ABSTRACT
Inorganic emissions from livestock production and subsequent deposition of these ions can be a major source of pollution, causing nitrogen enrichment, eutrophication, acidification of soils and surface waters, and aerosol formation. In the poultry house, ammonia and hydrogen sulfide emissions can also adversely affect the health, performance, and welfare of both animals and human operators. The persistence and long life expectancy of ammonia, odors and toxic pollutants from poultry houses may be due to the ability of suspended particulate matter (SPM) to serve as carriers for odorous compounds such as ammonium ions and other inorganic compounds (e.g., phosphate, sulfate, nitrate, etc.). SPM is generated from the feed, animal manure, and the birds themselves. A large portion of odor associated with exhaust air from poultry houses is SPM that has absorbed odors from within the houses. Understanding the fate and transport processes of ammonia and other inorganic emissions in poultry houses is a necessary first step in utilizing the appropriate abatement strategies. In this study, the examination and characterization of ammonium ions, major components of odors and toxic gases from poultry operations, and other ions in suspended particulate matter in a broiler house were carried out using particle trap impactors. The SPM from the particle trap impactors was extracted and analyzed for its ionic species using ion chromatography (IC). The results showed concentrations of polyatomic ions in suspended particulate matter were found to increase with successive flocks and were highly concentrated in the larger size particulate matter. In addition, the ions concentrations appeared to reach a maximum at the middle of flock age (around the fourth week), tapering off toward the end in a given flock (possibly due to ventilation rates to cool off larger birds). Thus, it can be inferred that aged of bedding materials affects the ionic concentrations in aerosol particulate matter more than the age of the birds.
Implications: In the poultry house, toxic gas emissions can adversely affect the health, performance, and welfare of both animals and human operators. The persistence of these toxic pollutants from poultry houses may be due to the ability of suspended particulate matter (SPM) to serve as carriers for these compounds (inorganic ions). Our study showed that polyatomic ions in suspended particulate matter were found to increase with successive flocks and were highly concentrated in the larger size SPM. Understanding the effect of management practices on poultry air emissions will lead to innovative best management practices to safeguard the health and welfare of the animals as well as those of the poultry operators, along with reducing the impact of potential air pollution on the environment.
Introduction
The increase in human population has created a parallel increase in demand for agricultural commodities. Satisfying the demand for food has already altered the way we raised crops and carried out livestock productions that had a confounding effect on the environment. For instance, the increased consumption of animal protein in developed and developing countries has resulted in concentrated production of poultry and other livestock, which has led to concentrated emissions of odorous pollutants and fugitive dust from these production facilities and has created a few concerns regarding health and welfare of animals as well as humans in or near these facilities. Odor from animal feeding operations is not caused by a single compound, but rather a large number of compounds, which include ammonia, volatile organic compounds, and sulfur compounds (National Academy of Sciences, Citation2003). Recently the World Health Organization (WHO) concluded that there is a causal relationship between suspended particulate matter exposure and health effects (WHO, 2003). Studies to date show that particulates (especially from industrial and agricultural sources) are strongly associated with mortality and other endpoints such as hospitalization for cardiopulmonary diseases (Pope et al., Citation2002; Hoek et al., Citation2002; Zanobetti et al., Citation2002). Suspended particulate matter in livestock buildings may carry biochemical agents such as bacteria, viruses, and odors (Hartung, Citation1985; Baekbo, Citation1989; Williams, Citation1989; Homes et al., Citation1996; Lovanh et al., Citation2012).
For livestock production, especially poultry operations, the emission of such particulate matter has a similar detrimental effect on animal and human health (Dosman et al., Citation1987; Iversen et al., Citation1988; Whyte and Williamson, Citation1993; Donham et al., Citation1984; Iversen and Pedersen, Citation1990; Hayter and Besch, Citation1974). The main concern at the local level, however, is the persistent odorous smell from poultry operations. The persistence and long life expectancy of odors and toxic pollutants from poultry houses may be due to the ability of suspended particulate matter (SPM) to serve as carriers for odorous compounds such as volatile fatty acids (VFA). SPM is generated from the feed, animal manure, and the birds themselves. A large portion of odor associated with exhaust air from poultry houses is SPM that has absorbed odors from within the houses. The air in a broiler house contains all of the materials emitted to the atmosphere, some of which are potential contaminants such as VFA, and inorganic ions such as phosphate, nitrate, sulfate, and ammonium in SPM. The emission rate of any specific contaminant from these poultry houses is the rate at which pollutants are expelled into the surrounding atmosphere and is the product of the concentration of the contaminant inside the houses and the rate of ventilation. Livestock operations are believed to contribute significantly to regional air pollution by emissions of ammonia and suspended particulate matter. Concentrated animal feeding operations (CAFO) in many countries are exempt from environmental regulations that industrial sources are under. As a result, the emissions from CAFO are poorly characterized, especially in the poultry sector.
Each type of poultry operation (e.g., layers, pullet, broiler, and etc.) generates different amounts of air pollutants into the atmosphere. Their emission rates are dependent on the different house management and operation practices. Data involving the effect of aged bedding materials on SPM generated along with inorganic ions that were excreted or formed in poultry houses are scarce and incomplete, especially in a poultry house using rice hulls as bedding materials. Therefore, the objective of this study was to examine and characterize the effect of aging litter materials on the indoor air quality of a broiler house (poultry operation to raise chickens for meat), in particular the distribution of various inorganic ions in fractionated particulate matter that may have important environmental impact. For instance, the deposition of ammonium ions can cause nitrogen enrichment and acidification of soils and surface waters. Although sulfate and nitrate have a negative radiative forcing (the quantitative strength of aerosol agents or greenhouse gases effect on climate change), they have other effects on the environment (Intergovernmental Panel on Climate Change [IPCC], Citation2007; Charlson et al., Citation1992). For example, sulfate can form acid rain. Nitrate, on the other hand, can cause blue baby syndrome in drinking water. Nitrate can also serve as a precursor to nitrite formation during the denitrification process, which leads to nitrous oxide (a greenhouse gas) production. Phosphate and nitrate can also form secondary particulate matter with other cations (e.g., ammonium) and be carried off site. The accumulation of phosphate and nitrate can also cause eutrophication in waterways (Selman, Citation2007). On the other hand, if the already-mentioned ions could be retained in the poultry litter and not lost to the environment via air pollution, this could increase the value of the litter as fertilizer for crops production. Thus, understanding the effect of management practice on the indoor and, consequently, the outdoor air emissions will lead to innovative best management practices for poultry operation and reduce the impact of livestock air pollution on animal health, human health, and the surrounding environment. In addition, the enhanced knowledge would lead to better estimates of inventory and emission factors of these environmental pollutants from a different type of poultry operation. However, the main focus of this study is on the broiler housing air emissions.
Materials and methods
Suspended particulate matter sampling
Air samples were taken inside a broiler house at 1.0 m above the floor using a deployable particulate sampler system (Leland Legacy sampling system and Airchek XR 5000 from SKC, Inc., Fullerton, CA) that consists of particle trap impactor based on venturi orifice, pump with volumetric flow controller, and nitrocellulose filters (). Air samplings were carried out once a week for the duration of four flocks (8 months) from February to October. Total cleanout of bedding materials occurred after the fourth flock when chickens were placed on new bedding materials. The sampling location was at the midpoint of the poultry house. The poultry houses (eight houses) were located in Kentucky, where samples were taken. Rice hulls were used as bedding materials for these poultry houses. The air temperature inside the house during brooding ranged from 29 to 32ºC, and 18 to 22ºC for the other period. During the brooding period (0–15 days), approximately 30,000 birds were placed in each house and were confined to the front half of the house to provide the young chicks with the ideal growing conditions. Once the young chicks matured to about 2 weeks old, they were then allowed to spread out throughout the house (20 m × 145 m). Deployable particulate sampler systems (DPSS) with Leland Legacy sample pumps (SKC, Inc., Fullerton, CA) were used to pull air (10 L/min) through nitrocellulose filters (1.2 μm; SKC, Inc.) for 2 hr. Samples were taken in duplicate and sometime in triplicate simultaneously. The DPSS were equipped with impactor heads with venturi orifices of 2.5 and 10.0 µm for sampling of particulate matter of aerodynamic diameters of less than 2.5 and 10.0 µm, respectively. For the total inhalable fraction (TIH) or total suspended particulate (TSP), the filters were housed in round cassettes (37 mm ID; Omega, MA) with inlet orifice of 4 mm in diameter. These filters meet Occupational Safety and Health Administration (OSHA) and National Institute for Occupational Safety and Health (NIOSH) specifications. The filters were oven dried at 105ºC for about 2 hr to remove any moisture before use. The collected SPM were quantified in terms of the net mass gained, airflow rate, and sampling time. The mass of SPM was determined using an analytical balance (sensitivity = 0.01 mg; Denver Instrument). The collected samples were transported using airtight petri dish slides and analyzed immediately upon arrival at the lab. Inorganic ions were extracted from the particulate matter and analyzed using ion chromatography (IC) (ICS 3000, Dionex Corporation, San Francisco, CA). Statistical analysis was performed using STATISTICA (ver. 7.0, Statsoft, Tulsa, OK). Tukey’s test was used to determine the significant differences among the means of inorganic ion concentrations at the 5% significance level.
IC analyses of polyatomic ions
Total suspended particulate samples were extracted in deionized water and analyzed using IC. The amount of water used for each extraction was the same. Anions were determined by IC (ICS 3000, Dionex Corporation, San Francisco, CA). Ten milliliters of each sample was filtered through a 0.2 µm-pore-size filter (Corning, Inc). The filtered sample (25 µL) was injected into IC via an autosampler (model AS-1, Dionex Corporation). AS22 eluent reagent (Dionex Product number 063965, 1%, AS22, Dionex Corp, Sunnyvale, CA) was used as the mobile phase for an isocratic run. The mobile phase was pumped at 1.0 mL/min through a 50 mm × 4.00 mm IonPac AG22 Guard and 250 mm × 4.00 mm IonPacAS22 analytical columns (Dionex Corp, Sunnyvale, CA) held at a temperature of 25ºC to a conductivity detector (model number 061830, Dionex Corporation) and an anion self-regenerating suppressor. Cations were determined in the similar fashion as the anions, except methanesulfonic acid (20 mM, Sigma-Aldrich, St. Louis, MO) was used as mobile phase for an isocratic run. The mobile phase was pumped at 1.2 mL/min through a 50 mm × 4.00 mm IonPac CG12A Guard and 250 mm × 4.00 mm IonPac CS12A analytical columns (Dionex Corp, Sunnyvale, CA) held at a temperature of 25ºC to a conductivity detector (model number 061830, Dionex Corporation) and a cation self-regenerating suppressor.
Filtration materials
Four different filter materials were used to test their capacity to retain suspended particulate matter (SPM) in the broiler house. The air inside the poultry house was sampled using impinger filter traps with a volumetric flow rate of three liters per minute. The air was pulled from the top of the impinger and down through the filter materials. The traps were set up simultaneously with different filters to collect air samples continuously for about 2 hr each time. Samples were taken three times over a period of 3 weeks. The results show that all materials were able to retain a similar amount of SPM. However, the nitrocellulose filter was able to retain a higher amount of SPM, about 15% more than quartz and about 7% more than glass fiber and Teflon filters (p < 0.05). The concentrations of SPM ranged from 3.3 to 3.8 mg/m3. The retention of other ionic species such as nitrate, sulfate, and phosphate was observed to be similar as well for all filter materials (data not shown) with a slight higher retention for nitrocellulose (roughly 5% higher than the other materials, p < 0.05). This experiment was carried out twice. The slight higher retention capacity of nitrocellulose may be due to its ability to reduce particle bounce at impact. The manufacture also specifies retention efficiency in the range of up to 99.9% for nitrocellulose. Therefore, nitrocellulose filters were used in this experiment.
Poultry house management
Broiler chicks (30,000) were raised in a tunnel-ventilated poultry house (20 m × 145 m) on rice hull bedding materials. Ventilation fans were turned on automatically when the inside air temperature was deemed too high (above 22ºC) for the birds, usually during the afternoon on hot days. The broilers were raised continuously using the same bedding materials over four flocks before a total cleanout. From each flock, the birds were removed after they reached market size. Decaking of the poultry litters was carried out after each flock to make the litter more friable (loose and free of excessive moisture). The accumulation of cake in the poultry house can trap moisture in the litter, which will promote bacterial growth, pathogen development, and ammonia release. Each flock lasted approximately 60 days to get the birds to market size.
Results and discussion
Polyatomic ion distribution in fractionated particulate matter
Air samples were taken in three different broiler houses once a week (rotating over the eight houses) over a four-flock growout cycle. Total cleanout of broiler bedding materials occurred after the fourth flock. DPSS air sampling systems were utilized simultaneously to determine the distribution of different particulate matter (PM) fractions (2.5 µm, 10.0 µm, and total inhalable fractions) and their corresponding inorganic ion concentrations during these air sampling events. Concentrations or mass fractions of inorganic polyatomic ions such as ammonium, nitrate, phosphate, and sulfate in the PM were determined using ion chromatography. The results show, in general, increasing ion concentrations in PM as the number of flocks on the litter increased. The ammonium (NH4+) concentrations ranged from 17.6 ± 3.2 during flock 1 to 85.6 ± 10.3 mg/g of the total inhalable fraction (TIH) for flock 4 (). This is an increase of about fivefold from flock 1 to flock 4 (p < .05). Mass fractions of nitrate (NO3−), on the other hand, increased over 30-fold from flock 1 to flock 4. The nitrate concentrations ranged from 2.9 ± 1.6 to 29.8 ± 10.6 mg/g of TIH. Ammonia and volatile amines are the product of deamination and decarboxylation of amino acids from the feces. The products of deamination are volatile fatty acids (VFA), carbon dioxide, hydrogen gas, and ammonia. We saw the increase of VFA during the life cycle of a broiler flock from our previous study (Lovanh et al., Citation2012). Biological breakdown of uric acid in broiler litter could also contribute to the increase in ammonia concentrations in the air emissions. However, in particulate matter analyses, only ammonium ions were being detected. The gaseous ammonia concentrations could not be accounted for during this experiment. Therefore, a smaller amount of ammonium ions was observed in comparison to the higher concentration of nitrate. Nitrate, on the other hand, could increase and accumulate in PM over time due to the nitrification process (the oxidation of ammonia to form nitrate).
Table 1. Mass fractions (mg/g) of polyatomic ions in the inhalable fraction (TIH) of suspended particulate matter (SPM).
Phosphate (PO4−3) concentrations in the inhalable dust showed a smaller increase of about threefold over the four-flock cycle (). Phosphate concentrations ranged from 14.6 ± 8.1 for flock 1 to 43.2 ± 10.5 mg/g for flock 4. These phosphate concentrations in the PM are as expected since most of the phosphate would be produced in the gut and bound to the litter once excreted and would only get airborne when disturbed by the birds and other physical means. The scavenging of phosphate by other cations could also contribute to the small quantity found in air emissions. A similar increase in sulfate (SO4−2) concentrations was also observed. The sulfate concentrations ranged from 11.8 ± 6.1 during the first flock to 33.6 ± 15.2 mg/g during the fourth flock. Due to the conditions of the litter over time, sulfate concentrations could be decreased significantly due to dissimilative sulfate reduction (the biological transformation of sulfate to hydrogen sulfide). This process could contribute significantly to the reduction in sulfate concentrations in PM over various flocks.
The concentration profiles of polyatomic ions in PM10 show a trend similar to those of the inhalable fractions (). The ammonium concentrations increased about sixfold from 8.1 ± 5.2 during flock 1 to 49.0 ± 17.2 mg/g during flock 4. However, the ammonium mass fractions in PM10 are, in general, still smaller than those from the inhalable fractions ( vs. ). The mass fractions of nitrate in PM10 ranged from 7.0 ± 2.3 during flock 1 to 23.0 ± 7.5 mg/g during flock 4, an increase of just threefold. Phosphate concentrations in PM10 showed an increase of roughly fourfold from 8.9 ± 3.2 during first flock to 36.9 ± 15.5 mg/g during the fourth flock (). Sulfate concentrations in PM10 showed a similar increase from flock 1 to flock 4 (an increase of roughly fivefold) of 16.7 ± 8.1 to 80.2 ± 25.6 mg/g, respectively. These appear to be a little bit higher than those from the inhalable fractions (p < 0.05).
Table 2. Mass fractions (mg/g) of polyatomic ions in PM10 fraction of suspended particulate matter (SPM).
The mass fractions of inorganic ions in PM2.5 follow a trend similar to those of PM10. However, the incremental increase in ionic concentrations in PM2.5 from one flock to the next is smaller than those in the PM10 (). For example, the ammonium concentrations increased less than twofold from first flock (16.8 ± 6.9 mg/g) to the last flock (27.5 ± 10.6 mg/g) in PM2.5, whereas the ammonium concentrations in PM10 increased more than sixfold from the first flock to the last flock (). Nitrate concentrations in PM2.5 show similar trend and magnitude of increment from the first flock to the last flock with concentrations of 9.9 ± 8.2 and 13.0 ± 2.3 mg/g, respectively (). However, the increase in nitrate concentrations is not statistically significant (p > 0.05). Similarly, phosphate concentrations in PM2.5 show a small increment of less than threefold from the first flock to the last flock with concentrations of 11.3 ± 6.6 to 28.8 ± 8.3 mg/g, respectively. Sulfate appears to have the largest incremental increase in its concentrations from flock 1 to flock 4 of about fivefold (10.8 ± 4.4 to 54.5 ± 23.1 mg/g).
Table 3. Mass fractions (mg/g) of polyatomic ions in PM2.5 fraction of suspended particulate matter (SPM).
Based on these results, ion concentrations in fractionated PM fluctuate quite considerably from one flock to the next. The fluctuation could be caused by many contributing factors, such as biochemical, physiological, and environmental influences. Since this study involved the commercial production of broilers, only certain parameters could be controlled (e.g., amount, locations, time of sampling, etc.). In addition, the increase in particulate concentrations in the air may be due to the increase in excretion by the birds as they age and the ventilation rates. Therefore, to discern the effect of bird age versus the age of litter used on the concentrations of ions in PM, temporal profiles of ammonium distributions in fractionated particulate matter were carried out. shows that ammonium ion concentrations in the inhalable fraction of PM appear to be increasing with flock age for all four flocks. However, the increase in concentrations reached a maximum (week 4 of growth) and tapered off near the end of flock age. Similar trends were observed for all four flocks except that the magnitudes of ion concentrations are higher during successive flocks at a given flock age. Ammonium concentrations in PM2.5 and PM10 from flock 2 show trends similar to the inhalable fractions (). These observations (the tapering off of dust particles at the end of a flock) corroborated well with other studies (Saleh et al., Citation2005; Vucemilo et al., Citation2007). Thus, it could be inferred that aged litter (from successive flock) has a major effect on the increase of ion concentrations in fractionated PM with minor contribution from flock age, and the tapering off of dust particles at the end of a flock may be attributable to the increase in ventilation rates as the birds became larger.
Figure 2. Temporal trends of mass fractions of ammonium ions in the inhalable fractions of suspended particulate matter. The error bars were obtained from a pool of at least three samples spanning over the week of sampling. Different letterings within the same cluster (age of flock) signifies statistically different at p < 0.05.
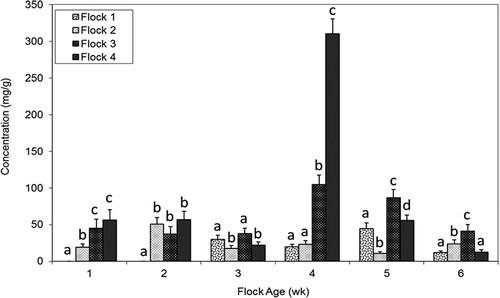
Figure 3. Comparison of average ammonium ions in fractionated particulate matter concentrations (n = 3) inside a broiler house during second flock of growth. PM2.5 = particulate matter of aerodynamic diameter of 2.5 µm or less; PM10 = particulate matter of aerodynamic diameter of 10.0 µm or less. Different letterings within the same cluster (flock age) signifies statistically different at p < 0.05.
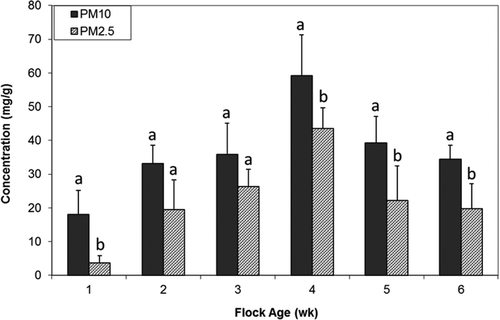
These findings show that ions in particulate matter appeared to be highly nonuniformly distributed. This is as expected since particulate matter formation is dependent on several environmental factors such as the type and location of PM sources (aged bedding materials increase potential for microbial activities to generate these ionic pollutants), environmental conditions, levels and distribution of animal activities (related to flock age). Several studies (Liao and Feddes, Citation1991; Maghirang and Manbeck, Citation1993; Walker et al., Citation2006) have shown that particulates in air are affected by a variety of forces such as aerodynamic, gravity, and buoyancy, and undergo turbulent coagulation, turbulent diffusive deposition, and gravitational sedimentation. In a high PM concentration environment such as poultry housing, particulates with small aerodynamic diameters may be affected by coagulation from PM with greater mass due to van der Waals forces (Tsai and Cheng, Citation1995). For this study, though, in general, higher concentrations of polyatomic ions are found in the larger PM sizes, as the flock aged, and during successive flocks (– and and ) except for sulfate. Higher sulfate mass fractions were found mostly in PM10 and PM2.5 ( and ). Our results show trends similar to those found by Walker et al. (Citation2006), that sulfate is highly concentrated in inorganic aerosol PM2.5 in the presence of excess ammonia and higher atmospheric temperature. These environmental conditions such as high ammonia concentrations and house temperature using photoacoustic gas analyzer were observed during our study (data not shown). Thus, from a combination of flock age and the reuse of old litters successively in the poultry house from one flock to the next, it appears that the increase in ionic concentrations in PM is due to biochemical and physiological factors of the aerosol sources, as well as to housing conditions (e.g., litter compaction over time, litter moisture, etc.). Although this study did not examine the correlations between microbial activities and particle ionic content in aerosols from poultry houses, several studies have shown the availability and proliferation of these various microorganisms that could produce these air pollutants in poultry litters. The aged litter and its conditions are major factors because the population of microorganisms responsible for airborne dust and its ionic content varies depending on the dynamics of litter exchange (Hartung, Citation1994; Seedorf et al., Citation1998; Baykov and Stoyanov, Citation1999; Radon et al., Citation2002; Thaxton et al., Citation2003; Bakutis et al., Citation2004; Dawkins et al., Citation2004; Lovanh et al., Citation2007).
Conclusions
An experiment was carried out to examine the effect of aged bedding materials on polyatomic ions distribution in fractionated particulate matter inside broiler houses using rice hulls bedding materials. Particle trap samplers with different venturi orifices (TSP, 10 µm, and 2.5 µm) were used to collect the suspended particulate matter over four successive flocks (one cycle of total cleanout). Suspended particulate matter was collected and analyzed for their ionic content. Based on these results, concentrations of polyatomic ions in suspended particulate matter were found to increase with successive flocks and highly concentrated in the larger size PM. In addition, the ions concentrations appeared to reach a maximum at the middle of flock age (around the fourth week), tapering off toward the end in a given flock (may be due to the increase in ventilation rates). Thus, it can be inferred that the age of bedding materials affects the ionic concentrations in aerosol particulate matter more than the age of the birds. Since different types of poultry operation require different management styles, understanding the effect of management practices (e.g., reusing of old bedding materials for successive flocks) from these different types of operation on the indoor and, consequently, the outdoor air emissions will lead to innovative best management practices for poultry operation to safeguard the health and welfare of the animals and of the poultry operators, along with reducing the impact of potential air pollution on the environment.
Acknowledgments
The authors thank Mike Bryant for his technical assistance. This research was part of USDA-ARS National Program 214: Agricultural and industrial byproducts. Mention of a trademark or product anywhere in this paper is to describe experimental procedures and does not constitute a guarantee or warranty of the product by the USDA and does not imply its approval to the exclusion of other products or vendors that may also be suitable.
Additional information
Notes on contributors
Nanh Lovanh
Nanh Lovanh, John Loughrin, and Phil Silva are scientists at the USDA-ARS in Bowling Green, KY.
References
- Baekbo, P. 1989. Air quality and pig herd health. Copenhagen, Denmark: Institute for Internal Medicine, The Royal Veterinary and Agricultural University.
- Bakutis, B., E. Monstviliene, and G. Januskeviciene. 2004. Analyses of airborne contamination with bacteria, endotoxins and dust in livestock barns and poultry houses. Acta Veterinaria Brno 73:283–89. doi:10.2754/avb200473020283
- Baykov, B., and M. Stoyanov. 1999. Microbial air pollution caused by intensive broiler chicken breeding. Microbial Ecol. 29:389–93. doi:10.1111/fem.1999.29.issue-4
- Charlson, R.J., S.E.Schwartz, J.M. Hales, R.D. Cess, J.A. Coakley, Jr., J.E. Hansen, and D.J. Hofmann. 1992. Climate forcing by anthropogenic aerosols. Science 255:423. doi:10.1126/science.255.5043.423
- Dawkins, M.S., C.A. Donnelly, and T.A. Jones. 2004. Chicken welfare is influenced more by housing conditions than by stocking density. Nature 427:342–344.
- Donham, K.J., D.C. Zavala, and J.A. Merchant. 1984. Acute effects of the work environment on pulmonary functions of swine confinement workers. Am. J. Ind. Med. 5:367–75. doi:10.1002/(ISSN)1097-0274
- Dosman, J.A., B.L. Gragam, D. Hall, P. Van Loop, P. Bhasin, and F. Froh. 1987. Respiratory symptoms and pulmonary function in farmers. J. Occup. Med. 29:39–43.
- Hartung, J. 1985. Gas chromatographic analysis of volatile fatty acids and phenolic/indolic compounds in pig house dust after ethanolic extraction. Environ. Technol. Lett. 6:21–30. doi:10.1080/09593338509384315
- Hartung, J. 1994. The effect of airborne particulates on livestock health and production. In Pollution in Livestock Systems, ed. I. Ap Dewi, R.F.E. Axford, I. Mara, H. Omed, 55–69. Wallingford, UK: CAB International.
- Hayter, R.B., and E.L. Besch. 1974. Airborne particle deposition in the respiratory tract of chickens. Poult. Sci. 53:1507–11. doi:10.3382/ps.0531507
- Hoek, G., B. Brunekreef, S. Goldbohm, P. Fischer, and P.A. van den Brandt. 2002. Association between mortality and indicators of traffic-related air pollution in the Netherlands: A cohort study. Lancet 360:1203–9. doi:10.1016/S0140-6736(02)11280-3
- Homes, M.J., A.J. Heber, C.C. Wu, L.K. Clark, R.H. Grant, N.J. Zimmerman, M.A. Hill, M.W. Strobel, M.W. Peugh, and D.D. Hones. 1996. Viability of bioaerosols produced from a swine facility. Proceedings of International Conference on Air Pollution from Agricultural Operations, Kansas City, MO, 127–31.
- Intergovernmental Panel on Climate Change (IPCC)/WMO/UNEP. 2007. Climate Change 2007: Impacts, Adaptation, and Mitigation of Climate Change: Scientific-Technical Analyses. Prepared by IPCC Working Group III. Cambridge, UK: Cambridge University Press.
- Iversen, M., R. Dahl, J. Korsgaard, T. Hallas, and E.J. Jensen. 1988. Respiratory symptoms in Danish farmers: An epidemiological study of risk factors. Thorax 43:872–77. doi:10.1136/thx.43.11.872
- Iversen, M. and B. Pedersen. 1990. The relationship between respiratory symptoms, type of farming and lung function disorders in farmers. Thorax 45:919–23. doi:10.1136/thx.45.12.919
- Liao, C.M., and J.J.R. Feddes. 1991. Modelling and analysis of airborne dust removal from a ventilated airspace. Can. Agric. Eng. 33(2): 355–61.
- Lovanh, N., K.L. Cook, M.J. Rothrock, D.M. Miles, and K. Sistani. 2007. Spatial shifts in microbial population structure within poultry litter associated with physicochemical properties. Poult. Sci. 86:1840–49. doi:10.1093/ps/86.9.1840
- Lovanh, N., J. Loughrin, and K. Sistani. 2012. Volatile fatty acids in suspended particulate matter from a poultry house using rice hulls as bedding materials—A profile of first flock after total cleanout. J. Civil Environ. Eng. 2:114. doi:10.4172/2165-784X.1000114
- Maghirang, R.G., and H.B. Manbeck. 1993. Modelling particle transport in slot-inlet ventilated airspaces. Trans. ASAE 36(5): 1449–59. doi:10.13031/2013.28485
- National Academy of Sciences. 2003. Air Emissions from Animal Feeding Operations: Current Knowledge, Future Needs. Washington, DC: National Academies Press.
- Pope, C.A., R.T. Burnett, M.J. Thun, E.E. Calle, D. Krewski, K. Ito, and G.D. Thurston. 2002. Lung cancer, cardio-pulmonary mortality, and long-term exposure to fine particulate air pollution. J. Am. Med. Assoc. 287:1132–41.
- Radon, K., B. Danuser, M. Iversen, E. Monso, C. Weber, J. Hartung, K.J. Donham, U. Palmgren, and D. Nowak. 2002. Air contaminants in different European farming environments. Ann. Agric. Environ. Med. 9:41–48.
- Saleh, M., J. Seedorf, and J. Hartung. 2005. Influence of animal age and season on bioaerosol concentrations in a broiler house. Proceedings of the 12th International Congress on Animal Hygiene, Warsaw, Poland, 2:37–40.
- Seedorf, J., J. Hartung, M. Schroder, K.H. Linkert, V.R. Phillips, M.R. Holden, R.W. Sneath, J.L. Short, R.P. White, and S. Pedersen. 1998. Concentrations and emissions of airborne endotoxins and microorganisms in livestock buildings in Northern Europe. J. Agric. Eng. Res. 70:97–109. doi:10.1006/jaer.1997.0281
- Selman, M. 2007. Eutrophication: An Overview of Status, Trends, Policies, and Strategies. Washington, DC: World Resources Institute.
- Thaxton, Y.V., C.L. Balzli, and J.D. Tankson. 2003. Relationship of broiler flock numbers to litter microflora. J. Appl. Poult. Res. 12:81–84.
- Tsai, C.J., and Y.H. Cheng. 1995. Solid particle collection characteristics on impaction surfaces of different designs. Aerosol Sci. Technol. 23:96–106. doi:10.1080/02786829508965297
- Vucemilo, M., K. Matkovic, B. Vinkovic, S. Jaksic, K. Granic, and N. Mas. 2007. The effect of animal age on air pollutant concentration in a broiler house. Czech J. Anim. Sci. 52(6):170–174.
- Walker, J.T., W.P. Robarge, A. Shendrikar, and H. Kimball. 2006. Inorganic PM2.5 at a U.S. agricultural site. Environ. Pollut. 139:258–271. doi:10.1016/j.envpol.2005.05.019
- Whyte, R.T., and P.A.M. Williamson. 1993. Aerial pollutant burdens and respiratory impairment of poultry house stockmen. Proceedings of International Livestock Environment Symposium IV, Warwick, England, 709–17.
- Williams, A.G. 1989. Dust and odour relationships in broiler house air. J. Agric. Eng. Res. 44:175–90. doi:10.1016/S0021-8634(89)80080-0
- World Health Organization. 2003. Health aspects of air pollution with particulate matter, ozone and nitrogen dioxide. Bonn, Germany: World Health Organization.
- Zanobetti, A., J. Schwartz, E. Samoli, A. Gryparis, G. Touloumi, R. Atkinson, A. Le Tertre, J. Bobros, M. Celko, A. Goren, B. Forsberg, P. Michelozzi, D. Rabczenko, E. Aranguez Ruiz, and K. Katsouyanni. 2002. The temporal pattern of mortality responses to air pollution: A multicity assessment of mortality displacement. Epidemiology 13(1): 87–93. doi:10.1097/00001648-200201000-00014