ABSTRACT
Research has been conducted to investigate the effects of daily aeration frequency on leachate quality and waste settlement in simulated hybrid landfill bioreactors. Four laboratory-scale reactors were constructed and operated for about 10 months to simulate different bioreactor operations, including one anaerobic bioreactor and three hybrid bioreactors with different aeration frequencies (one, two, and four times per day). Chemical oxygen demand (COD) and biochemical oxygen demand (BOD5) reduced more than 96% of the initial concentrations in all aerated bioreactors. The differences of COD and BOD5 reductions among tested aeration frequencies were relatively small. For ammonia nitrogen, the higher aeration frequency (two or four times per day) resulted in the quicker reduction. Overall, the concentrations of heavy metals (Cr, Co, Cu, Mn, Ni, and Zn) decreased over time except Cd and Pb. The reduction of redox-sensitive metal concentrations (Mn, Co, Ni, and Cu) was greater in aerated bioreactors than in anaerobic bioreactor. Settlement of municipal solid waste (MSW) was enhanced with higher frequency of aeration events (four times per day).
Implications: In recent years, hybird bioreactor landfill technology has gained a lot of attention. Appropriate aeration rate is crucial for hybrid bioreactor operation, but few studies have been done and different results were obtained. Research was conducted to investigate the effects of daily aeration frequency on leachate quality and waste settlement. Results indicated that aeration can effectively accelerate waste stabilization and remove organic carbon concentration and total nitrogen in the leachate.
Introduction
In China, the amount of municipal solid waste (MSW) collected by authorities reached 178.6 million tons in 2014, and about 65% of the collected MSW was landfilled (National Bureau of Statistics of China, Citation2015) . As a cost-effective MSW management option, landfilling will remain as a dominant waste disposal option in China for the next few decades (Chen et al., Citation2010; Zhang et al., Citation2010). Food waste is a major component of typical Chinese MSW, accounting for more than 50% of MSW by weight. Due to the high moisture content of the food waste (about 50~80% by weight), many MSW landfills in China, especially in the areas with high annual rainfall, are facing serious challenges of leachate management (Jiang et al., Citation2010; Zhang et al., Citation2013).
To enhance MSW biodegradation and leachate treatment in landfills, bioreactor landfill technologies have been studied with different modes: anaerobic, aerobic, and hybrid types (Benson et al., Citation2007; Cossu et al., Citation2015; Erses et al., Citation2008; Hirata et al., Citation2012; Long et al., Citation2009b; Slezak et al., Citation2015; Xu et al., Citation2014). Among those technologies, the anaerobic bioreactor is the most commonly used. However, an anaerobic bioreactor does not provide a biological pathway for ammonia-nitrogen removal, resulting in elevated ammonia-nitrogen concentrations in leachate, especially for the landfills containing a high fraction of food waste.
Research has been conducted to investigate waste decomposition and leachate quality using aerobic bioreactors (Bilgili et al., Citation2006; Heyer et al., Citation2005; Ko et al., Citation2013; Ritzkowski et al., Citation2006; Ritzkowski and Stegmann, Citation2010; Slezak et al., Citation2015; Xu et al., Citation2015; Yazdani et al., Citation2010). The advantages of aerobic systems over anaerobic systems include rapidly improving leachate quality, such as ammonia-nitrogen and chemical oxygen demand (COD) removal, and reducing methane emissions (Mertoglu et al., Citation2006; Ritzkowski and Stegmann, Citation2010; Shao et al., Citation2008; Tong et al., Citation2015). The use of discontinuous aerobic and anaerobic conditions in landfills has been studied and suggested as an appropriate bioreactor option for wet Chinese MSW (He et al., Citation2012; Long et al., Citation2009a, Citation2009b). Such hybrid bioreactors can be operated by on-off aeration cycles (intermittent aeration) and physically separate the waste in aerobic and anaerobic conditions. The primary goal of these hybrid bioreactors was to remove nitrogen from leachate using biological nitrification-denitrification processes.
In order to promote biological ammonia-nitrogen removal in landfills, appropriate aeration rates should be determined. Although a few studies have been done on bioreactor aeration rates, different results were obtained (Prantl et al., Citation2006; Slezak et al., Citation2010). Slezak et al. (Citation2010) showed there were no significant differences among leachate qualities of bioreactors aerated with the flow rate range (continuous aeration) of 2–10 L/hr (0.003–0.017 L/min-kg). However, Prantl et al. (Citation2006) concluded that leachate quality of simulated landfills with higher aeration rate (1.4 L/min-kgDM [liters per minute per kilogram dry matter]) was slightly better than those with low aeration rate (0.0007 L/min-kgDM). The ranges of aeration rates reported in literature show an order of magnitude difference. Indeed, it is very difficult to determine an optimum aeration rate for aerobic operation of a MSW landfill on a quantitative basis. That is because the effect of MSW aeration depends on not only the quantity of aeration but also other factors, including MSW composition and conditions (organic content, moisture level, MSW age, temperature, etc.), the conditions of air (temperature and humidity), moisture distribution system (leachate injection), and air distribution and off-gas collection systems (air injection technologies and off-gas collection systems). Aeration-frequency-based approaches may be useful with respect to operating hybrid bioreactor landfills. However, there is insufficient information available on the impact of aeration frequency on the performance of a hybrid bioreactor landfill.
In addition, the aeration can affect the mobility of heavy metals in MSW landfills (Gadd, Citation2010; Kim et al., Citation2011; Qu et al., Citation2008; Yao et al., Citation2014). Giannis et al. (Citation2008) reported that heavy metal concentrations (Ni, Cd, Pb, Zn, and As) in leachate of simulated aerobic landfills greatly decreased. In contrast, Shao et al. (2009) presented that autotrophic denitrification could increase the mobility of Zn, Cu, and Pb by transferring to the more mobile bound-to-carbonate and reducible phases. Other research reported that the change of oxidation state by aerobic conditions could result in some metals be more mobile than by anaerobic conditions (Flyhammar and Håkansson, Citation1999; Mårtensson et al., Citation1999). Because of various pathways of the heavy metal migration in aerobic and anaerobic conditions, the mobility of metals caused by the insufficient and/or temporary aeration would be complicated.
The objective of this study was to evaluate the performance of hybrid bioreactor landfills with different aeration frequencies on typical Chinese MSW. In this study, simulated bioreactors with sectioned waste layers were constructed: the upper layer is preferable for aerobic conditions, whereas the bottom layer is preferable for anaerobic conditions. The leachate quality (organic carbon, ammonia nitrogen, and heavy metals) and MSW settlement with different aeration conditions were compared. The results provide insight into the effects of aeration frequency on waste degradation and leachate quality, which might be useful for landfill operation in hybrid bioreactors.
Materials and methods
Materials
Waste components were collected from Shenzhen University Town (Shenzhen, China) and synthesized to represent the typical MSW composition in Shenzhen, China. These components included food waste, paper, plastics, glass, metal, and inert material. Collected waste components were screened and shredded to reduce particle size to less than 5 cm. The initial moisture content of the synthesized waste was 49.7%, and the total volatile solid of biodegradable components in each bioreactor was about 3.4 kg. Moisture content, volatile solid (VS), and total solid (TS) of each component are listed in .
Table 1. Composition of MSW in simulated bioreactors.
Table2. Summary of operational conditions of hybrid bioreactors.
Experimental design
Four laboratory-scale columns were built to simulate different bioreactor operations, including one anaerobic bioreactor as control (C1) and three aerobic bioreactors (A1, A2, A3) with different aeration frequencies. shows the schematic diagram of a laboratory hybrid bioreactor. The bioreactor was constructed using 20-cm-diameter polyacrylic plastic pipe with a total height of 100 cm. A gravel layer, 5 cm thick, was placed at the bottom of each column as a drainage layer. Each bioreactor was initially packed with a total of 13.2 kg synthesized MSW. The bulk density of the compacted waste was about 600 kg/m3. A layer of gravel was placed on the top of the loaded waste to facilitate the even distribution of recirculated leachate. A 10-cm-thick gravel layer was placed at the middle of the waste layer for aeration. A 0.5-cm-diameter polyacrylic plastic tube was located at the center of the upper waste layer to carry air into the aeration layer. A leachate injection port and a gas collection port were installed on the top of each column. Another port was located at the bottom of each column for leachate collection.
Figure 1. Schematic of simulated bioreactor landfills: (left) anaerobic reactor (C1) and (right) aerated reactors (A1, A2, and A3).
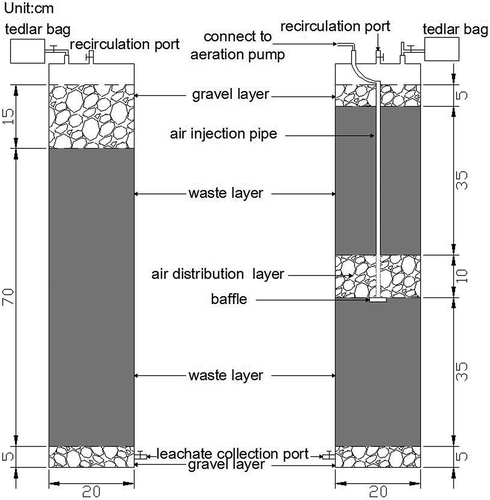
All simulated bioreactors were operated for approximately 10 months in a thermostatically controlled room (30 ± 5 °C). Leachate collected from each column was recirculated with a flow rate of 500 mL/day. For each aeration event, air was supplied using an air pump with a flow rate of 30 L/hr for 2 hr. The frequency of aeration events for A1, A2, and A3 were one, two, and four times per day, respectively ().
Sample collection and analysis
Leachate was collected from the leachate collection port on a weekly basis, and leachate samples were analyzed for different parameters, including pH (Sartorius PB-10; Beijing, China), chemical oxygen demand (COD), biochemical oxygen demand (BOD5), and nitrogen-containing compounds (ammonia nitrogen [NH3-N], nitrate nitrogen [NO3-N], and nitrite nitrogen [NO2-N]). Leachate COD was measured by the fast digestion–spectrophotometric method (HJ/T 399-2007; EPA of China, Citation2007). BOD5 was measured using a dilution and seeding method (HJ 505-2009; EPA of China, Citation2009b), and NH3-N was analyzed by Nessler’s reagent colorimetric method (HJ 535-2009; EPA of China, Citation2009a). Blanks, replicates, and calibration check samples were performed as appropriate.
Heavy metals were measured by inductively coupled plasma mass spectrometry (ICP-MS; Thermo Fisher, X series II) after microwave digestion pretreatment. The microwave digestion process was determined based on the U.S. Environmental Protection Agency (EPA) standard method 3015a (EPA, Citation2007). Gas composition (O2, CH4, CO2) was monitored by a gas chromatograph (Fuli GC9790; Hangzhou, China) equipped with a thermal conductivity detector (TCD). CH4 and CO2 were separated by Porapak Q column (3 m × 3 mm), and O2 was analyzed by 5Å molecular sieve column. The operational temperatures of injector, column, and TCD were 50, 70, and 100 °C, respectively, with an applied current of 110 mA.
Results and discussion
Gas composition and oxygen utilization rate
presents the change of O2 and CO2 of aerated reactors at the initial aeration phase (measured at day 5). During the aeration period, O2 content in off-gases was about 17%. After the aeration stopped, O2 content in each column dropped to 2% within a few hours and CO2 content inversely increased with the consumption of O2. Before the next aeration started, CO2 contents increased to approximately 25%, 17%, and 15% in A1, A2, and A3, respectively. In comparison, the reactor C1 was operated under anaerobic conditions without any aeration, CO2 concentration was as high as 74.8% due to the decomposition of easy biodegradable materials and no methane was detected in day 5.
It was noticed that although O2 content decreased after ceasing aeration in all aerated columns, the decreasing O2 rates were different. Oxygen utilization rates (OURs) were determined using the change of O2 content over time, which indicated the extent of aerobic decomposition of MSW. All aerated reactors had similar OUR values at the beginning of the experiment, ranging from 4.55%/hr to 4.95%/hr. At day 45, it was observed that the OURs of A2 and A3 decreased more than half of those at day 5, to 2.25%/hr and 1.9%/hr, respectively. However, OUR of A1 at day 45 was similar to that of day 5. The decrease of OUR in A2 and A3 indicated that readily decomposable organic waste matter could be decomposed more in those reactors (A2 and A3) with the higher aeration frequencies.
With continuing the insufficient aeration, it was observed that methane content started increasing when the aeration ceased, due to the formation of aerobic and anaerobic zones in the columns. Methane was detected up to 15.6%, 3.0%, and 0.3% in A1, A2, and A3, respectively, when air addition stopped (at day 45). With the start of next aeration cycle, methane concentration quickly decreased (). However, the methane concentration in the anaerobic reactor C1 remained in the range of 3–6%, which was mainly attributed to the acid condition in leachate.
pH, COD, BOD5, and BOD5/COD in leachate
presents the change of COD, BOD5, pH, and BOD5/COD ratio in leachate of each bioreactor. In the early stage of the experiment, pH values in leachate of all bioreactors dropped rapidly to about 5.6, indicating that organic acids were accumulated. It was noticed that pH of leachate in the aerobic columns rapidly increased to over 7.0 within 10 weeks, whereas pH of C1 increased slowly and reached about 6.3 at day 300. Similarly, Xu et al. (Citation2015) reported that aeration increased pH and decreased Volatile Fatty Acids (VFA) concentrations in leachate. The results indicate that intermittent aeration in hybrid bioreactor can shorten the acid phase of waste degradation, which provides better condition for methane generation. As shown in , A2 and A3 reached the first peak of pH (A2 = 7.75 and A3 = 7.55) around day 110; pH of A1 slowly increased to pH 8.2 until day 250 and then decreased. The decreasing of pH corresponded to the decreasing of NH3-N concentration in leachate. Under aerobic conditions, NH3-N can be converted into NO3-N and release H+, as shown in eq 1.
On the other hand, the NH4+ in leachate can be air stripped during the aeration process, results in pH decreasing, as shown in eq 2.
Therefore, aeration was attributed to the decreasing of pH in leachate. Due to the high aeration frequency in A2 and A3, the pH of A2 and A3 were lower than that of A1 after day 110.
The initial COD and BOD5 in the reactors were >60,000 and 50,000 mg/L, respectively. The COD and BOD5 of leachate dropped quickly in all aerated reactors within 100 days. The 99% of BOD5 removal was achieved in A1, A2, and A3 at 284, 216, and 160 days, respectively. The BOD5/COD ratios of A1, A2, and A3 at the completion of the experiment reached 0.15, 0.12, and 0.09, respectively. However, in the anaerobic reactor, COD and BOD5 did not change much compared with the initial concentrations, and the BOD5/COD ratio fluctuated around 0.7 during the experiment.
NH3-N, NO3-N, and NO2-N in leachate
The variations of NH3-N, NO3-N, and NO2-N concentrations in leachate are shown in . NH3-N increased in all reactors during the initial stage of the experiment. The maximum NH3-N concentrations of A1, A2, and A3 were 2800, 2300, and 2500 mg/L, respectively. In addition, the time to reach the maximum concentration varied in the aerated bioreactors and NH3-N in A1, A2, and A3 started to decrease around days 250, 100, and 50, respectively. The decreasing of NH3-N can be attributed in part to biological nitrification. It was reported that heterotrophic microorganisms could outcompete nitrifiers for oxygen and nutrients under high organic carbon concentrations (Berge et al., Citation2005, Citation2006). However, the slow start of nitrogen reduction in A1 could be caused by the insufficient amount of air, since BOD5 removal of A1 was comparable to those of A2 and A3. The times required for 95% NH3-N removal in A2 and A3 were 190 and 142 days, respectively. However, until the end of the experiment, NH3-N removal in A1 had not achieved 95% yet. The results imply that not only decreasing organic carbon concentrations but also providing sufficient air should be necessary to enhance the nitrification process.
In contrast to NH3-N concentration, the NO3-N concentration increased with increasing the aeration rate. During the experiment, NO3-N concentration in the anaerobic reactor was maintained at around 12 mg/L. The NO3-N concentrations of A1, A2, and A3 increased to around 25, 30, and 40 mg/L, respectively. Comparing NO3-N with NH3-N of the aerated reactors, it was evident that the magnitude of nitrification enhanced with increasing aeration frequency. The concentrations of NO2-N in leachate of all bioreactors were minimal over the period of time.
It was noticed that the decreasing of NH3-N concentrations in the aerated bioreactors corresponded to pH drops in leachate. For example, of NH3-N concentration in A1 started to decrease from the peak of 2800 mg/L around day 250, which corresponded with the decreasing of leachate pH. Similar trends were also observed in A2 and A3 (). The change of pH may be explained by the oxidation of NH4+ to NO3−, resulting in the destruction of alkalinity (Giannis et al., Citation2008). Due to the high aeration frequency, a stable NH3-N concentration (around 50 mg/L) was achieved around 200 and 160 days in A2 and A3, respectively. However, the final NH3-N concentration was 1000 mg/L in A1. As shown in , levels of NO3-N and NO2-N were relatively small comparing with the reduction of NH3-N in the same period. It suggests that NO3-N and NO2-N were decomposed by denitrification process.
Heavy metals
The concentrations of selected heavy metals (Cr, Co, Cd, Cu, Mn, Ni, Pb, and Zn) in leachate over time and with pH are presented in . The heavy metal concentrations were in the order of Zn > Mn >> Ni > Cr, Co, Cu > Pb, Cd. The concentrations of heavy metals in all reactors decreased rapidly within 50 days except Cd and Pb. Among the measured heavy metals, the levels of Co, Cu, Mn, and Ni of the aerated bioreactors were lower than those of anaerobic bioreactor. It is known that microbial reduction of metal oxides, such as Fe and Mn, plays an important role in mobilizing metals absorbed on the metal oxides (Gadd, Citation2010). The different levels of Co and Ni between C1 and aerated reactors (A1, A2, and A3) indicated that Co and Ni could be released through the reductive dissolution of Mn (and/or Fe) in C1. also shows the heavy metal concentrations as a function of pH. The lowest concentrations of many heavy metals were measured in the range of pH 7–8. Mn, Cu, and Ni showed a tendency of decrease at the measured pH range. Due to a relative narrow pH range (from pH 5.6 to pH 8.1), amphoteric behavior of metal concentrations was not clearly observed in this study. However, in general, the concentrations of heavy metals decreased over time. The concentration reduction may result from the pH increasing in the leachate, especially in those aerobic bioreactors. On the other hand, other mechanisms, such as precipitation, sorption, and redox conditions, may also be involved in the process.
Landfill settlement
Landfill settlement is an important indicator of MSW stabilization. shows the settlement (%) of each bioreactor. The primary settlement was about 5% for the first 10 days in all bioreactors, which mainly was caused by the self-weight of solid waste and compressive forces from the above layers. Settlement of C1, A1, A2, and A3 at the end of the experiment were 7.1%, 12.9%, 20.0%, and 25.7%, respectively. The degree of settlement in A3 was almost double that in A1, indicating that the settlement of waste was enhanced by using higher aeration frequency.
It was noticed that the settlement degree of A3 was similar to the degree of organic carbon reduction (e.g., BOD5). For example, about 56% and 94% of the total settlement of A3 coincidently occurred at days 21 and 138; similarly, about 48% and 97% reduction of the initial BOD5 observed at days 23 and 133, respectively. However, for A1 and A2, the settlement rates did not decrease rapidly even though their BOD5 reduction rates were similar to that of A3 at the end of the experiment. The results indicated that the degree of settlement in aerated waste might rely not only on the amount of decomposed organics, but also on the rate of degradation (the faster decomposition might cause sudden collapses of waste structure). Moisture content variation with different amount of air addition could be another reason of the different settlement rate. The greater amount of moisture could be removed with the greater aeration rate. Even though leachate was recirculated regularly, higher aeration rate could result in drying of some portions of MSW, which could cause drying shrinkage.
Impact of aeration frequency
shows BOD5/COD, NH3-N concentrations, and settlement measured in A1, A2, and A3 as a function of number of aeration events. As shown in , it took about 240, 460, and 660 aeration events for A1, A2, and A3, respectively, to reach BOD5/COD ratio of 0.2. This result indicates that the biological stabilization per unit volume of air was more efficient in A1 (low frequency of aeration) than in A3 (high frequency). In terms of NH3-N removal, A1 was almost catching up A2 at the end of the experiment. The nitrogen removal efficiency per unit volume of air of A1 was similar to A2 but greater than A3. Regarding settlement, the greater settlement was observed with the greater frequency of aeration events per day. For example, with 200 aeration events, A3 achieved 18.6% of settlement but only 8.6% in A1 (). With regard to determining aeration frequency, air emission during the aeration should be considered because the increase of aeration events results in increasing the volume of off-gas and affects on the quality of off-gas. The emission during aeration is not in the scope of this study but can be found elsewhere (He et al., Citation2012; Nag et al., Citation2016; Tong et al., Citation2015).
Summary and conclusion
In this study, the performance of aerated bioreactors with different daily aeration frequency was compared with that of an anaerobic reactor. The anaerobic landfill remained in the acid phase over the period of the experiment and resulted in high concentrations of organic carbons and nitrogen in the leachate. In all the aerated bioreactors, organic carbon concentrations (COD and BOD5) reduced by greater than 96% of the initial concentration. Concentrations of NH3-N with frequent aeration (A2 and A3) were reduced quicker than that with a less frequent aeration (A1). For the selected heavy metals (Cr, Co, Cd, Ni, Cu, Pb, Mn, Zn), concentrations generally decreased over time in all bioreactors. Co, Mn, and Ni concentrations in the aerated bioreactor were lower than those in the anaerobic bioreactors. The degree of waste settlement increased with increasing the aeration rate, indicating aeration can effectively accelerate waste stabilization. Considering the cost of aerating MSW landfill, it was suggested to use low daily aeration frequency if the landfill operator would target to remove organic carbon concentration and total nitrogen in the leachate. However, high daily aeration events can provide relatively large space gain and fast removal of nitrogen. It is difficult to quantitatively optimize aeration rates for maximizing all the performance of hybrid bioreactors. So, aeration-frequency-based approaches may be more practical in terms of operating hybrid bioreactor landfills. The various results of hybrid bioreactor performance with different aeration frequency imply that aeration frequency should be adjusted by responding to performance parameters observed during the aeration. Also, a performance-specific aeration approach is proposed: high aeration frequency for targeting fast nitrogen removal and low frequency for carbon removal.
Funding
This research was supported by the Shenzhen government of China with grant nos. JCYJ20150616145013931, CXZZ20151117141320317, and JCYJ20150806112326712.
Additional information
Funding
Notes on contributors
Jae Hac Ko
Jae Hac Ko is an assistant professor at the Peking University Shenzhen Graduate School.
Zeyu Ma
Zeyu Ma is a graduate student at the Peking University Shenzhen Graduate School.
Xiao Jin
Xiao Jin is a graduate student at the Peking University Shenzhen Graduate School.
Qiyong Xu
Qiyong Xu is an associate professor at the Peking University Shenzhen Graduate School.
References
- American Public Health Association (APHA). 1997. Standard methods 2540 B. Total solids.
- American Society for Testing and Materials (ASTM). 2010. 2010 ASTM D2216–10. Standard test methods for laboratory determination of water (moisture) content of soil and rock by mass.
- Benson, C.H., M.A. Barlaz, D.T. Lane, and J.M. Rawe. 2007. Practice review of five bioreactor/recirculation landfills. Waste Manage. 27:13–29. doi:10.1016/j.wasman.2006.04.005
- Berge, N.D., D.R. Reinhart, J. Dietz, and T. Townsend. 2006. In situ ammonia removal in bioreactor landfill leachate. Waste Manage. 26:334–43. doi:10.1016/j.wasman.2005.11.003
- Berge, N.D., D.R. Reinhart, and T.G. Townsend. 2005. The fate of nitrogen in bioreactor landfills. Crit. Rev. Env. Sci. Technol. 35:365–99. doi:10.1080/10643380590945003
- Bilgili, M.S., A. Demir, and B. Ozkaya. 2006. Quality and quantity of leachate in aerobic pilot-scale landfills. Environ. Manage. 38:189–96. doi:10.1007/s00267-005-0179-1
- Chen, X., Y. Geng, and T. Fujita. 2010. An overview of municipal solid waste management in China. Waste Manage. 30:716–24. doi:10.1016/j.wasman.2009.10.011
- Cossu, R., L. Morello, R. Raga, and G. Cerminara. 2015. Biogas production enhancement using semi-aerobic pre-aeration in a hybrid bioreactor landfill. Waste Manage. 55 (September): 83–92. doi:10.1016/j.wasman.2015.10.025
- EPA of China. 2007. Water quality - Determination of the chemical oxygen demand-Fast digistion-spectrophotometric method. HJ/T 399-2007.
- EPA of China. 2009a. Water quality - Determination of ammonia nitrogen-Nessler’s reagent spectrophotometry. HJ 535-2009.
- EPA of China. 2009b. Water quality - Determination of biochemical oxygen demand after 5 days (BOD5) for dilution and seeding method. HJ 505-2009.
- Erses, A.S., T.T. Onay, and O. Yenigun. 2008. Comparison of aerobic and anaerobic degradation of municipal solid waste in bioreactor landfills. Bioresour. Technol. 99:5418–26. doi:10.1016/j.biortech.2007.11.008
- Flyhammar, P., and K. Håkansson. 1999. The release of heavy metals in stabilised MSW by oxidation. Sci. Total. Enviorn. 243–244:291–303. doi:10.1016/S0048-9697(99)00405-2
- G.M. Gadd. 2010. Metals, minerals and microbes: Geomicrobiology and bioremediation. Microbiology 156:609–43. doi:10.1099/mic.0.037143-0
- Giannis, A., G. Makripodis, F. Simantiraki, M. Somara, and E. Gidarakos. 2008. Monitoring operational and leachate characteristics of an aerobic simulated landfill bioreactor. Waste Manage. 28:1346–54. doi:10.1016/j.wasman.2007.06.024
- He, P.-J., J.-F. Tang, N. Yang, J.-J. Fang, X. He, and L.-M. Shao. 2012. The emission patterns of volatile organic compounds during aerobic biotreatment of municipal solid waste using continuous and intermittent aeration. J. Air Waste Manage. Assoc. 62:461–470. doi:10.1080/10962247.2012.658954
- Heyer, K.U., K. Hupe, M. Ritzkowski, and R. Stegmann. 2005. Pollutant release and pollutant reduction - Impact of the aeration of landfills. Waste Manage. 25:353–59. doi:10.1016/j.wasman.2005.02.007
- Hirata, O., Y. Matsufuji, A. Tachifuji, and R. Yanase. 2012. Waste stabilization mechanism by a recirculatory semi-aerobic landfill with the aeration system. J. Mater. Cycles Waste Manage. 14:47–51. doi:10.1007/s10163-011-0036-7
- Jiang, J., Y. Yong, Y. Shihui, Y. Bin, and Z. Chang. 2010. Effects of leachate accumulation on landfill stability in humid regions of China. Waste Manage. 30:848–55. doi:10.1016/j.wasman.2009.12.005
- Kim, H., Y.-C. Jang, and T. Townsend. 2011. The behavior and long-term fate of metals in simulated landfill bioreactors under aerobic and anaerobic conditions. J. Hazard. Mater. 194:369–77. doi:10.1016/j.jhazmat.2011.07.119
- Ko, J.H., J. Powell, P. Jain, H. Kim, T. Townsend, and D. Reinhart. 2013. Case study of controlled air addition into landfilled municipal solid waste: Design, operation, and control. J. Hazard. Toxic Radioact. Waste 17:351–59. doi:10.1061/(ASCE)HZ.2153-5515.0000183
- Long, Y., L.-f. Hu, and D.S. Shen. 2009a. Nitrogen transformation in the hybrid bioreactor landfill. Bioresour. Technol. 100: 2527–33. doi:10.1016/j.biortech.2008.11.036
- Long, Y., Y. Long, H.C. Liu, and D.S. Shen. 2009b. Degradation of refuse in hybrid bioreactor landfill. Biomed. Environ. Sci. 22:303–10. doi:10.1016/S0895-3988(09)60060-X
- Mårtensson, A.M., C. Aulin, O. Wahlberg, and S. Ågren. 1999. Effect of humic substances on the mobility of toxic metals in a mature landfill. Waste Manage. Res. 17:296–304. doi:10.1177/0734242X9901700406
- Mertoglu, B., B. Calli, B. Inanc, and I. Ozturk. 2006. Evaluation of in situ ammonia removal in an aerated landfill bioreactor. Process Biochem. 41:2359–66. doi:10.1016/j.procbio.2006.06.014
- Nag, M., T. Shimaoka, H. Nakayama, T. Komiya, and C. Xiaoli. 2016. Field study of nitrous oxide production with in situ aeration in a closed landfill site. J. Air Waste Manage. Assoc. 66:280–87. doi:10.1080/10962247.2015.1130664
- National Bureau of Statistics of China. 2015. China Statistical Yearbook. Beijing, China: China Statistics Press.
- Prantl, R., M. Tesar, M. Huber-Humer, and P. Lechner. 2006. Changes in carbon and nitrogen pool during in-situ aeration of old landfills under varying conditions. Waste Manage. 26:373–80. doi:10.1016/j.wasman.2005.11.010
- Qu, X., P.-J. He, L.-M. Shao, and D.-J. Lee. 2008. Heavy metals mobility in full-scale bioreactor landfill: Initial stage. Chemosphere 70:769–77. doi:10.1016/j.chemosphere.2007.07.013
- Ritzkowski, M., K.U. Heyer, and R. Stegmann. 2006. Fundamental processes and implications during in situ aeration of old landfills. Waste Manage. 26:356–72. doi:10.1016/j.wasman.2005.11.009
- Ritzkowski, M., and R. Stegmann. 2010. Generating CO2-credits through landfill in situ aeration. Waste Manage. 30:702–06. doi:10.1016/j.wasman.2009.11.014
- Shao, L.-M., P.-J. He, and G.-J. Li. 2008. In situ nitrogen removal from leachate by bioreactor landfill with limited aeration. Waste Manage. 28:1000–07. doi:10.1016/j.wasman.2007.02.028
- Slezak, R., L. Krzystek, and S. Ledakowicz. 2010. Simulation of aerobic landfill in laboratory scale lysimeters—Effect of aeration rate. Chem. Pap. 64:223–29. doi:10.2478/s11696-009-0113-8
- Slezak, R., L. Krzystek, and S. Ledakowicz 2015. Degradation of municipal solid waste in simulated landfill bioreactors under aerobic conditions. Waste Manage. 43:293–99. doi:10.1016/j.wasman.2015.06.017
- Tong, H., K. Yin, A. Giannis, L. Ge, and J.-Y. Wang. 2015. Influence of temperature on carbon and nitrogen dynamics during in situ aeration of aged waste in simulated landfill bioreactors. Bioresour. Technol. 192:149–56. doi:10.1016/j.biortech.2015.05.049
- U.S. Environmental Protection Agency [EPA]. 2007. SW-846 Test Method 3015a. Microwave assisted acid digestion of aqueous samples and extracts.
- Xu, Q., X. Jin, Z. Ma, H. Tao, and J.H. Ko. 2014. Methane production in simulated hybrid bioreactor landfill. Bioresour. Technol. 168:92–6. doi:10.1016/j.biortech.2014.03.036
- Xu, Q., Y. Tian, S. Wang, and J.H. Ko. 2015. A comparative study of leachate quality and biogas generation in simulated anaerobic and hybrid bioreactors. Waste Manage. 41:94–100. doi:10.1016/j.wasman.2015.03.023
- Yao, J., Q. Kong, W. Li, H. Zhu, and D. Shen. 2014. Effect of leachate recirculation on the migration of copper and zinc in municipal solid waste and municipal solid waste incineration bottom ash co-disposed landfill. J. Mater. Cycles Waste Manage. 16:775–83. doi:10.1007/s10163-013-0217-7
- Yazdani, R., M.E. Mostafid, B. Han, P.T. Imhoff, P. Chiu, D. Augenstein, M. Kayhanian, and G. Tchobanoglous. 2010. Quantifying factors limiting aerobic degradation during aerobic bioreactor landfilling. Environ. Sci. Technol. 44(16):6215–6220. doi:10.1021/es1022398
- Zhang, D.Q., S.K., Tan, and R.M. Gersberg. 2010. Municipal solid waste management in China: Status, problems and challenges. J. Environ. Manage. 91:1623–33. doi:10.1016/j.jenvman.2010.03.012
- W.-j Zhang., G.-g Zhang, and Chen, Y.-m. 2013. Analyses on a high leachate mound in a landfill of municipal solid waste in China. Environ. Earth. Sci. 70:1747–52. doi:10.1007/s12665-013-2262-x