Abstract
Sugar beet pulp residues (SBPR) from hydrolysis and dewatering of beet pulp were co-digested with municipal sewage sludge (MSS). The highest biogas yields of nearly 512 dm3/kg VSfed (volatile solids fed) were achieved for SBPR, treated both as the monosubstrate and as a mixture with MSS (1 : 1 by weight). Simultaneously, the highest methane production of 348 dm3 CH4/kg VSfed was determined when the sewage sludge was co-digested with 35% SBPR. The analysis of digestate showed that neither ammonia nor volatile fatty acids destabilized the biogas production.
Implications: Processing of sugar beet pulp into bioethanol via enzymatic hydrolysis and microbial fermentation has become increasingly attractive. However, in this process, only the liquid fraction derived from hydrolysis is subjected into alcoholic fermentation, whereas the remaining solid fraction needs to be utilized. This study demonstrated that sugar beet pulp residues after bioethanol production can successfully be co-digested with sewage sludge to increase biogas productivity of anaerobic digesters located at wastewater treatment plants.
Introduction
Rising concern over depletion of fossil fuels and greenhouse gas limits has resulted in great interest in alternative fuels produced from renewable resources, including sugars, starches, and lignocellulosic biomass. The latter materials represent one of the most promising feedstocks due to their great availability and low cost (Balat et al., Citation2008). Among lignocellulosic biomass, sugar beet pulp (SBP) seems to be a feedstock of particular interest because it contains as much as 85% of carbohydrates (cellulose, hemicelluloses, pectin, and others) (Zheng et al., Citation2012). Sugar beet pulp is an important by-product of the sugar industry and represents the fibrous portion of sugar beet after extraction of water-soluble components. According to the Central Statistical Office, in 2013 the yield of sugar beets in Poland exceeded 112 million tonnes. This gives around 37 million tonnes of SBP assuming that 1 ton of the processed sugar beet generates approximately 0.33 tonne of beet pulp (Fang et al., Citation2011). At present, in most sugar factories SBP is dehydrated, palletized, and utilized for animal nutrition. However, due to a relatively low protein content compared with the requirements of most ruminants, sugar beet pulp has to be supplemented with an extra protein source (Panagiotopoulos et al., Citation2010). Moreover, 30–40% of the overall energy cost of sugar beet processing is devoted to dehydrating and pelletizing the pulp (Doran and Foster, Citation2000; Zheng et al., Citation2012). Consequently, the disposal of SBP as animal feed is often unprofitable, and sugar factories are increasingly using the pulp for the production of biofuels, including bioethanol and biogas. Processing of sugar beet pulp into fuel ethanol via enzymatic hydrolysis and microbial fermentation has become increasingly attractive (Donkoh et al., Citation2012; Doran and Foster, Citation2000; Ivetic et al., Citation2012; Zheng et al., Citation2012). High carbohydrate and low lignin contents determine an effective bioconversion of SBP into bioethanol with minimal energy consumption. In this process, either only the liquid fraction derived from the sugar beet pulp hydrolysis (rich in monosugars) is subjected into alcoholic fermentation, or bioethanol is produced from the whole mass of hydrolyzed SBP. In both cases, the remaining solid fraction (hereinafter referred to as sugar beet pulp residue, SBPR) needs to be further utilized.
There are numerous studies in the literature regarding the anaerobic digestion of sugar beet pulp (Brooks et al., Citation2008; Hutnan et al., Citation2000; Koppar and Pullammanappallil, Citation2008; Stoyanova et al., Citation2014; Suhartini et al., Citation2014). The literature also describes the co-digestion of SBP with other organic wastes, including cow manure (Fang et al., Citation2011), sewage sludge (Montanes et al., Citation2013), starch potato processing waste (Kryvoruchko et al., Citation2009), and sugar processing wastewater (Alkaya and Demirer, Citation2011).
However, to the best of the authors’ knowledge, there are no studies on the anaerobic treatment of sugar beet pulp residues after bioethanol production. Therefore, in the following study, the SBPR after enzymatic hydrolysis and dewatering of beet pulp were investigated in order to establish their potential for biogas production. Furthermore, the SBPR were also co-digested with municipal sewage sludge in order to recognize the potential benefits of co-digestion as well as separate digestion of these wastes.
Apart from obvious merits of co-digestion such as the improved carbon-to-nitrogen (C/N) balance of the mixture, higher biogas and methane yield, or dilution of potential toxic substances, the treatment of SBPR together with sewage sludge may be beneficial because most biogas installations in Poland are located at municipal wastewater treatment plants, and the digesters are often oversized. Also, sugar beet pulp and hence SBPR are produced only during a short-term campaign and therefore have to be utilized as rapidly as possible. Otherwise, the storage and the ensiling of a substrate with high moisture and low pH will increase the costs for the operation of the biogas plant (Stoyanova et al., Citation2014). Therefore the co-digestion of SBPR with municipal sewage sludge seems to be a reasonable approach.
Methods
Materials
Sugar beet pulp residues were obtained as a by-product of enzymatic hydrolysis and dewatering of sugar beet pulp, collected from the Dobrzelin Sugar Factory, which is a part of the National Sugar Company (KSC), Poland. After hydrolysis of SBP, the released liquid fraction, rich in soluble sugars, was used for bioethanol production, performed in a separate investigation within the same research project, whereas the dewatered SBPR were investigated in this study.
The hydrolysis of SBP was performed in a specially manufactured stainless steel tank with a 3-m3 active volume. The reactor was equipped with a mixing system and a heating mantle to provide a constant temperature of 50 °C. A mixture (1:1) of two commercial preparations: Viscozyme and UltrafloMax, produced by Novozymes Corporation (Bagsvaerd, Denmark), were used to hydrolyze carbohydrates within the sugar beet pulp. Both products are composed of a combination of enzymes with cellulase, xylanase, pectinase, and invertase activities. The reaction mixture during enzymatic saccharification contained 250 kg of SBP (50 kg total solids [TS]), 300 dm3 of water, and 2.7 dm3 of each preparation. After 24 hr of saccharification, the hydrolyzate was heated to 80 °C in order to inactivate potential microbiological infections before filtering. Then, the hydrolyzate was filtered to obtain 375 dm3 of liquid fraction and 175 g of insoluble SBPR, and the latter was then used for anaerobic digestion (AD) experiments.
Mixed sewage sludge (MSS) used for the experiments was delivered from the Municipal Wastewater Treatment Plant at Łódź, serving a population equivalent of 820,000 inhabitants. The sludge was composed of primary and secondary sewage sludge in the average volume proportion of 1:1.
Experiments
Batch experiments were carried out to determine the biochemical methane and biogas potential of individual substrates. Each biomethane potential (BMP) test was performed in a 1-dm3 glass bottle, which was connected to a 1-dm3 gas collecting tank to provide anaerobic conditions and to measure the biogas yield by a water displacement method. For the purpose of batch experiments, the anaerobically digested sewage sludge, collected from the Municipal Wastewater Treatment Plant in Łódź, was used as inoculum. Each reactor was initially filled with a 500-cm3 batch of the inoculum and then the substrate was added to achieve an inoculum-to-substrate ratio of 2:1 based on volatile solids (VS) contents. The inoculum-to-substrate (I/S) ratio of 2 is suggested as mandatory for standardized BMP test, since it has never been reported as inhibitory for methane production (Cabbai et al., Citation2013; Esposito et al., Citation2012). Then, the headspace of each reactor was purged with nitrogen prior to its closing and connecting to the biogas collecting tank. The batch reactors were maintained at 35 °C in a thermostat. They were shaken two times per day, and the volume of biogas produced was recorded every day. The duration of experiments was determined by the point at which biogas production stopped completely. All batch experiments were performed in triplicate; additionally, three blanks with only 500 cm3 of inoculum were investigated, to determine the gas productivity of the inoculum.
Semicontinuous experiments were performed in a laboratory reactor with a 3-dm3 active volume. The reactor was coupled with a 4-dm3 gas collecting tank to enable strict anaerobic conditions and to control the biogas yield by a water displacement method. The digester was fed once a day (a semicontinuous operation) using a peristaltic pump. Prior to each feeding, a volume equal to the feeding volume was removed from the reactor to maintain constant volume. At startup, the reactor was filled with inoculum (the same as in case of batch experiments) and then operated with sewage sludge at a solids retention time (SRT) of 30 days for a month to provide a suitable acclimation of anaerobic biomass prior to experiments.
Each experimental run was continued for at least three consecutive SRTs under steady-state conditions, characterized by stable biogas production and relatively constant pH and volatile fatty acid concentrations throughout the run.
Analyses
The analyses of total and volatile solids, chemical oxygen demand (COD), total alkalinity (TAL), and pH were performed according to standard methods (American Public Health Association [APHA], Citation2005). The total ammonium nitrogen (TAN), free ammonia (FAN), orthophosphates (PO4), and total volatile fatty acids (TVFA) were analyzed using a DR2800 spectrophotometer (Hach Lange, Düsseldorf, Germany) and Hach Lange tests as previously described (Borowski and Weatherley, Citation2013). Individual volatile fatty acids were also quantified with a high-performance liquid chromatography (HPLC) using a Finnigan Surveyor chromatograph (Thermo Scientific, San Jose, CA, USA) coupled with an Aminex HPX 87H column (Bio-Rad, Hercules, CA, USA) and a refractive index detector. Separation during the HPLC tests was performed using a mobile phase of sulfuric acid (5 mmol/L) at a flow rate of 0.6 cm3/min.
Elemental analysis (C, N, P, H, and S) was performed with a NA 2500 elemental analyzer (CE Instruments, Wigan, UK).
Cellulose content was determined by the Kürschner-Hoffer method, which relies on the treatment of the sample with a hot concentrated mixture of HNO3 and C2H5OH with a total duration of 4 hr, wherein the lignin is converted into nitrolignin, which is soluble in ethanol (Kürschner and Hoffer, Citation1967).
Hemicellulose content was determined according to the Ermakov method in which hemicelluloses were sugared by a 2% solution of H2SO4 and then the resulting sugars were quantified by Miller method (Arashimovich and Ermakov, Citation1987).
The concentrations of acid-soluble lignin (ASL) and acid-insoluble lignin (AIL) in SBPR were determined by Laboratory Analytical Procedures (LAPs) from the National Renewable Energy Laboratory (NREL) (Ehrman, Citation1996; Templeton and Ehrman, Citation1995). Pectin content was analyzed according to the method described by Concha et al. (Citation2013).
The concentrations of mono- and disaccharides in the liquid fraction of SBPR were measured using a high-performance liquid chromatography (HPLC; Waters 600S; Milford, MA). The HPLC system was equipped with a Waters 717 autosampler and a light scattering detector (PrepELS II; Gibson, Middleton, WI). Separation during HPLC measurement was performed using a Rezex column (Phenomenex, Torrance, CA, USA) consisting of cross-linked sulfonated styrene-divinylbenzene copolymer in the calcium form, and deionized water as a mobile phase. Prior to the HPLC analysis, the SBPR samples were centrifuged (Rotofix 32 laboratory centrifuge; Hettich, Tuttlingen, Germany) at 4226 × g for 10 min and then filtered to obtain the liquid fraction of SBPR.
The daily biogas production was measured by a water displacement method as described in numerous papers. An SRI Instrument (Torrance, CA) model 8610C gas chromatograph (GC) was used to analyze the content of methane in biogas. The chromatograph was equipped with a molecular sieve-packed column (1 m × 1/8 inch), a silica gel-packed column (1 m × 1/8 inch outer diameter [OD]) and a thermal conductivity detector (TCD). The column and detector temperatures were 60 and 150 °C, respectively. Helium was used as the carrier gas at a flow rate of 10 cm3/min.
The analyses of individual samples were performed in at least triplicates. The composition of effluent was determined once a week except for the pH, which was monitored daily. The calculations of averages, standard deviations, and the analysis of variance (ANOVA) were performed using Microsoft Excel 2007. A confidence level of 0.05 was selected for all statistical comparisons.
Results and Discussion
Characteristics of substrates
The compositions of SBPR and mixed sewage sludge are depicted in . SBPR differed considerably from sugar beet pulp. The former had a moister content of around 13%, with volatile solids constituting comprising of 86.5% total solids (TS), whereas a typical pressed beet pulp is denser and usually contains more than 90% of organics in its total solids. On the other hand, the concentrations of nitrogen and phosphorus in SBPR were higher than those of beet pulp as reported in the literature (Fang et al., Citation2011; Hutnan et al., Citation2000; Kryvoruchko et al., Citation2009; Stoyanova et al., Citation2014). However, the differences between SBPR and SBP are especially evident in terms of carbohydrate and lignin contents. Typically, sugar beet pulp consists of 20–30% cellulose, 22–36% hemicelluloses, 20–32% pectic substances, and 1–4% lignin (Donkoh et al., Citation2012; Hutnan et al., Citation2000; Ivetic et al., Citation2012), whereas the corresponding values for SBPR were much lower (except lignins). The SBPR contained 12.5% cellulose, 6.06% pectins, and as low as 0.48% hemicelluloses. This is consistent with the properties of enzymatic preparations used in this study, which are particularly active against hemicelluloses and pectins. These polymers are readily hydrolyzed because of their amorphous structures. A higher cellulose content in SBPR after hydrolysis can be linked to its strong, crystalline matrix structure additionally strengthened by the extensive hydrogen linkages among glucose molecules, which makes this polymer difficult to break (Balat, Citation2011; Limayem and Ricke, Citation2012). Furthermore, the rate of enzymatic hydrolysis of cellulosic materials decreases relatively quickly because glucose and primarily cellobiose released from cellulose strongly inhibit cellulases (Balat et al., Citation2008). It was also found that lignin components could affect hydrolysis of cellulose. Lignin can adsorb on to cellulases and generate a nonproductive enzyme attachment that limits the accessibility of cellulose to cellulose. Moreover, phenolic groups derived from the degradation of lignin considerably deactivate cellulolytic enzymes and therefore affect hydrolysis of cellulose (Limayem and Ricke, Citation2012). also depicts the composition of selected mono- and disaccharides in the liquid fraction of SBPR. The predominant monosaccharides determined were galacturonic acid, glucose, and arabinose, next to galactose and xylose found in lower amounts. This is in perfect agreement with the literature data according to which the total hydrolysis of beet pulp releases predominantly glucose, arabinose, and galacturonic acid, as the components of the main chains of cellulose, hemicelluloses, and pectins (Leijdekkers et al., Citation2013; Spagnuolo et al., Citation1997).
Table 1. Characteristics of mixed sewage sludge and sugar beet pulp residues used for the experiments.
The sewage sludge used in this study derived from the wastewater treatment plant, which operates with a biological nutrient removal system. Therefore, it displayed high quantities of nutrients—nitrogen and especially phosphorus—6.25% TS and 1.95% TS, respectively, and these figures were 2-fold and nearly 14-fold higher than the corresponding values for SBPR. Conversely, the concentrations of carbon and organic material were lower in MSS, in contrast to SBPR.
Batch experiments
Batch experiments were undertaken to establish the maximal biogas and methane yield from individual substrates. The results of these tests are illustrated in . A very low pH of SBPR justified the need for its adjustment prior to digestion. Therefore, batch experiments were performed using SBPR with no pH control as well as after the pH was adjusted to 7. The pH was adjusted using 30% solution of sodium hydroxide in a dose of 3.3 cm3/kg fresh beet pulp residues, which gave around 0.57 g Na/kg SBPR.
Table 2. Parameters of the batch digestion tests.
The lower methane yield obtained for beet pulp residues after pH adjustment, compared with the value for fresh SBPR, might be linked to a slight inhibition of methanogenesis by sodium ions. A moderate inhibition of methanogenesis is observed at sodium contents ranging between 3.5 and 5.5 g/dm3, whereas a concentration of 8.8 g/dm3 strongly inhibits the growth of methanogens during mesophilic digestion (Appels et al., Citation2008). On the other hand, the differences between biogas yields from pulp residues without pH control and after pH adjustment were insignificant (P = 0.882). Moreover, the biogas yields obtained in batch digestion are higher than the gas productions obtained in reactor experiments because the high contribution of inoculum dilutes potential toxic substances that could inhibit methanogenesis, provides necessary nutrients, and neutralizes low pH (Fang et al., Citation2011). Therefore, in semicontinuous operation, the digestion of highly acidic SBPR might not be possible without pH control.
Semicontinuous experiments
The batch tests confirmed a high biogas and methane potential of SBPR. However, in order to obtain accurate biogas production data and establish digestion stability criteria, semicontinuous reactor experiments need to be undertaken. In the following research, the semicontinuous process was divided into seven experimental periods during which various mixtures of sludge and SBPR were delivered to the reactor. Data of these trials are summarized in and and and . The biogas volume was monitored daily; however, for clarity, the points on the plots represent the mean values of the weekly productions. After the startup period, the reactor was operated over a period of 82 weeks. Initially sewage sludge was treated as monosubstrate with a solids retention time (SRT) of 20 days, which was then reduced to 15 days. The corresponding values of an organic loading rate (OLR) were 1.76 and 2.07 kg VS/m3·day, respectively. During that period, the specific biogas production (SGP) was 333 and 348 dm3/kg VSfed for experiments 1 and 2, respectively, and the corresponding values of specific methane production (SMP) were 255 and 261 dm3 CH4/kg VSfed. Similar biogas and methane yields were obtained from the mixtures of primary and secondary sludge in previous studies (Bolzonella et al., Citation2005; Borowski and Weatherley, Citation2013). Simultaneously, the average VS removal efficiency was relatively low and did not exceed 43%. Moderate biogas yield and VS removal rate obtained in runs R1 and R2 can be linked to the specific nature of sewage sludge, which was mainly composed of waste activated sludge. As stated in the literature, the anaerobic digestion of waste activated sludge usually gives biogas yields and VS reduction rates not exceeding 300 dm3/kg VSfed for and 35%, respectively, and this can be attributed to a high content of slowly biodegradable and inert material (e.g., cell walls) in that substrate (Athanasoulia et al., Citation2012; Bolzonella et al., Citation2005).
Table 3. Operating parameters and performances of the semicontinuous experiments.
Table 4. Characteristics of digestate from semicontinuous experiments.
Figure 1. Weekly average volumetric gas production rate (GPR) and specific biogas yield (SGP) reported during the semicontinuous experiments.
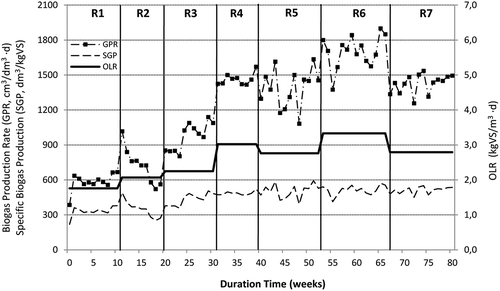
Figure 2. Changes in TVFA concentration and volatile fatty acid to alkalinity ratio reported in the semicontinuous experiments.
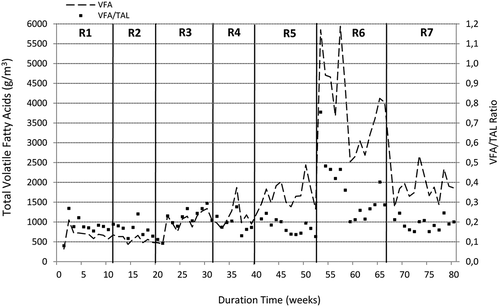
Figure 4. Changes in total and volatile solids concentrations during the semicontinuous experiments.
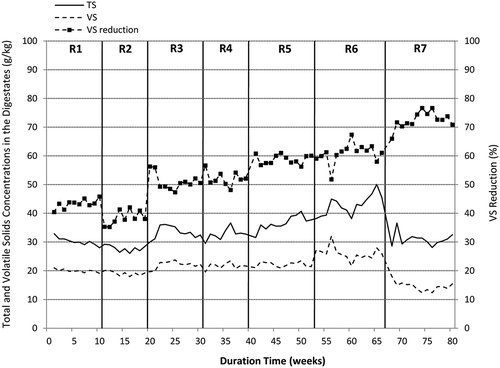
In the next two runs (R3 and R4), the reactor was operated with a mixture of sewage sludge and SBPR in a weight ratio of 80:20. For the corresponding SRT values, the OLR increased to 2.25 and 3.02 kg VS/m3·day, as a result of SBPR addition to the sludge. Moreover, since the beginning of the experiment R3, sodium hydroxide was regularly used in a dose of 0.75–1.5 g NaOH/kg SBPR to adjust the pH of pulp residues. As a result, the digester performed stable operation within the pH of over 7 during the whole study. A supplementation of sewage sludge with 20% SBPR resulted in a significant (P < 0.01) biogas and methane yield increase. At 20 days of SRT (run R3), the reactor exhibited SGP and SMP levels of 432 dm3/kg VSfed and 311 dm3 CH4/kg VSfed, respectively. These yields increased to 485 dm3/kg VSfed and 359 dm3 CH4/kg VSfed when the SRT value was reduced to 15 days (run R4). The average methane content of the digester biogas was around 71%; however, that value was lower by around 5 percentage points that the average methane content determined in biogas yielded from sewage sludge (). A further, significant (P < 0.01) increase in both biogas yield and VS removal rate was reported when sewage sludge was co-digested with 35% SBPR (experiment R5). In that period, the reactor was operated with an SRT of only 20 days due to a relatively high loading rate of 2.76 kg VS/m3·day, since a recommended value of OLR for wet fermentation processes treating energy crops and plant residues should not exceed 3.5 kg VS/m3·day (Weiland, Citation2010). The experiment R5 gave the greatest methane yield of 348 dm3 CH4/kg VSfed as a result of a relatively high SGP (over 500 dm3/kg VSfed) and still high methane content in biogas (nearly 70%). The corresponding VS removal rate was nearly 60%, compared with around 52% determined in run R4. In the run R6, the SBPR content in the digested mixture was increased to 50% (w/w) and the reactor was operated with an SRT of 20 days and an OLR of 3.33 kg VS/m3·day. During this period, the gas production rate as calculated per reactor volume (GPR) reached 1704 cm3/dm3·day (), which corresponded to the SGP of 512 dm3/kg VSfed and this yield was the highest reported in the whole study. Simultaneously, the specific methane yield was slightly lower than the one reported in experiment R5 due to a lower methane content in the biogas (66% on average). The VS removal rate did not change significantly (P = 0.08) compared with that reported in experiment R5. In the final period of the semicontinuous operation, the reactor was exclusively fed with SBPR, which were diluted with water to around 6.5% TS. In that run, the solids retention time and organic loading rate were established on 20 days and 2.79 kg VS/m3·day, respectively. The specific biogas yield was identical to that achieved in experiment R6, but the specific methane production significantly (P < 0.01) dropped to 295 dm3 CH4/kg VSfed due to the lower percentage of methane in the biogas stream (57.7% on average). Conversely, the volatile solids removal rate of nearly 74% was achieved for SBPR monodigestion, and this was the highest value recorded during the whole semicontinuous operation (, ). Therefore, one can notice that the more SBPR was added to the feed, the higher VS removal rate and the lower methane content of the biogas were achieved. Furthermore, with the increased SBPR contribution in the digesting mixture, the specific biogas production was also increasing but only until the experiment R6 in which the mixture of 50% pulp residues with sewage sludge (w/w) was treated. A full statistical comparison of specific biogas productions and volatile solids removal rates reported in semicontinuous experiments is summarized in . Due to the lack of available literature concerning the anaerobic digestion of beet pulp residues derived from enzymatic hydrolysis and dewatering of SBP, the results described in this study can be discussed with the reports on the anaerobic digestion and co-digestion of sugar beet pulp and similar wastes of plant origin. The specific biogas and methane yields from SBPR obtained in this study are comparable to the gas productions from beet pulp reported in the literature (Brooks et al., Citation2008; Fang et al., Citation2011; Stoyanova et al., Citation2014; Suhartini et al., Citation2014). Simultaneously, these authors also recorded the low percentage of methane in biogas (45–55%), which can be linked to the high sugar concentration and the low fat and protein contents in both substrates. The theoretical methane content in biogas produced from carbohydrates is about 50%, whereas the values for proteins and fats reach 70% and more (Weiland et al., Citation2010). Co-digestion of SBPR with sewage sludge did not increase the specific biogas production but considerably improved specific methane yield due to its greater content in the digester biogas. The highest methane production was achieved at 35% SBPR in the digested mixture (experiment R5), whereas the specific gas production in that experiment was only slightly lower than maximal value obtained in run R6. At 50% SBPR (experiment R6), the substrate might not have been fully utilized due to a possible overload of the digester. This can be supported by a relatively high TVFA concentrations of nearly 4000 g/m3 () in that experiment (see the next section). A similar observation was also described by Fang et al. (Citation2011). The co-digestion experiments can be compared with the results reported by Montanes et al. (Citation2013). The authors demonstrated the improved methane productivity in the system treating the mixture of sewage sludge with SBP compared with the monodigestion of beet pulp. For the same SRT of 20 days, the reported biogas and methane yields (1300 m3/m3·day, 230 dm3 CH4/kg COD, and around 70% CH4 in biogas) were similar to the corresponding values obtained in experiment R5 of our study.
Table 5. Statistical comparison of specific gas production and VS removal rates in the semicontinuous experiments (P-value matrix).
Behavior of ammonia and VFA during the semicontinuous experiments
The characteristics of digestate from semicontinuous experiments are depicted in , whereas variations in ammonium nitrogen, phosphates, and volatile fatty acids are illustrated in and . It is generally known, that the stability of anaerobic digestion mainly depends upon the concentrations of ammonia and volatile fatty acids. In the following study, the total ammonium concentration remained at a similar level of around 800–950 g N/m3 throughout the semicontinuous operation except for the last run (R7) in which SBPR were digested as the monosubstrate. In experiment R7, the average TAN content was 215 g N/m3, but in the last 7 weeks of that experiment, it dropped to 63 g N/m3 (, ). Combined with the low phosphate content, the digestion process of SBPR remained stable and productive, despite no external supplementation with ammonium and phosphate salts. On the other hand, the digestion process of nutrient-rich materials such as sewage sludge may be inhibited by free ammonia, which has a much higher toxicity than the ammonium ion (NH4+), since it easily passes into cells and affects their metabolism. As stated in the literature, the inhibition of methanogenesis may start at an ammonia concentration of 150 g NH3/m3 (Calli et al., Citation2005); however, stable digestion operations were also reported at much higher values of up to 1100 g NH3/m3 (Hansen et al., Citation1998). In the following study, the FAN concentrations throughout the whole experimental period were far below the concentrations that could cause the digestion inhibition. Generally, the free ammonia concentrations reported in experiments R1–R4 varied between 9.7 and 13.9 g NH3/m3 with only insignificant differences between the runs ( and ). In experiments R5 and R6, these concentrations significantly increased to around 20 g NH3/m3, which was attributed to a simultaneous pH increase mainly due to the regular dosing of sodium hydroxide solution to the reactor, but were still below the values regarded as inhibitory for methanogenesis. In the last experimental run, the free ammonia concentration dropped to around 4 g NH3/m3 as a result of the low nitrogen content in sugar beet pulp residues, as mentioned earlier. Considering volatile fatty acids, their concentrations in runs with sewage sludge were relatively low and did not exceed 1000 g/m3, with the average values of 673 and 569 g/m3 in experiments R1 and R2, respectively (). The addition of SBPR to the sewage sludge resulted in a significant increase in the concentrations of TVFA, which is illustrated in and statistically confirmed (). The highest TVFA concentrations were detected in experiment R6, in which sewage sludge was treated with 50% SBPR. The average TVFA content in that run was 3934 g/m3 (as acetic), with the peak of 6000 g/m3 at the beginning of the run, which was even 6 times higher than that for sewage sludge (R1 and R2). These high levels corresponded to the greater OLR applied in the semicontinuous process and were attributed with a slight overload of the reactor. However, in the last experimental run during which diluted SBPR were treated as a monosubstrate, the average TVFA concentration was more than twice lower than the corresponding value reported in experiment R6. This can be explained by lower organic loading rate applied in run 7. The OLR applied in that run was similar to that of Run 5, which corresponded to the comparable TVFA levels reported in these experiments ( and ). Apart from regular determination of TVFA, individual fatty acids were also measured, three times in each experimental run. Acetic acid was found to be predominant VFA in experiments R1 and R2, constituting around 65% TVFA. It was followed by n-butyric and propionic acids at approximately 17% and 10%, respectively. However, with the increased SBPR content in the digesting mixture, the concentrations of acetic and propionic acids also increased. In experiment R7, the percentage concentrations of acetic, propionic, and n-butyric acids in TVFA were 76%, 18%, and 7%, respectively. In addition, a considerable amount of lactic acid with the peak of 2150 g/m3 was also detected. Lactic acid is a precursor of propionic acid, which in turn is regarded as the most inhibitory among all volatile fatty acids. It degrades slowly and its accumulation often leads to the digester failure. The reported concentrations of propionic acid that could inhibit biogas production are between 900 and 1500 g/m3 (Ma et al., Citation2009; Wang et al., Citation2009), which is far greater than the contents measured in this study.
Table 6. Statistical comparison of free ammonia release and volatile fatty acid productions in the semicontinuous experiments (P-value matrix).
The literature also states the TVFA levels that could inhibit methanogenesis at 2500–4000 g/m3; however, stable digestion operations are even possible at TVFA concentrations of up to 10,000 g/m3 (Appels et al., Citation2008; Kafle and Kim, Citation2013). Therefore, the anaerobic digestion only in experiment R6 might have been slightly inhibited, especially at the beginning of that run. On the other hand, due to a regular dosage of NaOH, the pH never dropped below 7 throughout the entire semicontinuous process. Moreover, the ratio of volatile fatty acids to total alkalinity was lower than 0.4, except the beginning of run R6 (, ), indicating safe and stable digestion operation (Callaghan el al., Citation2002; Kafle and Kim, Citation2013).
Conclusion
Sugar beet pulp residues derived from hydrolysis and dewatering of SBP have a high potential for biogas production and were successfully digested as the monosubstrate. However, the co-digestion of SBPR with sewage sludge significantly improved methane yield, due to the increased CH4 content in the digester gas. The maximum methane production of 350 dm3 CH4/kg VSfed was reported when the sewage sludge was co-digested with 35% SBPR. The digestion operation was stable throughout the entire experimental period, even at the highest applied OLR of 3.33 kg VS/m3·day, when the TVFA concentration temporarily reached nearly 6000 g/m3.
Funding
This research was performed as a part of the project no. PBS1/B8/0/2012 (“Biomass of sugar beet pulp as a new raw material for the production of fermentation media”) financed by the Applied Research Program of the National Centre for Research and Development (Poland).
Acknowledgment
The authors greatly appreciate Prof. Laurence R. Weatherley from the University of Kansas for proofreading and language correction.
Additional information
Funding
Notes on contributors
Sebastian Borowski
Sebastian Borowski is an assistant professor at the Institute of Fermentation Technology and Microbiology, Lodz University of Technology, in Łódź, Poland.
Marcin Kucner
Marcin Kucner is a Ph.D. student at the Institute of Fermentation Technology and Microbiology, Lodz University of Technology, in Łódź, Poland.
References
- Alkaya, E., and G.N. Demirer. 2011. Anaerobic mesophilic co-digestion of sugar-beet processing wastewater and beet-pulp in batch reactors. Renew. Energy 36:971–975. doi:10.1016/j.renene.2010.08.040
- American Public Health Association. 2005. Standard Methods for the Examination of Water and Wastewater, 21st ed. Washington, DC: American Public Health Association.
- Appels, L., J. Baeyens, J. Degreve, and R. Dewil. 2008. Principles and potential of the anaerobic digestion of waste-activated sludge. Prog. Energy Combust. Sci. 34:755–781. doi:10.1016/j.pecs.2008.06.002
- Arasimovich, V.V., and A.I. Ermakov. 1987. Measurement of polysaccharides and lignin. In Methods for Biochemical Studies of Plants, ed. A.I. Ermakov, 166–170. Leningrad: Agropromizdat.
- Athanasoulia, E., P. Melidis, and A. Aivasidis. 2012. Optimization of biogas production from waste activated sludge through serial digestion. Renew. Energy 47:147–151. doi:10.1016/j.renene.2012.04.038
- Balat, M. 2011. Production of bioethanol from lignocellulosic materials via the biochemical pathway: A review. Energy Convers. Manage. 52:858–875. doi:10.1016/j.enconman.2010.08.013
- Balat, M., H. Balat, and C. Oz. 2008. Progress in bioethanol processing. Prog. Energy Combust. Sci. 34:551–573. doi:10.1016/j.pecs.2007.11.001
- Bolzonella, D., P. Pavan, P. Battistoni, and F. Cecchi. 2005. Mesophilic anaerobic digestion of waste activated sludge: Influence of the solid retention time in the wastewater treatment process. Process Biochem. 40:1453–1460. doi:10.1016/j.procbio.2004.06.036
- Borowski, S., and L. Weatherley. 2013. Co-digestion of solid poultry manure with municipal sewage sludge. Bioresour. Technol. 142:345–352. doi:10.1016/j.biortech.2013.05.047
- Brooks, L., V. Parravicini, K. Svardal, H. Kroiss, and L. Prendl. 2008. Biogas from sugar beet press pulp as substitute for fossil fuel in sugar beet factories. Water Sci. Technol. 58:1497–1504. doi:10.2166/wst.2008.516
- Cabbai, V., M. Ballico, E. Aneggi, and D. Goi. 2013. BMP test of source selected OFMSW to evaluate anaerobic codigestion with sewage sludge. Waste Manage. 33:1626–1632. doi:10.1016/j.wasman.2013.03.020
- Callaghan, F.J., D.A.J. Wase, K. Thayanithy, and C.F. Forster. 2002. Continuous co-digestion of cattle slurry with fruit and vegetable wastes and chicken manure. Biomass Bioenergy 27:71–77. doi:10.1016/S0961-9534(01)00057-5
- Calli, B., B. Mertoglu, B. Inanc, and O. Yenigun. 2005. Effects of high free ammonia concentrations on the performances of anaerobic bioreactors. Process Biochem. 40:1285–1292. doi:10.1016/j.procbio.2004.05.008
- Concha, J., C. Weinstein, and M.E. Zuniga. 2013. Production of pectic extracts from sugar beet pulp with antiproliferative activity on a breast cancer cell line. Front. Chem. Sci. Eng. 7:482–489. doi:10.1007/s11705-013-1342-5
- Donkoh, E., J. Degenstein, and Y. Ji. 2012. Process integration and economics evaluation of sugar beet pulp conversion into ethanol. Int. J. Agric. Biol. Eng. 5:52–61 ( open access at http://www.ijabe.org). doi:10.3965/j.ijabe.20120502.007
- Doran, J., and B. Foster. 2000. Ethanol production from sugar beet pulp using engineered bacteria. Int. Sugar J. 102:336–340. doi:0020-8841(200007)102:1219<336:EPFSBP>2.0.ZU;2-8.
- Ehrman, T. 1996. Determination of Acid-Soluble Lignin in Biomass. Laboratory Analytical Procedure No. LAP-004. Golden, CO: National Renewable Energy Laboratory.
- Esposito, G., L. Frunzo, A. Panico, and F. Pirozzi. 2012. Enhanced bio-methane production from codigestion of different organic wastes. Environ. Technol. 33:2733–2740. doi:10.1080/09593330.2012.676077
- Fang, C., K. Boe, and I. Angelidaki. 2011. Anaerobic co-digestion of by-products from sugar production with cow manure. Water Res. 45:3473–3480. doi:10.1016/j.watres.2011.04.008
- Hansen, K.H., I. Angelidaki, and B.K. Ahring. 1998. Anaerobic digestion of swine manure: Inhibition by ammonia. Water Res. 32:5–12. doi:10.1016/S0043-1354(97)00201-7
- Hutnan, M., M. Drtil, and L. Mrafkova. 2000. Anaerobic biodegradation of sugar beet pulp. Biodegradation 11:203–211. doi:10.1023/A:1011139621329
- Ivetic, D.Z., B.M. Sciban, and M.G. Antov. 2012. Enzymatic hydrolysis of pretreated sugar beet shreds: Statistical modeling of the experimental results. Biomass Bioenergy 47:387–394. doi:10.1016/j.biombioe.2012.09.020
- Kafle, G.K., and S.H. Kim. 2013. Anaerobic treatment of apple waste with swine manure for biogas production: Batch and continuous operations. Appl. Energy 103:61–72. doi:10.1016/j.apenergy.2012.10.018
- Koppar., A., and P. Pullammanappallil. 2008. Single-stage, batch, leach-bed, thermophilic anaerobic digestion of spent sugar beet pulp. Bioresour. Technol. 99:2831–2839. doi:10.1016/j.biortech.2007.06.051
- Kryvoruchko, V., A. Machmuller, V. Bodiroza, B. Amon, and T. Amon. 2009. Anaerobic digestion of by-products of sugar beet and starch potato processing. Biomass Bioenergy 33:620–627. doi:10.1016/j.biombioe.2008.10.003
- Kürschner, K., and A. Hoffer. 1967. The isolation and determination of cellulose. In Methods of Wood Chemistry, Vol. 2, ed. B.L. Browning, 406–407. New York: John Wiley.
- Leijdekkers, A.G.M., J.P.M. Bink, S. Geutjes, H.A. Schols, and H. Gruppen. 2013. Enzymatic saccharification of sugar beet pulp for the production of galacturonic acid and arabinose; a study on the impact of the formation of recalcitrant oligosaccharides. Bioresour. Technol. 128:518–525. doi:10.1016/j.biortech.2012.10.126
- Limayem, A., and S.C. Ricke. 2012, Lignocellulosic biomass for bioethanol production. Current perspectives, potential issues and future prospects. Prog. Energy Combust. Sci. 38:449–467. doi:10.1016/j.pecs.2012.03.002
- Ma, J., M. Carballa, P. Van De Caveye, and W. Verstraete. 2009. Enhanced propionic acid degradation (EPAD) system: Proof of principle and feasibility. Water Res. 43:3239–3248. doi:10.1016/j.watres.2009.04.046
- Montanes, R., M. Perez, and R. Solera. 2013. Mesophilic anaerobic co-digestion of sewage sludge and a lixiviation of sugar beet pulp: Optimisation of the semi-continuous process. Bioresour. Technol. 142:655–662. doi:10.1016/j.biortech.2013.05.017
- Panagiotopoulos, J.A., R.R. Bakker, T. de Vrije, K. Urbaniec, E.G. Koukios, and P.A.M. Claassen. 2010. Prospects of utilization of sugar beet carbohydrates for biological hydrogen production in the EU. J. Clean. Prod. 18:S9–S14. doi:10.1016/j.jclepro.2010.02.025
- Spagnuolo, M., C. Crecchio, M.D.R. Pizzigallo, and P. Ruggiero. 1997. Synergistic effects of cellulolytic and pectinolytic enzymes in degrading sugar beet pulp. Bioresour. Technol. 60:215–222. doi:10.1016/S0960-8524(97)00013-8
- Stoyanova, E., B. Forsthuber, S. Pohn, C. Schwarz, W. Fuchs, and G. Bochmann. 2014. Reducing the risk of foaming and decreasing viscosity by two-stage anaerobic digestion of sugar beet pressed pulp. Biodegradation 25:277–289. doi:10.1007/s10532-013-9659-9
- Suhartini, S., S. Heaven, and C.J. Banks. 2014. Comparison of mesophilic and thermophilic anaerobic digestion of sugar beet pulp: Performance, dewaterability and foam control. Bioresour. Technol. 152:202–211. doi:10.1016/j.biortech.2013.11.010
- Templeton, D., and T. Ehrman. 1995. Determination of Acid-Insoluble Lignin in Biomass. Laboratory Analytical Procedure No. LAP-003. Golden, CO: National Renewable Energy Laboratory.
- Wang, Y., Y. Zhang, J. Wang, and L. Meng. 2009. Effect of volatile fatty acid concentrations on methane yield and methanogenic bacteria. Biomass Bioenergy 33:848–853. doi:10.1016/j.biombioe.2009.01.007
- Weiland, P. 2010. Biogas production: Current state and perspectives. Appl. Microbiol. Biotechnol. 85:849–860. doi:10.1007/s00253-009-2246-7
- Zheng, Y., C. Yu, Y. Cheng, C. Lee, C.W. Simmons, T.M. Dooley, R. Zhang, B.M. Jenkins, and J.S. VanderGheynst. 2012. Integrating sugar beet pulp storage, hydrolysis and fermentation for fuel ethanol production. Appl. Energy 93:168–175. doi:10.1016/j.apenergy.2011.12.084