ABSTRACT
Nitrous acid (HONO) is an important precursor of OH radicals in the atmosphere. In urban areas, emissions from vehicles are the main source of air pollutants, including reactive nitrogen. Previously reported emission ratios of HONO (HONO/NOx) from vehicles were measured in the late 1990s and need to be updated due to the significant changes in emission control technologies. We measured the emission ratio of a fleet of vehicles (38% diesel on average) from March 11 to 21, 2015, in a road tunnel in Hong Kong. The emission ratio of 1.24% (±0.35%) obtained is greater than the commonly adopted 0.8% or 0.3%. The elevated emission ratio is found to be related to the presence of vehicles equipped with diesel particle filters (DPFs). Positive correlation between HONO and black carbon (BC) shows that HONO and BC were emitted together, while the lack of correlation or even anticorrelation between HONO/NOx and BC indicates that the BC-mediated conversion of NO2 to HONO in the dark was insignificant in the immediate vicinity of the emission sources.
Implications: Vehicular emission is a key source for HONO in the urban atmosphere. However, the most commonly used emission ratio HONO/NOx in modeling studies was measured more than 15 years ago. Our tunnel study suggests that a mixed fleet nowadays has a higher emission ratio, possibly because of the diesel particle filter (DPF) retrofit program and the growing share of Euro IV or more advanced diesel vehicles. Our study also provides new insight into the role of black carbon in HONO formation from vehicles.
Introduction
Nitrous acid (HONO) is an important reservoir of radicals in the atmosphere. Upon its photolysis at wavelengths smaller than 390 nm, hydroxyl radical (OH), which acts as an important cleansing agent in the atmosphere and promotes the formation of photo-oxidants such as ozone and peroxyacetylnitrates (PANs), will be produced (Kleffmann, Citation2007). The mechanisms by which HONO accumulates in the atmosphere are still not well understood. The homogeneous reaction between NO and OH alone cannot fully explain the high concentration (up to ppbv level) of daytime HONO present in the atmosphere (e.g., Kleffmann, Citation2007). For primary HONO emitted by vehicles, the emission ratio ERHONO = HONO/NOx, in which NOx = NO + NO2, is the most commonly used parameter for quantifying its strength. Previous measurement studies showed the emission ratios fell between 0.3% and 0.8% (Pitts et al., Citation1984; Sjödin and Ferm, Citation1986; Kirchstetter et al., Citation1996; Kurtenbach et al., Citation2001). Since these values are significantly lower than HONO/NOx observed in the atmosphere, a number of secondary HONO sources were proposed (Spataro and Ianniello, Citation2014). Although incorporating these sources into models can improve the simulation of HONO (e.g., Wong et al., Citation2013), the uncertainties in quantifying these sources and inability of the models to simulate certain cases necessitate a reevaluation of the traditional sources, in addition to the search for new emission and formation pathways.
Emission ratios (ERs) from vehicles (HONO/NOx) can be determined in several ways, such as dynamometer tests, roadside measurements, and tunnel measurements. The tunnel measurement has advantages of being able to capture a large enough sample of on-road vehicles and not be influenced by chemical processes triggered by sunlight (Ropkins et al., Citation2009). Kirchstetter et al. (Citation1996) measured HONO using denuders in a road tunnel in California (with more than 99% gasoline vehicles), and derived an ER of 0.3%. Kurtenbach et al. (Citation2001) used differential optical absorption spectroscopy (DOAS) to measure HONO in a tunnel in Germany with 24.3% share of diesel vehicles in traffic, which yielded an ER of 0.8%. These two ratios, although obtained in an era with only relatively primitive emission control technologies, have been used in many recent modeling studies. For instance, in the MOVES model for traffic emission used by the U.S. Environmental Protection Agency (EPA), the 0.8% ratio was assigned to all the on-road vehicles throughout the day (Rappenglück et al., Citation2013). The low emission ratios made researchers exclude traffic emission as an important source of HONO (Pusede et al., Citation2015). However, the vehicle fleets of in the two tunnels were both gasoline dominated, which may not reflect the composition of fleet today and elsewhere. In the last two decades, the heavy tax on gasoline has made diesel vehicles increasingly popular in the European countries. According to the European Automobile Manufacturers Association (available at: http://www.acea.be/statistics), the share of diesel in new passenger cars has grown from 23.1% in 1994 to 52.1% in 2015. It is commonly believed that diesel vehicles have a higher emission ratio of HONO because the semivolatile organics in diesel exhaust can enhance secondary HONO formation at tailpipe by reaction 1, where [C-H]red stands for the reductive functional groups on soot surfaces (Gutzwiller et al., Citation2002, Khalizov et al., Citation2010):
In addition, many developments of emission control technologies (especially exhaust after-treatment) have taken place since 2000. For example, diesel particle filters (DPFs) at tailpipes, which trap soot and remove them through oxidation, have drastically changed the composition of diesel exhaust. The soot removal in the DPFs is either achieved by active regeneration, in which fuel is injected to burn the carbon, or by passive regeneration, in which NO is catalytically oxidized to NO2 and the latter then oxidizes soot continuously (van Setten et al., Citation2001). Although the installation of DPFs can reduce the emission of particulate matter (PM) by more than 90% (Barone et al., Citation2010; Herner et al., Citation2011), it leads to a higher ratio of NO2/NOx at tailpipe (Dallmann et al., Citation2011), which may significantly increase the roadside oxidative capacity and promote ozone formation (Carslaw, Citation2005; Millstein and Harley, Citation2010). Installing DPFs may have affected HONO emission in two ways. First, in the commonly used passive filter regeneration process, a by-product of the NO2 + soot reaction is HONO (Gerecke et al., Citation1998, Khalizov et al., Citation2010). Although this reaction in the dark cannot explain the HONO/NOx ratio under atmospheric conditions due to the fast deactivation of limited reactive sites on the surface of black carbon, it may have noticeable impact on tailpipe HONO/NOx (Arens et al., Citation2001; Aubin and Abbatt, Citation2007). Second, the DPF-equipped vehicles can also cause an increment of secondary HONO formation in the atmosphere because NO2 is a direct precursor of HONO.
Despite the important role of vehicle-emitted HONO in urban atmosphere and the vast change of vehicle exhaust composition, there were only limited studies quantifying HONO emission ratio in the last 15 years. Rappenglück et al. (Citation2013) measured HONO and NOx at an urban highway junction in Houston, TX, in 2009, and found that during the morning peak (4 a.m. to 8 a.m. on weekdays), the buildup of HONO was linearly correlated with that of NOx with a slope 1.7% and R2 = 0.75. Wormhoudt et al. (Citation2015) used the data collected in the same year at a 70-m tower on the campus of University of Houston and derived a slope of 1.4% with R2 = 0.67. Xu et al. (Citation2015) analyzed 21 fresh emission plumes (with NO/NOx > 0.80) near an expressway with 33% diesel vehicles in Tung Chung, a suburban area in Hong Kong. The emission ratios were 0.5–1.6%, and such ratios were positively correlated with BC emitted.
Hong Kong is a highly urbanized city with heavy traffic density, suffering from severe roadside pollution problems. Although the diesel vehicles only account for 18% of vehicles registered, they contribute to more than 50% of NOx emitted by the road transport sector (HK Environment Bureau, Citation2013). In certain downtown areas, such as the two major roads at Mong Kok (an urban center), the diesel vehicles can exceed 35% of vehicles (Lee et al., Citation2015), closer to that in the European countries, leading to a greater dominance of diesel NOx. In Hong Kong, all the pre-Euro and Euro I diesel buses and most Euro II and Euro III buses had been retrofitted with passive regeneration DPF by 2010 and 2012, respectively (HK Environment Bureau, Citation2013; Heimark et al., Citation2009). For Euro IV or advanced diesel vehicles, DPF has become a common or even required configuration to meet the more stringent emission standards (Johnson, Citation2008; Tzamkiozis et al., Citation2010). These changes have led roadside NO2 to grow by 20% from 1999 to 2013 and made it the most frequent top pollutant in the three roadside air quality stations (HK Environment Bureau, Citation2013).
In this paper, we present the measurement result for HONO carried out in a busy road tunnel in Hong Kong. The objectives of this study are to measure the emission ratio of a modern fleet with a higher percentage of diesel vehicles, and to examine whether the new emission control technologies have impact on HONO. We also aim to evaluate the role of soot in HONO emission and formation. Our result can possibly inform the modelers dealing with HONO sources in urban areas with heavy diesel traffic, such as certain regions in Hong Kong and many European cities.
Methods
Field measurement site
The measurements were conducted at two points (A and B) in the north bore of Shing Mun Tunnel (1600 m in length, cross section area A = 70 m2), which carries one-way traffic through Needle Hill, connecting Tsuen Wan and Sha Tin, two densely populated districts in Hong Kong (). The tunnel has a 1% gradient from the entrance to the exit. The vehicle speed in the Shing Mun Tunnel was in the 60–70 km/hr range (Ho et al., Citation2009). As axial ventilation (induced by both the fans overhead and vehicle flow) from A to B was used in the tunnel, no air was lost in the middle of the two sampling points.
Measurement period and instruments
The measurement took place from March 11 to March 21, 2015. HONO was continuously measured by a commercial long-path absorption photometer (LOPAP) at point B. Detailed description of the LOPAP system can be found elsewhere (Heland et al., Citation2001; Zha et al., Citation2014; Xu et al., Citation2015). To fit the polluted environment, the upper range of our LOPAP was changed from ~2 ppbv to ~40 ppbv by reducing the sensitivity through adjusting the detecting wavelength from 550 nm to 610 nm (personal communication with Dr. J. Kleffman at Bergische Universität Wuppertal). The detection limit of this system was approximately 20 pptv with effective time resolution of ~5 min and accuracy of ±10%. Automatic zero checks with synthetic air were performed three times per day, and manual calibrations with 0.4 μg L−1 liquid standard were conducted on March 11, 12, 16, and 19. Time delay and response time of the LOPAP were corrected in data analysis each day. The data were averaged to a time resolution of 10 min for the following analysis.
NO and NO2 were simultaneously at both point A and point B, while BC and HONO were measured at point B. NOx was measured by an API-T200 chemiluminescence analyzer with a molybdenum converter with time resolution of 1 min. Our previous study has demonstrated that the thermal catalytic conversion gives NO2 results similar to those obtained from a more selective photolytic method when the sampling point is very close to the emission source (Xu et al., Citation2013); therefore, the data measured by the Mo oxide NOx analyzers in the present tunnel study were used without correction. BC was measured by an AE-51 aethalometer with time resolution of 10 sec. It is worth noting that the aethalometer, which measures attenuation of light through a filter where particles deposit, may report a lower than actual BC concentration especially when highly absorbing aerosols (like diesel soot) are present. Thus, eq 2 proposed by Kirchstetter and Novakov (Citation2007) was used for the correction of BC:
Here, BCraw is the raw concentration of black carbon reported by the aethalometer, and ATN refers to the light attenuation at the measurement wavelength of 880 nm in this instrument. Due to the malfunctioning of the aethalometer, BC data were only available for March 11–14.
Results and discussion
Traffic counting
shows the diurnal hourly vehicle flow during the measurement period. Each day 26,970 vehicles on average passed through the tunnel, with two apparent peaks in the morning and afternoon rush hours. We therefore define 6:00 a.m. to 11:59 p.m. as “emission-intensive hours,” during which the average hourly vehicle flow was larger than 1000 vehicles/hr. This time period also corresponds well with the hours with average wind velocity (in vehicle flow direction) higher than 3.6 m/sec, making the tunnel like a plug flow reactor. displays the composition of traffic fleet monitored on March 13 and March 14 during the emission-intensive hours. The share of diesel vehicles in the tunnel was 38%, around half of which were DPF-equipped (buses and Euro IV or above goods vehicles) in our estimation, using data from HK Environment Bureau (Citation2013).
Emission ratio
Two approaches have been used to determine the emission ratio of HONO based on measurements in a tunnel. Kurtenbach et al. (Citation2001) performed least-square linear regression of measured [HONO] versus [NOx], and reported the slope as the emission ratio. This approach might underestimate the emission ratio due to the very large intercept on the [HONO] axis (~10 ppbv). Kirchstetter et al. (Citation1996) calculated the emission ratio by
where Δ[HONO] and Δ[NOx] are the background-subtracted concentrations of HONO and NOx, respectively. Since the ambient concentrations of HONO and NOx were much lower than that in the tunnel, the authors found that HONO/NOx measured in the middle of the tunnel was nearly the same as ERHONO. In the present study, we choose the method by Kirchstetter et al. (Citation1996), which we believe is more appropriate for emission ratio calculation.
The mean concentrations of HONO and NOx measured at point B deep inside the tunnel were 15.7 (± 4.2) ppbv and 1.31 (± 0.32) ppmv, respectively, significantly higher than that those measured at the suburban Tung Chung site (mean HONO = 0.35 ppbv, mean NOx = 0.16 ppmv) in Hong Kong (Xu et al., Citation2015), which can be seen as a proxy for the background of the Shing Mun Tunnel. Therefore, we believe that HONO/NOx measured at point B can provide a reasonable representation of tailpipe emission ratio that is close to Δ[HONO]/Δ[NOx].
The diurnal pattern of HONO/NOx at point B is shown in . This ratio was highest in the period between midnight and dawn, when traffic flow was minimal, dropped quickly after the start of morning traffic, and remained stable from 7 a.m. to 11 p.m. (the emission-intensive hours). The high HONO/NOx ratios in the early hours are attributed to additional sources of HONO in incoming ambient air, apart from vehicle emissions. Therefore, the average HONO/NOx in the emission intense period, 1.24% (±0.35%), can be taken as the emission ratio of HONO. We also compute this emission ratio with an alternative approach. The 10-min averaged HONO (between 7 a.m. and 11 p.m.) was plotted against NOx in . To take account of measurement uncertainties in both HONO and NOx, the reduced major axis regression was used to determine the slope and intercept (Cantrell, Citation2008). The resulting slope 1.31% (standard error = 0.43%) is very close to the emission ratio we determined based on the ratio of concentrations, with the y-intercept close to 0. In the remaining analysis, we use the concentration ratio as the emission ratio. The emission ratio measured in this study is 50% higher than that reported in Kurtenbach et al. (Citation2001), but smaller than that measured at the highway junction in Houston (Rappenglück et al., Citation2013), despite the fact that in Shing Mun tunnel the share of diesel vehicles was more than three times higher compared with that junction.
Figure 3. (a) Hourly averaged HONO/NOx as a function of time of day. As an example, the data point at time of day = 7 shows HONO/NOx averaged over 7:00 a.m. to 8:00 a.m. Error bars on this plot show 1σ variability. (b) The 10-min average HONO for 7 a.m. and 11 p.m., plotted against NOx, RMA regression line is [HONO] = 0.0134[NOx] – 1.8169 (N = 791).
![Figure 3. (a) Hourly averaged HONO/NOx as a function of time of day. As an example, the data point at time of day = 7 shows HONO/NOx averaged over 7:00 a.m. to 8:00 a.m. Error bars on this plot show 1σ variability. (b) The 10-min average HONO for 7 a.m. and 11 p.m., plotted against NOx, RMA regression line is [HONO] = 0.0134[NOx] – 1.8169 (N = 791).](/cms/asset/afbe928f-0f3f-4f8f-ad30-8cac80d1d6a8/uawm_a_1293573_f0003_b.gif)
Nitrous acid can be produced by the uptake of NO2 on humid wall surfaces by reaction 4, which needs to be considered in ERHONO calculation. This reaction is first order to NO2 (Finlayson-Pitts et al., Citation2003):
The overall rate of this reaction can be calculated by
where is the mean speed of NO2 molecules, S/V is the surface to volume ratio, and γgeo is the uptake coefficient of NO2 on the wall surface. Kurtenbach et al. (Citation2001) measured γgeo ≈ 10−6 in a road tunnel with wall composition similar to that of Shing Mun Tunnel (both steel sheets covered by enamel). Using this value as NO2 uptake coefficient, and given that S/V equals 0.436 m−1 in the Shing Mun tunnel (estimated according to Ho et al., Citation2009), the pseudo-first-order rate constant k*het in eq 5 was found to be 1.31 × 10−3 min−1. The residence time of gases between the tunnel entrance and point B can be estimated as τ = Lentrance-to-B/vwind = 4.4 min (length from the entrance to B divided by average wind speed). Thus, it is reasonable to assume that NOx, after being emitted from vehicles, stayed half of τ, that is, 2.2 min on average in the tunnel before reaching point B. Substitute these parameters and [NO2]/[NOx] ≈ 0.15 as measured into eq 5, the increment of HONO/NOx via the heterogeneous reaction is estimated as 0.04%. This correction can be neglected because it is much smaller than the variation in HONO/NOx itself (σ = 0.35%).
The increase in HONO emission ratio can have a significant impact on the HONO mixing ratio and oxidative capacity in urban areas. For example, Czader et al. (Citation2015) compared two emission ratios, that is, 0.8% and 1.6%, in a WRF-CMAQ model for Houston. They found that in the increased emission ratio case, the modeled mean HONO level increased by 0.11 ppbv at the 70-m Moody Tower in Houston, which was 16% closer to the observed value. The increased HONO emission also led to a 14% increase of OH at that tower, and 3% if averaged over the whole urban area. Though the increment of emission ratio in our study is smaller, it may still have a noticeable impact on the oxidative capacity of urban atmosphere, especially in street canyons. This topic warrants further investigation.
Effect of diesel particle filter on HONO emission
To explore the effect of DPF installations on HONO emission, we attempt to isolate the measurements that were heavily affected by the emissions with DPF vehicles. Previous studies have shown that DPF-equipped vehicles emit NO2/NOx ratio exceeding 30% (Herner et al., Citation2009; Khalek et al., Citation2011; Bishop et al., Citation2012) and that for other vehicles, the ratio was smaller than 10% (Shorter et al., Citation2005). The emission ratio of NO2/NOx can be estimated by
where the subscripts A and B refers to the pollutant concentrations at A and B, respectively (Ban-Weiss et al., Citation2008). This method may not give very accurate emission ratio for NO2 due to the influence of the inflowing ambient ozone via reaction of O3 with NO (Yao et al., Citation2005), but it is reasonable for the current study because this ratio is only used as a criterion for selecting NO2-rich air parcels, and it is the increment of NO2 and NOx between A and B, not the NO2/NOx at a single point, that is utilized. displays mean HONO/NOx in different bins of ΔNO2/ΔNOx: 0–0.15, 0.15–0.20, 0.20–0.25, 0.25–0.3, 0.3 or above. The number of samples is 458, 254, 59, 10, and 8, respectively. An overall positive correlation between the two ratios indicates that the HONO emission ratios depend on ΔNO2/ΔNOx, with larger values toward the high NO2/NOx samples that we believe are indictors of DPF vehicles. For samples with ERNO2 > 0.25 (77 of out of the 789 data points), the average HONO emission ratio is 1.38% (±0.33%). A one-tailed z-test shows that the mean emission ratio of these cases is significantly larger than the population mean 1.24% (p < 0.001). Since the DPF-equipped vehicles emit lower BC but similar NOx compared with other diesel vehicles, it is possible that the reaction of NO2 with trapped BC took place inside the filter, giving larger HONO/NOx. This reaction is of negligible importance in the atmosphere due to limited surface area of BC (Arens et al., Citation2001; Aubin and Abbatt, Citation2007). However, as BC particles are oxidized layer by layer by NO2 during the regeneration of DPF, the heterogeneous reaction can be significant at the tailpipe. The mean BC concentration for the high NO2/NOx cases is even smaller than the population mean, suggesting that the higher HONO/NOx in the high ΔNO2/ΔNOx samples was probably not caused by the reaction occurring outside the tailpipe while in the tunnel.
HONO emission versus BC
To assess the role of BC in HONO emission and chemistry, we plotted HONO and NOx versus BC and HONO/NOx versus BC. The concentrations of HONO and NOx are positively correlated to BC (). However, higher BC was not accompanied by larger HONO/NOx. In fact, their correlation is almost negative, as shown by . Several factors may explain this phenomenon:
Diesel vehicles without DPF emit large amounts of BC, HONO, and NOx, whereas DPF vehicles, in which BC particles are trapped, would emit high NO2 and HONO, but low BC. The mixing of the two types of vehicles results in lacking overall correlation between the HONO emission ratios with BC.
Reaction 1 seemed to be too weak to alter the HONO/NOx ratio in the dark. The average amount of HONO generated per unit area by NO2 uptake on fresh soot was 8.2 × 1013 molecules cm−2 (Aubin and Abbatt, Citation2007). If we assume the uptake was limited by BC, and soot particles had a specific surface area of 106 cm2 g−1 (Aubin and Abbatt, Citation2007) and BC = 30 μg m−3 in the tunnel (approximately 80th percentile of BC as was measured), then only 0.1 ppbv HONO will be produced, which can be neglected given that the average concentration of HONO in the tunnel was 15.72 ppbv. In addition, DPF can change the surface chemistry of soot by removing the condensed polycyclic aromatic hydrocarbons (PAHs) or other aromatics (Heeb et al., Citation2008). As the aromatic groups are key players in NO2 to HONO conversion both in the dark and under sunlight (Monge et al., Citation2010; Han et al., Citation2013a; Citation2013b), the soot in the effluent of DPF may be even less reactive compared with normal diesel soot.
The sampling point was very close to the tailpipe, which means only very fresh BC was sampled. However, the heterogeneous reaction takes more than 10 min to induce an observable increase of HONO/NOx, according to uptake experiments (Han et al., Citation2013b). A previous study of our group reported positive correlation between HONO/NOx and BC based on measurements made at the top of a four-story building 80 m away from the expressway (Xu et al., Citation2015). It is possible that when air was relatively stagnant, it took 20–30 min for fresh plumes to reach the sampling point, and the conversion happened during the transport.
Figure 5. (a) Correlation of BC vs. NOx at point B from March 11 to March 14. (b) HONO vs. BC at point B from March 11 to March 14.
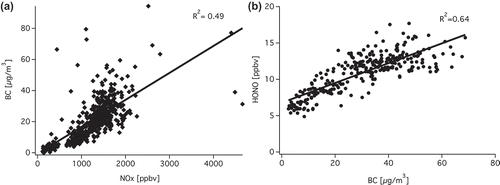
We therefore suggest that in the emission based models for HONO budget in urban areas, instead of using BC data to correct for HONO at the emission source, modelers could use precise emission ratio determined at source to account for primary HONO and treat such heterogeneous conversion in the atmospheric chemistry module, no matter in the dark or in sunlight.
Limitations of the current study
It is important to note that the HONO emission ratio from our study may only be applicable to regions having high share of diesel vehicles with moderate emission control technology, and for a vehicle fleet traveling at 60–70 km/hr with little acceleration. As the emission ratio of HONO is dependent on the engine load (Wood et al., Citation2008; Trinh et al., Citation2016), and engine load is dependent on acceleration and speed (Kean et al., Citation2003), the emission ratio in the congested urban area may be different from that determined in the present road tunnel. Also, the exact effect of the NO2 + soot reaction in DPF on HONO emission needs to be studied in controlled experiments.
Summary and conclusion
In this study HONO, NOx, and BC were continuously measured during March 11–21, 2015, in a road tunnel in Hong Kong. The emission ratio measured (1.24 ± 0.35%) was around 50% higher than the most commonly used 0.8%. Using ΔNO2/ΔNOx ratios as criteria to identify emission plumes from DPF-equipped vehicles, we found statistically significant increase of ERHONO from these vehicles. Positive correlation between HONO and black carbon (BC) suggests that HONO and BC were emitted together, while the lack of correlation between HONO/NOx and BC, which differs from our previous finding at the suburban Tung Chung site (Xu et al., Citation2015), suggests that the BC-mediated conversion of NO2 to HONO in the dark was insignificant immediately after emission. It is suggested that modeling studies handle the BC-mediated HONO formation in the atmospheric chemistry module rather than make correction at the emission source.
In view of the rapid development of emission control technologies nowadays, the emission ratio of HONO needs to be updated regularly. In Hong Kong, a selective catalytic reduction (SCR) retrofit program has been implemented in phases since 2015 to further reduce the high NO2 emissions from diesel vehicles equipped with DPF, and the government has proposed enforcing Euro VI standard in 2017–2018 (HK ACE, Citation2013). It will be interesting to observe how HONO emission ratio will change when diesel vehicles with SCR systems become more popular.
Acknowledgment
The authors thank the Hong Kong Environmental Protection Department for providing the LOPAP instrument, and Xiaoliang Wang and Steven D. Kohl from the Desert Research Institute, Reno, NV for providing the wind speed data.
Funding
The work was supported by PolyU Project of Strategic Importance (1-ZE13), the Collaborative Research Fund of the Hong Kong Research Grants Council (C5022-14G), and the fund from Health Effects Institute (4947-RFPA14-1/15-1).
Additional information
Funding
Notes on contributors
Yutong Liang
Yutong Liang is a research assistant, Qiaozhi Zha, Weihao Wang, and Long Cui are graduate students in the Department of Civil and Environmental Engineering at The Hong Kong Polytechnic University.
Qiaozhi Zha
Yutong Liang is a research assistant, Qiaozhi Zha, Weihao Wang, and Long Cui are graduate students in the Department of Civil and Environmental Engineering at The Hong Kong Polytechnic University.
Qiaozhi Zha is now a PhD student in the Department of Physics, University of Helsinki.
Weihao Wang
Yutong Liang is a research assistant, Qiaozhi Zha, Weihao Wang, and Long Cui are graduate students in the Department of Civil and Environmental Engineering at The Hong Kong Polytechnic University.
Long Cui
Yutong Liang is a research assistant, Qiaozhi Zha, Weihao Wang, and Long Cui are graduate students in the Department of Civil and Environmental Engineering at The Hong Kong Polytechnic University.
Ka Hei Lui
Ka Hei Lui is a researcher and Kin Fai Ho is an assistant professor in the Jockey Club School of Public Health and Primary Care at The Chinese University of Hong Kong.
Kin Fai Ho
Ka Hei Lui is a researcher and Kin Fai Ho is an assistant professor in the Jockey Club School of Public Health and Primary Care at The Chinese University of Hong Kong.
Zhe Wang
Zhe Wang is a research assistant professor, Shun-cheng Lee is a professor, and Tao Wang is a Chair Professor of Atmospheric Environment in the Department of Civil and Environmental Engineering at The Hong Kong Polytechnic University.
Shun-cheng Lee
Zhe Wang is a research assistant professor, Shun-cheng Lee is a professor, and Tao Wang is a Chair Professor of Atmospheric Environment in the Department of Civil and Environmental Engineering at The Hong Kong Polytechnic University.
Tao Wang
Zhe Wang is a research assistant professor, Shun-cheng Lee is a professor, and Tao Wang is a Chair Professor of Atmospheric Environment in the Department of Civil and Environmental Engineering at The Hong Kong Polytechnic University.
References
- Arens, F., L. Gutzwiller, U. Baltensperger, H.W. Gäggeler, and M. Ammann. 2001. Heterogeneous reaction of NO2 on diesel soot particles. Environ. Sci. Technol. 35(11):2191–9. doi:10.1021/es000207s.
- Aubin, D.G., and J.P.D. Abbatt. 2007. Interaction of NO2 with hydrocarbon soot: Focus on HONO yield, surface modification, and mechanism. J. Phys. Chem. A 111(28):6263–73. doi:10.1021/jp068884h.
- Ban-Weiss, G.A., J.P. McLaughlin, R.A. Harley, A.J. Kean, E. Grosjean, and D. Grosjean. 2008. Carbonyl and nitrogen dioxide emissions from gasoline- and diesel-powered motor vehicles. Environ. Sci. Technol. 42(11):3944–50. doi:10.1021/es8002487.
- Barone, T.L., J.M.E. Storey, and N. Domingo. 2010. An analysis of field-aged diesel particulate filter performance: Particle emissions before, during, and after regeneration. J. Air Waste Manage. Assoc. 60(8):968–76. doi:10.3155/1047-3289.60.8.968.
- Bishop, G.A., B.G. Schuchmann, D.H. Stedman, and D.R. Lawson. 2012. Emission Changes resulting from the San Pedro Bay, California Ports Truck Retirement Program. Environ. Sci. Technol. 46(1):551–58. doi:10.1021/es202392g.
- Cantrell, C. A. 2008. Technical note: Review of methods for linear least-squares fitting of data and application to atmospheric chemistry problems. Atmos. Chem. Phys. 8(17):5477–87. doi:10.5194/acp-8-5477-2008.
- Carslaw, D.C. 2005. Evidence of an increasing NO2/NOx emissions ratio from road traffic emissions. Atmos. Environ. 39(26):4793–802. doi:10.1016/j.atmosenv.2005.06.023.
- Czader, B.H., Y. Choi, X. Li, S. Alvarez, and B. Lefer. 2015. Impact of updated traffic emissions on HONO mixing ratios simulated for urban site in Houston, Texas. Atmos. Chem. Phys. 15(3):1253–63. doi:10.5194/acp-15-1253-2015.
- Dallmann, T.R., R.A. Harley, and T.W. Kirchstetter. 2011. Effects of diesel particle filter retrofits and accelerated fleet turnover on drayage truck emissions at the Port of Oakland. Environ. Sci. Technol. 45(24): 10773–9. doi:10.1021/es202609q.
- Finlayson-Pitts, B.J., L.M. Wingen, A.L. Sumner, D. Syomin, and K.A. Ramazan. 2003. The heterogeneous hydrolysis of NO2 in laboratory systems and in outdoor and indoor atmospheres: An integrated mechanism. Phys. Chem. Chem. Phys. 5(2):223–42. doi:10.1039/B208564J.
- Gerecke, A., A. Thielmann, L. Gutzwiller, and M.J. Rossi. 1998. The chemical kinetics of HONO formation resulting from heterogeneous interaction of NO2 with flame soot. Geophys. Res. Lett. 25(13):2453–6. doi:10.1029/98GL01796.
- Gutzwiller, L., F. Arens, U. Baltensperger, H.W. Gäggeler, and M. Ammann. 2002. Significance of semivolatile diesel exhaust organics for secondary HONO formation. Environ. Sci. Technol. 36(4):677–82. doi:10.1021/es015673b.
- Han, C., Y. Liu., and H. He. 2013a. Heterogeneous photochemical aging of soot by NO2 under simulated sunlight. Atmos. Environ. 64:270–6. doi:10.1016/j.atmosenv.2012.10.008.
- Han, C., Y. Liu, and H. He. 2013b. Role of organic carbon in heterogeneous reaction of NO2 with soot. Environ. Sci. Technol. 47(7):3174–81. doi:10.1021/es304468n.
- Heeb, N.V., P. Schmid, M. Kohler, E. Gujer, M. Zennegg, D. Wenger, A. Wichser, A. Ulrich, U. Gfeller, P. Honegger, et al. 2008. Secondary effects of catalytic diesel particulate filters: Conversion of PAHs versus formation of nitro-PAHs. Environ. Sci. Technol. 42(10):3773–9. doi:10.1021/es7026949.
- Heimark, E., H. Lalogianni, M. Kilburn, and C. Loh. 2009. Paying for a Cleaner Bus Fleet—How Government Can Break the Log Jam. Hong Kong: Civic Exchange.
- Heland, J., J. Kleffmann, R. Kurtenbach, and P. Wiesen. 2001. A new instrument to measure gaseous nitrous acid (HONO) in the atmosphere. Environ. Sci. Technol. 35(15):3207–12. doi:10.1021/es000303t.
- Herner, J.D., S. Hu, W.H. Robertson, T. Huai, M.C O. Chang, P. Rieger, and A. Ayala. 2011. Effect of advanced aftertreatment for PM and NOx Reduction on heavy-duty diesel engine ultrafine particle emissions. Environ. Sci. Technol. 45(6):2413–9. doi:10.1021/es102792y.
- Herner, J.D., S. Hu, W.H. Robertson, T. Huai, J.F. Collins, H. Dwyer, and A. Ayala. 2009. Effect of advanced aftertreatment for PM and NOx control on heavy-duty diesel truck emissions. Environ. Sci. Technol. 43(15):5928–33. doi:10.1021/es9008294.
- HK ACE. 2013. Retrofitting Franchised Buses with Selective Catalytic Reduction Devices. Hong Kong: Advisory Council on the Environment.
- HK Environment Bureau. 2013. A Clean Air Plan for Hong Kong. Hong Kong: Environment Bureau.
- Ho, K.F., S.C. Lee, W.K. Ho, D.R. Blake, Y. Cheng, Y.S. Li, S.S.H. Ho, K. Fung, P.K.K. Louie, and D. Park. 2009. Vehicular emission of volatile organic compounds (VOCs) from a tunnel study in Hong Kong. Atmos. Chem. Phys. 9(19):7491–504. doi:10.5194/acp-9-7491-2009.
- Johnson, T.V. 2008. Diesel emission control in review. 2008 SAE World Congress, SAE Technical Paper, April, Detroit, Michigan.
- Kean, A.J., R.A. Harley, and G.R. Kendall. 2003. Effects of vehicle speed and engine load on motor vehicle emissions. Environ. Sci. Technol. 37(17):3739–46. doi:10.1021/es0263588.
- Khalek, I., T.L. Bougher, P.M. Merritt, and B. Zielinska. 2011. Regulated and unregulated emissions from highway heavy-duty diesel engines complying with U.S. Environmental Protection Agency 2007 emissions standards. J. Air Waste Manage. Assoc. 61(4):427–42. doi:10.3155/1047-3289.61.4.427.
- Khalizov, A.F., M. Cruz-Quinones, and R. Zhang. 2010. Heterogeneous reaction of NO2 on fresh and coated soot surfaces. J. Phys. Chem. A 114(28):7516–24. doi:10.1021/jp1021938.
- Kirchstetter, T.W., R.A. Harley, and D. Littlejohn. 1996. Measurement of nitrous acid in motor vehicle exhaust. Environ. Sci. Technol. 30(9):2843–9. doi:10.1021/es960135y.
- Kirchstetter, T.W., and T. Novakov. 2007. Controlled generation of black carbon particles from a diffusion flame and applications in evaluating black carbon measurement methods. Atmos. Environ. 41(9):1874–88. doi:10.1016/j.atmosenv.2006.10.067.
- Kleffmann, J. 2007. Daytime sources of nitrous acid (HONO) in the atmospheric boundary layer. Chem. Phys. Chem. 8(8):1137–44. doi:10.1002/cphc.200700016.
- Kurtenbach, R., K.H. Becker, J.A.G. Gomes, J. Kleffmann, J.C. Lörzer, M. Spittler, P. Wiesen, R. Ackermann, A. Geyer, and U. Platt. 2001. Investigations of emissions and heterogeneous formation of HONO in a road traffic tunnel. Atmos. Environ. 35(20):3385–94. doi:10.1016/S1352-2310(01)00138-8.
- Lee, B.P., Y.J. Li, J.Z. Yu, P.K.K. Louie, and C.K. Chan. 2015. Characteristics of submicron particulate matter at the urban roadside in downtown Hong Kong—Overview of 4 months of continuous high-resolution aerosol mass spectrometer measurements. J. Geophys. Res.: Atmos. 120(14):7040–58. doi:10.1002/2015JD023311.
- Millstein, D.E., and R.A. Harley. 2010. Effects of retrofitting emission control systems on in-use heavy diesel vehicles. Environ. Sci. Technol. 44(13):5042–8. doi:10.1021/es1006669.
- Monge, M.E., B. D’Anna, L. Mazri, A. Giroir-Fendler, M. Ammann, D.J. Donaldson, and C. George. 2010. Light changes the atmospheric reactivity of soot. Proc. Natl. Acad. Sci. USA 107(15):6605–9. doi:10.1073/pnas.0908341107.
- Pitts, J.N., H.W. Biermann, A.M. Winer, and E.C. Tuazon. 1984. Spectroscopic identification and measurement of gaseous nitrous acid in dilute auto exhaust. Atmos. Environ. (1967) 18(4):847–54. doi:10.1016/0004-6981(84)90270-1.
- Pusede, S.E., T.C. VandenBoer, J.G. Murphy, M.Z. Markovic, C.J. Young, P.R. Veres, J.M. Roberts, R.A. Washenfelder, S.S. Brown, X. Ren, et al. 2015. An atmospheric constraint on the NO2 dependence of daytime near-surface nitrous acid (HONO). Environ. Sci. Technol. 49(21):12774–81. doi:10.1021/acs.est.5b02511.
- Rappenglück, B., G. Lubertino, S. Alvarez, J. Golovko, B. Czader, and L. Ackermann. 2013. Radical precursors and related species from traffic as observed and modeled at an urban highway junction. J. Air Waste Manage. Assoc. 63(11):1270–86. doi:10.1080/10962247.2013.822438.
- Ropkins, K., J. Beebe, H. Li, B. Daham, J. Tate, M. Bell, and G. Andrews. 2009. Real-world vehicle exhaust emissions monitoring: Review and critical discussion. Crit. Rev. Environ. Sci. Technol. 39(2):79–152. doi:10.1080/10643380701413377.
- Shorter, J.H., S. Herndon, M.S. Zahniser, D.D. Nelson, J. Wormhoudt, K.L. Demerjian, and C.E. Kolb. 2005. Real-time measurements of nitrogen oxide emissions from in-use New York City transit buses using a chase vehicle. Environ. Sci. Technol. 39(20):7991–8000. doi:10.1021/es048295u.
- Sjödin, Å., and M. Ferm. 1986. Authors’ reply. Atmos. Environ. (1967) 20(2):409–11. doi:10.1016/0004-6981(86)90048-X.
- Spataro, F., and A. Ianniello. 2014. Sources of atmospheric nitrous acid: State of the science, current research needs, and future prospects. J. Air Waste Manage. Assoc. 64(11):1232–50. doi:10.1080/10962247.2014.952846.
- Trinh, H.T., K. Imanishi, T. Morikawa, H. Hagino, and N. Takenaka. 2016. Gaseous nitrous acid (HONO) and nitrogen oxides (NOx) emission from gasoline and diesel vehicles under real-world driving test cycles. J. Air Waste Manage. Assoc. 67 (4):412–20. doi:10.1080/10962247.2016.1240726.
- Tzamkiozis, T., L. Ntziachristos, and Z. Samaras. 2010. Diesel passenger car PM emissions: From Euro 1 to Euro 4 with particle filter. Atmos. Environ. 44(7):909–16. doi:10.1016/j.atmosenv.2009.12.003.
- van Setten, B.A.A.L., M. Makkee, and J.A. Moulijn. 2001. Science and technology of catalytic diesel particulate filters. Catal. Rev. 43(4):489–564. doi:10.1081/CR-120001810.
- Wong, K.W., C. Tsai, B. Lefer, N. Grossberg, and J. Stutz. 2013. Modeling of daytime HONO vertical gradients during SHARP 2009. Atmos. Chem. Phys. 13(7):3587–601. doi:10.5194/acp-13-3587-2013.
- Wood, E.C., S.C. Herndon, M.T. Timko, P.E. Yelvington, and R.C. Miake-Lye. 2008. Speciation and Chemical evolution of nitrogen oxides in aircraft exhaust near airports. Environ. Sci. Technol. 42(6):1884–91. doi:10.1021/es072050a.
- Wormhoudt, J., E.C. Wood, W.B. Knighton, C.E. Kolb, S.C. Herndon, and E.P. Olaguer. 2015. Vehicle emissions of radical precursors and related species observed in the 2009 SHARP campaign. J. Air Waste Manage. Assoc. 65(6):699–706. doi:10.1080/10962247.2015.1008654.
- Xu, Z., T. Wang, J. Wu, L. Xue, J. Chan, Q. Zha, S. Zhou, P.K.K. Louie, and C.W.Y. Luk. 2015. Nitrous acid (HONO) in a polluted subtropical atmosphere: Seasonal variability, direct vehicle emissions and heterogeneous production at ground surface. Atmos. Environ. 106:100–9. doi:10.1016/j.atmosenv.2015.01.061.
- Xu, Z., T. Wang, L.K. Xue, P.K.K. Louie, C.W.Y. Luk, J. Gao, S.L. Wang, F.H. Chai, and W.X. Wang. 2013. Evaluating the uncertainties of thermal catalytic conversion in measuring atmospheric nitrogen dioxide at four differently polluted sites in China. Atmos. Environ. 76:221–6. doi:10.1016/j.atmosenv.2012.09.043.
- Yao, X., N.T. Lau, C.K. Chan, and M. Fang. 2005. The use of tunnel concentration profile data to determine the ratio of NO2/NO directly emitted from vehicles. Atmos. Chem. Phys. Discuss. 2005:12723–40. doi:10.5194/acpd-5-12723-2005.
- Zha, Q., L.T. Xue, Z. Wang, Z. Xu, C. Yeung, P.K.K. Louie, and C.W.Y. Luk. 2014. Large conversion rates of NO2 to HNO2 observed in air masses from the South China Sea: Evidence of strong production at sea surface? Geophys. Res. Lett. 41(21):7710–5. doi:10.1002/2014GL061429