ABSTRACT
The open lots and manure stockpiles of dairy farm are major sources of greenhouse gas (GHG) emissions in typical dairy cow housing and manure management system in China. GHG (CO2, CH4 and N2O) emissions from the ground level of brick-paved open lots and uncovered manure stockpiles were estimated according to the field measurements of a typical dairy farm in Beijing by closed chambers in four consecutive seasons. Location variation and manure removal strategy impacts were assessed on GHG emissions from the open lots. Estimated CO2, CH4 and N2O emissions from the ground level of the open lots were 137.5±64.7 kg hd-1 yr-1, 0.45±0.21 kg hd-1 yr-1 and 0.13±0.08 kg hd-1 yr-1, respectively. There were remarkable location variations of GHG emissions from different zones (cubicle zone vs. aisle zone) of the open lot. However, the emissions from the whole open lot were less affected by the locations. After manure removal, lower CH4 but higher N2O emitted from the open lot. Estimated CO2, CH4 and N2O emissions from stockpile with a stacking height of 55±12 cm were 858.9±375.8 kg hd-1 yr-1, 8.5±5.4 kg hd-1 yr-1 and 2.3±1.1 kg hd-1 yr-1, respectively. In situ storage duration, which estimated by manure volatile solid contents (VS), would affect GHG emissions from stockpiles. Much higher N2O was emitted from stockpiles in summer due to longer manure storage.
Implications: This study deals with greenhouse gas (GHG) emissions from open lots and stockpiles. It’s an increasing area of concern in some livestock producing countries. The Intergovernmental Panel on Climate Change (IPCC) methodology is commonly used for estimation of national GHG emission inventories. There is a shortage of on-farm information to evaluate the accuracy of these equations and default emission factors. This work provides valuable information for improving accounting practices within China or for similar manure management practice in other countries.
Introduction
Manure in livestock production is an important greenhouse gas (GHG) emission source, which accounts for 18 of total emissions related to agriculture (U.S. Environmental Protection Agency [EPA], 2012). The most popular dairy housing and manure management system in China houses cows in naturally ventilated barns with an outdoor open lot (about 20 m2 hd−1 or more), where animal is freely accessible. Cows normally stay on the brick- or soil-paved open lot for most of the day, even in winter, when barns are only used for feeding. Hence, nearly all the manure is deposited onto the open lots in this housing operation. After deposition, manure may remain on the open lots from days to months, depending on the different manure collection schemes, and cause a lot of gaseous emissions. As reported, the open lots may contribute to 51% of carbon dioxide (CO2), 72% of nitrous oxide (N2O), and 63–95% of ammonia (NH3) emissions at ground level in dairy farms (Mukhtar et al., Citation2008; Borhan et al., Citation2011b). In China, solid manure on the open lots is usually collected and stockpiled somewhere in the farm without any cover, for days to months, before being applied to arable land, depending on the seasons and cropping time. Comparing with the emission after manure field application, more than 99% of methane (CH4) emissions may come from manure storage (Amon et al., 2006b). So the ground-level GHG emissions from this management system mainly come from manure on the open lots and uncovered stockpiles.
Figure 1. Layout of dairy farm and experimental section in field measurements (surveyed open lots and manure stockpiles are marked with cross lines).
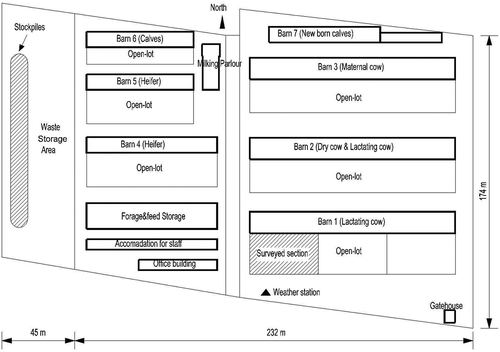
The Intergovernmental Panel on Climate Change (IPCC) default value is commonly used in assessing China’s GHG emissions from manure on dairy farm. However, it does not fully describe the emissions from the system mentioned above. Therefore, field measurements are needed to accurately determine the global warming impact of the dairy sector in China. This is also important for an accurate global GHG emission inventory due to the large population of dairy cows in China. Due to the shortage of effective measurement equipments, little fundamental information on GHG emissions is available for the dairy sector in China, especially from the open lot system and manure stockpiles.
The objective of this study was to characterize and estimate GHG emissions from the ground level of an open lot and from uncovered manure stockpiles from a dairy farm, based on the case study of a typical dairy farm in northern China.
Materials and methods
Field measurements in different seasons were performed to estimate the GHG emissions from the open lot and manure stockpiles. The closed-chamber method was used in the field, even though some disadvantages on the method still exist (Lovanh et al., Citation2010; Nkongolo et al., Citation2010). To improve the measuring accuracy of the closed chamber, chamber configuration, headspace mixing, and vent effects on chamber performance were evaluated at the laboratory-scale to optimize design and operation prior to field measurements (Ding et al., 2013, Citation2015).
Farm description
The case study was carried out at a commercial dairy farm (115°56′15″~115°56′29″E longitude and 40°29′54″~40°30′N latitude), located in Yanqing County of Beijing, China. Average liquid milk production was approximately 8 tons per year per cow. Rations for lactating cows contained 66% silage, 10% Chinese wildrye, 12% corn, 7.2% soya meal, 1.2% rapeseed meal, 2.4% wheat bran, and 1.2% minerals and additives. Field measurements were conducted in the left section of lactating cow pen and waste storage area in the west section of the farm ().
The surveyed open lot is 40 m long, 27 m wide, with 45 lactation cows on it and brick-paved (). A sunshade equipped with sand bedded cubicles underneath locates almost exactly in the center of the open lot (). Dairy cows were kept on the lots except during the feeding time. Cows were fed three times a day inside the barn and milked about 1 hr prior to each feeding. Manure deposited inside the houses was manually scraped to the open lots after each feeding. During the field measurements, manure on the open lot was collected daily by a tractor at 1:00 p.m. Afterwards, the manure was removed to waste storage area and stockpiled. Waste storage area was located in the west of the farm ().
Gas sampling in field measurements
Chambers for gas sampling
The closed cylindrical chambers, made of acrylic, were used for field measurement in this study. Chamber diameter was 0.5 m, and its height was 0.3 m. Air temperature (T) and relative humidity (RH) in chamber headspace were collected every 5 min by a T&RH sensor (model: Apresys 179A-TH; GA, USA; range: −40–85°C, 0–100%; accuracy: ±0.2 °C, ±1.8% RH). Sampling tube was set in the middle of chamber at a height of 0.15 m above the bottom. Gas (200 mL) was manually sampled by using a 100-mL glass syringe and stored in FlexFoil (Dalian Hede Technologies LTD., China) gas sampling bags. Gas samples were analyzed immediately after finishing all the gas sampling events by gas chromatography (Agilent Technologies; detectors: FID [flame ionization detector] and ECD [electron capture detector]; heater: 375 °C; carrier gas: hydrogen [45.0 mL min−1], air [40.0 mL min−1], nitrogen [12.4 mL min−1]). Each gas sample had two replicates for gas chromatography analysis.
Chambers were insulated by aluminum foil–coated heat insulation cotton to prevent air temperature increases in the headspace caused by solar radiation. To avoid air leakage in the headspace of the chambers, water-sealed bases were applied for stockpile measurement and they were inserted into the manure at a depth of 0.2 m 24 hr prior to the test. For the open lot, chambers were placed on the floor surface and sealed by sand with an air-filled porosity of about 21%. Control test were performed before field measurement to make sure there were no target GHG emissions from the sealed sand. New sand was used for each sampling.
Gas sampling on the open lot
Due to the variation of the manure accumulated on the floor surface, which theoretically resulted in emission difference, the open lot was divided into a cubicle zone and an aisle zone for a better clarification of the emission sources. The 1.5 m width area surrounding the cubicles (shown in ) was defined as cubicle zone, where some 5 times more manure was accumulated and the manure moisture content, around 86%, was also much higher than in the aisle zone. The remaining area was defined as the aisle zone, and the moisture content of the accumulated manure on it was approximately 70%. Gases were sampled at only one location in each zone, due to the disturbance of dairy cow, the high labor intensity of manually gas sampling, and relatively small area (40 × 27 m) of surveyed open lot. Gas sampling was performed in five consecutive days and two or three times per day in each season (). To improve the representativeness of sampling, the location was chosen randomly and without duplication during the five days ().
Table 1. Sampling schedules and the number of sampling events on GHG emissions from the open lot and manure stockpile in a typical dairy farm in four different seasons.
As shown in , sampling schedules varied with season according to the results of last season and air temperature. Measurements between 10:00 a.m. and 12:00 p.m. were made to reflect the daily average (de Klein and Harvey, Citation2013). Thus, measurements were performed only during daytime.
Manure depth and the amount of manure enclosed at each measurement before manure removal were recorded as reference information. Manure depth was measured at nine points distributed within the enclosed area. Enclosed manure was manually scraped into trays and weighted. No manure was enclosed, and measured GHG emissions came from floor surface immediately after manure removal.
Gas sampling on the stockpiles
Manure stockpiles were usually heaped up as a 7-m-wide strip at a height of around 60 cm. Three (or two) sampling locations were evenly distributed along the strip according to its size within different seasons (). Sampling schedules for the stockpiles are also shown in . Reference factors of manure stockpiles such as stockpile surface area, stacking height, manure bulk density, dry matter (DM), and volatile solid (VS) are showed in . For fresh manure, its bulk density, pH, DM, VS, TOC (total organic carbon), and TN (total nitrogen) were 1.1 g cm−3, 7.38, 15.9%, 86.1% (on DM basis), 39.1%, and 1.63% (on DM basis), respectively.
Table 2. Surface area and stacking height of stockpiles, manure bulk density, dry matter (DM), volatile solid (VS), as well as number of sampling points during the field measurements in four seasons.
Gas emission estimation
GHG emission rates at sampling locations were calculated by
Greenhouse gas emissions from the whole surveyed open lot (Eol, kg hd−1 yr−1) were estimated based on its area, stocking density, and the fluxes before and after manure removal from different measurement locations in each season by
And gas emissions from stockpiles (Es, kg hd−1 yr−1) were estimated based on manure discharge rate, stockpile characteristics, and average flux from sampling locations that might have different manure ages or core temperatures in each season by
Global warming potential
Total carbon dioxide–equivalent emissions for methane and nitrous oxide were calculated using global warming potentials (GWPs) over a given time compared with the same mass of carbon dioxide. IPCC updated the GWP values in 2013. However, the results in recent studies were reported using the GWPs from IPCC, 2007. To make the results comparable, GWPs used in this study were 25 for CH4 and 298 for N2O (Forster et al., Citation2007).
Micrometeorological measurements
Background environment, including temperature, relative humidity, wind speed and direction of ambient air, and solar radiation as well, were measured by a weather station (; HOBO H21-001, Onset Computer Corporation, USA). Air temperature, RH, and floor surface temperature in the surveyed open lot as well as manure temperature of the stockpiles were collected to be references for greenhouse gas emission estimations.
Statistical analyses
SPSS (version 20.0; IBM, Armonk, NY, USA) was used for statistical analyses. According to the normality test, CO2 emissions from the open lots in different seasons were not normally distributed. Log10 transformation was used to satisfy the normal distribution of CO2 emissions. Analysis of variance (ANOVA) had been carried out to analyze the impacts of location variation and manure removal on CO2, CH4, and N2O emission rates from open lots. Statistical significance was based on P < 0.05. Results were expressed as mean ± SD.
Results and discussion
Environmental conditions
Background air temperature (T), relative humidity (RH), wind speed, and solar radiation during field measurements for estimation are shown in .
Table 3. Background air temperature (T), relative humidity (RH), wind speed, and solar radiation during field measurements in four different seasons (N = 5).
shows the daily air T and RH variation during measurements in different seasons. This is the typical weather for the region except the last two sampling days in winter. The last two days in winter measurement had a warmer weather than usual.
GHG emissions from the open lots
Location variation on GHG emissions
The emission rates of GHG, including CO2, CH4, and N2O, from different measurement locations (cubicle zone and aisle zone) within surveyed open lots are shown in . Measurement locations and manure removal practice had a significant impact on GHG emission rates from the open lot (P < 0.05). Compared with the aisle zone, CO2 and CH4 emissions from cubicle zone were higher (P < 0.05), whereas N2O emissions showed a disparity in different seasons.
Table 4. CO2, CH4, and N2O emissions from brick-paved dairy open lots in different seasons before and after manure removal at different measurement locations by closed chamber (N = 5).
CH4 emissions are highly correlated to organic matter contents (Sommer et al., Citation2004b; IPCC, Citation2006). The aisle zone had a relatively stable manure depth in all seasons (0.4 ± 0.1 cm) whereas manure depth in the cubicle zone varied and averaged at 1.8 ± 0.9 cm in different seasons. Thus, higher CH4 emissions were measured for the cubicle zone.
Except in winter, averaged N2O emissions in other seasons from the cubicle zone were higher than the aisle zone. Due to solar radiation, floor temperature in the aisle zone was higher than the cubicle zone. Manure in the cubicle zone was frozen in winter during field measurements (−5.0 ± 6.5 °C) due to its higher moisture content and height, but this did not happen in the aisle zone (−1.6 ± 6.2 °C). This may explain the higher N2O emissions in aisle zone in winter.
Impacts of manure removal strategy on GHG emissions
GHG emissions before and after manure removal from open lot are shown in . Comparing CO2 and CH4 emissions before and after manure removal, CH4 emissions were lower after manure removal (P < 0.05), whereas CO2 emissions were in the same level (). As documented, CH4 emission is largely dependent on the amount of organic matter and the height of the stocked manure (Dong et al., Citation2011). Due to there being more organic matter enclosed before manure removal, similarly, higher emissions were found before manure removal comparing with that after manure removal at both measurement zones. For CO2, it’s not clear why emissions before and after manure removal were close to each other.
Figure 4. (a) GHG emissions at cubicle and aisle zones before manure removal. (b) GHG emissions at cubicle and aisle zones after manure removal. (c) GHG emissions before or after manure removal at cubicle zone. (d) GHG emissions before or after manure removal at aisle zone.
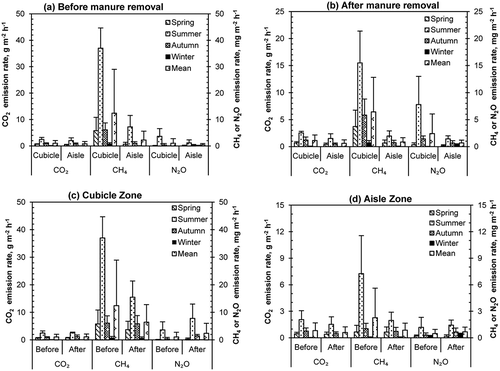
After manure removal, N2O emissions increased both at the aisle and cubicle zones (P < 0.05). Previous studies illustrate that N2O emissions mainly come from soil with manure and urine deposition (Monteny et al., Citation2006; Chadwick et al., Citation2011). Higher N2O is produced with better oxygen status, warmer temperature combined with moist environment in the process of manure and urine deposition (Maag and Vinther, Citation1996; Owen and Silver, Citation2014). For the surveyed brick-paved lot, the ground surface was considered to be the major source of N2O emission due to the deposition process of manure/urine on it, although there was some manure and urine penetrating into the soil. After the accumulated manure was removed, a new excreta-affected surface and/or the floor was exposed to ambient air. The change of the microenvironment at the manure surface, oxygen status in particular, as well as the increased surface temperature that follows manure removal might have led to higher emission rates during the measurements. This is suggested to be the main reason for N2O emissions from the open lot being higher after manure removal.
Impacts of temperature and manure height on GHG emission
GHG emissions from the surveyed open lot showed seasonal variations for all the gas species (, ). For all the gases, the highest emission rates generally occurred in summer and the lowest was in winter. This was in agreement with the results from the laboratory tests by Pereira et al. (Citation2011, Citation2012). They found that CO2, CH4, N2O, and NH3 emissions increased significantly with air temperature (from 5 to 35°C) at laboratory-scale studies.
Table 5. Estimated CO2, CH4, and N2O emissions and global warming potential (GWP) from dairy open lot (N = 5).
shows the relationship between open lot or cubicle zone CH4 emission and manure height or air temperature from spring to autumn (manure height not available in winter). Generally, cubicle zone CH4 emission rates in each season were positively related to manure height (), whereas the emission per unit height (1 cm) was highly correlated to air temperature (). Manure height averaged from the whole open lot was 0.5 ± 0.1 cm and close to the average manure height in the aisle zone (0.4 ± 0.1 cm). Similarly, CH4 emissions averaged from the whole open lot were relatively stable in each season (), but per unit height emission was still highly correlated to air temperature (). This might suggest that air temperature was a key factor affecting CH4 emissions from the brick-paved open lot. There would be location variation (cubicle or aisle zone) for CH4 emission and manure height, but the averaged emission or depth of the whole open lot was less affected and relatively stable.
GHG emissions from the ground level of open lot
Estimated CO2, CH4, and N2O emission rates from ground level of the investigated open lot were 137.5 ± 64.7 kg hd−1 yr−1, 452.7 ± 210.6 g hd−1 yr−1, and 129.7 ± 82.4 g hd−1 yr−1, respectively, which were equivalent to 5.7 ± 2.7 kg m−2 yr−1, 18.8 ± 8.7 g m−2 yr−1 and 5.4 ± 3.4 g m−2 yr−1 based on the lot area. Compared with other estimation based on field measurements by flux chamber in different dairy farms, the emissions estimated in our study were higher than the results from Misselbrook et al. (Citation2001) and lower than those from Borhan et al. (Citation2011a, Citation2011b), which were 3.5 g m−2 yr−1 or 4.6 kg hd−1 yr−1 for CH4 and 0.13 g m−2 yr−1 or 1.4 kg hd−1 yr−1 for N2O, respectively. CO2 emission was not available in the investigation of Misselbrook et al. (Citation2001), and it was 1588.6 kg hd−1 yr−1 according to Borhan et al. (Citation2011a, Citation2011b).
According to Misselbrook’s investigation (Citation2001), CH4 and N2O emissions from dairy collecting or feeding yards were within 0.2~0.7 mg m−2 hr−1 and 2.9~39.3 μg m−2 hr−1, respectively, when the ambient temperature was 5~25 °C. In this study, the air temperature was close to their investigation in the spring and autumn measurements (), whereas the emission rates of both gases were higher. It might be because of different measurement systems of the two farms investigated, which resulted in the different amount of manure on the floor surface. For the field measurement by Misselbrook et al. (Citation2001), CH4 and N2O emission rates were detected from an outdoor concrete yard, and nearly no manure was able to penetrate into the soil, which may lead to potential GHG emissions. Furthermore, dairy cows stayed much less time on the collecting and feeding yard compared with the open lot system, and the manure accumulated on the yard was also less. CH4 emission rates from the outdoor yard were similar to those from the aisle zone of the open lot in spring and autumn measurements. Unlike CH4 emissions, floor type could affect CO2, NH3, and N2O emissions (Pereira et al., Citation2011). Little ground type information could be found in Misselbrook et al.’s investigation. It’s hard to explain the reason for higher N2O emission in this research.
Borhan et al. (Citation2011a, Citation2011b) also investigated GHG emissions from an open dairy lot using flux chambers, and reported that CO2, CH4, and N2O emissions were in the range of 2824~5392, 5.1~21.5, 2.2~7.5 g hd−1 day−1, respectively, in winter and summer. It was unpaved ground in Borhan et al.’s investigations, and manure management was different as well. Manure was removed twice a week from the surface, and the open lots were cleaned only twice a year. Different ground type and manure removal strategy were the main reasons for lower GHG emission rates in this investigation.
Compared with results measured by using open-path Fourier transform infrared (FTIR) (Bjorneberg et al., Citation2009; Leytem et al., Citation2011, Citation2013), CO2 and CH4 emissions estimated by flux chambers were relatively lower. It was because chambers are only able to capture the emissions from ground level, whereas the FTIR will also capture the CO2 emissions due to animal respiration and CH4 from enteric fermentation, even though CO2 from livestock are not included in GHG inventory. For N2O, Bjoreberg et al. (Citation2009) failed to determine the concentration difference between the measured space and the background by FTIR; consequently, no emission data were available in their studies. Same thing happened in some cases in the investigations of Leytem et al. (Citation2013). Hence, flux chambers maybe a better choice to measure N2O emissions from open lot due to its low emission and that it only comes from ground level.
GHG emissions from stockpile
Seasonal variation of GHG emission
GHG emissions from manure stockpile of dairy farm in four consecutive seasons are shown in , which indicated a seasonal variation for all gas emissions. CO2, CH4, and N2O emissions from stockpiles ranged from 1.4 to 639.9, 0.003 to 62.1, and 0.4 to 1385.4 mg m−2 day−1, respectively, in different seasons (). Equivalent GHG emissions from stockpiles were 9.6 ± 3.8, 295.0 ± 153.0, 3.2 ± 1.1, and 3.6 ± 3.6 g CO2-eq m−2 day−1 from spring to winter. CH4 and N2O emissions in summer were much higher than the fluxes in other three seasons ().
Table 6. Average CO2, CH4, N2O emissions and global warming potential (GWP) as well as core manure temperature (Tcore) and VS from stockpiles in four consecutive seasons (N = 9).
Figure 6. (a) CO2-, CH4-, and N2O-equivalent emissions from manure stockpile vs. manure or ambient temperature. (b) Percentage of CO2, CH4, and N2O from stockpile in total GHG-equivalent emissions vs. manure or ambient temperature.
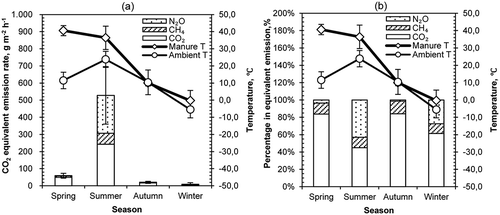
Similar to emissions from open lot, GHG emissions in summer were much higher than the fluxes in the other three seasons (, ). CO2, CH4, and N2O emissions in autumn were lower than spring, and in those two measurements air temperatures were close to each other (). Thick and dry crust was observed on the manure surface during field measurement in autumn. Except for lower VS concentration and manure temperature (, ), the crust could be responsible for much lower GHG emissions in autumn. Some studies indicated that crust would suppress CH4 emission and suggested measures to ensure crust formation, which could be a GHG mitigation option (Husted, Citation1994; Petersen et al., 2005).
Emission percentages of CO2, CH4, and N2O in total GHG-equivalent emission from the surveyed manure stockpile were plotted in . CO2 has the largest contribution in the total GHG-equivalent emissions (more than half). Unlike the seasonal variations in emission rates, CO2, CH4, and N2O emission proportions in different seasons didn’t show a correlation with the ambient temperature or manure temperature. CH4 emissions varied a lot in different seasons (, ). However, its proportions in total GHG-equivalent emissions were stable (about 12%) in different seasons. N2O had very small contributions to GHG emissions in spring and summer (<4%), whereas it has higher proportions in summer and winter. Compared with the other three seasons, N2O emission in summer was high () due to the different principle of N2O generation and release from the manure (explained in Storage Duration and N2O Emissions), and its proportion to total GHG was also significantly larger. Nearly half GHG-equivalent emissions resulted from N2O in summer.
Location variation of GHG emission
Normally, manure removed from the open lot or the barn was stored in one strip and the length was extended accordingly. Time intervals of manure applications in four different seasons differ because of land cultivation strategy. Thus, manure in each sampling point or measurement in different seasons might have different ages and age of manure will affect results. Organic matter degradation occurs during manure storage (or composting), and its volatile solid (VS) content decreases along composting (Li et al., Citation2008). VS concentration in manure was used as a reference for manure age or storage duration in this field measurement. Manure with lower VS concentration was older and stored longer than that with higher VS content.
Average GHG emission rates came from different sampling locations in each season. Due to different manure age and fermentation stage of each sampling point, coefficients of variation (CVs) for GHG emissions in each season were calculated to be a reference for location variation of GHG emissions.
Except for CH4 emissions in summer, CVs of CO2, CH4, and N2O emissions from spring to autumn were <50%. This suggests that GHG emissions measured from different sampling locations were relatively comparable in these seasons (except CH4 in summer). Coefficient of variation for CH4 emission in summer was >50%. Large variation in VS concentration (52.6%; ) in manure from different sampling locations may be responsible for it. Coefficient of variation for GHG emissions in winter was quite large due to the large variation of manure core temperature (). Manure temperature of the sampling point in the middle of crib was above 5 °C, whereas the other two points were below zero.
Storage duration and N2O emissions
As indicated, N2O emissions in summer were 100 times higher than the other three seasons (). As a result of nitrogen concentration changes (NH4+-N and NO3−-N) during manure storage, storage duration or manure age may have been responsible for the much higher N2O emissions in summer. Average manure VS concentration was lower (18.6~52.8%; ) in summer, which indicated that some manure was quite old (or well fermented). Differing from composting, NH4+-N in stockpile decreases and NO3−-N increases in the process of manure storage (Patty et al., Citation2005). For manure storage without pile mixing, the initial N2O emissions mainly come from nitrification of NH4+-N, whereas denitrification of NO3−-N in anaerobic condition would be dominant in N2O production in the latter phase (Patty et al., Citation2005; Monteny et al., Citation2006; Chadwick et al., Citation2011). Moreover, NH4+-N is a primary N source of methanogenic bacteria (Patty et al., Citation2005). There may be a competition on NH4+-N between methanogenic bacteria and nitrobacteria in the first few days of storage. Due to the changing of NH4+-N and NO3−-N concentration and the possibility of N source competition during manure storage, there may have been more N source available for N2O production in latter phase of manure storage. Thus, older manure might produce more N2O. The reason why coefficient of variation for N2O emission in summer was very large could also be explained by different manure ages at different sampling locations. Ahn et al. (Citation2011) studied the effect of pile mixing on GHG emissions during dairy manure composting, and they found that 80~95% of N2O emissions occurred after 23 days from manure piles. This could be a proof for that older manure would have higher N2O emission.
shows the relationship between N2O emission rates and manure VS concentration of different sampling locations from stockpiles. In the same season, N2O emissions were higher when manure VS concentration was lower. But this only happened when VS difference between two sampling points were higher than 10%, and surface crust (in autumn) would weaken the difference of emission rate ().
Estimated GHG emissions
Estimated CO2, CH4, and N2O emissions and GWP from stockpile in this investigation were 858.9 ± 375.8, 8.5 ± 5.4 kg, and 2.3 ± 1.1 kg hd−1 yr−1 and 906.8 ± 470.5 kg CO2-eq hd−1 yr−1, respectively. Owen and Silver (Citation2014) summarized the means (754 ± 695, 3 ± 11, and 1.1 ± 0.7 kg hd−1 yr−1, respectively) and ranges (59~3546, 0~99, and 0.02~27 kg hd−1 yr−1, respectively) of CO2, CH4, and N2O emission rates from solid manure field measurements. CH4 and N2O emission rates measured in this study were comparable to the mean values given by Owen and Silver (Citation2014), and also within the ranges.
GHG emissions from manure management
In this case, total GHG emission (Et) from manure management was estimated by the sum of the emissions from open lot and stockpile: 9.0 kg hd−1 yr−1 CH4 and 2.75 kg hd−1 yr−1 N2O emitted from this typical Chinese dairy manure management system based on this field measurement. According to the IPCC default value for manure solid storage and tier 1 methodology (IPCC, Citation2006), estimated CH4 and N2O emissions were 10 and 2.05 kg hd−1 yr−1. CH4 emission was a slightly overestimated and N2O emission was underestimated by the IPCC estimation.
Summary
A case study was performed to estimate and characterize greenhouse gas (CO2, CH4, and N2O) emissions from a dairy open lot and manure stockpiles in northern China, with field measurements in spring, summer, autumn, and winter by chamber technique. In this case, the estimated global warming potential from a typical dairy manure management system was 956.8 kg CO2-eq hd−1 yr−1 in northern China. More than 90% of emissions came from stockpiles when manure on the open lot was removed once a day. However, the storage duration and manure removal frequency on the open lot would change the proportion.
Funding
This study was funded by the National Nature Science Foundation of China (grant no. 31172244, 31472132), China Agricultural Research System (CARS-37), Chinese Universities Scientific Fund (2013YJ009), and Ph.D. research grants from Climate Food and Farming (CLIFF) Research Network in Denmark.
Additional information
Funding
Notes on contributors
Luyu Ding
Luyu Ding is a Ph.D. student, Qikun Lu, Lina Xie, and Jie Liu are graduate students, Wei Cao, Zhengxiang Shi, and Baoming Li are professors, and Chaoyuan Wang is an associate professor in the Department of Agricultural Structure and Bioenvironmental Engineering, College of Water Resources and Civil Engineering, China Agricultural University, People’s Republic of China.
Qikun Lu
Luyu Ding is a Ph.D. student, Qikun Lu, Lina Xie, and Jie Liu are graduate students, Wei Cao, Zhengxiang Shi, and Baoming Li are professors, and Chaoyuan Wang is an associate professor in the Department of Agricultural Structure and Bioenvironmental Engineering, College of Water Resources and Civil Engineering, China Agricultural University, People’s Republic of China.
Lina Xie
Luyu Ding is a Ph.D. student, Qikun Lu, Lina Xie, and Jie Liu are graduate students, Wei Cao, Zhengxiang Shi, and Baoming Li are professors, and Chaoyuan Wang is an associate professor in the Department of Agricultural Structure and Bioenvironmental Engineering, College of Water Resources and Civil Engineering, China Agricultural University, People’s Republic of China.
Jie Liu
Luyu Ding is a Ph.D. student, Qikun Lu, Lina Xie, and Jie Liu are graduate students, Wei Cao, Zhengxiang Shi, and Baoming Li are professors, and Chaoyuan Wang is an associate professor in the Department of Agricultural Structure and Bioenvironmental Engineering, College of Water Resources and Civil Engineering, China Agricultural University, People’s Republic of China.
Wei Cao
Luyu Ding is a Ph.D. student, Qikun Lu, Lina Xie, and Jie Liu are graduate students, Wei Cao, Zhengxiang Shi, and Baoming Li are professors, and Chaoyuan Wang is an associate professor in the Department of Agricultural Structure and Bioenvironmental Engineering, College of Water Resources and Civil Engineering, China Agricultural University, People’s Republic of China.
Zhengxiang Shi
Luyu Ding is a Ph.D. student, Qikun Lu, Lina Xie, and Jie Liu are graduate students, Wei Cao, Zhengxiang Shi, and Baoming Li are professors, and Chaoyuan Wang is an associate professor in the Department of Agricultural Structure and Bioenvironmental Engineering, College of Water Resources and Civil Engineering, China Agricultural University, People’s Republic of China.
Baoming Li
Luyu Ding is a Ph.D. student, Qikun Lu, Lina Xie, and Jie Liu are graduate students, Wei Cao, Zhengxiang Shi, and Baoming Li are professors, and Chaoyuan Wang is an associate professor in the Department of Agricultural Structure and Bioenvironmental Engineering, College of Water Resources and Civil Engineering, China Agricultural University, People’s Republic of China.
Guoqiang Zhang
Guoqiang Zhang is a senior scientist in the Department of Engineering, Aarhus University, Aarhus, Denmark.
Shixi Ren
Shixi Ren is a technician in the Agricultural Bureau of Yanqing County, Beijing, People’s Republic of China.
References
- Ahn, H. K., W. Mulbry, J.W. White, and S.L. Kondrad. 2011. Pile mixing increases greenhouse gas emissions during composting of dairy manure. Bioresour. Technol. 102:2904–2909. doi:10.1016/j.biortech.2010.10.142
- Amon, B., V. Kryvoruchko, T. Amon, and S. Zechmeister-Boltenstern. 2006. Methane, nitrous oxide and ammonia emissions during storage and after application of dairy cattle slurry and influence of slurry treatment. Agric. Ecosyst. Environ. 112:153–162. doi:10.1016/j.agee.2005.08.030
- Bjorneberg, D. L., A.B. Leytem, D.T. Westermann, P.R. Griffith, L. Shao, and M.G. Pollard. 2009. Measurement of atmospheric ammonia, methane, and nitrous oxide at a concentrated dairy production facility in Southern Idaho using open-path FTIR spectrometry. Trans. ASABE 52:1749–1756. doi:10.13031/2013.29137
- Borhan, M.S., D.P. Gautam, C. Engel, V.L. Anderson, and S. Rahman. 2013. Effects of pen bedding and feeding high crude protein diets on manure composition and greenhouse gas emissions from a feedlot pen surface. J. Air Waste Manage. Assoc. 63:1457–1468. doi:10.1080/10962247.2013.831384
- Borhan, M.S., C.S. Capareda, S. Mukhtar, W.B. Faulkner, R. McGee, and C.B. Parnell. 2011a. Determining seasonal greenhouse gas emissions from ground-level area sources in a dairy operation in Central Texas. J. Air Waste Manage. Assoc. 61:786–795. doi:10.3155/1047-3289.61.7.786
- Borhan M.S., C.S. Capareda, S. Mukhtar, W.B. Faulkner, R. McGee, and C.B. Parnell. 2011b. Greenhouse gas emissions from ground level area sources in dairy and cattle feedyard operations. Atmosphere 2:303–329. doi:10.3390/atmos2030303
- Caro, D., S.J. Davis, S. Bastianoni, and K. Caldeira. 2014. Global and regional trends in greenhouse gas emissions from livestock. Clim. Change 126:203–216. doi:10.1007/s10584-014-1197-x
- Chadwick, D., S. Sommer, R. Thorman, D. Fangueiro, L. Cardenas, B. Amon, and T. Misselbrook. 2011. Manure management: Implications for greenhouse gas emissions. Anim. Feed Sci. Technol. 166:514–531. doi:10.1016/j.anifeedsci.2011.04.036
- de Klein, C., and M. Harvey (eds.). 2013. Nitrous Oxide Chamber Methodology Guidelines. Wellington, New Zealand: Ministry for Primary Industries.
- Ding, L., Q. Lu, C. Wang, Z. Shi, W. Cao, and B. Li. 2015. Effect of configuration and headspace mixing on the accuracy of closed chambers for dairy farm gas emission measurement. Appl. Eng. Agric. 31:153–162.
- Ding, L., C. Wang, Q. Lu, Z. Yan, and Z. Shi. 2013. Effects of configuration and headspace wind profile on the performances of flux chambers in gas emission measurement: A laboratory study. Presented at ASABE Annual Meeting, Kansas City, Missouri, July 21–24, 2013. Paper number 131619989.
- Dong, H., Z. Zhu, Z. Zhou, H. Xin, and Y. Chen. 2011. Greenhouse gas emissions from swine manure stored at different stack heights. Anim. Feed Sci. Technol. 166:557–561. doi:10.1016/j.anifeedsci.2011.04.039
- Forster, P., V. Ramaswamy, P. Artaxo, T. Berntsen, R. Betts, D.W. Fahey, J. Haywood, J. Lean, D.C. Lowe, G. Myhre, J. Nganga, R. Prinn, G. Raga, M. Schulz, and R. Van Dorland, 2007: Changes in atmospheric constituents and in radiative forcing. In Climate Change 2007: The Physical Science Basis. Contribution of Working Group I to the Fourth Assessment Report of the Intergovernmental Panel on Climate Change, ed. S. Solomon, D. Qin, M. Manning, Z. Chen, M. Marquis, K.B. Averyt, M. Tignor, and H.L. Miller, 129–234. Cambridge (UK) and New York: Cambridge University Press.
- Gonzalez-Avalos, E., and L.G. Ruiz-Suarez. 2001. Methane emission factors from cattle manure in Mexico. Bioresour. Technol. 80:63–71. doi:10.1016/S0960-8524(01)00052-9
- Husted, S. 1994. Seasonal variation in methane emission from stored slurry and solid manures. J. Environ. Qual. 23:585–592. doi:10.2134/jeq1994.00472425002300030026x
- IBM Corp. 2011. IBM SPSS Statistics for Windows, Version 20.0. Armonk, NY: IBM Corp.
- Intergovernmental Panel on Climate Change. 2006. Guidelines for National Greenhouse Gas Inventories. General Guidance and Reporting of Intergovernmental Panel on Climate: Chapter 10, 1–86. Geneva: United Nations.
- Leytem, A.B., R.S. Dungan, D.L. Bjorneberg, and A.C. Koehn. 2011. Emissions of ammonia, methane, carbon dioxide, and nitrous oxide from dairy cattle housing and manure management systems. J. Environ. Qual. 40:1383–1394. doi:10.2134/jeq2009.0515
- Leytem, A.B., R.S. Dungan, D.L. Bjorneberg, and A.C. Koehn. 2013. Greenhouse gas and ammonia emissions from an open-freestall dairy in Southern Idaho. J. Environ. Qual. 42:10–20. doi:10.2134/jeq2012.0106
- Li, X., R. Zhang, and Y. Pang. 2008. Characteristics of dairy manure composting with rice straw. Bioresour. Technol. 99:359–367. doi:10.1016/j.biortech.2006.12.009
- Lovanh, N., J. Warren, and K. Sistani. 2010. Determination of ammonia and greenhouse gas emissions from land application of swine slurry: A comparison of three application methods. Bioresour. Technol. 101:1662–1667. doi:10.1016/j.biortech.2009.09.078
- Luo, J., and S. Saggar. 2008. Nitrous oxide and methane emissions from a dairy farm stand-off pad. Aust. J. Exp. Agric. 48:179–182. doi:10.1071/EA07242
- Maag, M., and F.P. Vinther. 1996. Nitrous oxide emission by nitrification and denitrification in different soil types and at different soil moisture contents and temperatures. Appl. Soil Ecol. 4:5–14. doi:10.1016/0929-1393(96)00106-0
- Misselbrook, T.H., J. Webb, D.R. Chadwick, S. Ellis, and B.F. Pain. 2001. Gaseous emissions from outdoor concrete yards used by livestock. Atmos. Environ. 35:5331–5338. doi:10.1016/S1352-2310(01)00289-8
- Monteny, G.J., A. Bannink, and D. Chadwick. 2006. Greenhouse gas abatement strategies for animal husbandry. Agric. Ecosyst. Environ. 112:163–170. doi:10.1016/j.agee.2005.08.015
- Mukhtar, S., A. Mutlu, S.C. Capareda, and C.B. Parnell. 2008. Seasonal and spatial variations of ammonia emissions from an open-lot dairy operation. J. Air Waste Manage. Assoc. 58:369–376. doi:10.3155/1047-3289.58.3.369
- Nkongolo, N.V, S. Johnson, K. Schmidt, and F. Eivazi. 2010. Greenhouse gases fluxes and soil thermal properties in a pasture in central Missouri. J. Environ. Sci. 22:1029–1039. doi:10.1016/S1001-0742(09)60214-X
- Owen, J.J., and W.L. Silver. 2014. Greenhouse gas emissions from dairy manure management: A review of field-based studies. Global Change Biol. 21:550–565. doi:10.1111/gcb.12687
- Parker, D., J. Ham, B. Woodbury, L. Cai, M. Spiehs, S.T. Rhoades, K. Casey, R. Todd, and A. Cole. 2013. Standardization of flux chamber and wind tunnel flux measurements for quantifying volatile organic compound and ammonia emissions from area sources at animal feeding operations. Atmos. Environ. 66:72–83. doi:10.1016/j.atmosenv.2012.03.068
- Pattey, E., M.K. Trzcinski, and R.L. Desjardins. 2005. Quantifying the reduction of greenhouse gas emissions as a result of composting dairy and beef cattle manure. Nutr. Cycl. Agroecosyst. 72:173–187. doi:10.1007/s10705-005-1268-5
- Pereira, J., D. Fangueiro, T.H. Misselbrook, D.R. Chadwick, J. Coutinbo, and H. Trindade. 2011. Ammonia and greenhouse gas emissions from slatted and solid floors in dairy cattle houses: A scale model study. Biosyst. Eng. 109:148–157. doi:10.1016/j.biosystemseng.2011.02.011
- Pereira, J., T.H. Misselbrook, D.R. Chadwick, J. Coutinbo, and H. Trindade. 2012. Effects of temperature and dairy cattle excreta characteristics on potential ammonia and greenhouse gas emissions from housing: A laboratory study. Biosyst. Eng. 112:138–150. doi:10.1016/j.biosystemseng.2012.03.011
- Petersen, S.O., and S.G. Sommer. 2011. Ammonia and nitrous oxide interactions: Roles of manure organic matter management. Anim. Feed Sci. Technol. 166:503–513. doi:10.1016/j.anifeedsci.2011.04.077
- Shi, P. 2009. Study on determination of pollutants producing coefficient and pollutants discharge coefficient of large scale dairy farm [in Chinese with English abstract]. Master’s thesis. Henan Agricultural University, Zhengzhou, Henan, China.
- Sommer, S.G., S.M. McGinn, X. Hao, and F.J. Larney. 2004a. Techniques for measuring gas emissions from a composting stockpile of cattle manure. Atmos. Environ. 38:4643–4652. doi:10.1016/j.atmosenv.2004.05.014
- Sommer, S.G., S.O. Petersen, and H.B. Møller. 2004b. Algorithms for calculating methane and nitrous oxide emissions from manure management. Nutr. Cycl. Agroecosyst. 69:143–154. doi:10.1023/B:FRES.0000029678.25083.fa
- U.S. Environmental Protection Agency. 2012. Summary Report: Global Anthropogenic Non-CO2 Greenhouse Gas Emissions: 1990–2030. Washington, DC: Office of Atomospheric Programs, Climate Change Division, U.S. Environmental Protection Agency.