carbon is considered a main contributor to the characteristics of the surface area, since the amount of carbon dominates and it is characterized by porous structure formation properties. Hence, by purifying and removing other elements, such as metals and other substances, it is feasible to increase the specific surface area for more efficient secondary use (Salma et al., Citation2014; Jia et al., Citation2014).
The distribution diagram of pores with diameter range from 2 to 27 nm with respect to pore volume is presented in . The pores with diameter of around 2 nm mostly dominate, while when increasing pore diameter up to 10 nm the surface area of micropores drastically decreases. This is due to the carbon contained in the sewage sludge slag.
Results of thermogravimetric analysis are shown in . Thermal behavior of the thermally untreated sample is represented by the thermogravimetric (TG) curve,which shows mass loss when heated under oxidizing atmosphere (air). Mass loss rate is represented by the mathematical derivative of the TG signal (DTG) curve, and change of composition of evolved gases is calculated and plotted as a Gram–Schmidt (GS) diagram. As can be seen, the transfer line does not cause significant delay of gases from TG to the FTIR detector since the Gram–Schmidt diagram correlates with the DTG curve.
The difference of temperature at the major mass loss peak is 5.6ºC, or about 32 sec. Analysis of evolved gases by the FTIR method produces a map of plotting time, wave number, and absorbance measurements every 14 sec ().
Figure 6. Map of plotting time, wave number, and FTIR absorbance measurements of evolved gases during TG analysis of the sewage sludge residue under oxidizing atmosphere.
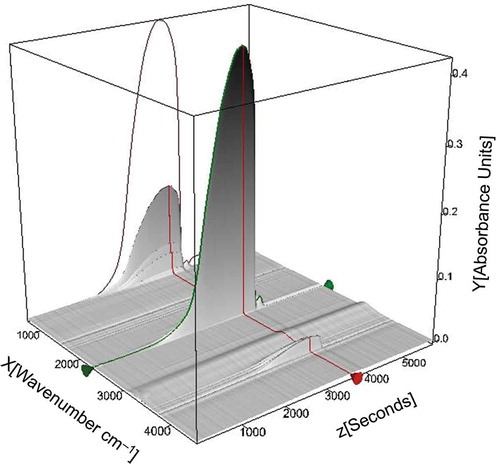
Two specific infrared (IR) spectra were selected from the array of results () for spectra evaluation at mass loss peak temperatures, and are represented in as GS diagram peaks, at 574.4ºC and 679.5ºC ().
The major mass loss of 84.48% up to about 650ºC was observed, with maximum mass loss rate 4.4%/min at 574.4ºC. This is considered to be due to oxidation and partial decomposition of the char, that is, release of CO2 and small amount of CO gases (Wang et al., Citation2014). This can be confirmed by FTIR absorption spectra at 574.4ºC ( and , blue curve). The spectrum has specific CO2 absorption bands at 3700–3600 and 2400 300 cm−1 and CO absorption bands at 2176 and 2120 cm−1. Another small stage of mass loss of 2.13% takes place at a temperature range 665–705ºC with maximum mass loss rate 0.63%/min at 679.5ºC (see DTG curve in ). Spectra taken at the Gram–Schmidt peak show release of CO2 (, red curve). Residual mass after thermal analysis of sewage sludge residue under oxidizing atmosphere was 13.74%. This is due to the relatively high content of mineral matter in the sample.
Conclusion
Gasification and combustion of sewage sludge produce slag as solid residue, which contains a considerable list of chemicals (metals and organometallic compounds). SEM analysis shows thermally untreated slag having a structure of irregularly shaped granules with sharp edges, while after thermal treatment the granules splits into smaller parts and the surface grid structure becomes evident. EDS analysis reveals metal elements (calcium, phosphorus, potassium, etc.). After thermal treatment, concentrations of many of the elements considerably decrease, and some are already below detection limits (e.g., aluminum, arsenic, sodium). It may be concluded that these elements react with other impurities that are present in the slag. Also, results of XRD analysis reveal characteristic peaks of calcium oxide and calcium, while other peaks of expected metal compounds were undetectable. It can be concluded, that concentrations of other elements are below the detection limits of the XRD analysis. The characteristic peak of carbon was not recorded by XRD. This may be explained by the amorphous structure of the carbon present in the sample. The XRD analysis shows the decrease of peaks of calcite in the thermally treated sewage sludge slag samples. This is due to decomposition of the calcite into volatile gases and calcium oxide. The specific surface area of the sewage sludge slag according to BET analysis is 6.15 m2/g. Porosity analysis reveals domination of mesopores, while micropores and macropores were not recorded. TG-FTIR with evolved gas analysis shows evolution of combustion product CO2. Mass loss under inert atmosphere from the TG analysis was 84.48wt%. It can be concluded that metals and organic carbon, which are present in the slag of sewage sludge after gasification, may be used in industry after proper treatment.
SEM images reveal that the powder porous structure is irregular in shape. According to BET analysis, the surface area is big enough to be suitable for supercondensers, and carbon presence is confirmed by EDS analysis. These properties (surface area, porous structure, and carbon) are the main components and conditions for production of supercondensers. Furthemore, there are some metal impurities, which is expected to improve the electrical conductivity of electrodes because of an increased amount of electric charge carriers, consequently resulting in higher electrical charge and higher capacity of condensers. Confirmation of these assumptions requires more experimental research.
Additional information
Notes on contributors
Zydrunas Kavaliauskas
Zydrunas Kavaliauskas, Vitas Valincius, Giedrius Straveinskas, Mindaugas Milieska, and Nerijus Striugas all work at the Lithuanian Energy Institute.
Vitas Valincius
Zydrunas Kavaliauskas, Vitas Valincius, Giedrius Straveinskas, Mindaugas Milieska, and Nerijus Striugas all work at the Lithuanian Energy Institute.
Giedrius Stravinskas
Zydrunas Kavaliauskas, Vitas Valincius, Giedrius Straveinskas, Mindaugas Milieska, and Nerijus Striugas all work at the Lithuanian Energy Institute.
Mindaugas Milieska
Zydrunas Kavaliauskas, Vitas Valincius, Giedrius Straveinskas, Mindaugas Milieska, and Nerijus Striugas all work at the Lithuanian Energy Institute.
Nerijus Striugas
Zydrunas Kavaliauskas, Vitas Valincius, Giedrius Straveinskas, Mindaugas Milieska, and Nerijus Striugas all work at the Lithuanian Energy Institute.
References
- Jiang B., J. Zheng, X. Lu, Q. Liu, M. Wu, Z. Yan, S. Qiu, Q. Xue, Z. Wei, H. Xiao, and M. Liu. 2013. Degradation of organic dye by pulsed discharge non-thermal plasma technology assisted with modified activated carbon fibers. Chem. Eng. J. 216:969. doi:10.1016/j.cej.2012.11.046
- Jia, Y., K. Li, S. Zhang, L. Li, and J. Ren. 2014. Microstructure and mechanical properties of multilayer-textured 2D carbon/carbon composites. J. Mater. Sci. Technol. 12:1202. doi:10.1016/j.jmst.2014.03.026
- Kong, L., J. Bai, Z. Bai, Z. Guo, and W. Li. 2013. Effects of CaCO on slag flow properties at high temperatures. Fuel 109(2013):76. doi:10.1016/j.fuel.2012.11.014
- Preis, S., D. Klauson, and A. Gregor. 2013. Potential of electric discharge plasma methods in abatement of volatile organic compounds originating from the food industry. J. Environ. Manage. 114:125. doi:10.1016/j.jenvman.2012.10.042
- Salman, M., O. Cizer, Y. Pontikes, L. Vandewalle, B. Blanpain, and K. Van Balen. 2014. Effect of curing temperatures on the alkali activation of crystalline continuous casting stainless steel slag’. Construst. Build. Mater. 71:308. doi:10.1016/j.conbuildmat.2014.08.067
- Striugas, N., K. Zakarauskas, G. Stravinskas, and V. Grigaitiene. 2012. Comparison of steam reforming and partial oxidation of biomass pyrolysis tars over activated carbon derived from waste tire. Catal. Today 196:67. doi:10.1016/j.cattod.2012.04.065
- Tang, L., H. Huang, H. Hao, and K. Zhao. 2013. Development of plasma pyrolysis/gasification systems for energy efficient and environmentally sound waste disposal. J. Electrostat. 71:839. doi:10.1016/j.elstat.2013.06.007
- Wang, Q., J. Yan, X. Tu, Y. Chi, X. Li, S. Lu, and K. Cen. 2009. Thermal treatment of municipal solid waste incinerator fly ash using DC double arc argon plasma. Fuel 88:955. doi:10.1016/j.fuel.2008.12.011
- Wang, S., X. Peng, L. Tang, L. Zeng, and C. Lan. 2014. Influence of inorganic admixtures on the 11 Å-tobermorite formation prepared from steel slags: XRD and FTIR analysis. Construct. Build. Mater. 60:42. doi:10.1016/j.conbuildmat.2014.03.002
- Zhang, Q., L. Dor, A. K. Biswas, W. Yang, and W. Blasiak. 2013a. Modeling of steam plasma gasification for municipal solid waste. Fuel Process. Technol. 106:546. doi:10.1016/j.fuproc.2012.09.026
- Zhang, Q., Y. Wua, L. Dor, W. Yang, and W. Blasiak. 2013b. A thermodynamic analysis of solid waste gasification in the plasma gasification melting process. Appl. Energ. doi:10.1016/j.apenergy.2013.03.054