Abstract
This study was conducted to examine the synthesis and application of novel nano-size calcium/iron-based composite material as an immobilizing and separation treatment of the heavy metals in fly ash from municipal solid waste incineration. After grinding with nano-Fe/Ca/CaO and with nano-Fe/Ca/CaO/[PO4], approximately 30 wt% and 25 wt% of magnetic fraction fly ash were separated. The highest amount of entrapped heavy metals was found in the lowest weight of the magnetically separated fly ash fraction (i.e., 91% in 25% of treated fly ash). Heavy metals in the magnetic or nonmagnetic fly ash fractions were about 98% and 100% immobilized, respectively. Additionally, scanning electron microscopy combined with energy-dispersive X-ray spectrometry (SEM-EDS) observations indicate that the main fraction of enclosed/bound materials on treated fly ash includes Ca/PO4-associated crystalline complexes. After nano-Fe/Ca/CaO/[PO4] treatment, the heavy metal concentrations in the fly ash leachate were much lower than the Japan standard regulatory limit for hazardous waste landfills. These results appear to be extremely promising. The addition of a nano-Fe/Ca/CaO/PO4 mixture with simple grinding technique is potentially applicable for the remediation and volume reduction of fly ash contaminated by heavy metals.
Implications: After grinding with nano-Fe/Ca/CaO and nano-Fe/Ca/CaO/[PO4], approximately 30 wt% and 25 wt% of magnetic fraction fly ash were separated. The highest amount of entrapped heavy metals was found in the lowest weight of the magnetically separated fly ash fraction (i.e., 91% in 25% of treated fly ash), whereas heavy metals either in the magnetic or nonmagnetic fly ash fractions were about 98% and 100% immobilized. These results appear to be very promising, and the addition of nano-Fe/Ca/CaO/PO4 mixture with simple grinding technique may be considered potentially applicable for the remediation and volume reduction of contaminated fly ash by heavy metals.
Introduction
In Japan, incineration is a commonly used method for treating municipal solid waste (MSW). It can reduce waste mass by 70% and volume by up to 90% while generating electricity from the combustion of waste. The amounts of ash produced are increasing because of the increasing number of incineration plants in Japan (Yang et al., Citation2014). The waste generated from MSW incineration is usually used in one of two ways: disposed as landfill or for reuse as secondary raw materials. Because land is scarce and environmental controls are tight in Japan, environmental policies tend to reduce landfill disposal to the greatest degree possible (Jung et al., Citation2004; Okada et al., Citation2007; Sakai, Citation1996).
Generally, MSW incineration produces two types of ash: bottom ash and fly ash (FA) (Hong et al., Citation2000), which is defined as the particulate residue collected from incinerator flue gases using state-of-the-art air pollution control devices (Hong et al., Citation2000; Yang et al., Citation2014). That ash residue might be regarded as comprising two phases: a carryover phase and a condensed phase (Boghetich et al., Citation2005). Fundamentally, the carryover phase is either unaffected by the high incineration temperatures or exists as a liquid or melt of uniform composition. The phase eventually becomes the fly ash matrix (Ecke, Citation2003). However, at elevated temperatures, the condensed phase is found in a volatile state. After cooling, it is deposited onto and diffuses into the nonvolatile matrix.
Homogeneous condensation is the formation of particles that consist entirely of condensed species. Heterogeneous condensation is the deposition of the condensed phase onto entrained particles found in the gas stream. The entrained particles are usually made up of carryover-phase materials. The combustion chamber environment is generally regarded as a reducing environment, although the incineration process is performed in highly oxidizing conditions. Under a localized reducing atmosphere, metal oxides can be reduced at the elevated temperatures of the process. Reduced species, such as Zn, are more readily evaporated than the initial oxide species. During cooling, and in a high oxygen environment, secondary reactions occur in which the newly reduced species revert to the original refractory compounds and condense (Hong et al., Citation2000; Yang et al., Citation2014).
It is noteworthy that the condensed phase is particularly important because of its constituent species. The fly ash particles include high concentrations of toxic heavy metals in the surface deposit. At the elevated temperatures of incineration, chlorinating agents such as HCI and Cl2 react with the metal oxides in the MSW to form chlorides, which are soluble and which are readily extracted from the ash. An equivalent reaction occurs also with SO3 to form surface sulfates (Wang et al., Citation2010). The small fly ash particles are rich in heavy metals because of the large specific surface area of the particles and the high propensity for these particles to adsorb the toxic, readily leachable compounds. Cahill and Newland (Citation1982) and Ontiveros et al. (Citation1989) have reported the ash particle formation mechanism. Lower-boiling-point metals such as lead and cadmium evaporate in the combustion chamber and condense on the surfaces of inert particles carried by combustion gases. The higher-boiling-point elements tend to remain in the nonvolatile state. They are incorporated into the ash particle matrix. The toxicity and leachability of the surface species necessitate that fly ash be regarded with concern. Current global environmental regulations have identified fly ash as hazardous waste (Chou et al., Citation2009; Wang et al., Citation2009).
The small respirable particles of fly ash are easily inhaled to the lungs, where the harmful condensed phases are extracted by body fluids and thereby pose a health hazard (Lauf, Citation1985; Nakane, Citation2012; Shim et al., Citation2012). At disposal in landfills and ash ponds, once exposed to moisture, leaching from the ash will occur and provide a pathway for toxic compounds to reach groundwater supplies (Jianguo and Wei, Citation1998; Jianguo et al., Citation2004). Furthermore, incorrect treatment can cause transformation of the heavy metals and can pollute the soil and air (Jianguo and Wei, Citation1998; Jianguo et al., Citation2004; Wang et al., Citation2010). The heavy metals in fly ash do not degrade naturally. Consequently, they strongly and persistently affect the environment.
The search for safe and effective methods of treating fly ash produced from municipal solid waste incineration is now an urgent social and environmental problem. Additionally, it is becoming increasingly important to find methods of using this fly ash. For the reuse of ash as filler for cement or pavement, only minimal leachability of metals is permitted by national legislation. Solidification and stabilization technology are keys to solving the problem of poisonous and hazardous waste. Solidification consists of cement solidification and asphalt solidification (Jiang et al., Citation2009; Polettini et al., Citation2001; Santanu and Pak, Citation2006; Sawada et al., Citation2001). This method is technically mature, easily performed, and entails low costs. However, the increasing waste capacity ratio of solidification renders subsequent transportation difficult. The subsequent landfilling results in tremendous waste of land resources. Moreover, heavy metals might leach out after weathering (Hong et al., Citation2000). Stabilization is achieved mainly using chemical agents, which might include sulfides, phosphates, silicates, and lime (Sukandar et al., Citation2009; Ying et al., Citation2013). Using this method, heavy metals leach out when the surrounding pH changes. Therefore, stabilization does not satisfy the requirement for long-term security. At present, the reported organic stabilizing chemical agents are mainly dithiocarbamate and its derivatives, organic polyphosphoric acid and its salt chemicals, and chitosan derivatives (Sukandar et al., Citation2009; Ying et al., Citation2013). Despite the adequate stabilization effects of these agents, numerous problems such as high production costs and the lack of raw material resources make this treatment extremely expensive and less applicable in industry. Therefore, finding easy-to-produce and readily obtainable materials and low-cost chemical agents with sufficient stabilizing effects is an important research topic related to fly ash treatment.
Our recent investigations revealed that a nanometallic Ca/CaO mixture was the most effective for hydrodechlorination of about 98% of polychlorinated dibenzodioxins (PCDDs), polychlorinated dibenzofurans (PCDFs), and polychlorinated biphenyls (PCBs) in contaminated fly ash, and also for cesium immobilization of about 96% in soil by ball-milling treatment (Mallampati et al., Citation2012a, Citation2014; Mitoma et al., Citation2011; Lee et al., Citation2015). For the present study, we developed a dry method for separating and immobilizing heavy metals in fly ash samples that is being applied to large volumes. This is a mixture of nano-scale particles of metallic calcium, calcium oxide, and metallic iron. Grinding a mixture of heavy metal–contaminated fly ash and nano-calcium reduces the particle size and coats each particle with nano-calcium, thereby immobilizing the heavy metals on surfaces. They might be enclosed or bound with Ca-associated (CaCO3/Ca(OH)2) salts, whereby high heavy metal immobilization can occur (Mallampati et al., Citation2012a, Citation2014). This process produces a dry sample that is separable by particle size at room temperature. This separation can be achieved through a balance of magnetic forces and gravity if the nano-calcium that is used contains iron or another paramagnetic material. The coated particles are placed in water underneath a strong magnet. The smallest particles, which have the highest concentrations of cesium, are attracted to the magnet even against gravity (Mallampati et al., Citation2012a, Citation2014). In addition, to diminish further heavy metal leaching, it was decided to evaluate the potential combination of Fe/Ca/CaO/NaH2PO4 [PO4] in the in situ formation of hydroxyapatite, which can further enhance heavy metal immobilization and harden the cemented shell (Mallampati et al., Citation2012a, Citation2014; Lee et al., Citation2015). This method concentrates heavy metals into a smaller volume of insoluble material in a dry condition that is amenable to storage. This paper reports the results of the dual separation and immobilization of heavy metals from a spiked MSWI fly ash sample. The degree of metal immobilization was evaluated by analyzing the leachable fraction of heavy metals obtained through the application of the Japanese leaching standards. Furthermore, details of heavy metal immobilization and separation mechanism were obtained.
Materials and Method
Sample of fly ash
The incineration-produced fly ash used in this research came from municipal solid waste (MSW) incinerator in Shobara City, Hiroshima Prefecture, Japan. This MSW facility, with treatment capacity of 30 tons per day, applies a semidry method and a bag dust collecting method to dispose of smoke. In accordance with the Technical Specifications on Sampling and Sample Preparation from Industry Solid Waste (HJ/T20-1998), 1 kg of fly ash was collected each day for three consecutive days during stable operation of the incinerator under normal conditions. The samples were evenly mixed, then they were set aside. Spiked/synthetically contaminated heavy metals (As, Cd, Cr, and Pb) MSWI fly ash sample were prepared in the laboratory, with standard solution spread to 1 kg of fly ash in a plastic bottle and mixed thoroughly for 24 hr to obtain each 100 mg/kg of As, Cd, Cr, and Pb desired concentrations. The inorganic chemical composition of the fly ash was analyzed using X-ray fluorescence spectrometry (EDX-720; Shimadzu Corp., Tokyo, Japan). The results are presented in .
Table 1. Main inorganic chemical composition in fly ash (% by weight).
Nano-Fe/CaO and nano-Fe/Ca/CaO preparation
Solvent-free production of the reagent, a dispersed mixture of nano-Fe/Ca/CaO, was prepared using planetary ball-milling processing. Unlike conventional methods such as chemical synthesis and vapor-phase condensation, which typically involve toxic chemicals, sophisticated equipment, and extensive labor to break down the nanometallic Fe/Ca/CaO particles, the precision ball-milling method relies solely on the mechanical impact forces generated by stainless steel beads in a high-speed rotary chamber. The system, which uses no toxic solvents, is completely scalable to large-scale manufacturing. Granular particles of metallic calcium (99%; 2–2.5 mm particle size distribution; 0.43–0.48 m2 g−1 surface area) and iron (size 0.15 mm) were purchased from Kishida Chemical Co. Ltd. (Osaka, Japan). Nano-Fe/Ca/CaO (dry system) systems were prepared by combining, respectively, Fe, Ca, and CaO through a mechanochemical process. At room temperature under Ar, dry CaO (preheated at 825 °C for 2 hr) and dry Fe/metallic Ca (Fe:Ca:CaO = 2:2:5) were introduced into a planetary ball mill (20 pieces SUS [System Usability Scale], 32 g/ball). Milling was conducted for 1 hr at 600 rpm to achieve a rotation-to-revolution speed ratio of 1 to –2. These conditions for the dispersed nano-systems preparation were established after performing several experiments to ascertain the optimal conditions. After preparation, Fe/Ca/CaO systems were sampled in Ar-filled storage glass bottles for additional study. Nano-Fe/Ca/CaO mixture particle size distribution and microstructure are presented in . The maximum particle size distribution was observed, respectively, as 206 nm for Fe/Ca/CaO.
Fly ash treatment
The fly ash samples (10 g) were mixed with either 1 g nano-Fe/Ca/CaO, 0.5 g nano-Fe/Ca/CaO, or 0.5 g NaH2PO4 ([PO4]). A blank experiment with only 10 g of heavy metal–contaminated fly ash was conducted simultaneously. The three mixtures were ground in a 500-mL ceramic tumbling mill along with 10 ceramic balls (10 mm diameter) for 2 hr at 100–150 rpm in open atmosphere. After the treatment, the samples were analyzed and compared with the untreated fly ash samples. The magnetic and nonmagnetic fly ash fractions were easily separated through a balance of magnetic forces and gravity with a laboratory magnetic stick. This separation can be achieved through a balance of magnetic forces and gravity if the nano-calcium that is used contains iron or some other paramagnetic material. The coated particles are placed in water underneath a strong magnet. The smallest particles, which have the highest concentrations of heavy metals, are attracted to the magnet, even against gravity. Magnetic separation is a physical separation process that segregates materials based on magnetic susceptibility. The technology relies only on physical properties. Therefore, separation can be achieved while producing a minimum of secondary waste.
Fly ash sample analysis
The total heavy metal concentrations in the in the fly ash samples were measured using the method reported by Baker and Amacher (Citation1982) for the analysis of heavy metals, which consisted of the digestion of fly ash samples in a mixture of HF-HNO3-HClO4-H2SO4 (Helmke and Sparks, Citation1996). First, fly ash (1 g) was introduced into a 250-mL Teflon beaker. Then, 4 mL of 40% hydrofluoric acid, 2 mL of 30% perchloric acid, 5 mL of 60% nitric acid, and 5 mL of 60% hydrochloric acid (analytical grade; Merck and Co. Inc., Tokyo, Japan) were added sequentially while stirring. The heterogeneous mixture was heated to dryness after the addition of each of the acids described above. The final residue was dissolved in 50 mL HCl 0.1 N to yield a solution of metals.
Determining the amounts of heavy metals leaching out of the FA
The Japanese standard leaching test (JLT-13) for the solid residues was performed to ascertain the amounts of heavy metals that leached out of the fly ash. For this test, extraction buffer composed of hydrochloric acid and sodium hydroxide of pH 6.00 ± 0.05 was prepared and used at a liquid-to-solid (L/S) ratio of 10:1. The buffer was added to the fly ash and was mechanically shaken continuously for 6 hr in an incubator shaker at 200 rpm. After 6 hr, the leachate samples were filtered using a 0.45-μm filter unit.
Total heavy metal concentrations (after acid digestion) and eluted solutions (after leaching test) in the fly ash were measured before and after treatment using inductively coupled plasma optical emission spectrometry (ICP-OES; 720-ES; Varian Inc., Tokyo, Japan). To verify reproducibility of this experiment, parallel determinations were performed three times for each experimental condition. These experiments were repeated three times, yielding similar results. The relative standard deviation of the samples was less than 1%.
To elucidate the immobilization mechanisms and to verify whether fly ash alterations occurred during the treatment or not, the following analyses were conducted. Scanning electron microscopy combined with energy-dispersive X-ray spectrometry (SEM-EDS) microanalysis and semiquantitative analysis were conducted (JSM6510A equipped with a Si (Li) probe with 138 eV resolution; JEOL, Tokyo, Japan) to analyze As, Cd, Cr, and Pb elemental distribution within the solid matrix. Generally, EDS was able to detect the fluorescent X-rays from the surface layer to 10 µm depth.
Results and Discussion
Heavy metal immobilization and separation
The total concentrations of As, Cd, Cr, Pb, and Fe in the solution, after acid digestion of untreated and treated fly ash with nano-Fe/Ca/CaO and nano-Fe/Ca/CaO/PO4, are presented in . All values presented in the table are averages of three replications. The condensed total concentrations of As, Cd, Cr, and Pb in the solution, after acid digestion of magnetic and nonmagnetic fractions fly ash after treatment with nano-Fe/Ca/CaO and nano-Fe/Ca/CaO/PO4, were about 85% and 15% and 91% and 9%, respectively, from the initial total heavy metal concentrations ( and ). However, as might be expected, the Fe concentration increased because of nano-Fe particles that were present in the treated materials (). After treatment with nano-Fe/Ca/CaO and nano-Fe/Ca/CaO/PO4, about 30 wt% and 25 wt% of magnetic fraction fly ash were separated. On the other hand, only by mixing, with no addition of additives, fly ash magnetic separation was not possible because of the absence of Fe particles. Results indicate that the presence of Fe/Ca/PO4 resulted in better magnetic separation. This fact indicates that the magnetic separated fraction described above contains highly condensed heavy metals (i.e., 30 wt% and 25 wt% of magnetic fraction fly ash contained about 85% and 91% of heavy metals). The nonmagnetic fraction fly ash contains extremely low concentrations of heavy metals (i.e., 70 wt% and 75 wt% of nonmagnetic fraction fly ash contained about 15% and 9% of heavy metals) (). In this way, highly heavy metal–contaminated fly ash volume can be reduced.
Table 2. Total heavy metal concentrations (mg/L) in fly ash samples before and after treatment.
Figure 2. Magnetic and nonmagnetic separated fractions of fly ash and its condensed heavy metals, As, Cd, Cr, and Pb, after treatment with various additives.
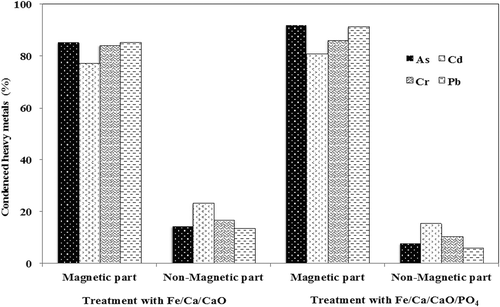
The concentrations of heavy metals in the eluted solution after treatment with nano-Fe/Ca/CaO and nano-Fe/Ca/CaO/PO4 are presented in . All values presented in the table are averages of three replications. By simple grinding, As, Cd, Cr, and Pb eluted concentrations that respectively decreased from 5.97, 2.6, 4.85, and 6.72 mg/L to 1.0, 0.96, 1.8, and 1.02 mg/L. However, when nano-Fe/Ca/CaO and nano-Fe/Ca/CaO/PO4 were added during grinding, leaching of heavy metals decreased (). The eluted concentrations of heavy metals corresponded to intrinsic heavy metal immobilization efficiencies of about 98–100% with nano-Fe/Ca/CaO/PO4 grinding treatment. Furthermore, it might be readily apparent from that the leachable heavy metal concentrations either in the magnetic or the nonmagnetic fly ash fraction was much lower than the Japan leachate standard regulatory threshold, <0.01 mg/L for As, Cd, and Pb and 0.05 mg/L for Cr, respectively (JLT-13). In fact, in our previous work examining heavy metal–contaminated soil, pH dependence test/acid resistance, and salt penetration properties of the final products were investigated to assess the product durability once landfilled. To ascertain the resistance to acid/alkaline penetration, the solidified soil specimen sample solutions were adjusted to pH 3/pH 12 using concentrated HCl/NaOH during the elution (JLT-13) test. Similar heavy metal immobilization efficiency was observed with soils including 5% and 10% moisture and at different pH (i.e., pH 3 and pH 12) conditions (Mallampati et al., Citation2012b).
Table 3. Eluted heavy metal concentrations (mg/L) by leaching test in fly ash after various treatments.
Compared with other solidification and stabilization (S/S) processes that use additive or binder to chemically or physically immobilize the hazardous contents present in waste (Wiles, Citation1996; Quina et al., Citation2014), this treatment processes is a simple, short-term, and inexpensive process. Many countries already use the addition of Portland cement for S/S. However, an important shortcoming is that this process is unsuitable for treating soluble salts. Long-term leaching can be anticipated as an environmental problem. In addition, the waste volume will be increased (almost doubled) using this method. Consequently, this method is much more suitable for treating highly toxic waste materials (Alba et al., Citation2001; Auer et al., Citation1995). A new integrated stabilization technique was developed in four stages: elimination of alkali chlorides by dissolution; addition of phosphoric acid; calcination; and solidification with cement. This technique can destroy toxic organics, reduce heavy metal reactivity, and solidify hazardous waste without exceeding the leaching limit (Derie, Citation1996; Lam et al., Citation2010). A combined washing-immobilization process for treating FA was investigated by Mangialardi (Citation2003). The water washing treatment can remove large amounts of chloride and sulfate from FA, promote the formation of a hydrate phase, and convert heavy metals into less reactive forms. Then, the wastewater can be treated by reducing the pH to 6.5–7.5. Precipitation of aluminum hydroxide occurs, with subsequent adsorption of cadmium, lead, and zinc ions onto the floc particles of aluminum hydroxide. The treated fly ash is then incorporated into cement for disposal (Mangialardi, Citation2003).
However, based on our current field study observations, treating 1 t of contaminated fly ash using the nano-Fe/Ca/CaO technique would cost approximately 4,500 Japanese yen (JPY)/t (about 40 USD) (the nano-Fe/Ca/CaO addition to polluted soil ratio would be approximately 1:10), which is a much lower cost than those associated with cement-based solidification processes. Indeed, these costs can reach values of 80–380 USD/t in the USA or 80–150 euro/t in Europe (Mallampati et al., Citation2012b). Aside from the lower cost, another benefit of the treatment presented herein is that solidification occurs quickly, in about 2 hr, using only naturally existing moisture, whereas cement-based process solidification/stabilization requires a longer time (at least 2 days), and requires excess water to solidify (engendering additional costs for secondary effluent treatment). In addition to its excellent immobilization capacity for heavy metals in FA, the nano-Fe/Ca/CaO system is effective for trapping other persistent organic pollutants such as polychlorinated biphenyls (PCBs) in different waste matrices, including FA (Mitoma et al., Citation2011). These results appear to be extremely promising. The addition of nano-Fe/Ca/CaO/PO4 mixture with a simple grinding technique might be regarded as potentially applicable for the remediation and volume reduction of contaminated fly ash by heavy metals.
Immobilization and separation mechanism of heavy metals
Semiquantitative SEM-EDS analysis clarified that As, Cd, Cr, and Pb amounts in the fly ash surface decreased after nano-Fe/Ca/CaO/PO4 treatment (in both magnetic and nonmagnetic fly ash fractions) (). These decreased mass percentages correspond to 99% Cr, 100% As, 99.6% Cd, and 99.7% for Pb. However, the Ca and Fe mass percentages respectively increased about 13% and 20%. As shown in the SEM-EDS elemental maps, the magnetic part shows high concentrations of heavy metals, although the nonmagnetic fraction shows low concentrations (). This fact, along with the previous observations (highest amounts of entrapped heavy metals are present in the lowest amounts of magnetically separated fly ash fraction), suggests that the magnetic separated fraction includes highly concentrated heavy metals (). The disappearance of heavy metals from the fly ash particle surface, correlated with the absence of heavy metal leachate, is an indication that the initial quantity of heavy metals is immobilized inside the enclosed particles with nano-Fe/Ca/CaO/PO4 treatment. Consequently, heavy metals (As, Cd, Cr, and Pb) are brought into the immobile Ca/PO4 salts.
Table 4. Scanning electron microscope combined with energy-dispersive X-ray spectrometry (SEM-EDS), semiquantitative analysis before and after treatment with nano-Fe/Ca/CaO and Fe/Ca/CaO/PO4 mixtures.
Figure 3. SEM-EDS elemental maps clearly representing the fact that the magnetic part shows high concentration of heavy metals whereas nonmagnetic fraction shows low concentration after treatment with nano-Fe/Ca/CaO/PO4.
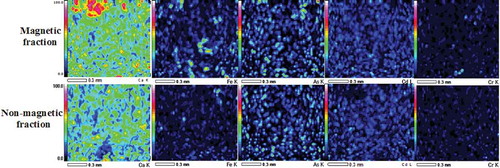
The most probable mechanisms for the enhanced heavy metal immobilization capacity with nano-Fe/Ca/CaO/PO4 treatment might result from adsorption and entrapment of heavy metals into newly formed aggregates because of the aggregation of fly ash particles and enclosure/binding with Ca-associated immobile salts and their separation, as portrayed schematically in . One can assume that when fly ash is contaminated, heavy metals are adsorbed onto fly ash particles through a surface coordination process (). By simple grinding, fly ash particles decrease to fine clay or silt particles. Therefore, the particle surface area increases and the heavy metal mobility decreases because the adsorption area increases (Orumwense, Citation2006). However, during grinding with nano-Fe/Ca/CaO/PO4 additives, fly ash particles are subjected to collisions that are expected to promote aggregation and breakage phenomena (Montinaro et al., Citation2007). When aggregation occurs in the presence of nano-Fe/Ca/CaO/PO4, the heavy metals adsorbed onto the surface of two overlapping particles might be entrapped within the newly formed aggregates. However, nano-Fe/Ca/CaO/PO4 can produce immobile salts with moisture and CO2 in atmosphere, including pozzolanic cement and hydraulic property. Therefore, the fly ash surfaces might enclose/bind with Ca/[PO4]/H2O/[CO3] association compounds (e.g., apatite, Ca10(PO4)6(OH)2); calcium carbonate, CaCO3; or calcium hydroxide, Ca(OH)2) () (Mallampati et al., Citation2012a, Citation2014). The X-ray diffraction (XRD) patterns of fly ash before treatment consisted mainly of a glassy phase and some minerals with crystalline phases of mullite and quartz (SiO2), magnetite (Fe3O4), anorthite (CaAl2Si2O8), and hematite (Fe2O3). When the same fly ash was subjected to mixing with nanometallic Ca/CaO/PO4, the peaks related to Ca/PO4, crystalline phases of portlandite (Ca(OH)2), and calcite (CaCO3)/Ca10(PO4)6(OH)2) appeared, in addition to quartz, magnetite, anorthite, and hematite, which probably indicates that most of the hydrates were crystallized and the main fraction of enclosed/bound materials included Ca/PO4-associated crystalline complexes (Lee et al., Citation2015). As a result, heavy metals (As, Cd, Cr, and Pb) would be brought into the immobile Ca/PO4 salts.
Figure 4. Schematic representation of possible mechanisms that may determine the remediation and separation of heavy metal–contaminated fly ash after simple mixing in dry conditions: (a) fly ash before treatment, heavy metal adsorption, and nano-Fe/Ca/CaO/PO4 addition; (b) fly ash surface covered/enclosed/heavy metals immobilization; and (c) high and low concentrated heavy metal fly ash separation by magnet.
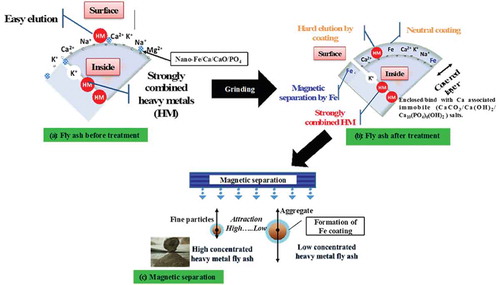
Furthermore, the controlling mechanism is a function of the standard redox potential of the contaminant metal in the presence of nano-iron (Fe). Heavy metals are either reduced at the nano-Fe surface, as Cu2+ or Ag2+, or are adsorbed directly to the nano-Fe surface where they are rendered immobile, as Zn2+, Cd2+, Cr6+, Cu2+, Pb2+, As3+, etc. (Jegadeesan et al., Citation2005; Kanel et al., Citation2005; Uzum et al., Citation2009). The standard redox potential of zerovalent iron is (−0.41 V), extremely close to that of Cd2+ (−0.40 V), Cr3+ (−0.42 V), Pb2+ (−0.13 V), and As3+ (−0.23 V). Therefore, the removal of As, Cd, Cr, and Pb ions by nano-Fe results from sorption (Li and Zhang, Citation2007). Moreover, the magnetic properties of nano-Fe facilitate the rapid separation of nano-Fe from fly ash, via a magnetic field (Liu et al., Citation2009; Yavuz et al., Citation2006). In this way, encapsulated nano-Fe particles in solidified (smaller/finer and larger/aggregate) fly ash fractions can also be separated (). Consequently, the grinding treatment of contaminated fly ash with nano-Fe/Ca/CaO/PO4 can reduce heavy metal leachability with the minimization of their solubility by the reduction of heavy metals, or fixing by adsorption, and precipitation of heavy metals. The amount of heavy metals exposed to leaching is reduced. Enhancing high immobilization and volume reduction (by separation) of heavy metals in contaminated fly ash was achieved with nano-Fe/Ca/CaO/PO4 treatment.
Conclusion
This study evaluated a nano-Fe/Ca/CaO/PO4 dispersion mixture as an immobilization treatment for heavy metal (As, Cd, Cr, and Pb)–contaminated fly ash and its separation. After treatment with nano-Fe/Ca/CaO and nano-Fe/Ca/CaO/PO4, about 30 wt% and 25 wt% of magnetic fraction fly ash were separated. Its condensed heavy metal concentrations were about 85% and 91%, respectively. Grinding with nano-Fe/Ca/CaO/PO4 could achieve about 98–100% heavy metal immobilization. The leachable heavy metal concentrations were reduced to concentrations lower than the Japan hazardous waste leaching standard regulatory threshold. The results obtained from this study suggest that the nano-Fe/Ca/CaO/PO4 mixture is a suitable treatment for the gentle immobilization and its volume reduction of heavy metals in contaminated fly ash.
Funding
The authors are grateful to the Japan Society for the Promotion of Science (JSPS), Tokyo, Japan (Project IDs: 24310059 and 25550058), and the National Research Foundation of Korea (NRF), Seoul, Korea, for providing financial support for this study through the Basic Science Research Program funded by the Ministry of Education (2014R1A1A2055487).
Additional information
Funding
Notes on contributors
Srinivasa Reddy Mallampati
Srinivasa Reddy Mallampati is an assistant professor at the Department of Civil and Environmental Engineering, University of Ulsan, Ulsan, Republic of Korea.
Yoshiharu Mitoma
Yoshiharu Mitoma is an associate professor at the Department of Environmental Sciences, Prefectural University of Hiroshima, Shobara City, Japan.
Cristian Simion
Cristian Simion is a professor at the Department of Organic Chemistry, Politehnica University of Bucharest, Bucharest, Romania.
Byoung Ho Lee
Byoung Ho Lee is a professor at the Department of Civil and Environmental Engineering, University of Ulsan, Ulsan, Republic of Korea.
References
- Alba, N., E. Vázquez, S. Gasso, and J.M. Baldasano. 2001. Stabilization/solidification of MSW incineration residues from facilities with different air pollution control systems. Durability of matrices versus carbonation. Waste Manage. 21:313–323. doi:10.1016/S0956-053X(00)00082-9
- Auer, S., H.J. Kuzel, H. Pollmann, and F. Sorrentino. 1995. Investigation on MSW fly ash treatment by reactive calcium aluminates and phases formed. Cem. Concr. Res. 25:1347–1359. doi:10.1016/0008-8846(95)00127-X
- Baker, D.E., and M.C. Amacher. 1982. Nickel, Copper, zinc, cadmium. In Methods of Soil Analysis, Part II. Chemical and Microbiological Properties, ed. R.H. Miller and D.R. Keeney, 323–334. Madison, WI: American Society of Agronomy–Soil Science Society of America.
- Boghetich, G., L. Liberti, M. Notarnicola, M. Palma, and D. Petruzzelli. 2005. Chloride extraction for quality improvement of municipal solid waste incinerator ash for the concrete industry. Waste Manage. Res. 23:57–61. doi:10.1177/0734242X05051017
- Boparai, H.K., M. Joseph, and D.M.O. Carroll. 2001. Kinetics and thermodynamics of cadmium ion removal by adsorption onto nano zerovalent iron particles. J. Hazard. Mater. 186:458–465. doi:10.1016/j.jhazmat.2010.11.029
- Cahill, C.A., and L.W. Newland. 1982. Comparative efficiencies of trace metal extraction from municipal incinerator ashes. Int. J. Environ. Anal. Chem. 11:227–239. doi:10.1080/03067318208078314
- Chou, J.D., W.Y. Ming, H.H. Liang, and S.H. Chang. 2009. Biotoxicity evaluation of flyash and bottom ash from different municipal solid waste incinerators. J. Hazard. Mater. 168:197–202. doi:10.1016/j.jhazmat.2009.02.023
- Derie, R. 1996. A new way to stabilize fly ash from municipal incinerators. Waste Manage. 16:711–716. doi:10.1016/S0956-053X(97)00013-5
- Ecke, H., N. Menad, and A. Lagerkvist. 2003. Carbonation of municipal solid waste incineration fly ash and the impact on metal mobility. J. Environ. Eng. 129:435–440. doi:10.1061/(ASCE)0733-9372(2003)129:5(435)
- Helmke, P.A., and D.L. Sparks. 1996. Lithium, sodium, potassium, rubidium, and cesium. In Methods of Soil Analysis, Part 3—Chemical Methods, ed. D.L. Sparks, 551–574. SSSA Book Series No. 5. Madison, WI: American Society of Agronomy–Soil Science Society of America.
- Hong, K.J., S. Tokunaga, Y. Ishigami, and T. Kajiuchi. 2000. Extraction of heavy metals from MSW incinerator fly ash using saponins. Chemosphere 41:345–352. doi:10.1016/S0045-6535(99)00489-0
- Hong, K.J., S. Tokunaga, and T. Kajiuchi. 2000. Extraction of heavy metals from MSW incinerator fly ash by chelating agents. J. Hazard. Mater. 75:57–73. doi:10.1016/S0304-3894(00)00171-0
- Japanese Leaching Test (JLT-13). Environment Agency Notification No. 13. Tokyo: Japan.
- Jegadeesan, G., K. Mondal, and S.B. Lalvani. 2005. Arsenate remediation using nanosized modified zerovalent iron particles. Environ. Prog. 24:289–296. doi:10.1002/ep.10072
- Jiang, Y.H., B.D. Xi, X.J. Li, L. Zhang, and Z.M. Wei. 2009. Effect of water-extraction on characteristics of melting and solidification of flyash from municipal solid waste incinerator. J. Hazard. Mater. 161:871–877.
- Jianguo, J., W. Jun, X. Xin, W. Wei, D. Zhou, and Z. Yan. 2004. Heavy metal stabilization in municipal solid waste incineration fly ash using heavy metal chelating agents. J. Hazard. Mater. B113:141–146.
- Jianguo, J., and W. Wei. 1998. The history, present status and development of solidification/stabilization technology in treating with hazardous waste. Adv. Environ. Sci. 6:55–59.
- Jung, C.H., T. Matsuto, N. Tanaka, and T. Okada. 2004. Metal distribution in incineration residues of municipal solid waste (MSW) in Japan. Waste Manage. 24:381–391. doi:10.1016/S0956-053X(03)00137-5
- Kanel, S.R., B. Manning, L. Charlet, and H. Choi. 2005. Removal of arsenic(III) from groundwater by nanoscale zero valent iron. Environ. Sci. Technol. 39:1291–1298. doi:10.1021/es048991u
- Lam, C.H.K., A.W.M. Lp, J.P. Barford, and G. McKay. 2010. Use of incineration MSW ash: A review. Sustainability 2:1943–1968. doi:10.3390/su2071943
- Lauf, R.J. 1985. Characterization of the mineralogy and microchemistry of fly ash. Nucl. Chem. Waste Manage. 5:231–236. doi:10.1016/0191-815X(85)90082-8
- Lee, C.H., N.T.T. Truc, B.K. Lee, Y. Mitoma, and S.R. Mallampati. 2015. Evaluation of heavy metals in hazardous automobile shredder residue thermal residue and immobilization with novel nano-size calcium dispersed reagent. J. Hazard. Mater. 296:239–247. doi:10.1016/j.jhazmat.2015.04.039
- Li, X.Q., and W.X. Zhang. 2007. Sequestration of metal cations with zerovalent iron nanoparticles—A study with high resolution X-ray photoelectron spectroscopy (HR-XPS). J. Phys. Chem. C 111:6939–6946. doi:10.1021/jp0702189
- Liu, Q.Y., L.Y. Bei, and F. Zhou. 2009. Removal of lead(II) from aqueous solution with amino-functionalized nanoscale zero-valent iron. Cent. Eur. J. Chem. 7:79–82. doi:10.2478/s11532-008-0097-1
- Mallampati, S.R., Y. Mitoma, T. Okuda, S. Sakita, and M. Kakeda. 2012a. High immobilization of soil cesium using ball milling with nano-metallic Ca/CaO/NaH2PO4: Implications for the remediation of radioactive soils. Environ. Chem. Lett. 10:201–207. doi:10.1007/s10311-012-0357-3
- Mallampati, S.R., Y. Mitoma, T. Okuda, S. Sakita, and C. Simion. 2014. Preferential removal and immobilization of stable and radioactive cesium in contaminated fly ash with nanometallic Ca/CaO methanolsuspension. J. Hazard. Mater. 279:52–59. doi:10.1016/j.jhazmat.2014.06.044
- Mallampati, S.R, T. Okuda, Y. Mitoma, S. Sakita, and M. Kakeda. 2012b. Enhanced heavy metal immobilization in soil by grinding with addition of nanometallic Ca/CaO dispersion mixture. Chemosphere 89:717–723. doi:10.1016/j.chemosphere.2012.06.030
- Mangialardi, T. 2003. Disposal of MSWI fly ash through a combined washing-immobilisation process. J. Hazard. Mater. 98:225–240. doi:10.1016/S0304-3894(02)00359-X
- Mitoma, Y., H. Miyata, N. Egashira, A. Simion, M. Kakeda, and C. Simion. 2011. Mechanochemical degradation of chlorinated contaminants in fly ash with a calcium-based degradation reagent. Chemosphere 83:1326–1330. doi:10.1016/j.chemosphere.2011.04.015
- Montinaro, S., A. Concas, M. Pisu, and G. Cao. 2007. Remediation of heavy metals contaminated soils by ball milling. Chemosphere 67:631–639. doi:10.1016/j.chemosphere.2006.11.009
- Nakane, H. 2012. Translocation of particles deposited in the respiratory system: A systematic review and statistical analysis. Environ. Health Prev. Med. 17:263–274. doi:10.1007/s12199-011-0252-8
- Okada, T., Y. Tojo, N. Tanaka, and T. Matsuto. 2007. Recovery of zinc and lead from fly ash from ash-melting and gasification-melting processes of MSW—Comparison and applicability of chemical leaching methods. Waste Manage. 27:69–80. doi:10.1016/j.wasman.2005.12.006
- Ontiveros, J.L., T.L. Clapp, and D.S. Kosson. 1989. Physical properties and chemical species distributions within municipal waste combuster ashes. Environ. Prog. 8:200–206. doi:10.1002/ep.3300080319
- Orumwense, A.O. 2006. Effect of media type on regrinding with stirred mills. Miner. Metall. Process. 23:40–44.
- Polettini, A., R. Pomi, P. Sirini, and F. Testa. 2001. Properties of Portland cement-stabilized MSWI fly ashes. J. Hazard. Mater. 88:123–138. doi:10.1016/S0304-3894(01)00292-8
- Quina, M.J., J.C.M. Bordado, and R.M. Quinta-Ferreira. 2014. Stabilisation/solidification of APC residues from MSW incineration with hydraulic binders and chemical additives. J. Hazard. Mater. 264:107–116. doi:10.1016/j.jhazmat.2013.11.014
- Sakai, S.I. 1996. Municipal solid waste management in Japan. Waste Manage. 16:395–405. doi:10.1016/S0956-053X(96)00107-9
- Santanu, P., and K. Pak. 2006. Solidification-stabilization of organic and inorganic contaminants using Portland cement: A literature review. Environ. Rev. 14:217–255.
- Sawada, K., H. Matsuda, and M. Mizutani. 2001. Immobilization of lead compounds in fly ash by mixing with asphalt, sulfur and sodium hydroxide. J. Chem. Eng. Japan 34:878–883. doi:10.1252/jcej.34.878
- Shim, I., E. Oh, S. Yang, T. Ryu, J. Soh, D. Sul, and P. Kim. 2012. Subacute inhalation toxicity assessment of fly ash from industrial waste incinerators. Inhal. Toxicol. 24:741–750. doi:10.3109/08958378.2012.716869
- Sukandar, T. Padmi, T. Masaru, and I. Aoyama. 2009. Chemical stabilization of medical waste fly ash using chelating agent and phosphates: Heavy metals and ecotoxicity evaluation. Waste Manage. 29:2065–2070. doi:10.1016/j.wasman.2009.03.005
- Uzum, C., T. Shahwan, A.E. Eroglu, K.R. Hallam, T.B. Scott, and I. Lieberwirth. 2009. Synthesis and characterization of kaolinite-supported zero-valent iron nanoparticles and their application for the removal of aqueous Cu2+ and Co2+ ions. Appl. Clay Sci. 43:172–181. doi:10.1016/j.clay.2008.07.030
- Wang, L., Y.Y. Jin, R.D. Li, and Y.F. Nie. 2010. Characterization of MSWI fly ash. Environ. Sci. Technol. 33:21–26.
- Wang, Q.H., J. Yang, Q. Wang, and T.J. Wu. 2009. Effects of water washing pretreatment on bioleaching of heavy metals from municipal solid waste incinerator fly ash. J. Hazard. Mater. 162:812–818. doi:10.1016/j.jhazmat.2008.05.125
- Wiles, C.C. 1996. Municipal solid waste combustion ash: State-of-the-knowledge. J. Hazard. Mater. 47:325–344. doi:10.1016/0304-3894(95)00120-4
- Yang, S., A. Saffarzadeh, T. Shimaoka, and T. Kawano. 2014. Existence of Cl in municipal solid waste incineration bottom ash and dechlorination effect of thermal treatment. J. Hazard. Mater. 267:214–220. doi:10.1016/j.jhazmat.2013.12.045
- Yavuz, C.T., J.T. Mayo, W.W. Yu, A. Prakash, J.C. Falkner, S. Yean, L.L. Cong, H.J. Shipley, A. Kan, M. Tomson, D. Natelson, and V.L. Colvin. 2006. Low-field magnetic separation of monodisperse Fe3O4 nano crystals. Science 314:964–967. doi:10.1126/science.1131475
- Ying, X., Y. Chen, and Y. Feng. 2013. Stabilization treatment of the heavy metals in fly ash from municipal solid waste incineration using diisopropyl dithiophosphate potassium. Environ. Technol. 34:1411–1419.