ABSTRACT
Introducing cow manure compost as an amendment in landfill-leachate-contaminated soils is proved to be an effective technique for the immobilization of Cd in this study. Landfill-leachate-contaminated soil was collected from an unlined landfill in China and amended with a different blending quantity of cow manure compost (0, 12, 24, 36, and 48 g per 200 g soil), which was made by mixing cow manure and chaff at a ratio of 1/1 and maturing for 6 months. pH values of five different blending quantity mixtures increased by 0.2–0.4, and the organic matter levels increased by 2.5–7%, during a remediation period of 5 weeks. Four fractions of Cd named exchangeable Cd, reducible Cd, oxidizable Cd, and residual Cd in soil were respectively analyzed by a sequential extraction procedure. Introducing the cow manure compost application resulted in more than 40% lower exchangeable Cd but a higher concentration of oxidizable Cd in soils, and mass balance results showed nearly no Cd absorption by applied material, indicating that transformation of exchangeable Cd into oxidization forms was the main mechanism of Cd immobilization when cow manure compost was used as an amendment. The Pearson correlation showed that increasing of pH values significantly improved the efficiency of Cd immobilization, with a correlation coefficiency of 0.940 (p < 0.05). This is the first attempt at heavy metal immobilization in landfill-leachate-contaminated soil by cow manure compost, and findings of this work can be integrated to guide the application.
Implications: Addition of cow manure compost (CMC) was effective in reducing exchangeable Cd in landfill-leachate-contaminated soils (LLCS). The immobilization effect of Cd was mainly assigned to the redistribution of labile soil Cd. Organic matter (OM) and pH value increased with CMC application. The pH values were more sensitive to Cd immobilization efficiency. It was proved that CMC can be safely and effectively used for the restoration of LLCS.
Introduction
Solid usually absorbs contamination from landfill leachates directly or indirectly. Among all contaminants in landfill-leachate-contaminated soil (LLCS), heavy metals are the most challenging, because of their properties of persistence, immutability, and not being degradable (Wang and Chen, Citation2015). As a consequence, heavy metals levels in the LLCS and surrounding soils were higher than the internationally acceptable limit for soils (Liu et al., Citation2013). Thus, techniques for target metals removal from LLCS are at top priority. Phosphorus-bearing materials have been widely applied in immobilization of heavy metals in landfill-leachate-contaminated soils (Cao et al., Citation2013). Plants can be potential phytoremediators for remediation of surface soil contaminated with landfill leachate (Meera and Agamuthu, Citation2012).
The application of organic matter for immobilization of Cd and other heavy metals in the contaminated soils has been recommended as an effective method in some earlier research (Pardo, Clemente, and Bernal, Citation2011; Martin Calvarro et al., Citation2014). Among different organic amendments, composts obtained from widely available wastes such as animal manure compost seem to be a good option for soil remediation techniques (Liu et al., Citation2009). First, animal manure compost was proved to have the ability to improve soil quality (physical and chemical properties, organic content) and to immobilize heavy metal ion (de la Fuente et al., Citation2010). Second, it can alter the distribution and availability of the metal elements in soil directly or indirectly (Bernal, Alburquerque, and Moral, Citation2009). What’s more, it has an ameliorative effect due to increased surface area and an increase in the number of specific adsorption sites (Shuman, Dudka, and Das, Citation2007). Lastly, various organic wastes such as animal wastes were disposed of by compost, which may offset the need to install conventional organic wastes treatment infrastructure with its higher construction and operation costs. The influence of manure compost amendment efficiency depends on the soil type and the characteristics of the amendment accord to earlier researches (Alburquerque, de la Fuente, and Bernal, Citation2011). Different amendments showed different effects on the leachability of different elements. General metal availability may be either increased or decreased by compost addition, depending on the type of amendment used (Farrell et al., Citation2010). Heavy metal distribution could be affected by changes in soil conditions such as pH, elemental carbon (EC), or P content as animal manure composts were added into soils (Chen et al., Citation2010). Soil remediated with animal manure composts was processed with significant increasing of pH value and organic matter (Zeng et al., Citation2011). The content of weak acid extractable heavy metal will decrease in alkaline or neutral soils, and more organic contents in soils may transfer weak acid extractable heavy metal into more stable patterns such as organic patterns and iron–manganese combination patterns (Olaniran, Balgobind, and Pillay, Citation2013). Research studies concerning mine and agriculture soils remediation using animal manure compost amendment were hot spots recently (Pérez-Esteban et al., Citation2012; Lashari et al., Citation2015). However, to the best of our knowledge, respiration for LLCS by animal manure compost was rarely reported.
This study chose cow manure compost (CMC) as soil conditioner because CMC has been shown by other researchers to be a good soil conditioner (Yang et al., Citation2013) and because there is a large amount of cow manure needing to be treated. Decomposed cow manure (DCM) application resulted in improvements of microbial biomass carbon, soil respiration, β-glucosidase, urease, acid phosphatase, and sulfatase activities in a lateritic soil in earlier work (Tripathy et al., Citation2008). Cow dung (CD) was used by other researchers to break down azadirachtin A in a tropical soil (Agyarko et al. Citation2006). In this study, pH values, organic matter (OM) levels, and different patterns of Cd were analyzed. Different added dosages of CMC were added as a conditioner. The objectives of this article were to evaluate immobilization effects of CMC at different rates on Cd of LLCS by remediation experiments and to analyze the correlation between immobilization efficiency and soil characteristics such as pH values and organic matter. These finding in this work can be integrated to guide the application of CMC for Cd immobilization in LLCS.
Materials and methods
LLCS and CMC collection and characterization
The study LLCS was collected from Jinkou Landfill (30º 38’ 64” N, 114º 12’ 65” E) in Wuhan, China. This site was an unlined landfill used for more than 10 years, where bottom soil contacted directly with landfill leachate, and was remediated into a park in 2015. Several duplicate soil samples (5–6 m in deep) from different parts of the sites were obtained from the landfill area and mixed well into a single composite sample. The soil sample was dried at 20ºC for 1 week in a drying oven, sieved (<0.053 mm), and stored in polyethylene bags in a desiccator with calcium chloride to avoid contamination before the remediation experiments (Tokalioglu, Yilmaz, and Kartal, Citation2009).
The CMC was obtained from Jingdu Agricultural Science and Technology Development Company (30º 46’ 72” N, 111º 23’ 35” E) in Hubei, China. It was made from raw cow manure and chaff at a mass ratio of 1/1. During the precomposting period, pH and temperature were monitored until the optimum level of pH 7 ± 1 and temperature of 27 ± 1ºC were achieved, and it was stabilized by manual turning (Azizi et al., Citation2015). It then was matured for 6 months, air-dried, and sieved (<0.149 mm).
presents the characterization of LLCS and CMC. The pH values of the lixivium by mixing LLCS and CMC samples with deionized water with ratio of 1:2.5 (w/v) were measured with a pH meter (S400 SevenExcellence, pH/Mv). The organic matter was analyzed by potassium dichromate oxidation and titration with ferrous ammonium sulfate (Labra et al., Citation2010).
Table 1. Chemical characteristics of LLCS and CMC.
Remediation experiments with soil conditioners
The simulated experiments were conducted in the laboratory. Five different blending quantity mixtures of LLCS and CMC were done by fully mixing 200 g LLCS with CMC at five mass ratios of 200/0, 200/12, 200/24, 200/36, and 200/48, respectively (). Dry LLCS was mixed with CMC and then wetted to 60% of its water- holding capacity with deionized water. The related indexes of the mixture, such as pH values, OM contents, and four patterns of Cd, were determined after 3 days of standing. The remediation experiments lasted for 5 weeks and appropriate amounts of remediated soil were sampled for determination of the indexes at the end of each week. All of the experiments were performed in triplicate, and average values are listed in this paper.
Total Cd and fractionation of Cd in soils
There is a consensus in the literature that total soil metal content alone is not a good measure of short-term bioavailability and not a very useful tool to determine potential risks from soil contamination (Beesley et al., Citation2010; Nemati, Abu Bakar, Abas, Sobhanzadeh, et al., Citation2011), as parts of the metal contents were in stable forms that showed low contamination risk. Thus, we chose the four-stage sequential extraction procedure described by proposed by the Commission of the European Communities Bureau of Reference (BCR) for determination of exchangeable, reducible, oxidizable, and residual Cd (Nemati, Abu Bakar, Abas, and Sobhanzadeh, Citation2011) and proved as a dependable method to separate active and stable metal contents. The detailed extraction procedure is described as follows.
Exchangeable Cd: Forty milliliters of 0.11 M acetic acid was added to 1 g of soil sample in a centrifuge tube and shaken for 16 hr at room temperature. The extract was then separated from the solid residue by centrifugation and the filtrate was separated by decantation for 20 min at 3000 rpm.
Reducible Cd: Forty milliliters of hydroxylammonium chloride was added to the residue from the previous step in the centrifuge tube, and resuspended by mechanical shaking for 16 hr. The separation of the extract, collection of the supernatant, and rinsing of residues were the same as described for exchangeable Cd.
Oxidizable Cd: The residue in reducible Cd was treated twice with 10 mL of 8.8 M hydrogen peroxide. First, 10 mL hydrogen peroxide was added to the residue from the reducible Cd in the centrifuge tube. The digestion was allowed to proceed for 1 hr with occasional manual shaking, followed by digestion at 85 ± 2ºC for another 1 hr in a water bath. During the digestion, the centrifuge tube was loosely covered to prevent substantial loss of hydrogen peroxide. Then the centrifuge tube was uncovered and heating was continued until the volume reduced to about 2–3 mL. An additional 10 mL of hydrogen peroxide was added to the tube, covered, and digested with cover at 85 ± 2ºC for 1 hr. Heating was continued as before until the volume reduced to 2–3 mL. Finally, 50 mL of 1.0 M ammonium acetate was added to the cold mixture and shaken for 16 hr at room temperature. The separation of the extract, collection of the supernatant, and rinsing of residues were the same as described for exchangeable Cd.
Residual Cd: The residue from the oxidizable Cd was digested using a mixture of aqua regia and HF (Mohamed et al. Citation2015).
All of the samples were determined by flame atomic absorption spectrometry (AAS), and effects on Cd concentration from different added amounts of CMC were deducted when resulted Cd concentrations were calculated. A brief summary of the procedure is presented in . All patterns of Cd were detected in triplicate, and average values are listed in this paper.
Table 2. Code names of CMC supplemented experiments with different CMC rates.
Table 3. Extractants used at each extraction step and the extraction phases of sediments in the sequential extraction procedure.
Chemicals
Acetic acid, hydrogen peroxide, nitric acid, ammonium acetate, ferrous ammonium sulfate, and hydrochloric acid were purchased from Wuhan Huashen of Glass Trade Co., Ltd. China. Hydroxylammonium chloride was purchased from Wuhan Hong Rui Kang Reagent Co., Ltd. China. All of the other chemicals used in this study were of analytical grade.
Statistical analysis
The data sets were analyzed using the software SPSS 18.0. Differences between means were determined using a least significant difference (LSD) test. Correlation analysis was also used to analyze the relationship between immobilization efficiencies (end exchangeable Cd and immobilization rates for exchangeable Cd) and other chemical parameters (pH values and OM). The Pearson correlation coefficient (p) was chosen for assessment of the relationships. Within the analysis, end exchangeable Cd and first exchangeable Cd mean the detected exchangeable Cd concentration at the fifth and first week, respectively, and immobilization rates were calculated using the following formula:
Results and discussion
pH values of the mixtures
Most relevant research studies were concerned with the relationship between the initial soil pH and its accumulation of heavy metals (Petersell et al., Citation2009; Kazlauskaitė-Jadzevičė et al., Citation2014); however, the change trend of soil sample pH value during the remediation was rarely reported. The pH values of five different blending quantity mixtures increased by 0.2–0.4 (), and the pH values of group N4F4, with the most CMC added in, increased most significantly, by 0.4 units. The pH values in CMC added groups increased by 0.1 to 0.4 units as soon as CMC was added into LLCS, and kept increasing during the period from week 1 to week 2. During the period from week 3 to week 5, pH values decreased a little, which may be attributed to CO2 erosion and acid production of soil microorganisms, and stayed at a comparatively higher level than that in group N0F0 with no CMC application. Increasing of soil pH may facilitate the adsorption of Cd on various soil binding sites, thus decreasing the partition of Cd to soil solution.
Along with pH values increasing, exchangeable Cd decreased markedly by about 20% during the first week, with high pH values of 8.8–9.1, and slowly declined thereafter by <5% with comparatively lower pH values, which showed Cd immobilization effect by CMC occurred mainly during the first week across pH value >8.8 (). As our results showed, pH values are significantly correlated with end exchangeable Cd and immobilization rate, with r = –0.954 (p = 0.012) and r = –0.94 (p = 0.018), respectively (). All the results indicated that the change of sample pH has a high correlation with the Cd immobilization.
Table 4. Efficiency of Cd immobilization by different CMC application rates.
Table 5. Pearson correlation between end exchangeable Cd, immobilization rates for exchangeable Cd and other chemical parameters of amended LLCS.
Consistent with earlier works, soil pH is the most important factor controlling the extractability of heavy metals (Xu et al., Citation2015; Jin, Wang, and Al-Tabbaa, Citation2016), which can be also deduced with the correlation analysis between pH values and Cd immobilization efficiency in this article. Soil pH was found to play the most important role in determining metal speciation, solubility from mineral surfaces, movement, and eventual bioavailability of metals, due to its strong effects on solubility and speciation of metals both in the soil as a whole and particularly in the soil solution (Zeng et al., Citation2011).
Organic matter of the mixtures
Different CMC application rates resulted in different OM levels, as values of >2.5%, >4.0%, >5.0% and >7.0% OM levels were detected in LLCS of groups N1F1, N2F2, N3F3, and N4F4, respectively (). Higher CMC application rates resulted in a higher OM level, which was mainly attributed to a large amount of OM in CMC used in the amending experiments. OM decreased slowly and steadily by 9–15% during the experiment periods, which may be a result of CMC organic matter mineralization. In group N4F4 with the highest CMC applied ratio and the highest tested OM level, exchangeable Cd decreased by the highest rate, 41.6%.
The organic solutions, humic acid,s and microrganisms will take part in ion-exchange reactions in soils. The physical–chemical reaction between OM and Cd will help in transformation of Cd into more stable forms. OM will improve metabolism of microorganisms in soils, which may accelerate the transformation indirectly (Matos-Moreira et al., Citation2011). OM was revealed that increased along with increasing of CMC application rates. Exchangeable and oxidizable Cd showed a decreasing trend as OM increased during the experiments. CMC-amended soils resulted in higher OM contents compared with the soils without compost, and OM rising was positively related to CMC application rates; however, the effects were not significant (). In addition, OM decreased a little during the experiments. The group with the highest CMC rate, labeled N4F4, resulted in most significant increasing of OM during the first week.
Cadmium fractions in soils
The amounts of Cd in CMC amending experiments, labeled N0F0, N1F1, N2F2, N3F3, and N4F4, are presented in , , , , and , respectively. Among the five experiment groups, amounts of exchangeable Cd were detected as 32.2% to 37.9% of the total amounts. In N0F0 soil with no CMC, no significant variation was detected among the four different fractions of Cd during the experiment period. For the N1F1 group, a decreasing rate of exchangeable Cd was observed as 38%, and rates were 38.8%, 38.7%, and 40.6%, respectively, in groups N2F2, N3F3, and N4F4. The lowest level of Cd concentration was detected at the fifth week in group N4F4 as 0.76 mg/kg, and for groups N1F1, N2F2, and N3F3, it was 0.85 mg/kg, 0.88 mg/kg, and 0.83mg/kg, respectively.
Figure 4. The amounts of Cd fractions in N1F1 soil with amending rate of 12:200 (w/w) CMC/soil by sequential extraction scheme.
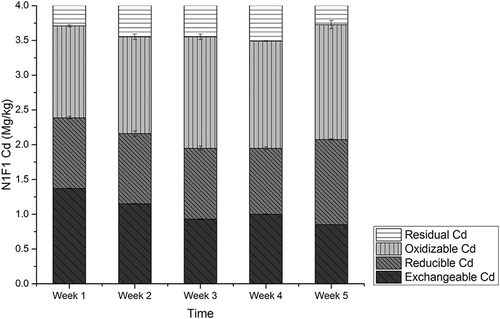
Figure 5. The amounts of Cd fractions in N2F2 soil with amending rate of 24:200 (w/w) CMC/soil by sequential extraction scheme.
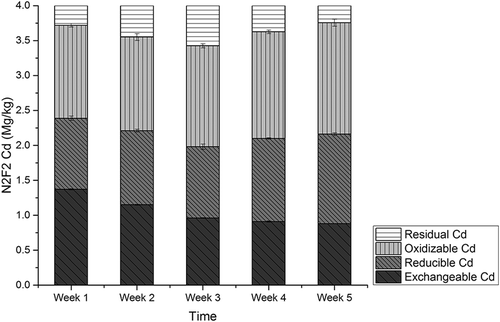
Figure 6. The amounts of Cd fractions in N3F3 soil with amending rate of 36:200 (w/w) CMC/soil by sequential extraction scheme.
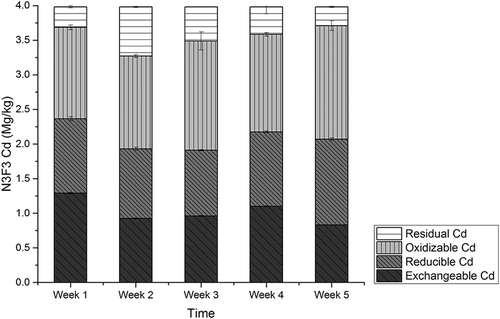
Figure 7. The amounts of Cd fractions in N4F4 soil with amending rate of 48:200 (w/w) CMC/soil by sequential extraction scheme.
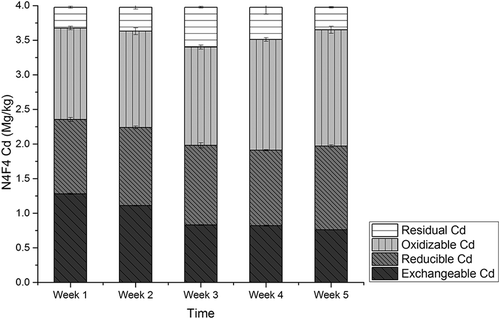
No significant variation was observed for reducible Cd; however, amounts of oxidizable Cd increased by 20.3%, 25.7%, 15.4%, and 12.6% for groups N1F1, N2F2, N3F3, and N4F4. The amounts of residual Cd increased first and decreased later during the experiments periods in all groups. The CMC application resulted in decreasing of exchangeable Cd by 35.7–41.6% and increasing of oxidizable Cd by 12.6–25.7%. These observations suggest that exchangeable Cd was converted to oxidizable form. The conversion of bioavailable Cd species to inert ones is vital in governing the uptake of Cd by plants and the phytotoxicity of the element (Mohamed et al., Citation2010). Cd mass balance analysis was conducted, and results showed that total Cd amounts from group N0F0 to N4F4 were unchanged, consistent with earlier research results (Liu et al., Citation2009), indicating that almost no Cd was adsorbed by CMC. Therefore, transformation of exchangeable Cd into oxidization forms may be the main mechanism of Cd immobilization with cow manure compost application.
Conclusion
Our results showed the promoting effect of introducing of CMC on the reduction of exchangeable Cd in LLCS. The alleviating effect of CMC on the toxicity of Cd could be assigned to the redistribution of Cd species in LLCS. Introducing CMC resulted in more than 40% exchangeable Cd decrease, with pH values increasing by 0.1–0.6 units and OM increasing by 2–5 times. Correlation analysis between parameters of pH values and the Cd immobilization efficiency in this study showed that increasing pH values promoted Cd immobilization processes. Increasing of oxidization Cd concentration and mass balance results suggested a remediation mechanism in which there was transformation from exchangeable Cd into oxidization Cd, rather than adsorption by CMC. Based on all the results described, CMC was proved as a safe and effective soil conditioner for Cd immobilization in LLCS.
Funding
The research reported here was supported by the Natural Science Foundation of China (number 51278212) and the Eleventh Five-Year National Technology Supporting Plan Program of China (number 2006BAC06B04).
Additional information
Funding
Notes on contributors
Zhuwei Liao
Zhuwei Liao and Jia Wang are Ph.D. candidates.
Jia Wang
Zhuwei Liao and Jia Wang are Ph.D. candidates.
Rui Wan
Rui Wan and Shuang Xi are engineers engaged in soil remediation.
Shuang Xi
Rui Wan and Shuang Xi are engineers engaged in soil remediation.
Zhuqi Chen
Zhuqi Chen is an associate professor.
Zhulei Chen
Zhulei Chen is a professor.
Yingjian Yu
Yingjian Yu is a Ph.D. candidate.
Sijie Long
Sijie Long is a master’s candidate.
Huabin Wang
Huabin Wang is a Ph.D. candidate.
References
- Agyarko, K., P.K. Kwakye, M. Bonsu, B.A. Osei, N. Asare Donkor, and E. Amanor. 2006. Breakdown of azadirachtin A in a tropical soil amended with neem leaves and animal manures. Pedosphere 16:230–36. doi:10.1016/S1002-0160(06)60048-9
- Alburquerque, J. A., C. de la Fuente, and M.P. Bernal. 2011. Improvement of soil quality after “alperujo” compost application to two contaminated soils characterised by differing heavy metal solubility. J. Environ. Manage. 92:733–41. doi:10.1016/j.jenvman.2010.10.018
- Azizi, A.B., M.Y. Choy, Z.M. Noor, and A. Noorlidah. 2015. Effect on heavy metals concentration from vermiconversion of agro-waste mixed with landfill leachate. Waste Manage. 38:431–35. doi:10.1016/j.wasman.2015.01.020
- Beesley, L., E. Moreno-Jimenez, R. Clemente, N. Lepp, and N. Dickinson. 2010. Mobility of arsenic, cadmium and zinc in a multi-element contaminated soil profile assessed by in-situ soil pore water sampling, column leaching and sequential extraction. Environ. Pollut. 158:155–60. doi:10.1016/j.envpol.2009.07.021
- Bernal, M.P., J.A. Alburquerque, and R. Moral. 2009. Composting of animal manures and chemical criteria for compost maturity assessment. A review. Bioresource Technol. 100:5444–53. doi:10.1016/j.biortech.2008.11.027
- Cao, X., Y. Liang, L. Zhao, and H. Le. 2013. Mobility of Pb, Cu, and Zn in the phosphorus-amended contaminated soils under simulated landfill and rainfall conditions. Environ. Sci. Pollut. Res. Int. 20:5913–21. doi:10.1007/s11356-012-1349-3
- Chen, G., G. Zeng, C. Du, D. Huang, L. Tang, L. Wang, and G. Shen. 2010. Transfer of heavy metals from compost to red soil and groundwater under simulated rainfall conditions. J. Hazard. Mater. 181:211–16. doi:10.1016/j.jhazmat.2010.04.118
- de la Fuente, C., R. Clemente, J. Martinez, and M. Pilar Bernal. 2010. Optimization of pig slurry application to heavy metal polluted soils monitoring nitrification processes. Chemosphere 81:603–10. doi:10.1016/j.chemosphere.2010.08.026
- Farrell, M., W.T. Perkins, P.J. Hobbs, G.W. Griffith, and D.L. Jones. 2010. Migration of heavy metals in soil as influenced by compost amendments. Environ. Pollut. 158:55–64. doi:10.1016/j.envpol.2009.08.027
- Jin, F., F. Wang, and A. Al-Tabbaa. 2016. Three-year performance of in-situ solidified/stabilised soil using novel MgO-bearing binders. Chemosphere 144:681–88. doi:10.1016/j.chemosphere.2015.09.046
- Kazlauskaitė-Jadzevičė, A., J. Volungevičius, V. Gregorauskienė, and S. Marcinkonis. 2014. The role of pH in heavy metal contamination of urban soil. J. Environ. Eng. Landscape Manage. 22:311–18. doi:10.3846/16486897.2013.872117
- Labra, M., F. De Mattia, M. Bernasconi, D. Bertacchi, F. Grassi, I. Bruni, and S. Citterio. 2010. The combined toxic and genotoxic effects of chromium and volatile organic contaminants to Pseudokirchneriella subcapitata. Water Air Soil Pollut. 213:57–70. doi:10.1007/s11270-010-0367-3
- Lashari, M.S., Y. Ye, H. Ji, L. Li, G.W. Kibue, H. Lu, J. Zheng, and G. Pan. 2015. Biochar-manure compost in conjunction with pyroligneous solution alleviated salt stress and improved leaf bioactivity of maize in a saline soil from central China: A 2-year field experiment. J. Sci. Food Agric. 95:1321–27. doi:10.1002/jsfa.2015.95.issue-6
- Liu, C., J. Cui, G. Jiang, X. Chen, L. Wang, and C. Fang. 2013. Soil heavy metal pollution assessment near the largest landfill of China. Soil Sediment Contam. 22:390–403. doi:10.1080/15320383.2013.733447
- Liu, L., H. Chen, P. Cai, W. Liang, and Q. Huang. 2009. Immobilization and phytotoxicity of Cd in contaminated soil amended with chicken manure compost. J. Hazard. Mater. 163:563–67. doi:10.1016/j.jhazmat.2008.07.004
- Martin Calvarro, L., A. de Santiago-Martin, J. Quiros Gomez, C. Gonzalez-Huecas, J. R. Quintana, A. Vazquez, A.L. Lafuente, T.M. Rodriguez Fernandez, and R. Ramirez Vera. 2014. Biological activity in metal-contaminated calcareous agricultural soils: the role of the organic matter composition and the particle size distribution. Environ. Sci. Pollut. Res. Int. 21:6176–87. doi:10.1007/s11356-014-2561-0
- Matos-Moreira, M., M.E. López-Mosquera, M. Cunha, M.J. Sáinz Osés, T. Rodríguez, and E.V. Carral. 2011. Effects of organic fertilizers on soil physicochemistry and on the yield and botanical composition of forage over 3 Years. J. Air Waste Manage. Assoc. 61:778–85. doi:10.3155/1047-3289.61.7.778
- Meera, M., and P. Agamuthu. 2012. Phytoextraction of As and Fe using Hibiscus cannabinus L. from soil polluted with landfill leachate. Int. J. Phytoremed. 14:186–99. doi:10.1080/15226514.2011.587481
- Mohamed, I., B. Ahamadou, M. Li, C. Gong, P. Cai, W. Liang, and Q. Huang. 2010. Fractionation of copper and cadmium and their binding with soil organic matter in a contaminated soil amended with organic materials. Journal of Soils and Sediments, 10:973–82. doi:10.1007/s11368-010-0199-1
- Mohamed, I., G.-s. Zhang, Z.-g. Li, Y. Liu, F. Chen, and K. Dai. 2015. Ecological restoration of an acidic Cd contaminated soil using bamboo biochar application. Ecol. Eng. 84:67–76. doi:10.1016/j.ecoleng.2015.07.009
- Nemati, K., N.K. Abu Bakar, M.R. Abas, and E. Sobhanzadeh. 2011. Speciation of heavy metals by modified BCR sequential extraction procedure in different depths of sediments from Sungai Buloh, Selangor, Malaysia. J. Hazard. Mater. 192:402–10. doi:10.1016/j.jhazmat.2011.05.039
- Nemati, K., N.K. Abu Bakar, M.R. Abas, E. Sobhanzadeh, and K.H. Low. 2011. Comparison of unmodified and modified BCR sequential extraction schemes for the fractionation of heavy metals in shrimp aquaculture sludge from Selangor, Malaysia. Environ. Monit. Assess. 176:313–20. doi:10.1007/s10661-010-1584-3
- Olaniran, A.O., A. Balgobind, and B. Pillay. 2013. Bioavailability of heavy metals in soil: impact on microbial biodegradation of organic compounds and possible improvement strategies. Int. J. Mol. Sci. 14:10197–228. doi:10.3390/ijms140510197
- Pardo, T., R. Clemente, and M.P. Bernal. 2011. Effects of compost, pig slurry and lime on trace element solubility and toxicity in two soils differently affected by mining activities. Chemosphere 84:642–50. doi:10.1016/j.chemosphere.2011.03.037
- Pérez-Esteban, J., C. Escolástico, A. Masaguer, and A. Moliner. 2012. Effects of sheep and horse manure and pine bark amendments on metal distribution and chemical properties of contaminated mine soils. Eur. J. Soil Sci. 63:733–42. doi:10.1111/ejs.2012.63.issue-5
- Petersell, V., U. Kirso, E. Steinnes, and N. Irha. 2009. Mobility of Cd, Pb, Cu, and Cr in some Estonian soil types. Estonian J. Earth Sci. 58:209. doi:10.3176/earth.2009.3.05
- Shuman, L.M., S. Dudka, and K. Das. 2007. Cadmium forms and plant availability in compost-amended soil. Commun. Soil Sci. Plant Anal. 33:737–48. doi:10.1081/CSS-120003062
- Tokalioglu, S., V. Yilmaz, and S. Kartal. 2009. Solid phase extraction of Cu(II), Ni(II), Pb(II), Cd(II) and Mn(II) ions with 1-(2-thiazolylazo)-2-naphthol loaded Amberlite XAD-1180. Environ. Monit. Assess. 152:369–77. doi:10.1007/s10661-008-0322-6
- Tripathy, S., P. Bhattacharyya, S.M. Equeenuddin, K. Kim, and H.D. Kulkarni. 2008. Comparison of microbial indicators under two water regimes in a soil amended with combined paper mill sludge and decomposed cow manure. Chemosphere 71:168–75. doi:10.1016/j.chemosphere.2007.10.042
- Wang, J., and C. Chen. 2015. The current status of heavy metal pollution and treatment technology development in China. Environ. Technol. Rev. 4:39–53. doi:10.1080/21622515.2015.1051136
- Xu, J., A.G. Bravo, A. Lagerkvist, S. Bertilsson, R. Sjoblom, and J. Kumpiene. 2015. Sources and remediation techniques for mercury contaminated soil. Environ. Int. 74:42–53. doi:10.1016/j.envint.2014.09.007
- Yang, L., Z. Chen, T. Liu, J. Jiang, B. Li, S. Chen, and J. Zhang. 2013. Soil respiratory and enzyme activities in leachate-contaminated soils with different application rate of cow manure compost: A laboratory study. Environ. Earth Sci. 71:225–31. doi:10.1007/s12665-013-2426-8
- Zeng, F., S. Ali, H. Zhang, Y. Ouyang, B. Qiu, F. Wu, and G. Zhang. 2011. The influence of pH and organic matter content in paddy soil on heavy metal availability and their uptake by rice plants. Environ. Pollut. 159:84–91. doi:10.1016/j.envpol.2010.09.019