ABSTRACT
Composting of agricultural wastes not only can reduce environmental pollution caused by improper disposal, but also can recycle agricultural wastes and transform them into highly valuable products, such as fertilizers or soil conditioners, for agricultural applications. However, the composting process and final product are easily affected by the limited oxygen supply that results from insufficient aeration, especially in the center of a large-scale windrow. Hence, a pilot-scale experiment was conducted to investigate the effects of the turning frequency on the composting efficiency and compost quality of used pig manure and fungus residue. Physical and chemical characteristics were measured over the course of 63 days of composting. The data indicate that higher temperatures and more rapid moisture removal generally result from a turning treatment of once every 2–4 days than in fewer, or no, turning treatments. The total nitrogen, total phosphorus, and total potassium contents increased in all windrows as the organic matter content decreased, but both the increases and decrease were greater in windrows that were turned more frequently. The reduction of the organic matter mass by 53.7–66.0% for a turning of once every 2–8 days is significantly higher than that for the static windrow (39.1%). Although there is an increase in nitrogen mass loss with an increased turning frequency, lower nitrogen mass losses (12.7–25.7%) in all treatments were noted compared with previous studies. A final compost product with less moisture, less weight, higher nutrient content (N, P, and K), and greater stability was obtained in windrows with turning frequencies of once every 2–4 days, which is recommended when composting pig manure and fungus residue.
Implications: Composting of agricultural wastes not only can reduce environmental pollution caused by improper disposal, but recycling of agricultural wastes transforms them into highly valuable products, such as fertilizers or soil conditioners, for agricultural applications. However, the composting process and final product are easily affected by the limited oxygen supply that results from insufficient aeration, especially in the center of a large-scale windrow. Hence, a pilot-scale experiment was conducted to investigate the effects of the turning frequency on the composting efficiency and compost quality of used pig manure and fungus residue, so as to capture an operational technique suitable for the effective co-composting pig manure and edible fungi residue for a large-scale composting plant.
Introduction
Organic solid waste, such as livestock manure, agricultural residues, fungus residue, and municipal wastes, has become a major environmental and social problem on the global scale (Rashad et al., Citation2010). In China, annual production of pig manure and fungus residue is estimated at 776 and 4.57 million tons (Yang et al., Citation2010; Wei et al., Citation2010), respectively, a fact that causes a great environmental challenge in terms of effective waste management. It is generally acknowledged that pig manure and fungus residue, with to their high organic matter content and availability of essential plant nutrients (e.g., N, P, and K), are valuable materials for improving the soil structure in tilled soils, for increasing the nutrient levels of soils, and for improving crop yields and quality (Guo et al., Citation2012; Jordan et al., Citation2008; Zhang and Sun, Citation2014). However, fresh pig manure and fungus residue have limited direct agricultural use because of pathogens, parasites, unstable nutrients, and transportation and preservation difficulties (Guo et al., Citation2012; Ogunwande et al., Citation2008a). Furthermore, fungus residue, which is connected in a compact bar shape, is more unsuitable for application to an agricultural region prior to disposal. Therefore, it is essential to find environmentally sound and economically feasible technologies for both recycling these available resources and minimizing their adverse impacts on the environment. Composting is an environmentally friendly and effective method for treatment of agricultural waste (especially for livestock manure) and has been applied with increasing popularity by farmers around the world. Composting also converts organic wastes into a stable final product that is not phytotoxic and is free of pathogens, to be used as organic fertilizers for plant growth or soil improvement (Ogunwande et al., Citation2008a; Bernal et al., Citation2009). An additional benefit to farmers is from profitable markets when composting products have been sold off as organic fertilizers.
Composting is commonly described as the aerobic degradation of organic wastes, where heat is released by oxygen-consuming microbial metabolism, resulting in increased temperature. Therefore, the aerobic condition during the composting process is a critical factor influencing successful composting, and a limited oxygen supply in composting materials has been found to lead to lower temperatures and reduced microbial activities (Kalamdhad and Kazmi, Citation2009; Kalemelawa et al., Citation2012). In practice, the oxygen in composting windrow interiors at large-scale composting plants is generally supplied by turning the windrow periodically, especially for large windrows. Previous studies demonstrate that composting pig manure with turning can enhance degradation rates of organic matter, reduce emissions of N2O and CH4, improve the quality of the final compost, and reduce the health risks for personnel working at composting sites by reducing Aspergillus fumigatus on the compost surface (Szanto et al., Citation2007; Chiumenti et al., Citation2007; Illmer and Schinner, Citation1997; Fischer et al., Citation1998). However, excessive turning frequency, especially in the early stage of composting, will lead to adverse effects, such as increased nutrient losses (ammonia volatilization), increased costs, and a diminished composting process (Cook et al., Citation2015; Guo et al., Citation2012; Parkinson et al., Citation2004; Ei Kader et al. Citation2007; Tirado and Michel, Citation2010). Conversely, an insufficient turning frequency can lead to anaerobic conditions caused by the lack of oxygen (Chiumenti et al., Citation2007), increased greenhouse gas emissions (such as N2O and CH4), production of malodor (H2S), prolonging the composting duration, and decreasing the quality of the compost (Guo et al., Citation2012; Szanto et al., Citation2007; Getahun et al., Citation2012; Yuan et al., Citation2015; Cook et al., Citation2015). Hence it is necessary to optimize the turning frequency, which will aid in controlling environmental pollution and in retaining N, while having minimal effects on the rate of compost production (Parkinson et al., Citation2004).
However, there are large previous studies of successful pig manure composting mainly using sawdusts, rice brans, and cornstalks as carbon source to mix with pig manure, and rare literature reportes of the co-composting with pig manure and fungus residue, except estimated ratios of pig manure to fungi residue in composting with pig manure and fungus residue (Zhou et al., Citation2016). In recent years, co-composting has been used commonly in China. The term “co-composting” refers to the simultaneous composting of several types of organic wastes that can improve the compost quality by the comprehensive use of diversified waste properties (Zhang and Sun, Citation2014). The period of composting can be shorter when two or more organic wastes are composted together, rather than separately (Das et al., Citation2011). Hence, the aims of this work are to investigate the effects of the turning frequency on the co-composting process of pig manure and fungus residues with rice bran (as a conditioning agent of carbon resources), as well as on the final compost properties, and to determine a strategy of generating an optimal turning frequency to increase efficiency and to improve the final product quality for large-scale farms.
Methods
Raw composting materials
Two tractor loads of pig manure weighing approximately 8400 kg, which were disposed of by means of liquid/solid separation, were collected from the Zhejiang TianPeng livestock industry limited company. Fungus residue, a by-product of enoki mushroom cultivating, which is rich in lignin due to its basic formulation consisting mainly of cottonseed hulls (approximately 85%) and wheat bran (approximately 10%), was obtained from the JiangShan GenGen edible fungi company. Rice bran, as an adjusting agent for the carbon source, was purchased from a rice processing plant near the experiment’s location. Selected physicochemical properties of the raw composting materials were measured before the start of the composting experiment (). The monitoring methods are described next.
Table 1. Main physicochemical properties of raw materials.
Composting setup and design
Before the start of the co-composting process, the fungus residues, which were compacted into a bar shape, were crushed by a hammer mill. Then all of the raw materials were mixed and homogenized via a large stirring machine, while the initial moisture content was adjusted to approximately 50–55% by water spraying. Subsequently, the mixture was divided into four parts that were used to form four windrows with triangle cross-section, with a bottom dimension of 1.5 m wide, 1.0 m high, and 4.8 m long. Windrows were placed on a concrete slab to prevent the dispersion of any leachate generated during the composting experiments. A schematic diagram of the windrows is shown in . Each treatment combination consisted of approximately 2100 kg pig manure, 500 kg fungus residues, and 600 kg rice bran. A previous study had concluded that this is the optimal mixing ratio that is suitable for efficient and quality composting (Zhou et al., Citation2016). Windrows were not duplicated because of the limitation of both the heavy work and a project budget and because the variability between the respective windrows was similar to that of duplicate samples of a single windrow (Tirado and Michel, Citation2010). The experiment was conducted for 63 days, from August 12 to October 13, 2015, in the workshop of the ZheJiang TianPeng livestock industry limited company. The moisture content of composts was monitored every 2 days throughout the process, with water sprayed onto materials as needed to maintain the appropriate moisture content during the early-stage mixture-homogenizing process (water was added on days 4, 8, 12, and 18). Four turning frequencies were applied to windrows: one treatment with no turning (TF0) as the control, and three treatments turned manually every 2 days (TF2), every 4 days (TF4), and every 8 days (TF8).
During the composting process, the ambient temperature and the temperature within each windrow at a depth of 0.4 m from the top were measured daily with a digital thermometer probe (±0.1°C) before turning. The temperatures were measured between the hours of 8:00 and 10:00 a.m., when the ambient temperature was fairly stable. Each reading represents the average of five different locations in a compost windrow.
On-site sampling and monitoring
One sample of each windrow was taken every 2 days during the composting process by using a stainless-steel sampler that was 0.5 m long and 0.25 m in diameter (). Three replicate samples were taken at both the beginning and the end of composting. Five subsamples (200 g each) were collected from five random locations along the length of each windrow by a sampler inserted to a depth of 0.4 m from the surface of the windrow (). The five subsamples were mixed to make one composite sample per windrow. Each composite sample was divided into two parts. One part was directly used to determine the moisture content (MC) and pH value, and the other part was air-dried, crushed in a small grinder, passed through a 0.25-mm sieve, and used for the determination of the total Kjeldahl nitrogen (TN), ammonium-nitrogen (NH4-N), nitrate-nitrogen (NO3-N), electrical conductivity (EC), organic matter (OM), total phosphorus (TP), and potassium (TK). All analyses were carried out in duplicate, and values were reported as the average of the two results. To determine mass loss during composting, the total mass of each pile was determined at the start and at the conclusion of the experiment.
Analytical methods
The MC was determined by drying 10 g of fresh samples at 105°C to a constant weight. The pH values were measured with a pH digital meter (pH-3C, Shanghai, China) in aqueous extract (Zhang and Sun, Citation2014). The aqueous extract of each air-dried sample was prepared by shaking a 1:10 (w/v) mixture of the prepared sample and distilled water using a horizontal shaker for 1 hr (at 130 rpm), then filtering the mixture through a 0.45-µm membrane filter (Shen et al., Citation2015). NH4+-N and NO3–N were determined by distillation in an alkaline medium (MgO) followed by ultraviolet spectrophotometry according to Chinese national standard NY/T 1116-2014, while EC was detected by using a DDS-11A conductivity meter (LeiCi, Shanghai, China) on these water extracts. The TN (Kjeldahl digestion in sulfuric acid and hydrogen peroxide, UDK 169, VELP, Italy), OM (potassium dichromate and sulfuric acid oxidation method), TP (spectrophotometry, 722, NingBou, Nanjing, China) and TK (flame photometry, FP640, Precision Science, Shanghai, China) were measured according to the Chinese national standard (NY 525-2012). The masses of nutrients (TN, TP, TK) and of OM in the windrows were computed (nutrient or OM concentration × dry weight of the pile), and the losses were calculated as follows (Tiquia, Citation2002):
All values reported are on a dry weight (DM) basis, except the moisture content, which is reported on a wet weight basis.
Statistical analysis
One-way analyses of variance (ANOVAs) were used to determine whether the mass loss of various nutrients differed among the treatments with the statistical software DPS v 9.5 (Zhejiang University, Hangzhou, China). When ANOVAs were significant, means were separated with an LSD test (p < 0.05). The dynamics of the parameters in windrows with different turning frequencies during the composting period were plotted with Origin Pro 8.6 software (OriginLab, Northampton, MA).
Results and discussion
Changes in temperature during composting
Temperature is a mirror that reflects the metabolism of the microorganisms in composting mixtures, and affects both the metabolism of microorganisms and the process of composting (Zhu, Citation2006) . As summarized in , the temperatures rapidly increased in all treatments due to intense microbial activities to reach a peak (>55°C) after 8–9 days from the formation of the windrow. The strongly biological activity maybe closely related to the appropriate water content in compost materials, as mentioned later. The thermophilic phase (>50°C) lasted 54 days for TF0, 46 days for TF2, 43 days for TF4, and 34 days for TF8. These temperatures were sufficient for pathogen elimination. However, after the second addition of water (on day 9), the temperature of TF0 dropped sharply and then fluctuated within 50–55°C almost until the end, while the TF2, TF4, and TF8 temperatures rose again to above 55°C on days 13, 18, and 27, respectively, and for 32, 20, and 20 days, respectively, maintained an optimum range of 55 to 60°C, which was the most favorable temperature range for decomposition (Zhu, Citation2006). The highest temperature observed corresponded with the highest turning frequency through the degradation process. When the easily degradable material of the windrow had been depleted, the temperatures of all treatments started to slowly approach the ambient temperature. This occurred earlier in the turning treatments than in the control. Throughout the degradation process, the lower observed temperatures corresponded with less frequent turning, probably because of reduced microbial activities caused by the insufficient oxygen supply in static or less frequent turning (Chiumenti et al., Citation2007; Sundberg, Citation2005), which resulted in reducing the decomposition of organic matter and prolonging the composting times.
Changes in pH, EC, and moisture
The pH of the four treatments remained from 7.4 to 8.4 during the composting process (), which was within an optimum range of 6.5 to 8.0 for composting as reported by Vakili et al. (Citation2012). The pH of all treatments increased slightly over the first 4 days, then declined quickly to the original value during the period of water addition. This may have been caused by a small amount of ammonia that was generated by the mineralization of organic matter, while on the other hand, the organic acid produced due to temporary anaerobic conditions that resulted from the addition of water was sufficient to promote a pH drop (Shen et al., Citation2011). Notably, the pH of TF0 dropped to the lowest value among the 4 treatments. Then, the pH in all four treatments increased again and fluctuated between 8.0 and 8.4 until the composting concluded. The results indicate that the pH is not significantly affected (p > 0.05) by the turning frequency during co-composting of pig manure and fungus residue, possibly because of rapid volatilizing of NH3 due to good porosity in the piles. Zhang and Sun (2010) had reported that fungus residue is an effective bulking agent with a low bulk density, which improved materials’ permeability in compost. The EC in all four windrows increased from 1770 to 1910 µS/cm to 1950 to 2280 µS/cm during composting (), which was likely due to chemicals being transformed into more soluble inorganic salt forms during organic matter decomposition and due to the concentration effect resulting from the net loss of dry mass (Kalemelawa et al., Citation2012). However, the EC of TF0 was significantly (p < 0.05) lower than that for the other treatments, while those of other treatments were not significantly (p > 0.05) different from each other. During the entire composting process, the EC values in four treatments were well below the upper limit of 3000 µS/cm, which is considered to be not toxic to plants (Soumarè et al. Citation2002). The water strongly affects the biological activity that converts composting materials into mature and stable compost. Liang et al. (Citation2003) believed that its effect was more influential than that of temperature. In aerobic composting, it is essential to obtain optimal range of MCs in composting materials, which contributes substantially to the high rates of decomposition achieved during processing. showed that MC in all treatments slightly increased from approximately 50% to 52.5–57.0% during the initial 20 days after the addition of the water, and these values were within optimal range of 50–60% reported by Tiquia et al. (Citation1996); then they decreased gradually until the end of composting due to water volatilization caused by aeration and high temperatures. However, the water loss in TF2 was highest among the four treatments, which suggests that an excessive turning frequency accelerates water removal. This finding is in agreement with the results reported by Szanto et al. (Citation2007).
Changes in nutrient contents
Knowledge of the mineral nutrient content of compost is of prime importance, as it varies widely and is an important determinant of the end use for compost (Kalemelawa et al., Citation2012). The changes in the primary nutrient contents (TN, NH4+-N, NO3–N, TP, and TK) during the composting process are shown in .
Figure 4. Changes in the contents of TN (a), TP (b), TK (c), NH4+-N (d), and NO3–N (e) in the compost piles with different turning frequencies.
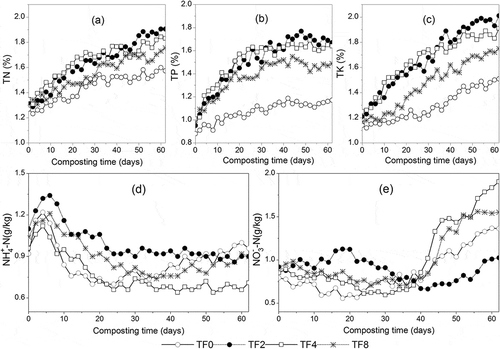
Nitrogen
shows that the TN contents in all of the treatments increased gradually during the entire composting process, due to the net loss of dry mass by conversion to carbon dioxide and to water loss by evaporation caused by heat that evolved during the oxidization of organic matter (Kalamdhad and Kazmi, Citation2009). At the end of composting, the TN contents in TF0, TF2, TF4, and TF8 were 1.57, 2.00, 1.76, and 1.81%, respectively, representing an increase of 28.9, 51.2, 34.3, and 34.3%, respectively, compared to the initial TN contents. Regarding a comparison among the four treatments, an increase in TN was associated with an increase in TF, indicating that the turning frequency has a signification effect (p < 0.05) on the TN content during the composting period. Similar results have been reported in previous studies (Vakili et al., Citation2012; Cook et al., Citation2015). The changes in the concentration of the two inorganic forms of nitrogen, NH4+-N and NO3–N, followed the typical trends in all four treatments with composting time ( and ). The accumulation of NH4+-N in all treatments increased rapidly to its peak (1.21–1.34 g/kg) over the first few days and then gradually decreased to 0.71–0.96 g/kg toward the end of composting. The initial rise was a result of the thermophilic decomposition of organic forms of nitrogen, such as proteins and amino acids, into ammonia (Kalemelawa et al., Citation2012). However, the decline in the latter part of the experiment was not consistent with the increase in the NO3–N concentration, which suggests that the majority of NH4+-N might have been lost through NH3 volatilization and/or microbial denitrification. The differences in the decrease of the NH4+-N contents (13.5–26.0%) were observed in treatments with different turning frequencies, except for TF0, where this increased by 4.4%. TF4 had the greatest decrease, possibly attributable to higher pH during the composting process (). NO3–N changes exhibited a clear trend only under aerobic composting conditions (). During the first 40 days, the NO3–N concentrations in all four treatments did not increase. This was then followed by a rapid increase that reached a maximum of 1.362 g/kg for TF0, 1.105 g/kg for TF2, 1.905 g/kg for TF4, and 1.553 g/kg for TF8 at the end of experiment, representing an increase of 72.0, 20.2, 123.6, and 69.8%, respectively, compared to the initial contents. The low NO3–N accumulation in the preliminary stage was mainly caused by the high temperature, which could have inhibited nitrification through microbial inactivation (Kalamdhad and Kazmi, Citation2009). Among the different treatments, the increased rate of NO3–N in TF4 was significantly (p < 0.05) higher than that of the others, which is coincident with the early decline of temperature in the latter stage.
Phosphorus and potassium
Like the change in the TN content, TP and TK contents display an upward tendency over composting time ( and ). The increases in TP and TK result from the concentration effect arising from a higher rate of carbon loss that occurs when organic matter has been decomposed or mineralized into CO2 or CH4 (Guo et al., Citation2012). The final TP and TK contents in compost with turning (TF2, TF4, and TF8) were significantly higher than those of TF0, but with no significant difference between the treatments with various turning frequencies. This indicates that higher microbial activities exist in turning treatments, resulting in increased mineralization. However, compared with the initial values, the final TP and TK contents increased by 33.0–75.8% and 32.5–66.1%, respectively.
Changes in organic matter content and in C/N ratios
Organic matter mineralized during composting, mostly due to the degradation of easily degradable compounds, such as proteins, cellulose, and hemicellulose, which are utilized by microorganisms as C and N sources (Kalamdhad and Kazmi, Citation2009). The data in indicate that the OM content in all treatments declined continually for 63 days, likely due to the loss of carbon as CO2 during composting. The statistical analysis reveals that the turning frequency had a significant (p < 0.05) effect on the degradation of OM. The highest decrease rate was observed for TF2 (34.4%), while the lowest rate was for TF0 (12.5%). This may be attributed to the increased oxygen provided by turning the windrow and by higher temperature () that enhanced microbial activity. An improved degradation of OM in compost with an increasing turning frequency was also reported by Ogunwande et al. (Citation2008b) and Getahun et al. (Citation2012).
Figure 5. Changes in the OM content and C/N ratios of the compost piles with different turning frequencies.
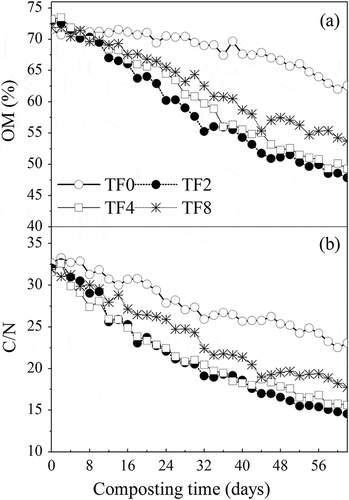
In this study, all treatments had an initial C/N ratio of approximately 32 (), which is an optimal value within the range of 25 to 35 for composting proposed by Bernal et al. (Citation2009). During the composting period of 63 days, the C/N ratios in all four treatments steadily declined to reach final values of 23.1 for TF0, 14.6 for TF2, 15.6 for TF4, and 17.6 for TF8. This decline is due to the mineralization of the substantial OM content () and increase in the TN content (). However, the C/N ratio was also significantly affected (p < 0.05) by the turning frequency. At the end of the experiment, the C/N ratio in all treatments with turning was less than 20, which indicates the achievement of a stable and mature compost (Huang et al., Citation2004).
Mass balance
It is widely known that aerobic composting is a process of waste reduction by multiform gaseous volatilization (such as of CO2, NH3, H2O, and N2O) and/or leaching losses (Shen et al., Citation2011; Ogunwande et al., Citation2008b; Parkinson et al., Citation2004). Thus, the absolute quantities of the various components continuously declined during the composting. presents the quantities of the materials at the beginning and at the end of the process, as reflected by the dry mass, OM, TN, TP, and TK. The results reveal that the dry mass in all four treatments declined from an initial weight of 1322.5 kg to 681.9 to 932.9 kg, a decrease of 29.5 to 48.4% compared to the initial weight. The loss of dry mass was evidently higher with more turning treatment than that with less or no turning. A substantial loss of OM in all treatments (38.3–66.2%) clearly indicates that this was a very successful composting study. The greatest OM loss was observed in TF2, while the least OM loss was in TF0 because, as previously mentioned, the turning frequency had a significant effect (p < 0.05) on OM degradation. The loss of TN followed a pattern similar to that of OM. Between 12.8% and 25.8% of the initial TN of material had been lost during the composting period. The greater TN loss in treatments with a higher turning frequency is probably due to turning, which stimulates aerobic decomposition processes and leads to elevated emission of NH3 (Ei Kader et al. Citation2007; Ogunwande et al., Citation2008b; Chiumenti et al., Citation2007; Kalamdhad and Kazmi, Citation2009). Statistical analysis indicated that the N loss had significantly positive correlation with turning frequency (R = 0.9624). However, the TN losses measured in this study were lower than those of other studies (Ogunwande et al., Citation2008a; Parkinson et al., Citation2004). This may be closely related to the lower pH (below 8.5) during the entire composting process (). Liu et al. (Citation2015) noted that NH3 emissions increased sharply, while the pH was more than 8.5 in composting livestock manure. In addition, the higher initial C/N ratio () favors decreased NH3 volatilization (Bernal et al. Citation2009; Gao et al., Citation2010). This helps to reduce the risk of potential environmental degradation by ammonium, a factor contributing to acidification (Parkinson et al., Citation2004), and helps to improve the value and use of the compost as a fertilizer in agricultural applications. Phosphorus and potassium element losses were both low in this study (), and the losses observed in TF2 and TF4 were slightly higher than those of TF0 and TF8. The somewhat higher loss may be attributed to the increased turning frequency. A similar finding has been reported by Parkinson et al. (Citation2004). Phosphorus and potassium retention in compost minimizes the risk of water pollution that may be caused by excess phosphorus losses to surface water and groundwater directly.
Table 2. Dry mass, OM, and nutrient mass losses during composting (means ± SD; n = 3).
Conclusion
The effects of the turning frequency on co-composting of pig manure and fungus residue were determined. The results indicate that the turning frequencies used in this study had significant impacts on temperatures, moisture contents, decomposition of organic matter, and nitrogen mass losses. As the turning frequency increased, so did the heating rate and the organic matter degradation rate, leading to a shorter composting period. However, the nitrogen mass loss via ammonia emission began to rise significantly. Therefore, the management of windrow turning should take into account the composting efficiency as well as nitrogen conservation when composting pig manure and fungus residue.
Acknowledgment
The author expresses heartfelt thanks to Zhejiang Tian Peng Livestock Co., LTD, for providing the testing ground, and for the large amount of work performed by technicians for investigation and analysis.
Funding
This study was supported by a project (2015C32123) funded by the Science and Technology Department of Zhejiang Province.
Additional information
Funding
Notes on contributors
Zhou Jiang-ming
Zhou Jiang-ming is an agricultural technician (associate professor) at the Agricultural Technique Popularization Centre of Jiangshan City, Jiangshan, Zhejiang, People’s Republic of China.
References
- Bernal, M.P., J.A. Alburquerque, and R. Moral. 2009. Composting of animal manures and chemical criteria for compost maturity assessment. A review. Bioresource Technol. 100:5444–53. doi:10.1016/j.biortech.2008.11.027.
- Chiumenti, A., F. Da Borso, T. Rodar, and R. Chiumenti. 2007. Swine manure composting by means of experimental turning equipment. Waste Manage. 27:1774–82. doi:10.1016/j.wasman.2006.10.017.
- Cook, K.L., E.L. Ritchey, J.H. Loughrin, M. Haley, K.R. Sistant, and C.H. Bolster. 2015. Effect of turning frequency and season on composting material from swine high-rise facilities. Waste Manage. 39:86–95. doi:10.1016/j.wasman.2015.02.019.
- Das, M., H.S. Uppal, R. Singh, S. Beri, K.S. Mohan, V.C. Gupta, and A. Adholeya. 2011. Co-composting of physic nut (Jatropha curcas) deoiled cake with rice straw and different animal dung. Bioresource Technol. 102:6541–46. doi:10.1016/j.biortech.2011.03.058.
- Ei Kader, N.A., P. Robin, J.M. Paillat, and P. Leterme. 2007. Turning, compacting and the addition of water as factors affecting gaseous emissions in farm manure composting. Bioresource Technol. 98:2619–28. doi:10.1016/j.biortech.2006.07.035.
- Gao, M.C., F.Y. Liang, A. Yu, B. Li, and L.J. Yang. 2010. Evaluation of stability and maturity during forced-aerotion composting of chicken manure and sawdust at different C/N ratios. Chemosphere 78:614–19. doi:10.1016/j.chemosphere.2009.10.056.
- Getahun, T., A. Nigusie, T. Entele, T. Van Gerven, and B. Van der Bruggen. 2012. Effect of turning frequencies on composting biodegradable municipal solid waste quality. Resources Conserv. Recycling 65:79–84. doi:10.1016/j.resconrec.2012.05.007.
- Guo, R., G.X. Li, T. Jiang, F. Schuchardt, T.B. Chen, Y.Q. Zhao, and Y.J. Shen. 2012. Effect of aeration rate, C/N ratio and moisture content on the stability and maturity of compost. Bioesource Technol. 112:171–78. doi:10.1016/j.biortech.2012.02.099.
- Huang, G.F., J.W.C. Wong, Q.T. Wu, and B.B. Nagar. 2004. Effect of C/N on composting of pig manure with sawdust. Waste Manage. 24(8): 805–13. doi:10.1016/j.wasman.2004.03.011.
- Fischer, J.L., T. Beffa, P.F. Lyon, and M. Aragno. 1998. Aspergillus fumigatus in windrow composting: Effect of turning frequency. Waste Manage. Res. 16(4): 320–29. doi:10.1177/0734242X9801600404.
- Jordan, S.N., G.J. Mullen, and M.C. Murphy. 2008. Composition variability of spent mushroom compost in Ireland. Bioresource Technol. 99:411–18. doi:10.1016/j.biortech.2006.12.012.
- Kalamdhad, A.S., and A.A. Kazmi. 2009. Effects of turning frequency on compost stability and some chemical characteristics in a rotary drum composter. Chemosphere 74:1327–34. doi:10.1016/j.chemosphere.2008.11.058.
- Kalemelawa, F., E. Nishihara, T. Endo, Z. Ahmad, R. Yeasmin, M.M. Tenywa, and S. Yamamoto. 2012. An evaluation of aerobic and anaerobic composting of banana peels treated with different inoculums for soil nutrient replenishment. Bioresource Technol. 126:375–82. doi:10.1016/j.biortech.2012.04.030.
- Liang, C., K.C. Das, and R.W. McClendon. 2003. The influence of temperature and moisture contents regimes on the aerobic microbial activity of a biosolids composting blend. Bioresource Technol. 86:131–37. doi:10.1016/S0960-8524(02)00153-0.
- Liu, L., H.M. Kong, B.B. Lu, J.B. Wang, Y. Xie, and P. Fang. 2015. The use of concentrated monosodium glutamate wastewater as a conditioning agent for adjusting acidity and minimizing ammonia volatilization in livestock manure composting. J. Environ. Manage. 161, 131–136. doi: 10.1016/j.jenvman.2015.06.029.
- Vakili, M., A.A.M. Haque, and Z. Gholami. 2012. Effect of manual turning frequency on physic-chemical parameters during the oil palm frond and cow dung composting. Caspian J. Appl. Sci. Res. 1(12): 49–59.
- Ogunwande, G.A., J.A. Osunade, K.O. Adekalu, and L.A.O. Ogunjimi. 2008a. Nitrogen loss in chicken litter compost as affected by carbon to nitrogen ratio and turning frequency. Bioresource Technol. 99:7495–503. doi:10.1016/j.biortech.2008.02.020.
- Ogunwande, G.A., L.A.O. Ogunjimi, and J.O. Hafiyebi. 2008b. Effects of turning frequency on composting of chicken litter in turned windrow piles. Int. Agrophys. 22:159–65.
- Parkinson, R., P. Gibbs, S. Burchett, and T. Misselbrook. 2004. Effect of turning regime and seasonal weather conditions on nitrogen and phosphorus losses during aerobic composting of cattle manure. Bioresource Technol. 91, 171–178. doi:10.1016/S0960-8524(03)00174-3.
- Illmer, P., and F. Schinner. 1997. Compost turning—A central factor for a rapid and high–quality degradation in household composting. Bioresource Technol. 59:157–62. doi:10.1016/S0960-8524(96)00156-3.
- Rashad, F.M., W.D. Saleh, and M.A. Moselhy. 2010. Bioconversion of rice straw and certain agro-industrial wastes to amendments for organic farming systems: 1. Composting, quality, stability and maturity indices. Bioresource Technol. 101:5952–60. doi:10.1016/j.biortech.2010.02.103.
- Shen, D.S., Y.Q. Yang, H.L. Huang, L.F. Hu, and Y.Y. Long. 2015. Water state changes during composting of kitchen waste. Waste Manage. 38, 381–387. doi:10.1016/j.wasman.2015.01.011.
- Shen, Y.J., L.M. Ren, G.X. Li, T.B. Chen, and R. Guo. 2011. Influence of aeration on CH4, N2O and NH3 emissions during aerobic composting of a chicken manure and high C/N waste mixture. Waste Manage. 31, 33–38. doi: 10.1016/j.wasman.2010.08.019.
- Soumarè, M., A. Demeyer, and F.M.G. Tack. 2002. Chemical characteristics of Malian and Belgian solid waste composts. Bioresource Technol. 81:97–101. doi: 10.1016/S0960-8524(01)00125-0
- Sundberg, C. 2005. Improving Compost Process Efficiency by Controlling Aeration, Temperature and pH. Uppsala, Sweden: Swedish University of Agricultural Sciences.
- Szanto, G.L., H.M. Hamelers, W.H. Rulkens, and A.H.M. Veeken. 2007. NH3, N2O and CH4 emissions during passively aerated composting of straw-rich pig manure. Bioresource Technol. 98, 2659–2670. doi:10.1016/j.biortech.2006.09.021.
- Tirado, S.M., and F.C. Michel, Jr. 2010. Effects of turning frequency, windrow size and season on the production of dairy manure/sawdust composts. Compost Sci. Utiliz. 18(2): 70–80. doi:10.1080/1065657X.2010.10736938.
- Tiquia, S.M., N.F.Y. Tam, and I.J. Hodgkiss. 1996. Microbial activities during composting of spent pig-manure sawdust litter at different moisture contents. Bioresource Technol. 55:201–6. doi:10.1016/0960-8524(95)00195-6.
- Tiquia, S.M., T.L. Richard, and M.S. Honeyman. 2002. Carbon, nutrient, and mass loss during composting. Nutrient Cycling Agroecosyst. 62(1): 15–24. doi:10.1023/A:1015137922816.
- Wei, Z.T., G.Y. Zhou, and Q.X. Hu. 2010. The research progress and utilization of edible fungus residue. Edible Fungus China 29:3–6,11.
- Yang, F., R. Li, Y. Cui, and Y.H. Duan. 2010. Utilization and develop strategy of organic fertilizer resources in China. Soil Fertilizer Sci. Chin. 4:77–82. doi:10.3969/j.issn.1673-6257.2010.04.017.
- Yuan, J., Q.Y. Yang, Z.Y. Zhang, G.X. Li, W.H. Luo, and D.F. Zhang. 2015. Use of additive and pretreatment to control odors in municipal kitchen waste during aerobic composting. J. Environ. Sci. 37:83–90. doi:10.1016/j.jes.2015.03.028.
- Zhang, L., and X.Y. Sun. 2014. Changes in physical, chemical and microbiological properties during the two-stage co-composting of green waste with spent mushroom compost and biochar. Bioresource Technol. 171, 274–284. doi:10.1016/j.biortech.2014.08.079.
- Zhou, J.M., L.T. Wang, H.M. Wang, L. Jiang, and X.Y. Jiang. 2016. Effects of different ratios of pig manure to fungus residue on physicochemical parameters during composting. J. Air Waste Manage. Assoc. 66(5): 499–507. doi:10.1080/10962247.2016.1149526.
- Zhu, N.W. 2006. Composting of high moisture content swine manure with corncob in a pilot-scale aerated static bin system. Bioresource Technol. 97:1870–75. doi:10.1016/j.biortech.2005.08.011.