ABSTRACT
Odor pollution is a big environmental problem caused by large-scale livestock production in China, and developing a practical way to reduce these odors is pressing. In this study, a combination of 0.2–1.0 U/mL lignin peroxidase (LiP) and one of three peroxides (H2O2, CaO2, 2Na3CO3·3H2O2) was examined for its efficiency in reducing the release of eight chemicals (propionic acid, isobutyric acid, isocaproic acid, isovaleric acid, phenol, p-cresol, indole, and skatole), NH3, H2S, and odor intensity from pig manure. The results showed an approximately 90% reduction in p-cresol, 40–60% reduction in odor intensity, 16.5–40% reduction in indolic compounds, and 25–40% reduction in volatile fatty acids. Being the electron acceptors of LiP, 2Na3CO3·3H2O2 and CaO2 performed better than H2O2 in reducing the concentration of eight chemicals, NH3, H2S, and odor intensity from pig manure. The effect of deodorization can last for up to 72 hr.
Implications: In China, one of the major environmental problems caused by confined feeding is odor pollution, which brings a major threat to the sustainability, profitability, and growth of the livestock industry. To couple the LiP with the electron acceptors, a low–cost, simple, and feasible method for odor removal was established in this study. Based on the study results, a practical treatment method was provided for odor pollution and supply the farm operators a more flexible time to dispose treated manure.
Introduction
Over the past few decades in China, livestock production has grown rapidly with a significant transition in feeding methods. The traditional small-farm practice of raising a few pigs is sharply declining (Ye et al., Citation2009), and the development of so-called confined pig-feeding operations has increased tremendously in recent years. On the traditional small farm, pigs were kept in pens where straw bedding absorbed manure; however, in high-density and confined feeding operations, the collection, storage, transport, and disposal of large amounts of pig manure becomes a serious management and environmental issue. One of the major environmental problems caused by confined feeding is odor pollution resulting from the decomposition of pig manure (Yan et al., Citation2013). Pig manure consists of concentrated organic materials, and the decomposition of these materials produces malodorous, volatile, low-molecular-weight compounds (e.g., volatile fatty acids [VFAs]), aromatic compounds (e.g., phenols, indoles), cresols, NH3, and H2S. More than 160 odorous compounds have been identified from livestock manures (O’Neill et al., 1992).
In China, the odors from livestock operations captive in a building are mainly caused by storing and transporting manure. Nearly 50% of all odor emissions can be traced back to manure storage units. When manure is stored, the strength of the odor increases, as does the concentration of malodorous compounds, which include those already mentioned (Williams et al., Citation1981; Zahn et al., Citation1997; Conn et al., Citation2007). In addition to being a nuisance and unpleasant, a high concentration of odor in a confinement building could lower the growth rate of livestock, increase the incidence of infection, and greatly affect the health of farm workers (Wood et al., Citation2001; Campagna et al., Citation2004; Ryer-Power, Citation1991). The public, farm operators, legislative branches, and environmental regulators have become increasingly concerned about odor because it brings a major threat to the sustainability, profitability, and growth of the livestock industry; therefore, it is necessary to establish a feasible approach for odor pollution control.
A few of methods have been applied to eliminate livestock manure odor, such as manure additives, diet modifications, aeration of manure, and odor ozonation (Hobbs et al., 1995; Wang et al., Citation2009; Sneath et al., Citation1992; Burton et al., 1995; Zhang et al., Citation2006); however, these solutions were neither convenient nor cost-effective. A cost-effective, convenient, and effective method by which to control odor from intensive livestock production is needed. The proposed enzymatic methods have generated great interest recently. Oxidoreductase, such as lignin peroxidase (LiP), and horseradish peroxidase (HRP) have been demonstrated to remove phenolic and nonphenolic aromatic compounds or transform those compounds into less toxic polymers, such as transforming phenols in industrial wastewater (Garyet al., 1983; Dec et al., Citation1994; Roper et al., Citation1995; Tonegawa et al., Citation2003). Subsequently, several other oxidoreductases, such as tyrosinase and laccase, have been successfully tested (Dec et al., Citation1994; Shuttleworth et al., Citation1986). Great progress has been made toward elucidating the mechanism of this so-called oxidative coupling reaction (Dec et al., Citation1994), but so far, LiP is the most widely studied enzyme used in the decontamination and pretreatment of lignocellulosic materials processes. The target phenol pollutants in the already-mentioned decontamination investigations are also known as the major odorants in pig manure (O’Neill et al., Citation1992; Ye et al., Citation2009). Gary et al. (Citation2003) applied the LiP to successfully detoxify the wastewater containing toxic halogenated phenols. Govere et al. (Citation2005) used minced horseradish roots containing large amounts of HRP to successfully deodorize pig manure. In addition, Ye et al. (Citation2009) used pure HRP to successfully deodorize pig manure. The objective of this study was to evaluate the application of LiP coupled with different peroxide (H2O2, CaO2, and 2Na3CO3·3H2O2) treatments for odor removal. The success of odor removal is estimated by the reduction of odor intensity and by the concentrations of two phenolic compounds (phenol and p-cresol), four VFAs (propionic acid, isobutyric acid, isovaleric acid, and isocaproic acid), and two indolic compounds (indole and skatole). These target chemicals were chosen because they were found to be positively correlated with malodors from livestock manure (Zahn et al., Citation1997; Zhu, Citation2000). NH3 and H2S are not always the sensitive components in manure odor, but they are tremendously hazardous for human health (Heber et al., Citation2002; Schiffman et al., Citation2001). Consequently, they are always used as evaluating indicators and were chosen as such in this study to evaluate effective odor removal. As known, LiP is easily produced by fermentation using functional strains, and the electron acceptors H2O2, CaO2, and 2Na3CO3·3H2O2 are inexpensive and environmentally safe. To couple the LiP with the electron acceptors, a low-cost, simple, and feasible method for odor removal was established in this study. Based on the study results, a practical treatment method was provided for odor pollution caused by intensive livestock and poultry farms in China.
Materials and methods
Pig manure treatment using LiP coupled with different peroxides
LiP was prepared in our laboratory including the following steps: The strain J-1 stored in our lab was inoculated into corncob solid medium, and incubated at 30°C for 7 days. The solid culture added by distilled water was centrifuged; the supernatant was the crude enzyme of LiP. 2Na2CO3·3H2O2 (powder, 99% w/w) and CaO2 (powder, 60% w/w) were purchased from Sigma-Aldrich Co. (St Louis, MO); H2O2 (30% w/w) was purchased from Tianjin Bodi Chemical Reagent Co. Ltd., Tianjin, China. The LiP solution was prepared and stored at 4°C until it was used. H2O2 was diluted when used.
Pig manure samples were collected from a concrete pig manure storage pit at a pig farm in Shuangliu County, Sichuan Province, China. The pig manure was well mixed for 20 min before the samples were collected, and 4.0 kg wet pig manure slurry having a pH of about 7.5 was distributed into 60-L Plexiglas boxes (50 cm long, 30 cm wide, 40 cm high) () and sprayed with 100 mL LiP solution.
Each box was sealed with a water-tight cover. The oxidation–reduction reaction was initiated with the addition of a specific amount of H2O2, CaO2, or 2Na3CO3·3H2O2. The experiments were arranged as follows:
LiP solution at different concentrations (0.2–1.0 U/mL) was sprayed onto the surface of the pig manure, after which it was sprayed with 100 mL H2O2 solution (2.0%) to assess the optimal dosage of LiP.
In nine of the Plexiglas boxes, 100 mL 0.6 U/mL LiP solution was sprayed on the surface of the pig manure, after which 3.1 g of 2Na3CO3·3H2O2 (99%) was sprayed into the first three boxes, 3.53 g CaO2 powder (60%) into the second three boxes, and 100 mL H2O2 solution (1.0%) into the last three boxes.
One hundred milliliters of 0.6 U/mL LiP solution was sprayed on the surface of the pig manure, after which it was sprayed with 2.0–10.0 g 2Na3CO3·3H2O2 powder to investigate the optimal dosage of 2Na3CO3·3H2O2.
One hundred milliliters of 0.6 U/mL LiP solution was sprayed on the surface of the pig manure, after which it was sprayed with 4.0 g 2Na3CO3·3H2O2 powder. The pig manure was incubated for 12, 24, 48, 72, and 96 hr after treatment to evaluate deodorization time.
In all experiments, three Plexiglas boxes were used as the control and 100 mL distilled water alone was sprayed on the surface of the pig manure. All tests were conducted under a constant room temperature of 30°C. If not specified, the treatment time was 24 hr.
Odor intensity
Odor intensity, NH3, and H2S concentrations were measured using a model SLC-OP020 odor detector purchased from Korea Science and Technology Analyzing Center. The odor detector measures odor intensity (olfactometry), and has been widely used in China to monitor odor.
Odor component extraction and quantification
Eight odor components (propionic acid, isobutyric acid, isocaproic acid, isovaleric acid, phenol, p-cresol, indole, and skatole) were extracted using the modified procedure by Ohta and Ikeda (Citation1978). A sample containing 20 mL pig manure was withdrawn and acidified with 4.0 mL of 1.0 mol L−1 HCl, to which 5.0 mL diethyl ether was added. The odor components were extracted (for 4.0 hr at 4.0°C) into a diethyl ether layer and quantified using gas chromatography using an Agilent Technologies (Santa Clara, CA) 7890A chromatograph with a flame ionization detector. The injection volume was 1.0 µL. The compounds were separated using the HP-5 capillary column (Agilent Technologies, Santa Clara, CA) (30 m, 0.32 mm ID).
Standard samples of the eight chemicals were purchased from Sigma-Aldrich Co. (St. Louis, MO). Each chemical was dissolved in ultrapure methanol. The standard solution was further diluted into five concentrations using pure diethyl ether. Each concentration of standards was prepared in triplicate. The retention times (min), R2 values of calibration curves, percent odorant recoveries, and precision of odorant measurements are shown in .
Table 1. Quantification parameters for the GC method.
Determination of total nitrogen organic nitrogen, ammonium nitrogen, and total sulfur
Two grams of the pig manure sample was withdrawn and dissolved in 20 mL KCl (2.0 mol/L). Ammonium nitrogen (AN) was extracted by shaking the sample at 150 rpm at 30°C for 2.0 hr and centrifuging at 5000 rpm for 10 min. Organic nitrogen (ON) was measured using the Kjeldahl method (Kjeldahl, Citation1883). Total nitrogen (TN) and total sulfur (TS) were determined using the vario MACRO cube elemental analyzer (Elementar, Hanau, Germany).
Results and discussion
Different concentrations of LiP treatment with 2.0% hydrogen peroxide solution
The concentrations of each compound and the odor intensities in the treated and control pig slurry (i.e., 0 U/mL LiP concentration) are presented in and .
Figure 2. Concentration (mg/L) of odorants and odor intensity in pig manure treated with different concentrations of LiP and with the same 2.0% H2O2 solution. (a) Concentration of volatile fatty acids and odor intensity. (b) Concentration of phenols and indoles.
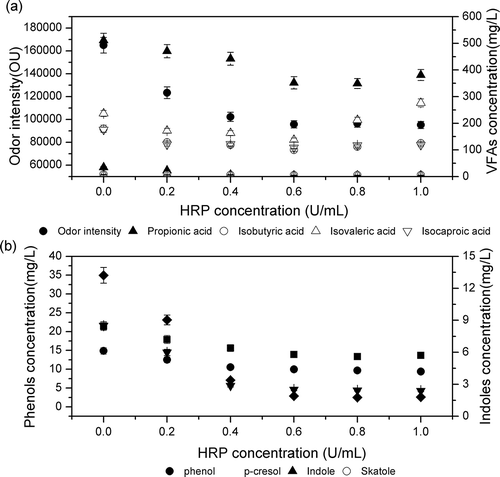
The results show that odor intensity was reduced in the eight compounds with an increase in the concentration of LiP; however, when the concentration of LiP was >0.6 U/mL, the odor reduction was not significant. When the pig manure was treated with 0.6 U/mL LiP and 6.0% H2O2, the removal rates of phenolic compounds, indolic compounds, and VFAs were 86.8, 30.1, and 36.7%, respectively, and odor intensity of the pig slurry was reduced by 41.94%. The treatment of LiP combined with H2O2 resulted in the highest (91.7%) removal of p-cresol. The results show that LiP with peroxides can significantly reduce the odor from pig manure, and that the optimum concentration of HRP is 0.6–0.8 U/mL, or 15–20 U/kg pig manure.
This result was different than that reported by Ye at al. (Citation2009), who found that the optimum dosage of LiP was 7.5–15 U per kg pig manure. The results also implied that odor intensity was not correlated only with single chemicals but that many chemicals contributed to pig manure odor. Spoelstra (Citation1980) considered p-cresol and VFAs to be the most suitable indicators of that odor. Williams (Citation1984) found that phenols, indoles, and VFAs correlated well with odor offensiveness of aerobically stored pig manure. Even with a single type of chemical, investigations have shown that different VFAs will have a different contribution to odor generation and that this also depends on the types and characteristics of certain acids, which do not necessarily exist in high concentrations in manure. The proportion of an individual VFA to total VFA concentration has been deemed extremely significant in relation to odor offensiveness (O’Neill et al., Citation1992). The VFAs responsible for odor are those with long or branching carbon chains (Spoelstra, Citation1980); therefore, one linear and three branching VFAs were chosen as odor indicators in this study.
According to previous reports, it is reasonable to choose several kinds of chemicals as indicators of pig manure odor. In addition, it is clear that the decrease in VFA and indolic odorant concentration was relatively unlike that of phenolic odorants. Previous studies indicated that the phenolic and nonphenolic aromatic compounds can be oxidized with a free radical chain reaction by LiP (Kersten et al., Citation1990; Roper et al., Citation1995). Phenolic compounds are generally the substrates preferred over indolic compounds and VFAs for LiP. Accordingly, when more reactive phenolic compounds are oxidized by LiP, the intermediate aromatic radicals, in turn, react with less reactive indolic compounds and VFAs; however, even if free aromatic radicals are produced by the oxidation of phenolic and nonphenolic aromatic compounds, large amounts of organic matter contained in pig manure might intercept the free radicals reaction with indolic compounds and VFAs (Govere et al., Citation2005). It is well known that natural organic matter can act as the major reservoir for hydroxyl free radicals, and the same might apply to free aromatic radicals (Schwarzenbach et al., Citation1993). In addition, Lindsey et al. (Citation2000) reported that the natural organic matter including humic and fulvic acids inhibits the degradation of aromatic compounds by means of the free hydroxyl radical, such as phenol and o-cresol; however, they suggested that the most likely reason for the observed inhibition was a result of decreased reactivity of aromatic compounds through the partitioning to natural organic matter and not the scavenging of the hydroxyl radical by natural organic matter.
The potential phenomenon of LiP treatment involves enzymatic oxidation of phenolic compounds, which leads to the formation of reactive phenoxyl radicals. The subsequent coupling of the oxidation products is completed without further involvement of peroxidase. The contaminants are transformed to less toxic polymers with this so-called oxidative coupling, both of which were expected to reduce the toxicity of the parent compounds.
Different electron acceptor treatments (CaO2, 2Na3CO3·3H2O2, and H2O2) with 0.6 U/mL LiP
, , and shows that the three different electron acceptors have different effects on the deodorization of LiP.
Figure 3. Concentration (mg/L) of odorants and odor intensity in pig manure treated with 0.6 U/mL LiP and with different electron acceptor treatments (CaO2, 2Na3CO3·3H2O2, and H2O2). (a) Odor intensity. (b) Concentration of volatile fatty acids. (c) Concentration of phenols and indoles.
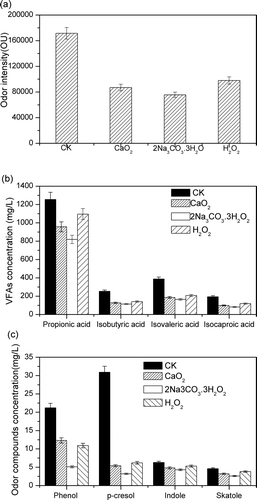
The concentrations of odor intensity, VFAs, phenolic compounds, and indolic compounds were reduced by 55.9, 43.6, 84.1, and 36.7%, respectively, when LiP was combined with 2Na3CO3·3H2O2 powder, but the concentrations of odor intensity, VFAs, phenolic compounds, and indolic compounds were reduced by 49.2, 34.6, 66.0, and 26.6%, respectively, when combined with CaO2 powder, and by 42.8, 25.2, 67.2, and 16.5%, respectively, when sprayed with H2O2 solution. According to these described deodorization tests on different electron acceptors, it appears that 2Na3CO3·3H2O2 and CaO2 are more effective than H2O2 in reducing the concentration of odor indicators in pig manure; therefore, either 2Na3CO3·3H2O2 or CaO2 can be selected as the optimum electron acceptor. As is known, H2O2 quickly decomposes once in contact with dissolved or particulate organic matter. Better performances of 2Na3CO3·3H2O2 and CaO2 might be because of the slow release of H2O2 from 2Na3CO3·3H2O2 or CaO2 powder into the aqueous phase of the pig manure (Govere et al., Citation2005). Flanders et al. (Citation1999), who investigated the similar enzyme to horseradish peroxidase, horseradish-mediated binding of 14C-labeled 2,4-dichlorophenol to soil, found that horseradish-mediated binding was enhanced by a factor of 2 when CaO2 was used instead of H2O2.
Different dosage of 2Na3CO3·3H2O2 treatment with 0.6 U/mL LiP
The results show that an increase in 2Na3CO3·3H2O2 dosage from 4.0 to 6.0 g had a significant effect on the concentration of VFAs and phenolic and indolic compounds and on odor intensity ( and ), but the effects on VFAs, phenolic and indolic compounds, and odor intensity were not significant when treated with >6.0 g 2Na3CO3·3H2O2.
Figure 4. Concentration (mg/L) of odorants and odor intensity in pig manure treated with different concentrations of 2Na3CO3·3H2O2 (2.0–10.0 g) and the same concentration of LiP (0.6 U/mL). (a) Concentration of volatile fatty acids and odor intensity. (b) Concentration of phenols and indoles.
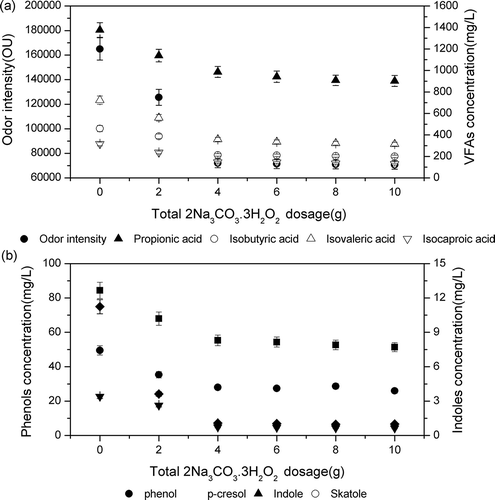
The concentrations of VFAs and phenolic and indolic compounds and the odor intensity of pig slurry after being treated with 4.0 g 2Na3CO3·3H2O2 were reduced by 40.7, 87.7, 37.8, and 56.4%, respectively; consequently, the suggested concentration of 2Na3CO3·3H2O2 is 4.0–6.0 g with economic considerations.
Duration of deodorization effect using LiP and 2Na3CO3·3H2O2 treatment
Odor is the primary by-product of the anaerobic decomposition of manure. In China, one of the more frequent sources of odor is manure storage on livestock farms. Regulations from Ministry of Environmental Protection, P.R. China, recommend that the manure be treated and disposed as soon as possible, but posttreatment manure storage is necessary in the highly centralized and congested livestock farm; therefore, it was essential to determine how long the deodorization effect would last during manure storage after being treated with LiP and peroxides. The results show that odorant concentration and odor intensity are not significantly different after 24- and 48-hr action times, and increase only slightly after 72 hr, but approach those of the untreated sample after 96 hr (, , and ).
Figure 5. Concentrations (mg/L) of odorants and odor intensity in pig manure after treatment for 2, 24, 48, 72, and 96 hr with 0.6 U/mL LiP and 4.0 g 2Na3CO3·3H2O2. (a) Odor intensity. (b) Concentration of volatile fatty acids. (c) Concentration of phenols and indoles.
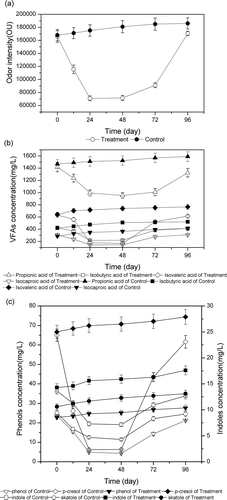
The results suggest that the deodorization effect of treating with LiP coupled with peroxides could provide about 3.0 days for livestock farm workers to dispose the treated manure. This deodorization stability might give farm operators a more flexible time frame during which to dispose of the treated manure by collecting manure from storage tanks, transporting it, and applying it to the field.
The preceding results show that treatment with LiP combined with 2Na3CO3·3H2O2 is the most effective in reducing the concentration of phenolic compounds, especially p-cresol removal. The concentration of VFAs and indolic odorants was not reduced as greatly as that of phenolic odorants because phenolic compounds are the substrates preferred over indolic compounds in terms of oxidation rate. As known, odors are a complex mixture of VFAs, phenolic and indolic compounds, amides, and sulfides (Zahn et al., Citation1997; Williams et al., Citation1981; Conn et al., Citation2007); therefore, it is not very accurate to choose one or two odorants as indicators of odors from pig manure. In this study, it was not confirmed that a significant decrease in the concentration of phenolic compounds was directly related to the decrease in odor intensity, but phenolic compounds were found to be the possible indicators of microbial community changes in pig manure storage systems (Merrill et al., Citation2002).
NH3 and H2S are not always the sensitive components in the odor from livestock manure, but they are tremendously hazardous (Heber et al., Citation2002; Schiffman et al., Citation2001); consequently, they are always used as important evaluating indicators for livestock manure odor. The results show that concentrations of NH3 and H2S from pig slurry after treatment with 4.0 g 2Na3CO3·3H2O2 were reduced by 32.7 and 44.1%, respectively ().
Figure 6. Concentrations of NH3 and H2S volatilized from pig manure and nitrogen and sulfur forms in pig manure. (a) Concentrations of NH3 and H2S. (b) Concentrations of total nitrogen (TN), organic nitrogen (ON), ammonium nitrogen (AN), and total sulfur (TS).
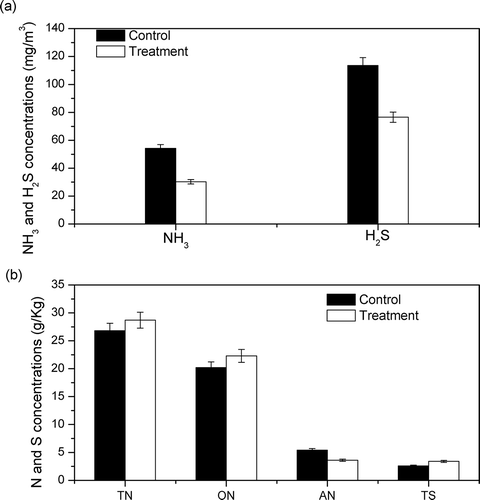
Odor is volatilized from solid manure, and higher concentrations of odor components in solid manure will result in higher concentrations of volatized odor. The odor concentrations from manure are positively correlated with the odor components in the manure.
In this study, all the concentrations of TN, ON, and TS in treatment samples were higher than those in untreated samples (), which implies that the forms of N and S were changed by treating with LiP combined with peroxides, and transformed into ON, nitrite and nitrate, sulfates, or other compounds that are not available for volatilization (Yan et al., Citation2013). Finally, the volatile intensities of NH3 and H2S were reduced with the N and S effectively reserved.
The use of LiP and peroxides to reduce odor from pig manure might be considered an environmentally friendly technique because it does not cause secondary pollution. H2O2, CaO2, and 2Na3CO3·3H2O2 are not only electron acceptors but also provide dissolved oxygen to aerobic bacteria in manure; therefore, the odorous compounds in manure could be actively decomposed by these aerobic bacteria, resulting in odor reduction (Sneath et al., Citation1992). Increasing the pH of manure by adding 2Na3CO3·3H2O2 or CaO2 can weaken the growth of the odor-producing bacteria, thus reducing odor emissions. Depending on the requirement, 2Na3CO3·3H2O2 or CaO2 can be chosen as the additive in removing the odor from pig manure. If the pig manure will be treated and used as a fertilizer, it is suggested that CaO2 be used. If the pig manure will be disposed to incineration or a landfill, it is suggested that 2Na3CO3·3H2O2 be used.
Conclusion
The results obtained from this study indicate that odor emission from pig manure can be reduced by using LiP combined with peroxides. All treatments reduced odor intensity by 40–60%, and phenolic compounds by approximately 90%, but the reduction of indolic and VFA compounds in pig manure was not as significant as that of phenolic compounds in this study. As the electron acceptors of LiP, 2Na3CO3·3H2O2 and CaO2 performed better than H2O2 in reducing the concentration of eight chemicals, NH3, H2S, and odor intensity from pig manure. The deodorization effect could last up to 72 hr, which can give farm operators a more flexible time frame during which to dispose of treated manure.
By comparisons with another similar study for HRP, the LiP is easily produced by biological fermentation, and the electron acceptors, CaO2, and 2Na3CO3·3H2O2 are inexpensive and environmentally safe. Thus, with LiP coupled with the electron acceptors, a low-cost, simple, and feasible method for odor removal was established in this study. Based on the study results, a practical treatment method was provided for odor pollution caused by intensive livestock and poultry farms in China.
Funding
This work was supported by the International Science & Technology Cooperation Program of China (2013DFA61260), the Key Project of Knowledge Innovation Program of the Chinese Academy of Sciences (KSCX2-EW-G-15-02; KSCX2-EW-G-15-01), and the Chinese Academy of Environmental and Application of Microbial Key Laboratory Fund Project (KLCAS-2013-05).
Additional information
Funding
Notes on contributors
ZhiYing Yan
ZhiYing Yan and XiuFeng Liu are professors at the Key Laboratory of Environmental and Applied Microbiology, Chengdu Institute of Biology, Chinese Academy of Science, Environmental Microbiology Key Laboratory of Sichuan Province.
XiuLi Wei
YueXiang Yuan, Dong Li, and ZhiDong Li are associate professors at the Key Laboratory of Environmental and Applied Microbiology, Chengdu Institute of Biology, Chinese Academy of Science, Environmental Microbiology Key Laboratory of Sichuan Province.
YueXiang Yuan
XiuLi Wei is an associate professor at Chongqing Academy of Agricultural Sciences.
ZhiDong Li
YueXiang Yuan, Dong Li, and ZhiDong Li are associate professors at the Key Laboratory of Environmental and Applied Microbiology, Chengdu Institute of Biology, Chinese Academy of Science, Environmental Microbiology Key Laboratory of Sichuan Province.
Dong Li
YueXiang Yuan, Dong Li, and ZhiDong Li are associate professors at the Key Laboratory of Environmental and Applied Microbiology, Chengdu Institute of Biology, Chinese Academy of Science, Environmental Microbiology Key Laboratory of Sichuan Province.
XiuFeng Liu
ZhiYing Yan and XiuFeng Liu are professors at the Key Laboratory of Environmental and Applied Microbiology, Chengdu Institute of Biology, Chinese Academy of Science, Environmental Microbiology Key Laboratory of Sichuan Province.
LiHong Gao
LiHong Gao is a professor at Chongqing Academy of Agricultural Sciences.
References
- Burton, C.H., and R.W. Sneath. 1995. Continuous farm scale aeration plant for reducing offensiveness odours from piggery slurry: Control and optimization of the process. J. Agric. Eng. Res. 60:271–79.doi:10.1006/jaer.1995.1021
- Campagna, D., S.J. Kathman, R. Pierson, S.G. Inserra, B.L. Phipher, D.C. Middleton, G.M. Zarus, and M.C. White. 2004. Ambient hydrogen sulfide, total reduced sulfur, and hospital visits for respiratory diseases in northeast Nebraska. J. Expos. Sci. Environ. Epidemiol. 14:180–87.doi:10.1038/sj.jea.7500313
- Conn, K.L., E. Topp, and G. Lazarovits. 2007. Factors influencing the concentration of volatile fatty acids, ammonia, and other nutrients in stored liquid pig manure. J. Environ. Qual. 36:440–47.doi:10.2134/jeq2006.0222
- Dec, J., and J.M. Bollag. 1994. Use of plant material for the decontamination of water polluted with phenols. Biotechnol. Bioeng. 44:1132–39.doi:10.1002/bit.260440915
- Flanders, C., J. Dec, and J.M. Bollag. 1999. Horseradish-mediated binding of 2,4-dichlorophenol to soil. Bioremediat. J. 3:315–21.doi:10.1080/10889869991219406
- Gary, W., H. Yitzhak, and G. Carlos. 2003. Lignin peroxidase-catalyzed polymerization and detoxification of toxic halogenated phenols. J. Chem. Technol. Biotechnol. 78(2): 1239–48.
- Govere, E.M., M. Tonegawa, M.A. Bruns, E. Wheeler, P.H. Heinemann, K.B. Kephart, and J. Dec. 2005. Deodorization of pig manure using minced horseradish roots and peroxides. J. Agric. Food Chem. 53:4880–89.doi:10.1021/jf0404290
- Heber, A., D. Jones and A. Sutton. 2002. Controlling ammonia gas in pig buildings. http://nasdonline.org (accessed June 2013).
- Hobbs, P.J., J. Webb, T.T. Motram, B. Grant, and T.M. Misselbrook. 2004. Emissions of volatile organic compounds originating from UK livestock agriculture. J. Sci. Food Agric. 84:1414–20.doi:10.1002/jsfa.1810
- Kersten, P.J. 1990. Glyoxal oxidase of Phanerochaete chrysosporium: Its characterization and activation by lignin peroxidase. Proc. Natl. Acad. Sci. USA 87:2936–40.doi:10.1073/pnas.87.8.2936
- Kjeldahl, J. 1883. New method for the determination of nitrogen in organic substances. Z. Anal. Chem. 22(1): 366–83.doi:10.1007/BF01338151
- Klibanov, A.M., T.M. Tu, and K.P. Scott. 1983. Peroxidase-catalyzed removal of phenols from coal-conversion wastes waters. Science 221:259–60.doi:10.1126/science.221.4607.259-a
- Lindsey, M.E., and M.A. Tarr. 2000. Inhibition of hydroxyl radical reaction with aromatics by dissolved natural organic matter. Environ. Sci. Technol. 34:444–49.doi:10.1021/es990457c
- Merrill, L., and L.J. Halverson. 2002. Seasonal variation in microbial communities and organic malodor indicator compound concentrations in various types of pig manure storage systems. J. Environ. Qual. 31:2074–85.doi:10.2134/jeq2002.2074
- Ohta Y., and M. Ikeda. 1978. Deodorization of pig feces by actinomycetes. Appl. Environ. Microbiol. 36:487–91.
- O’Neill, D.H., and V.R. Phillips .1992. A review of the control of odor nuisance from livestock buildings. Part 3. Properties of the odorous substances which have been identified in livestock wastes or in the air around them. J. Agric. Eng. Res. 53:23–50.doi:10.1016/0021-8634(92)80072-Z
- Roper, J.C., J.M. Sarkar, J. Dec, and J.M. Bollag. 1995. Enhanced enzymatic removal of chlorophenols in the presence of co-substrates. Water Res. 29:2720–24.doi:10.1016/0043-1354(95)00101-P
- Ryer-Power, J.E. 1991. Health effects of ammonia. Plant Oper. Prog. 10:228–32.doi:10.1002/prsb.720100411
- Schiffman, S.S., B.W. Auvermann, and R.W. Bottcher. 2001. Health effects of aerial emissions from animal production waste management systems. Proc. Int. Symp. on Production and Environmental Issues, Research Triangle Park, NC.
- Schwarzenbach, R.P., P.M. Gschwend, and D.M. Imboden. 1993. Environmental Organic Chemistry. New York, NY: John Wiley & Sons.
- Shuttleworth, K.L., and J.M. Bollag. 1986. Soluble and immobilized laccase as catalysts for the transformation of substituted phenols. Enzyme Microb. Technol. 8:171–77.doi:10.1016/0141-0229(86)90108-0
- Sneath, R.W., C.H. Burton, and A.G. Williams. 1992. Continuous aerobic treatment of piggery slurry for odour control scaled up to a farm-size unit. J. Agric. Eng. Res. 53:81–92.doi:10.1016/0021-8634(92)80075-4
- Spoelstra, S.F. 1980. Origin of objectionable odorous components in piggery wastes and the possibility of applying indicator components for studying odor development. Agric. Environ. 5: 241–60.doi:10.1016/0304-1131(80)90004-1
- Tonegawa, M., J. Dec, and J.M. Bollag. 2003. Use of additives to enhance the removal of phenols fromwater treated with horseradish and hydrogen peroxide. J. Environ. Qual. 32:1222–27.doi:10.2134/jeq2003.1222
- Wang, Y., J.H. Cho, Y.J. Chen, J.S. Yoo, Y. Huang, H.J. Kim, and I.H. Kim. 2009. The effect of probiotic BioPlus 2B® on growth performance, dry matter and nitrogen digestibility and slurry noxious gas emission in growing pigs. Livestock Sci. 120:35–42.doi:10.1016/j.livsci.2008.04.018
- Williams, A.G. 1984. Indicators of piggery slurry odor offensiveness. Agric. Wastes 10:15–36.doi:10.1016/0141-4607(84)90016-7
- Williams, A.G., and M.R. Evans. 1981. Storage of piggery slurry. Agric. Wastes 3:311–21.doi:10.1016/0141-4607(81)90017-2
- Wood, S.L., K.A. Janni, C.J. Clanton, D.R. Schmidt, L.D. Jacobson, and S. Weisberg. 2001. Odor and air emissions from animal production systems. Paper no. 014043. St. Joseph, MI: ASAE.
- Yan, Z.Y., X.F. Liu, Y.X. Yuan, Y.Z. Liao, and X.D. Li. 2013. Deodorization study of the swine manure with two yeast strains. Biotechnol. Bioproc. Eng. 18:135–43.doi:10.1007/s12257-012-0313-x
- Ye, F.X., R.F. Zhu, and Y. Li. 2009. Deodorization of pig manure slurry using horseradish peroxidase and peroxides. J. Hazard. Mater. 167:148–53.doi:10.1016/j.jhazmat.2008.12.096
- Zahn, J.A., J.L. Hatfield, Y.S. Do, A.A. DiSpirito, D.A. Laird, and R.L. Pfeiffer.1997. Characterization of volatile organic emission and wastes from a pig production facility. J. Environ. Qual. 26:1687–96.doi:10.2134/jeq1997.00472425002600060032x
- Zhang, Z.J., J. Zhu, and K. Park. 2006. A bench-scale aeration study using batch reactors on pig manure stabilization to control odor in post treatment storage. Water Res. 40:162–74.doi:10.1016/j.watres.2005.11.004
- Zhu, J. 2000. A review of microbiology in pig manure odor control. Agric. Ecosyst. Environ. 78:93–106.doi:10.1016/S0167-8809(99)00116-4