ABSTRACT
A long-term monitoring of composition of landfill gases in the region with high rainfall was conducted using an argon assay in order to discuss air intrusion into the dump site. Gas samples were taken from vertical gas monitoring pipes installed along transects at two sections (called new and old) of an abandoned waste dump site in Sri Lanka. N2O concentrations varied especially widely, by more than three orders of magnitude (0.046–140 ppmv). The nitrogen/argon ratio of landfill gas was normally higher than that of fresh air, implying that denitrification occurred in the dump site. Argon assays indicate that both N2 and N2O production occurred inside waste and more significantly in the old section. The Ar assay would help for evaluations of N2O emission in developing countries.
Implications: A long-term monitoring of composition of landfill gases in the region with high rainfall was conducted using an argon assay in order to discuss air intrusion into the dump site. Argon assays indicate that both N2 and N2O production occurred inside waste and more significantly in the old section.
Introduction
Gases emitted from solid waste dump sites consist of mainly methane (CH4) and carbon dioxide (CO2), which are generated by microbial processes within a waste body and/or a soil cover (Bogner et al., Citation1995). Several studies reported that the landfill gas also includes trace organic gases such as aromatic carbons, nonmethane hydrocarbons, and inorganic gases such as hydrogen sulfide (H2S) and nitrous oxide (N2O), creating a high greenhouse effect (Redfearn et al., Citation2002; Eklund et al., Citation1998). Based on investigations of the composition and flux of landfill gases such as CH4 and CO2, various studies have examined the stabilization of buried waste and the contribution of landfill gas emission to global warming (Widory et al., Citation2012; Capaccioni et al., Citation2011). On the other hand, the 2006 Intergovernmental Panel on Climate Change (IPCC) Guidelines did not include a methodology for estimating N2O emissions from solid waste dump sites due to the lack of a reasonable model for evaluating greenhouse gas emissions incorporated with N2O emissions. This is partly because there are few studies that have investigated landfill gas compositions including N2O and N2O emissions in parallel with CH4 (Bogner et al., Citation2011; Zhang et al., Citation2013).
In developing countries, open dumping of waste is the most common practice, and collected solid waste is mostly dumped without any treatment or segregation. The generation of landfill gas including N2O seems to be significant due to the decomposition of dumped waste because the waste is normally rich in organic components with high nitrogen content (e.g., Wijewardane et al., Citation2013; Chandrappa and Das, Citation2012). However, due to the lack of long-term monitoring data, temporal variations in landfill gases including N2O have not been fully understood. Furthermore, very limited data and knowledge are available, especially on air intrusion into the open solid waste dump site and microbial processes inside the buried waste.
N2O is produced predominantly by microbial processes as a by-product of nitrification (Smith et al., Citation2003) and as a product of the incomplete denitrification that occurs at the boundary between aerobic and anaerobic zones (Desloover et al., Citation2011). N2O emissions are affected by several factors, such as concentrations of oxygen and nitrite in both nitrification and denitrification mechanisms (Beline et al., Citation2001; Kampschreur et al., Citation2008), availability of organic carbon as substrates for heterotrophic denitrifying bacteria in the denitrification mechanisms (Itokawa et al., Citation2001; Park et al., Citation2000), soil pH, temperature, and water content (Goodroad and Keeney, Citation1984). Several studies of waste dump sites and landfills suggested that ammonium oxidation by methanotrophs in the soil cover might be one of the processes responsible for N2O emission (Bogner et al., Citation2011; Zhang et al., Citation2009; Borjesson and Svensson, Citation1997). It should be noted, however, that the nitrogen source for the N2O emissions might be insufficient in the soil cover even if we supply sludge/compost to the soil cover and recirculate landfill leachate into landfills as a nitrogen source (Lee et al., Citation2002). On the other hand, it has been reported that CH4 and N2O were generated from both buried waste and soil cover after a rainfall due to several processes, that is, by anaerobic digestion of buried waste and by nitrification and denitrification in the soil cover (Zhang et al., Citation2013). In addition, Tsujimoto et al. (Citation1994) supposed that the high N2O emission from a sea area waste disposal site was attributable to the increase in N2O production under the anaerobic condition and to evaporation of dissolved N2O from water retained inside. Further investigations about N2O production from waste disposal sites located in not only sea areas but also regions with high rainfall are required.
In order to assess the origin of a target gas, inert gases such as argon (Ar), helium (He), and their isotopes have been utilized in various fields, including geochemistry and waste management research (Kiyosu et al., Citation1986; Minissale et al., Citation1999; Kobashi et al., Citation2008; Chun, Citation2014). This is because these inert gases will not interfere with and/or participate in biochemical reactions in either soil strata or waste landfills. For example, Kiyosu et al. (Citation1986) investigated the nitrogen (N2)/Ar and He/Ar ratios of volcanic and geothermal gases from several volcanic areas in Japan and revealed that their ratios were higher than those of atmospheric air dissolved in groundwater. This implies that the N2 and He in these volcanic and geothermal gases were mainly derived from the magmatic gas discharged and were not contributed by the atmospheric air dissolved in groundwater. This approach can be applied to investigate the actual composition of landfill gas without the effect of air intrusion from the atmosphere. When using Ar (which is the third largest component of atmospheric gas) as an inert gas, the method is called an argon assay. For example, Chun (Citation2014) conducted argon assays of landfill gas samples and examined whether N2 gases in the landfill originated from the denitrification process inside the waste or air intrusion from the atmosphere.
The objectives of this study were therefore (i) to characterize temporal variations in landfill gas based on a long-term monitoring at an abandoned waste dump site in Sri Lanka located in the region with high rainfall, and (ii) to conduct argon assays in order to discuss air intrusion into the dump site and microbial processes of waste, particularly focusing on correlations between N2O and other major gases. It has been reported that a significant linear correlation between CH4 emission rates and mean CH4 concentrations in the waste layers (Hrad et al., Citation2012). Thus, our results would also contribute to evaluate landfill gas emission and its temporal variation from an abandoned waste dump site.
Materials and methods
Site description and gas monitoring wells
Monitoring of landfill gas was conducted at an abandoned waste dump site (N 7º09’, E 80º35’) located on a steeply sloping right bank of the Mahaweli River in Udapalatha Pradeshiya Sabha in the Central Province of Sri Lanka. The annual rainfalls recorded at a weather station at the Evalgolla Estate of the Gampola Urban Council about 9 km away from the dump site were 3239 mm and 3529 mm in 2013 and 2014, respectively (Department of Meteorology, Sri Lanka, 2015). The dump site has been used by both Gampola Urban Council and Udapalatha Pradeshiya Sabha for around 8 years. The outline of the dump site is summarized in , and the locations of gas monitoring wells are shown in . The dump site consists of two sections, namely, the old and new sections. The old section was used for waste dumping for 7 years, 2003–2010, and the new section was used for 0.5 year in 2011. The majority of dumped waste was domestic garbage such as kitchen waste or plastics, but construction and demolition waste was also found. There were no daily covers, final cap, or gas extraction wells at this site. At the old section, most of the ground surface was covered with a loamy local soil (less than 1 m thickness) and weeds. At the new section, the ground surface was partially covered with soil and weeds, but the dumped garbage was mostly exposed to the surface. A landslide from torrential rains in February 2012 occurred at the new section before this study.
Table 1. Outline of the dump site.
In total, five gas monitoring wells, PBH1–PBH5, were installed along transect lines at the old and new sections (). PBH1 and PBH2 were located on the transect line of lower and upper slopes of the new section. PBH3, PBH4, and PBH5 were located on the transect line on lower, middle, and upper slopes, respectively, of the old section. The plots near PBH2 and PBH5 had been used previously for measuring surface fluxes of CH4 and N2O (Nagamori et al., Citation2012). A dry rotary bore was used to establish a vertical monitoring well with a casing size of 127 mm. The longitudinal section of the monitoring well is shown in . A perforated high-density polyethylene pipe with inner and outer diameters of 50 mm and 63 mm was used for the monitoring well. The gap surrounding the pipe was backfilled with gravel (size 8–12.5 mm). The length of the monitoring well was adjusted according to the thickness of the waste layer: The length of PBH5 was 8.5 m, while other monitoring wells were 3.8–4.5 m long. During the gas monitoring period, perched water was observed inside the monitoring wells. In the old section PBH3 and PBH4 had shallow water tables less than 1 m depth from the ground surface, but PBH5 had no water or a deeper water table. In the new section, water tables fluctuated with time and were 1.5–2.2 m for PBH1 and 1.8–3.3 m for PBH2 (see more details in Nagamori et al., Citation2015).
Gas sample collection and analysis
The landfill gas was monitored for 2 years from March 2013 at approximately 1-month intervals. The internal gas of each monitoring well was sampled using a gas pump (MP-15CF; Shibata Scientific, Tokyo, Japan) into an airtight aluminum bag equipped with a silicone sampling tube at a flow rate of 0.5–1.0 L min−1. In order to avoid the intrusion of ambient air and perched water as much as possible, the inlet of the sampling tube was set just above the water table. In addition, the preexisting gas inside the sampling tube was fully removed before sampling the landfill gas. At the same time as gas sampling, temperatures of gas in the monitoring well PBH5 and perched water (except PBH5) were measured.
The gas samples were used for analyzing typical landfill gas components, such as CH4, CO2, O2 (including Ar), and N2, using gas chromatography (GC) (Nagamori et al., Citation2008). shows the GC conditions set for measuring trace gases such as hydrogen (H2), H2S, N2O, and Ar. In order to determine Ar concentrations of the samples, the gas samples taken from April 2014 were analyzed by separating Ar from O2 following the method shown in .
Table 2. GC conditions for measuring landfill gas components of H2S, N2O, Ar, and O2.
Evaluation of actual generation gas using an argon assay
Only Ar is considered to be an inert or unchanged gas in waste dump sites, because there is a possibility of N2 production due to denitrification and/or nitrification (Lee et al., Citation2009). The component gas originating only from biochemical processes in the waste layer, (C/Ar)LFG, which excludes the effect of air intrusion from the sampled gas, can be represented as
where [C] and [Cair] are the concentrations of each gas component in the sampled gas and the ambient air, and [Ar] and [Arair] are the concentrations of Ar in the sampled gas and the ambient air (Kiyosu et al., Citation1986). On a volume basis, dry air contains 78.09% [N2-air], 20.95% [O2-air], and 0.934% [Arair] (National Oceanic and Atmospheric Administration [NOAA], 1976). For other components, 1.82 ppmv of [CH4-air], 326 ppbv of [N2Oair] (World Meteorological Organization [WMO], 2014), and 398 ppmv of [CO2-air] were reported in June 2014 (NOAA, 2015).
Results and discussion
Variations in concentrations of major components of landfill gas
Temporal variations in concentrations of major components of landfill gas, CH4, CO2, O2 + Ar, and N2, are shown in . In the figure, the ratio of CH4 to the sum of CH4 and CO2, RCH4, which is used to evaluate the degree of stabilization of buried waste (Chun, Citation2014), is also shown. At PBH1 and PBH2 of the new section, high concentrations of CH4 (approximately 60%) and CO2 (20–30%) can be observed in parallel with low concentrations of O2 + Ar and N2, probably due to active biodegradation of buried waste during the first 10 months after March 2013. At PBH3 and PBH4 of the old section (located at the lower slope), on the other hand, N2 concentrations were approximately 80% (similar to that of atmospheric air), suggesting that air intrusion into both monitoring wells was more significant than the biodegradation of buried waste. On the other hand, during the first 10 months, the CH4 and CO2 concentration ranges were 30–60% and 10–20%, respectively, at PBH5 located at the upper slope of the old section, implying that the buried waste was decomposed steadily under anaerobic biodegradation. This might be attributed to the thickness of buried waste at the old section; that is, the thicker waste layer of PBH5 (8.5 m) restricted the air intrusion into the deeper waste layer.
Figure 3. Variations in concentrations of major gas components (CH4, CO2, O2 + Ar, N2), and RCH4 at new (left) and old (right) sections from April 2013 to March 2015. Rainfall precipitation data are also shown.
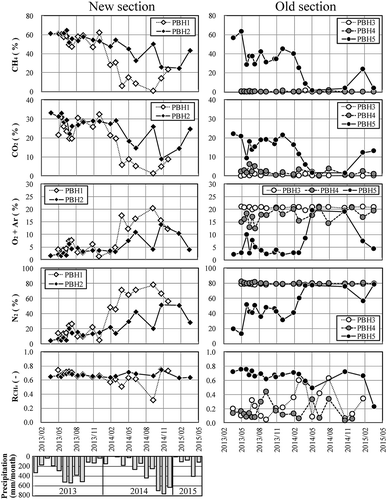
Decreases in CH4 concentration can be observed at PBH1 (new section) and PBH5 (old section), and a gradual decrease in CH4 concentration can be observed at PBH2 (new section) during the first 10 months after March 2013. The reason is not clear, but very little rainfall was recorded at the weather station during this period. This might have caused air intrusion into the monitoring wells and subsequently reduced those monitored CH4 concentrations. There were no other factors recorded that would affect the CH4 concentrations: no significant decrease in the perched water level in the monitoring wells (Nagamori et al., Citation2015), and no drastic change in the concentration of dissolved organic carbon in the perched water (unpublished data). Moreover, the CH4 concentrations at PBH1 and PBH5 increased again after the drop, implying that some temporary factors, such as an increase/decrease in the quantity of rainwater penetrating the waste layer and fluctuation in atmospheric pressure, might also affect the landfill gas composition (Gebert et al., Citation2011).
RCH4 values typically higher than 0.6 were recorded at PBH1 and PBH2 in the new section. This evidence demonstrates that the anaerobic gasification due to biodegradation of buried waste continued irrespective of high and low amounts of rainfall precipitation. On the other hand, the RCH4 values at PBH3 and PBH4 of the old section were mostly lower than 0.4, with some fluctuations. The low RCH4 values of PBH3 and PBH4 indicate methanogenic phase almost ceased. The RCH4 values for PBH5 of the old section were higher than 0.6 during the initial several months of the monitoring; however, they tended to gradually decrease with increasing O2 + Ar and N2 after February 2014.
Variations in concentrations of trace gas components
Temporal variations in concentrations of H2S and N2O are shown in . The H2S concentrations at all monitoring wells were less than 50 ppmv, and the higher concentrations (>1.0 ppmv) were observed at PBH1 and PBH2 (new section) and at PBH5 (old section). At PBH3 and PBH4 (located at the lower slope) of the old section, measured H2S became low, and sometimes those values became less than the 0.01 ppmv of the minimum limit of determination. All measured H2 concentrations were less than the minimum limit of determination (0.01%; data not shown).
Figure 4. Variations in concentrations of trace gases, H2S and N2O, at new (left side) and old (right side) sections from April 2013 to March 2015.
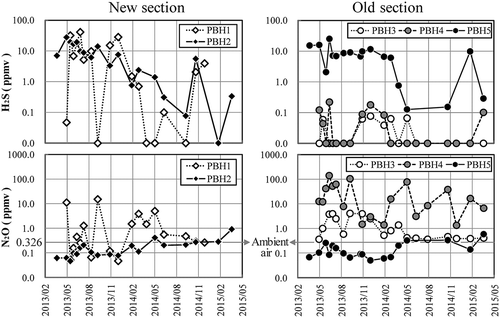
It is noted that the N2O concentrations varied widely, by more than three orders of magnitude (0.046–140 ppmv). Especially, measured values for PBH4 became higher compared to others and in a range of 1.3–140 ppmv with fluctuations. In contrast, the N2O concentrations at PBH2 and PBH5 (located at the upper slope) were lower and mostly were less than the N2O concentration of ambient air (= 0.326 ppmv). Additionally, it is noted that no rapid decreases in the N2O concentrations were observed as for CH4 and CO2 concentrations during the first 10 months after March 2013 (see ). In this site, the increase or decrease of the N2O in the period with a little rainfall was not seen clearly.
The monitored results of N2O concentrations in this study were partially in accordance with the previous reported data on the measured gas flux from the ground surface. Nagamori et al. (Citation2012) measured N2O fluxes at several points of new and old sections of the dump site and reported that higher values of N2O flux (>0.01 mL m−2 min−1 [0.028 g m−2 d−1]) were observed around PBH3 and PBH4 (lower slope) of the old section and PBH1 (lower slope) of the new section than those for other monitoring wells.
shows a negative correlation between concentrations of H2S and N2O of all the samples. This was in agreement with a previous study (Senga et al., Citation2006) using two strains of N2O-producing bacteria isolated from the sediment in brackish lake, which obtained higher production rates of N2O when the H2S inhibited the N2O reduction to N2 during denitrification. One of the bacteria, Aeromonas sp. was also found in the waste landfill leachates of tropical climate zones (Sawamura et al., Citation2007). A simulated intermittent aerated semi-aerobic bioreactor landfill (Sun et al., Citation2013) has also reported that the periods of production of H2S and N2O are different from each other.
Variations in argon concentration and its ratios to oxygen and nitrogen
Variations in the concentration of Ar and its ratios to O2 and N2, [O2]/[Ar] and [N2]/[Ar], are shown in . As described in the preceding, analysis of the Ar concentration began with the gas samples taken from April 2014. For all samples, the measured Ar concentrations were less than that of ambient air (= 0.934%). Especially low Ar concentrations, ranging from 0.252 to 0.579%, were observed at PBH2 of the new section. For PBH1 and PBH2 of the new section, the Ar concentrations tended to vary following the gradual increase or decrease in O2 and N2 concentrations. The lower [O2]/[Ar] value of PBH2 after February 2015 may be due to the larger O2 consumption in the waste layer.
Figure 6. Variations in concentrations of Ar, O2, and N2, and ratios of O2 and N2 to Ar, O2/Ar and N2/Ar, at new (left) and old (right) sections from April 2014 to March 2015.
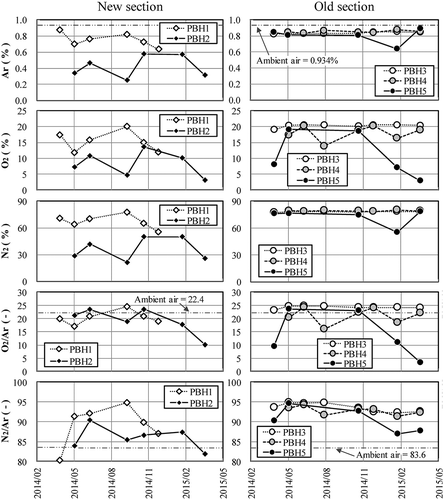
The [N2]/[Ar] values were normally higher than those of fresh air (= 83.6%), implying that denitrification occurred in the dump site. In the old section, on the other hand, the [N2]/[Ar] values tended to decrease slightly with time irrespective of the constant concentrations of Ar. This indicates that a reduced production of N2 gas (reduction in denitrification) might have occurred in the waste layer.
Evaluation of nitrous oxide production based on argon assays
Using measured concentrations of component gases, N2O, CH4, CO2, O2, and N2, from April 2014 to March 2015, the (C/Ar)LFG values were calculated by eq 1 and are shown in . In the figure, log(N2O/Ar)LFG values were plotted against log(C/Ar)LFG (where C corresponds to CH4, CO2, O2, and N2) to show the relationship between N2O production and reduction potentials. Pearson correlation coefficients between log(N2O/Ar)LFG and log(C/Ar)LFG were calculated and are summarized in .
Figure 7. Relationships between log(N2O/Ar)LFG and log(C/Ar)LFG (where C corresponds to CH4, CO2, O2, and N2) at new (left) and old (right) sections from April 2014 to March 2015. The origin of coordinate axes, (0,0), shows that the gas concentration of the measured sample became same as that of ambient air. Regression lines with |r2|> 0.68 are also shown in the figure.
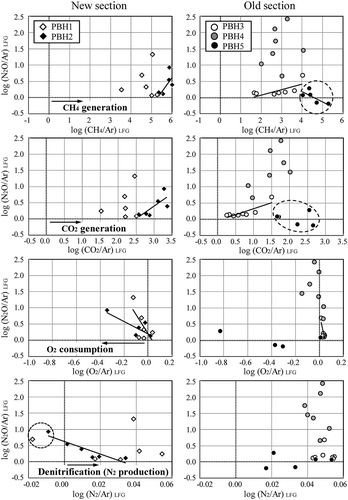
Table 3. Pearson correlation coefficients (r2) between log(N2O/Ar)LFG and log(C/Ar)LFG (where C corresponds to CH4, CO2, O2, and N2) at each well.
As shown in , all log(CH4/Ar)LFG and log(CO2/Ar)LFG values became greater than 0, which means that CH4 and CO2 generation was dominant in the dump site. Except for PBH5, the log(N2O/Ar)LFG normally increased with increases in either log(CH4/Ar)LFG or log(CO2/Ar)LFG, and relatively high linear correlations (r2 > 0.68) are observed for PBH2 of the new section and PBH3 of the old section (). This suggests that microbial processes under a strict anaerobic condition restricted at our monitored waste dump site, at which waste layers were relatively thin (3.8–4.5 m) except for the location of PBH5. For PBH5, which was located at the upper slope of the old section, negative correlations between log(N2O/Ar)LFG and either log(CH4/Ar)LFG or log(CO2/Ar)LFG were found. The reason for this is not clear, but the thick buried waste at PBH5 (8.5 m) might have blocked air intrusion into the deeper waste layer, resulting in a less suitable condition for N2O production. Measured log(O2/Ar)LFG values became mostly smaller than 0, indicating that O2 consumption occurred in the waste dump site. Especially, the higher O2 consumption observed at PBH3 and PBH4 of the old section (located at lower slope) can be attributed to higher air intrusion into monitoring wells, as explained earlier.
Except for a few plots from the new section (circled with broken line in the figure), measured log(N2/Ar)LFG became greater than 0, suggesting that denitrification (N2 production) occurred dominantly in the waste dump site. In particular, denitrification seems to have been more significant at PBH3 and PBH4 of the old section with high log(N2/Ar)LFG values. However, there were no clear correlations between log(N2O/Ar)LFG and log(N2/Ar)LFG (|r2|< 0.18) except for PBH2 (r2 = 0.91). It is noted that our measured (N2/Ar)LFG values became similar to those reported in Chun (Citation2014) (differences < |0.06|).
Based on our monitored data, it is difficult to clarify whether the N2O was produced by either nitrification or denitrification because N2O is normally produced by microbial processes as a by-product of nitrification and as an intermediate product of denitrification (Smith et al., Citation2003). However, overall, it can be said that N2 and N2O production resulted predominantly in denitrification in the old section, irrespective of the effect of air intrusion into monitoring wells. Once the biodegradable carbon sources were insufficient, which limited the activity of denitrifying organisms, higher N2O production began (Sun et al., Citation2013). The median chemical oxygen demand (COD) values of the perched water in the old section (PBH3 = 106, PBH4 = 162), lower than those in the new section (PBH1 = 656, PBH2 = 639), exhibited a situation similar to the previous study. Moreover, it is interesting that N2 consumption occurred at monitoring wells of the new section (circled with a broken line in the figure). The reason for this is not clear, and further studies are needed to determine which environmental factors control the N2 production and consumption under natural conditions.
Conclusions
Landfill gas was monitored for 2 years at a waste dump site in Sri Lanka. Results showed that temporal variations in landfill gas components were highly affected by air intrusion into monitoring wells and microbial processes in the waste layer. Measured N2O concentrations varied especially widely, by more than three orders of magnitude (0.046–140 ppmv). The highest N2O concentration was measured on the middle slope of the old section in a range of 1.3–140 ppmv. Low Ar concentrations at PBH2 located on the top of the new section within 3 years after dumping showed small amounts of air intrusion into the dump site had occurred. Based on the results of argon assays, it is indicated that microbial processes inside the waste under a strict anaerobic condition were restricted at our monitored waste dump site. It is implied that both N2 and N2O were produced predominantly in denitrification, and that denitrification in the waste layer tended to slightly decrease with time. The Ar assay would help for evaluations of N2O emission in developing countries.
Funding
This work was supported by a research grant from the JST/JICA Science and Technology Research Partnership for Sustainable Development (SATREPS).
Additional information
Funding
Notes on contributors
Masanao Nagamori
Masanao Nagamori is a senior researcher at the Material Cycles and Waste Management Group, Center for Environmental Science in Saitama, in Saitama, Japan.
M.I.M. Mowjood
M.I.M. Mowjood is a director for the Department of Agricultural Engineering, Faculty of Agriculture, University of Peradeniya, in Peradeniya, Sri Lanka.
Youichi Watanabe
Youichi Watanabe and Yugo Isobe are senior researchers at the Material Cycles and Waste Management Group, Center for Environmental Science in Saitama, in Saitama, Japan.
Tomonori Ishigaki
Tomonori Ishigaki is a senior researcher at the Center for Material Cycles and Waste Management Research, National Institute for Environmental Studies, in Ibaraki, Japan.
Ken Kawamoto
Ken Kawamoto is a research professor at the Graduate School of Science and Engineering, Saitama University, in Saitama, Japan.
References
- Abushammala, M.F.M., N.E.A. Basri, R. Elfithri, M.K. Younes, and D. Irwan. 2014. Modeling of methane oxidation in landfill cover soil using an artificial neural network. J. Air Waste Manage. Assoc. 64:150–59. doi:10.1080/10962247.2013.842510.
- Beline, F., J. Martinez, C. Marol, and G. Guiraud. 2001. Application of the 15N technique to determine the contributions of nitrification and denitrification to the flux of nitrous oxide from aerated pig slurry. Water Res. 35:2774–78. doi:10.1016/S0043-1354(00)00561-3.
- Bogner, J.E., K.A. Spokas, and J.P. Chanton. 2011. Seasonal greenhouse gas emissions (methane, carbon dioxide, nitrous oxide) from engineered landfills: Daily, intermediate, and final California cover soils. J. Environ. Qual. 40:1010–20. doi:10.2134/jeq2010.0407.
- Bogner, J.E., K.A. Spokas, E. Burton, R. Sweeney, and V. Corona. 1995. Landfills as atmospheric methane sources and sinks. Chemosphere 31:4119–30. doi:10.1016/0045-6535(95)80012-A.
- Borjesson, G., and B.H. Svensson. 1997. Nitrous oxide emissions from landfill cover soils in Sweden. Tellus 49B:357–63. doi:10.3402/tellusb.v49i4.15974.
- Capaccioni, B., C. Caramelli, F. Tatàno, and A. Viscione. 2011. Effects of a temporary HDPE cover on landfill gas emissions: Multiyear evaluation with the static chamber approach at an Italian landfill. Waste Manage. 31:956–965.
- Chandrappa, R., and D.B. Das. 2012. Waste quantities and characteristics. In Solid Waste Management, ed. R. Chandrappa and J. Brown, 57–62. Heidelberg, Germany: Springer.
- Chun, S.K. 2014. The influence of air inflow on CH4 composition ratio in landfill gas. J. Material Cycle Waste Manage. 16:172–77. doi:10.1007/s10163-013-0163-4.
- Department of Meteorology, 2015. Climate in Sri Lanka. http://www.meteo.gov.lk/index.php?option=com_content&view=article&id=106&Itemid=81&lang=en (accessed February 18, 2016).
- Desloover, J., H. De Clippeleir, P. Boeckx, G. Du Laing, J. Colsen, W. Verstraete, and S.E. Vlaeminck. 2011. Floc-based sequential partial nitritation and anammox at full scale with contrasting N2O emissions. Water Res. 45:2811–21. doi:10.1016/j.watres.2011.02.028.
- Eklund, B., E.P. Anderson, B.L. Walker, and D.B. Burrows. 1998. Characterization of landfill gas composition at the Fresh Kills municipal solid waste landfill. Environ. Sci. Technol. 32:2233–37. doi:10.1021/es980004s.
- Gebert, J., I. Rachor, I. Gröngröft, and E.M. Pfeiffer. 2011. Temporal variability of soil gas composition in landfill covers. Waste Manage. 31:935–45. doi:10.1016/j.wasman.2010.10.007.
- Goodroad, L.L., and D.R. Keeney. 1984. Nitrous oxide production in aerobic soils under varying pH, temperature and water content. Soil Biol. Biochem. 16:39–43. doi:10.1016/0038-0717(84)90123-8.
- Hrad, M., M. Huber-Humer, B. Wimmer, and T.G. Reichenauer. 2012. Design of top covers supporting aerobic in situ stabilization of old landfills—An experimental simulation in lysimeters. Waste Manage. 32:2324–35. doi:10.1016/j.wasman.2012.06.004.
- Itokawa, H., K. Hanaki, and T. Matsuo. 2001. Nitrous oxide production in high-loading biological nitrogen removal process under low COD/N ratio condition. Water Res. 35:657–64. doi:10.1016/S0043-1354(00)00309-2.
- Kampschreur, M.J., W.R.L. van der Star, H.A. Wielders, J.W. Mulder, M.S.M. Jetten, and M.C.M. van Loosdrecht. 2008. Dynamics of nitric oxide and nitrous oxide emission during full-scale reject water treatment. Water Res. 42:812–26. doi:10.1016/j.watres.2007.08.022.
- Kiyosu, Y. 1986. Variations in N2/Ar and He/Ar ratios of gases from some volcanic areas in northeastern Japan. Geochem. J. 19:275–81. doi:10.2343/geochemj.19.275.
- Kobashi, T., J.P. Severinghaus, and K. Kawamura. 2008. Argon and nitrogen isotopes of trapped air in the GISP2 ice core during the Holocene epoch (0–11,500 B.P.): Methodology and implications for gas loss processes. Geochim. Cosmochim. Acta 72:4675–86. doi:10.1016/j.gca.2008.07.006.
- Lee, C.M., X.R. Lin, C.Y. Lan, S.C.L. Lo, and G.Y.S. Chan. 2002. Evaluation of leachate recirculation on nitrous oxide production in the Likang landfill, China. J. Environ. Qual. 31:1502–8. doi:10.2134/jeq2002.1502.
- Lee, S. W., J. Im, A.A. DiSpirito, L. Bodrossy, M.J. Barcelona, and J.D. Semrau. 2009. Effect of nutrient and selective inhibitor amendments on methane oxidation, nitrous oxide production, and key gene presence and expression in landfill cover soils: Characterization of the role of methanotrophs, nitrifiers, and denitrifiers. Appl. Microbiol. Biotechnol. 85:389–403. doi:10.1007/s00253-009-2238-7.
- Lizik, W., J. Im, J.D. Semrau, and M.J. Barcelona. 2013. A field trial of nutrient stimulation of methanotrophs to reduce greenhouse gas emissions from landfill cover soils. J. Air Waste Manage. Assoc. 63:300–9. doi:10.1080/10962247.2012.755137.
- Minissale, A., G. Magro, F. Tassi, F. Frau, and O. Vaselli. 1999. The origin of natural gas emissions from Sardinia island, Italy. Geochem. J. 33:1–12. doi:10.2343/geochemj.33.1.
- Nagamori, M., Y. Ono, K. Kawamura, M. Yamada, T. Ishigaki, and Y. Ono. 2008. Changes in composition of gases emitted from a final landfill site—Major components and nonmethane hydrocarbons (C2–C6). J. Mater. Cycles Waste Manage. 19: 244–54(in Japanese). doi:10.3985/jswme.19.244.
- Nagamori, M., T. Koide, N.K. Wijewardane, Y. Watanabe, Y. Isobe, M.I.M. Mowjood, T. Ishigaki, and K. Kawamoto. 2012. Flux measurements of greenhouse gases from an abandoned open dumping site of solid waste in Sri Lanka. Proc. ICSBE 2012 International Conference on Sustainable Built Environment, Kandy, Sri Lanka, SBE/12/239.
- Nagamori, M., U. Kumarasinghe, S. Hettiarachchi, M.I.M. Mowjood, G.B.B. Herath, Y. Isobe, Y. Watanabe, Y. Inoue, and K. Kawamoto. 2015. Spatiotemporal variation of water quality around and inside an open solid waste dumpsite in Sri Lanka. Proceedings of the 3rd International Symposium on Advances in Civil and Environmental Engineering Practices for Sustainable Development (ACEPS 2015), Galle, Sri Lanka, 148–54.
- National Oceanic and Atmospheric Administration. 1976. Defining constants and equations. U.S. Standard Atmosphere, 1976. Washington, DC: U.S. Government Printing Office.
- National Oceanic and Atmospheric Administration. 2015. Trends in Atmospheric Carbon Dioxide. Global Greenhouse Gas Reference Network, ESRL Global Monitoring Division. http://www.esrl.noaa.gov/gmd/ccgg/trends/global.html (accessed February 18, 2016).
- Park, K.Y., Y. Inamori, M. Mizuochi, and K.H. Ahn. 2000. Emission and control of nitrous oxide from a biological wastewater treatment plant bioreactors. J. Biosci. Bioeng. 90:247–52. doi:10.1263/jbb.90.247.
- Redfearn, A., R.D. Roberts, J.C. Dockerty, M. May, and S.H. Hughes. 2002. Predictive health risk assessment for landfill gas. Waste Manage. CIWM Scientific & Technical Review December:14–27.
- Sawamura, H., M. Yamada, T. Miyagi, T. Ishigaki, and M. Ike. 2007. Microbial community analysis in waste landfill in tropical and subtropical climate zones. J. Jpn. Soc.Water Environ. 30:621–28 ( in Japanese). doi:10.2965/jswe.30.621.
- Senga, Y., K. Mochida, R. Fukumori, N. Okamoto, and Y. Seike. 2006. N2O accumulation in estuarine and coastal sediments: The influence of H2S on dissimilatory nitrate reduction. Estuarine Coastal Shelf Sci. 67:231–38. doi:10.1016/j.ecss.2005.11.021.
- Smith, K. A., T. Ball, F. Conen, K.E. Dobbie, H. Massheder, and A. Rey, 2003. Exchange of greenhouse gases between soil and atmosphere: interactions of soil physical factors and biological processes. Eur. J. Soil Sci. 54:779–91. doi:10.1046/j.1365-2389.2003.00567.x.
- Sun, Y., Y.N. Wang, X. Sun, H. Wu, and H. Zhang. 2013. Production characteristics of N2O during stabilization of municipal solid waste in an intermittent aerated semi-aerobic bioreactor landfill. Waste Manage. 33:2729–36. doi:10.1016/j.wasman.2013.08.013.
- Tsujimoto, Y., J. Masuda, J. Fukuyama, and H. Ito. 1994. N2O emissions at solid waste disposal sites in Osaka City. J. Air Waste Manage. Assoc. 44:1313–14. doi:10.1080/10473289.1994.10467327.
- Widory, D., E. Proust, G. Bellenfant, and O. Bour. 2012. Assessing methane oxidation under landfill covers and its contribution to the above atmospheric CO2 levels: The added value of the isotope (δ13C and δ18O CO2; δ13C and δD CH4) approach. Waste Manage. 32:1685–92. doi:10.1016/j.wasman.2012.04.008.
- Wijewardane, N.K., T. Koide, M.I.M. Mowjood, M. Nagamori, K. Kawamoto, and G.B.B. Herath. 2013. Clustering open dumpsites in Sri Lanka based on waste characteristics. Proceedings of Fourteenth International Waste Management and Landfill Symposium ( Sardinia 2013), Sardinia, Italy, 150.
- World Meteorological Organization. 2014. The state of greenhouse gases in the atmosphere. Based on global observations through 2013. Greenhouse Gas Bulletin, No. 10. http://www.wmo.int/pages/prog/arep/gaw/ghg/documents/GHG_Bulletin_10_Nov2014_EN.pdf (accessed February 18, 2016).
- Zhang, H., X. Yan, Z. Cai, and Y. Zhang. 2013. Effect of rainfall on the diurnal variations of CH4, CO2, and N2O fluxes from a municipal solid waste landfill. Sci. Total Environ. 442:73–76. doi:10.1016/j.scitotenv.2012.10.041.
- Zhang, H., P. He, and L. Shao. 2009. N2O emissions at municipal solid waste landfill sites: Effects of CH4 emissions and cover soil. Atmos. Environ. 43:2623–31. doi:10.1016/j.atmosenv.2009.02.011.