Abstract
This paper presents a survey of pesticide residues in tanks following application and throughout the cleanout procedure as conducted by 46 volunteer operators across Colorado. While many pesticides were detected, this paper focuses on dicamba and 2,4-D, which were detected by liquid chromatography/tandem mass spectroscopy (LC-MS/MS). An exponential decrease in concentration was observed with sequential rinses, although this decrease may be more rapid for more water-soluble pesticides. More than 95% of the pesticide in the prerinse solution was removed by the end of the third rinse in all but three operator samples. Concentrations after three rinses were 0.41 ± 0.25 and 3.3 ± 1.1 mg/L for dicamba and 2,4-D, respectively. These concentrations suggest that the recommended practice of three rinses may not be adequate to eliminate off-target effects or point sources of pesticide waste, and that the recommended standard of personal protective equipment is essential to prevent worker exposure to the chemicals.
Implications: This paper demonstrates that the waste generated during cleanout of pesticide application devices constitutes a potential source of pollution and worker exposure. In particular, while the first rinse of pesticide containers is often treated as hazardous waste and reapplied to crops, the remaining rinses are not. This work demonstrates that the wastewater generated in subsequent rinses can have high enough concentrations to impact worker health, cause off-target effects on crops, and potentially constitute a point source of pesticides. The practical implication is for improved recommendations and regulations regarding pesticide applicators and their cleanout process.
Introduction
The preparation of pesticide containing solutions for agricultural application and the subsequent cleanout of pesticide tanks by operators is typically repeatedly performed at the same farmyard site (Castillo et al., Citation2008). While runoff of pesticide-containing water, or the transport of pesticides through soils and into groundwater, has been the focus of much research, evidence is emerging that the preparation and cleanout, or repeated rinsing, of pesticide tanks may act as a point source of pesticides to the environment (Helweg et al., Citation1998; Higginbotham et al., Citation1999; Poggi-Varaldo Citation1999; Suciu et al., Citation2011), elevating concentrations in groundwater and runoff (Gan et al., Citation1996). The application of pesticides to farms typically involves three stages: (i) preparation of pesticide solution in the tank, (ii) spraying pesticide on cropland, and (iii) the cleanout of the pesticide tank. While pesticide solution preparation has the potential to introduce small volumes of highly concentrated liquids into the environment, the cleanout phase could introduce larger volumes of more dilute liquids (Castillo et al., Citation2008). Agricultural workers are encouraged to follow guidelines for preparation described on the label by the pesticide companies and are typically required to wear personal protective equipment, reducing the potential for both point sources and worker exposure during the first stage of pesticide application. While the focus of pesticide point sources is typically the spraying phase of application, this phase is highly regulated. However, the third and final phase of the application process, cleanout of the pesticide tank, remains poorly informed by research. While questions have been raised about the best practices for pesticide cleanout to minimize both worker exposure and point sources to the environment, little research has been done on this stage, either in idealized conditions or to identify the actual practices employed by agricultural workers. Several studies of pesticide concentrations found that washing of the exterior of spray tanks may constitute a point source of pesticides (Jensen and Spliid, Citation2004; Ramwell et al., Citation2007), consistent with the suggestion that washing the interior of the same spray tanks will likely lead to even higher concentrations.
Unsurprisingly, the soil underneath pesticide preparation and cleanout areas exhibits higher pesticide concentrations than other farmyard areas (Helweg et al., Citation2002). This is likely due to a combination of spills, drips, and release of the dilute rinsates during the cleanout phase of application (Higginbotham et al., Citation1999). Much research has focused on the use of biobeds, which are mixtures of peat, straw, and topsoil, covered by grass, that are designed to retain and degrade pesticide-containing waste produced during solution preparation and cleanout (Castillo and Torstensson, Citation2007; Castillo et al., Citation2008; Fogg et al., Citation2003). These biobeds are typically tested with pesticide solutions intended to mimic real-world conditions of pesticide preparation and cleanout (Spliid et al., Citation2006). At least one study (Spliid et al., Citation2006) designed its testing of biobeds based on concentrations derived from testing the exterior of field sprayers for pesticides—potentially leading to an overestimate of concentrations applied during the cleanout process. However, while pesticide solutions for application follow formulations specified by the manufacturer, and should be replicable, there are few studies of pesticide concentrations of rinsate generated throughout the cleanout process. Without accurate concentrations of pesticides in rinsate generated by agricultural workers, it is difficult to assess the effectiveness of different biobed forumulations, the potential of cleanout waste to act as a point source to the environment, or the potential for rinsate to contribute to occupational exposure to pesticides by agricultural workers.
In the United States, pesticide education programs typically recommend three rinses of pesticide tanks. The first rinse should be reapplied to crops, but the second and third rinses are often disposed of in a designated area. The cleanout procedures sometimes include commercial detergents, either specific to the pesticides used or, with surprising frequency, ammonia, bleach, or hand detergent (Walker and Farmer, Citation2014). To the best of our knowledge, only a single study of pesticide tank cleanout investigated rinsate concentration as a function of number of rinses for six pesticide mixes (Pydrin, Lorsban, Comite, Orthene, Kelthane, and Phosdrin) for two aerial applicators (Woodrow et al., Citation1989). This study used the regression coefficients between concentration and number of rinses to suggest that three rinses was adequate to reduce rinsate concentration to <1% of the original mix, but that final rinsate concentrations for two of the pesticides, Pydrin and Lorsban, were higher than the LC50 for rainbow trout (Woodrow et al., Citation1989). However, this study investigated only a limited number of pesticides under very controlled conditions, and did not necessarily capture rinsate concentrations of actual cleanout practices. This study did, however, raise questions about the extent to which pesticide residues left in applicator tanks may act as point sources or cause unintended off-target impacts.
A clear understanding of potential pathways for pesticides to enter the environment is essential in determining the best practices for pesticide applicators to reduce environmental contamination and worker exposure (Higginbotham et al., Citation1999). Here, we present new data of pesticide concentrations collected during cleanout from 46 applicators in Colorado as part of a larger survey of 1102 applicators on cleanout practices, the largest ever such study of cleanout practices and pesticide concentrations in rinsates. We focus our analysis on dicamba and 2,4-D, though results from multiple other pesticides are also described. Dicamba and 2,4-D are herbicides that are widely used for agriculture. Dicamba is a pre- and postemergent broad-leaf herbicide in the benzoic acid and chlorophenoxy classes of compounds; it is widely used on crops, including wheat, corn, and soybean. 2,4-D is in the phenoxyacetic acid class of compounds, and is used to control broad-leaf weeds in cereal crops. With the advent of genetically engineered resistant crop strains (Cao et al., Citation2011), usage of these two pesticides in particular is predicted to increase in the United States.
These data allow us to determine the efficacy of cleanout procedures by actual operators, as opposed to idealized simulations, for dicamba and 2,4-D, and to quantify the potential for pesticide rinsates to act as point sources of pesticides to the environment and as contributors to occupational pesticide exposure by agricultural workers.
Materials and Methods
Samples from 46 anonymous Colorado pesticide applicators were collected as part of a larger survey on pesticide tank cleanout, in which applicators were given the opportunity to volunteer as anonymous participants in a study of pesticide cleanout efficacy. The information obtained from sample submission was not directly linked to the submitters. Participants were provided with informed consent, which was included with the sampling methods—if a participant did not agree with the informed consent, the participant did not conduct the sampling and was not included in this study. However, due to incomplete information from surveys or ambiguous labeling, nine sets of samples were excluded from the analysis. The study and protocols for contacting applicators were approved by the Colorado State University (CSU) Institutional Review Board, and carried out by CSU Cooperative Extension. Volunteer pesticide applicators were provided with sample jars and detailed instructions to collect samples of (1) spray solution from the tank, (2) water sample from water source, (3a–3c) samples from up to three tank rinses, and (4) a sample of tank cleaner, if used. Samples were returned in insulated containers with icepacks. Volunteers answered a short survey to determine applicator type and most recently used pesticides, and returned the samples in coolers to the CSU Department of Chemistry for analysis.
Samples (jars 1, 2, 3a–3c) were tested by high-performance liquid chromatography–tandem mass spectroscopy (HPLC-MS/MS) for the pesticides noted as “recently used” by applicators. The instrument used was a Hewlett-Packard Agilent 1100 series high-performance liquid chromatograph coupled to mass spectrometric detection (HPLC–ultraviolet [UV]–electrospray ionization [ESI]–MS/MS) using a 0.45-µm polytetrafluoroethylene (PTFE) filter and a Waters XBridge BEH C18 XP column (130 Å, 3.5 µm, 2.1 mm × 150 mm). The tandem mass spectrometer settings were optimized using 10 µM to 25 µM pesticide standards in 1% acetonitrile and 99% water. The total runtime of the chromatography was 30 min (flow rate of 0.5 mL/min, injection volume of 20 μL), with mobile phase including (A) a mixture of 0.1% formic acid in water and (B) a mixture of 0.1% formic acid in acetonitrile. Elution followed a linear gradient from a mixture of 80% A and 20% B to 100% B over 20 mins; 100% B for 2 min; and equilibration back to starting conditions over 8 min. Dicamba was detected at 9.9 min, with a parent ion at m/z 219.3 and a product ion at m/z 175. 2,4-D was detected at 12.95 min, with a parent ion at m/z 219.4 and a product ion at m/z 161.
Calibration curves in ESI negative mode for pesticide mixed standards levels of 100–10,000 μg/L and a 20% water/80% acetonitrile blank showed linear responses for 17 pesticides (Figure S1). Pesticide standards were provided by the Colorado Department of Agriculture from the Environmental Protection Agency. Both dicamba and 2,4-D were detected with negative mode electrospray ionization with a detection limit of 10 μg/L and 22 μg/L.
Samples were also measured by a novel HPLC–UV/visible (Vis) measurement; however, this measurement did not include dicamba or 2,4-D, and is thus described in the Supplemental Information.
Results
While 26 pesticides were investigated using LC-MS/MS and LC-UV/Vis detectors, we noted that only pesticides in a subset were listed as recently used by multiple applicators. 2,4-D and dicamba were the most widely used of the measured pesticides, and had the largest number of samples ( and ). These two herbicides became the focus of our analysis (). For 2,4-D, concentrations of the initial sample prior to the first rinse ranged from 2,089 to 1,054,738 μg/L (average = 31,200, standard error [SE] = 57,000), while third and final rinsate concentrations ranged from 0 to 29,207 μg/L (average = 3,300, SE =1,200). Comparison of the final rinsate to prerinse concentrations showed that, on average, 2,4-D was removed with 98% efficiency (range 73–100%, n = 26). For dicamba, precleaning initial samples ranged from 408 to 650,450 μg/L (average = 245,000, SE = 55,000), resulting in an average 100% cleanout efficiency (range 98-100%, n = 21).
Table 1. The average (standard error) and range of concentrations (mg/L) observed in samples for dicamba and 2,4-D.
Figure 1. Concentrations of dicamba (μg/L, or ppb) in background water, prerinse application solution, and following the first, second, and third rinses. Each individual sample is represented by the gray dots, while the average for each component is represented with a black circle. The y-axis is logarithmic to incorporate the dynamic range of magnitude in concentrations observed.
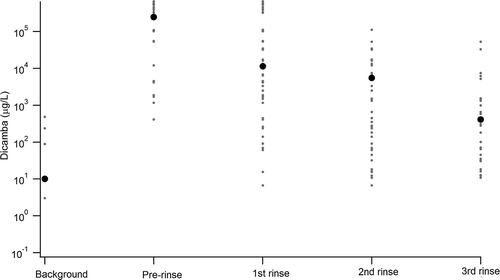
Figure 2. Concentrations of 2,4-D (μg/L, or ppb) in background water, prerinse application solution, and following the first, second, and third rinses. Each individual sample is represented by the gray dots, while the average for each component is represented with a black circle.
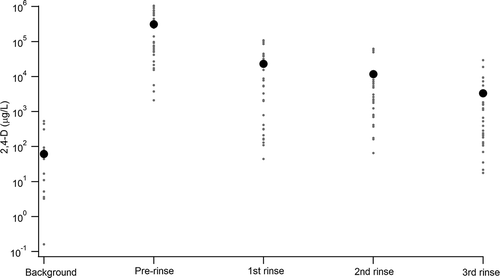
Figure 3. The change in concentration of dicamba (black) and 2,4-D (red) as a function of number of rinses (0–3 rinses) is fitted to an exponential decay. Circle markers represent the average, with error bars representing the standard error in concentration.
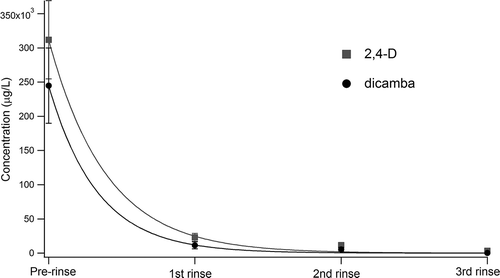
All but three sample sets from the 47 applicators showed that applicator cleanout was able to remove >95% of the pesticide from the initial prerinsing solution by the final rinse. While only a single rinse typically removes 90–95% of the pesticide, the recommended three rinses appears to remove the bulk of the pesticide initially placed in containers. Of the three applicator samples that removed <95% of the initial pesticide by the third rinse, all contained 2,4-D and one additionally contained dicamba. The sample set with the lowest cleanout rate (79% 2,4-D) was washed three times, and the initial concentration was 70 ppm, substantially lower than the 2,4-D initial sample average of 312 ppm. This outlier is likely due to inadequate volumes of cleanout liquid being used by this particular applicator.
Complete sample sets for all pesticides demonstrated decreasing pesticide concentrations for each rinse ( and ). Average rinsate concentrations for the prerinse sample, and samples following the first, second, and third rinses, show an exponential decrease in pesticide concentration, as do rinsate concentrations for each individual. For example, a logarithmic regression for dicamba concentrations from each applicator resulted in correlation coefficients (r2) ranging from 0.046 to 0.9998 (mean = 0.815; median = 0.882; only 1 of 25 applicators showed r2 < 0.5). Thus, the samples from a majority of the applicators showed an exponential decrease in concentration with number of rinses. The exponential fit follows eq 1,
Table 2. Summary of chemical and physical properties for seven pesticides, including their aqueous solubility (g/L at pH 7), and parameters derived from exponential decay fits
Discussion
Exponential fits for the data show that dicamba may be more rapidly cleaned out than 2,4-D (evidenced by the lower τ of the fit), potentially due to its higher water solubility (6.5 g/L for dicamba vs. 0.9 g/L for 2,4-D in water; Kamrin, Citation2010) (). While other pesticides do not have more than five samples, exponential fits for average picloram, metsulfuron-methyl, and mecoprop-p rinsates all showed τ values between 0.19 and 0.48, in contrast to the single sample set containing pendimethalin (, solubility = 0.0003 g/L), for which the exponential fit showed much less efficient cleanout (τ = 1.2 ± 0.2). This observation of decay constants (τ) on the order of 0.3 is consistent with results from Woodrow et al., though that study concluded that cleanout rates were consistent across the variety of pesticides tested, potentially due to similar water solubilities of the pesticides under study (Woodrow et al., Citation1989). Instead, we suggest that pesticides with particularly poor water solubility may require substantially more rinses, or use of an organic cleaning solvent such as acetone, to achieve the same cleanout efficiencies as relatively soluble pesticides like dicamba and 2,4-D, though more investigation is need to verify this hypothesis. shows rinse constants as a function of water solubility for each pesticide, and suggests that pesticides with water solubilities less than 0.2 g/L may have rinse constants, or τ, greater than 1 and will thus be particularly challenging to efficiently clean solely with water. For a compound like pendimethalin with a rinse constant of 1.2, six water rinses would be required to reduce the concentration to 1% of the original concentration. However, the solubility of many pesticides is pH dependent, and acidification or alkalinization of rinse water may increase the solubility and improve cleanout efficacy. The nature of the exponential nature of the decay means that the target cleanout rate will depend on the starting concentrations (preexponential factor, A)—that is, more dilute pesticide mixtures need fewer rinses to reach the same concentration of rinsate than a more concentrated mixture. We note that in the limited sample set in this study, this preexponential factor is smallest for the two least water-soluble pesticides, atrazine and pendimethalin. Thus, while they may be more difficult to clean out, these two pesticides are present in lower concentrations in the first place.
Figure 4. The rinse constant, τ, is derived from the exponential fits and indicates the number of rinses required to decrease the concentration to ~37% (1/e) of the initial pesticide concentration. This constant is plotted against pesticide water solubility (g/L). Errors in the fit are reflected in the y-axis error bars.
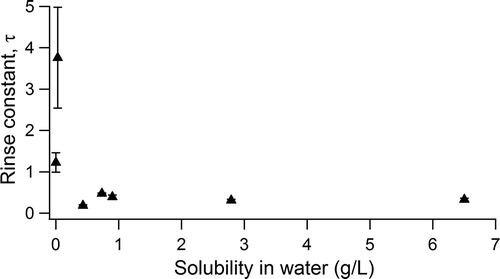
Despite high cleanout efficiency, the final rinses for many applicators still showed pesticide levels substantially higher than the background samples of clean water used to rinse the pesticide tanks (). These data show that after a single rinse of dicamba, at least one sample was below the detection limit of the instrument, while this occurred only after three rinses of 2,4-D, consistent with the dicamba being more easily cleaned out due to its higher water solubility. Concentrations of dicamba after three rinses were substantially lower than 2,4-D, but still an average of 410 μg/L. This final observation raises the question of whether three rinses are adequate to eliminate unintended application when pesticide residues from a previous application are unintentionally applied on agricultural areas and have unintended, negative consequences. Such effects have been implicated in herbicide-contaminated manure (Kylin et al., Citation2007). While the addition of new pesticide mix to the tank will substantially dilute the rinsate, a fourth rinse may be necessary to prevent accidental application of pesticide to particularly susceptible nontarget crops. This may be a greater problem for less water soluble pesticides such as pendimethalin. This idea is consistent with observations of external pesticide concentrations on surfaces of field spray applicators, in which more soluble pesticides were observed in higher concentration following washings (Jensen and Spliid, Citation2004).
An important question for pesticide applicators is whether the current best practices recommendation of three rinses is adequate for effective cleanout, and whether the common recommendation that only the first rinse be reapplied to crops is appropriate. To examine this, the averages, standard error and range of concentrations observed for dicamba and 2,4-D are presented in . The first rinsate is typically reapplied to the crops, and the relatively high concentrations suggest that is an appropriate action. However, similar disposal techniques may be appropriate for the second rinsate, as concentrations average 3.0–3.6 mg/L of dicamba and 2,4-D. While these rinsate concentrations may seem high, it is difficult to assess the potential impact of these rinsates on aquatic or terrestrial life if not reapplied to crops, as they will be rapidly diluted in the environment, and likely pose a minimal environmental threat. However, these results suggest that post-cleanout rinses may act as point sources for pesticides in the environment. The importance of residues generated during cleanout of applicator tanks on public health, environment, and agricultural worker safety depends on the toxicity and environmental mobility and persistence of the pesticide. These final concentrations are all substantially (i.e., multiple orders of magnitude) lower in concentration than the concentrations in the initial pesticide solution or first rinsate. Thus, these second and third rinsates will have only a small impact as point sources, having shorter environmental persistence and faster degradation rates than the initial solution or first rinsate (Felsot and Dzantor, Citation1995; Gan et al., Citation1996; Helweg et al., Citation1998; Higginbotham et al., Citation1999).
The direct exposure of humans to the first or second rinsates is of concern in terms of worker exposure and safety. The applicator survey accompanying sample collection revealed multiple applicators who did not wear full personal protective equipment during the entire cleanout process, suggesting a potential mechanism for exposure of pesticide applicators to high concentrations of even the more soluble pesticides. The authors must recommend that all rinses be treated with care using proper personal protective equipment, although further directed studies on occupational exposure during cleanout are needed. While much attention has been paid to the potential for worker exposure during pesticide application (Choi et al., Citation2013; Moon et al., Citation2013), few studies have considered worker exposure during other stages of pesticide application (Harris et al., Citation2010). A study of worker exposure to pesticides on external surfaces of sprayers showed that even for short exposure times, large enough concentrations of pesticides could be transferred to workers so as to pose a potentially significant occupational exposure (Ramwell et al., Citation2005). While the concentrations of the rinsates are likely much lower than the concentrations on the external surfaces of sprayers, the potential exposure time is much longer. Both dicamba and 2,4-D have relatively low toxicity toward humans compared to other pesticides, but both have been linked to eye irritation upon contact. Thus, handling of even the third cleanout rinse may constitute a previously unconsidered occupational exposure for pesticide applicators. These observations are consistent with anecdotal reports of unintended pesticide exposure during nozzle calibration of aerial application systems.
The exponential decay in pesticide concentrations with multiple rinses demonstrates that there are diminishing benefits to encouraging more than three rinses with the relatively soluble dicamba and 2,4-D. Further, agricultural workers must balance water usage with cleanout, so more than three rinses may be reasonable only in the case of less water-soluble pesticides that may cause off-target effects.
Biobeds present an intriguing approach to minimizing the point source presented by disposal of pesticide-containing rinsates. Further investigation of the ability of biobeds to remove dicamba and 2,4-D, in particular, is required, along with the impact of co-application of these two herbicides to microbial degradation in the biobed, as there is evidence that multiple pesticides can lead to nonlinear effects on microbial populations and degradation rates.
The results of this survey of pesticide cleanout efficacy demonstrate that sequential rinses exponentially decrease the concentration of pesticide in the tank. While three rinses typically remove >95% of the pesticide from the tank, the final concentrations observed in many of the samples were high enough to impact occupational exposure to the pesticide by workers if personal protective equipment is not worn throughout the entire cleanout process. Further, the pesticide applicators who only use one or two rinses are at risk for experiencing off-target effects. This study raises questions about the efficacy of water rinses for less water-soluble pesticides, which may require more rinses than dicamba or 2,4-D for equally efficient cleanout.
Funding
The authors thank the High Plains Intermountain Center for Agricultural Health and Safety (HICAHS) organization and the Hermann Frasch Foundation (708-HF12) for funding. The authors thank the NSF REU program (CHE-1004924) for supporting KDS. In addition, The authors thank Dr. Chuck Henry and his group at CSU for access and assistance with the LC-UV/Vis, Eric Petty at the Colorado Department of Agriculture, and the staff of the CSU Central Instrument Facility for assistance with the LC-MS/MS analysis.
Supplemental data
Supplemental data for this article can be accessed at publisher’s website
Supplementary Material
Download PDF (441.1 KB)Additional information
Funding
Notes on contributors
Penny P. Osborne
Penny P. Osborne was a graduate student at Colorado State University, and is currently an analytical chemist in Fort Collins, CO.
Zichun Xu
Zichun Xu is an undergraduate student at Colorado State University.
Kenneth D. Swanson
Kenneth D. Swanson was an NSF Research Experience for Undergraduate student at Colorado State University, and is currently a graduate student at the University of North Carolina.
Thia Walker
Thia Walker is an Extension Specialist and program manager for the Colorado Environmental Pesticide Education Program (CEPEP) at Colorado State University.
Delphine K. Farmer
Delphine K. Farmer is an Assistant Professor of Chemistry at Colorado State University.
References
- Cao, M. X., S. J. Sato, M. Behrens, W. Z. Jiang, T. E. Clemente, and D. P. Weeks. 2011. Genetic engineering of maize (Zea mays) for high-level tolerance to treatment with the herbicide dicamba. J. Agric. Food Chem. L\59(11):5830–34. doi:10.1021/Jf104233h
- Castillo, M. D., and L. Torstensson. 2007. Effect of biobed composition, moisture, and temperature on the degradation of pesticides. J. Agric. Food Chem. 55(14):5725–33. doi:10.1021/Jf0707637
- Castillo, M. D. P., L. Torstensson, and J. Stenstrom. 2008. Biobeds for environmental protection from pesticide use—A review. J. Agric. Food Chem. 56(15):6206–19. doi:10.1021/Jf800844x
- Choi, H., J. K. Moon, and J. H. Kim. 2013. Assessment of the exposure of workers to the insecticide imidacloprid during application on various field crops by a hand-held power sprayer. J. Agric. Food Chem. 61(45): 10642–48. doi:10.1021/Jf403169t
- Felsot, A. S., and E. K. Dzantor. 1995. Effect of Alachlor concentration and an organic amendment on soil dehydrogenase-activity and pesticide degradation rate. Environ. Toxicol. Chem. 14(1):23–28. doi:10.1897/1552-8618(1995)14[23:Eoacaa]2.0.Co;2
- Fogg, P., A. B. A. Boxall, and A. Walker. 2003. Degradation of pesticides in biobeds: The effect of concentration and pesticide mixtures. J. Agric. Food Chem. 51(18):5344–49. doi:10.1021/Jf030060z
- Gan, J., L. Becker, W. C. Koskinen, and D. D. Buhler. 1996. Degradation of atrazine in two soils as a function of concentration. J. Environ. Qual. 25(5):1064–72. doi:10.2134/jeq1996.00472425002500050019x
- Harris, S. A., P. J. Villeneuve, C. D. Crawley, J. E. Mays, R. A. Yeary, K. A. Hurto, and J. D. Meeker. 2010. National study of exposure to pesticides among professional applicators: An investigation based on urinary biomarkers. J. Agric. Food Chem. 58(18):10253–61. doi:10.1021/Jf101209g
- Helweg, A., H. Bay, H. P. B. Hansen, M. Rabolle, A. Sonnenborg, and L. Stenvang. 2002. Pollution at and Below Sites Used for Mixing and Loading of Pesticides. Int. J. Environ. Anal. Chem. 82(8–9):583–90. doi:10.1080/03067310290009497
- Helweg, A., I. S. Fomsgaard, T. K. Reffstrup, and H. Sorensen. 1998. Degradation of mecoprop and isoproturon in soil influence of initial concentration. Int. J. Environ. Anal. Chem. 70(1–4):133–148. doi:10.1080/03067319808032610
- Higginbotham, S., R. L. Jones, E. Gatzweiler, and P. J. Mason. 1999. Point-source pesticide contamination: Quantification and practical solutions. 1999 Brighton Conference: Weeds, Vols. 1–3, 681–686.
- Jensen, P. K., and N. H. Spliid. 2004. External deposits of different pesticides on field sprayers. Asp. Appl. Biol. 71:365–70.
- Kamrin, M. S. 2010. Pesticide Profiles: Toxicity, Environmental Impact, and Fate, 704. Boca Raton, FL: CRC Press.
- Kylin, H., L. Torstensson, A. Ramberg, L. Volkova, and P. Sundin. 2007. Crop damage may occur after herbicide disposal in manure: A preliminary study. Acta Agric. Scand. B-S P 57(3):243–47. doi:10.1080/09064710600914244
- Leike, A. 2002. Demonstration of the exponential decay law using beer froth. Eur. J. Phys. 23:21–26. doi:10.1088/0143-0807/23/1/304
- Moon, J. K., S. Park, E. Kim, H. Lee, and J. H. Kim. 2013. Risk assessment of the exposure of insecticide operators to fenvalerate during treatment in apple orchards. J. Agric. Food Chem. 61(2):307–11. doi:10.1021/Jf3043083
- Poggi-Varaldo, H. M. 1999. Agricultural wastes. Water Environ. Res. 71(5): 737–85. doi:10.2175/106143099X133767
- Ramwell, C. T., P. D. Johnson, A. B. A. Boxall, and D. A. Rimmer. 2005. Pesticide residues on the external surfaces of field crop sprayers: Occupational exposure. Ann. Occup. Hyg. 49(4):345–50. doi:10.1093/annhyg/meh101
- Ramwell, C. T., J. Leak, S. E. Cooper, and W. A. Taylor. 2007. The potential environmental impact of pesticides removed from sprayers during cleaning. Pestic. Manag. Sci. 63:1146–52. doi:10.1002/ps.1447
- Spliid, N. H., A. Helweg, and K. Heinrichson. 2006. Leaching and degradation of 21 pesticides in a full-scale model biobed. Chemosphere 65(11): 2223–32. doi:10.1016/j.chemosphere.2006.05.049
- Suciu, N. A., T. Ferrari, F. Ferrari, M. Trevisan, and E. Capri. 2011. Pesticide removal from waste spray-tank water by organoclay adsorption after field application to vineyards. Environ. Sci. Pollut. Res. Int. 18(8):1374–83. doi:10.1007/s11356-011-0494-4
- Walker, T., and D. K. Farmer. 2014. Final report: Survey and effectiveness of pesticide application equipment cleanout methods. 2012-2013 HICAHS Pilot Grant Program. http://csu-cvmbs.colostate.edu/academics/erhs/agricultural-health-and-safety/Pages/default.aspx
- Woodrow, J. E., J. M. Wong, and J. N. Seiber. 1989. Pesticide residues in spray aircraft tank rinses and aircraft exterior washes. Bull. Environ. Contam. Toxicol. 42:22–29. doi:10.1007/BF01699199