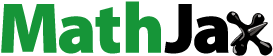
ABSTRACT
Method 30B and the Ontario Hydro Method (OHM) were used to sample the mercury in the flue gas discharged from the seven power plants in Guizhou Province, southwest China. In order to investigate the mercury migration and transformation during coal combustion and pollution control process, the contents of mercury in coal samples, bottom ash, fly ash, and gypsum were measured. The mercury in the flue gas released into the atmosphere mainly existed in the form of Hg°. The precipitator shows a superior ability to remove Hgp (particulate mercury) from flue gas. The removal efficiency of Hg2+ by wet flue gas desulfurization (WFGD) was significantly higher than that for the other two forms of mercury. The synergistic removal efficiency of mercury by the air pollution control devices (APCDs) installed in the studied power plants is 66.69–97.56%. The Hg mass balance for the tested seven coal-fired power plants varied from 72.87% to 109.67% during the sampling time. After flue gas flowing through APCDs, most of the mercury in coal was enriched in fly ash and gypsum, with only a small portion released into the atmosphere with the flue gas. The maximum discharge source of Hg for power plants was fly ash and gypsum instead of Hg emitted with flue gas through the chimney into the atmosphere. With the continuous upgrading of APCDs, more and more mercury will be enriched in fly ash and gypsum. Extra attention should be paid to the re-release of mercury from the reutilization of by-products from APCDs.
Implications: Method 30B and the Ontario Hydro Method (OHM) were used to test the mercury concentration in the flue gas discharged from seven power plants in Guizhou Province, China. The concentrations of mercury in coal samples, bottom ash, fly ash, and gypsum were also measured. By comparison of the mercury content of different products, we found that the maximum discharge source of Hg for power plants was fly ash and gypsum, instead of Hg emitted with flue gas through the chimney into the atmosphere. With the continuous upgrading of APCDs, more and more mercury will be enriched in fly ash and gypsum. Extra attention should be paid to the re-release of mercury from the reutilization of by-products from APCDs.
Introduction
Mercury (Hg) can be widely dispersed and transported thousands of miles away from the sources of emissions, endangering human health and the environment severely (Christian Seigneur et al. Citation2004; Wu et al. Citation2012, Citation2010). Both natural processes and anthropogenic activities can accelerate the migration and transformation of mercury (Riget et al. Citation2000). Mercury has been increasingly released from anthropogenic sources, which would increase the accumulation of mercury in living organisms and ultimately do serious harm to all living creatures on the earth (Pacyna et al. Citation2006; Pavlish et al. Citation2003). The modern model of atmosphere Hg circulation revealed that the Hg budget had tripled since preindustrial times. The increase of Hg in the atmospheric environment is mainly from industrial production (Kumari et al. Citation2015; Pacyna et al. Citation2016), such as mineral mining and fuel combustion, mercury and other nonferrous metal smelting, plastics industry, electronics industry, and chlor-alkali industry production. Fossil fuel combustion, especially the utilization of coal in coal-fired power plants, is the most important source of anthropogenic mercury pollution.
China is experiencing rapid economic growth and industrial development, and the amount of coal used has been rising yearly (Wu et al. Citation2015). Now China has become the largest coal producer and consumer in the world (Li Citation2012). Coal combustion accounts for one-third of the global Hg emission into the atmosphere and is the single largest Hg source in China (Burmistrz et al. Citation2016; Wang, Wenguo Shen, and Ma Citation2000). In recent years, as the coal used for power generation will continue to increase along with the low pretreatment rate of raw coal in China, a large amount of mercury would be released into the atmosphere from coal-fired power plants (Bai et al. Citation2017a, Citation2017b; Jiang, Shi, and Feng Citation2006; Tian et al. Citation2011). Consequently, China has been developing and using special mercury control equipment to limit the emission of mercury from coal-fired power plants (Srivastava et al. Citation2006; Wdowin et al. Citation2015, Citation2014; Wiatros-Motyka et al. Citation2013). Mercury in flue gas from coal-fired power plant is speciated into elemental mercury (Hg°), oxidized mercury (Hg2+), and particulate mercury (Hgp) (Galbreath and Zygarlicke Citation2000). Present-day coal-fired power plants are installed with selective catalytic reduction (SCR), electrostatic precipitators (ESPs) and flue gas desulfurization (FGD) to control NOx, particles, and SOx, respectively. The selective catalytic reduction (SCR) devices, which are widely used in the coal-fired power plants, can oxidize Hg° to Hg2+. Hg2+ and HgP can be removed by air pollution control devices (APCDs) in coal-fired power plants, where wet flue gas desulfurization (WFGD) can remove up to 95% of Hg2+, and dust removal equipment such as the electrostatic precipitator (ESP) and fabric filter (FF) can remove HgP in the flue gas effectively (Durnford et al. Citation2010; Mark Loewen et al. Citation2007; Temme et al. Citation2003).
In China, air pollution is becoming more and more serious. Thus, a series of policies was implemented to control mercury and other pollutants emission from coal-fired power plants (Shuxiao and Jiming Citation2012; Wang et al. Citation2014). The “Emission standard of air pollutants for thermal power plants” (GB 13223–2011) in China, which was implemented in 2015, specified a limit of mercury emission from coal-fired power plants (Tao et al. Citation2012). Many research results confirmed that due to the implementation of these regulations, the emission of mercury from coal-fired power plants has been greatly reduced (Li et al. Citation2010; Shuxiao et al. Citation2014). At present, most of the research is focused on mercury emission from coal-fired plants, but the actual measurements of mercury species, concentration in flue gases, and Hg captured by APCDs are still lacking in China.
Guizhou is the largest coal production province in southwest China. Because of its special geochemical background, the mercury content of coal in Guizhou is higher than that in other provinces (Qiu et al. Citation2006). Feng et al. (Citation2002) investigated 48 crude coal samples from the four major types of coal in Guizhou, and found that the mercury content in coal was between 100 and 2670 ng/g with an average value of 530 ng/g, which is much higher than the average mercury content of 210 ng/g in the coal of China (Wang, Wenguo Shen, and Ma Citation2000; Zhang et al. Citation2012). With the implementation of the Chinese western development strategy, Guizhou had built more coal-fired power plants using Hg-riched coal, leading to a large amount of mercury emitted into the atmosphere (Tang et al. Citation2007). The purpose of this paper is to investigate the mercury migration and transformation in flue gas in seven coal-fired power plants in Guizhou Province, southwest China. Seven typical power plants were chosen to investigate the mercury emission characteristics. Hg species in flue gases from the DF and QX power plants were directly determined. The contents of Hg in coal and the by-products of coal combustion and APCDs (including bottom ash, fly ash, gypsum (sulfuric acid) and flue gas) from the seven typical coal-fired plants were analyzed, respectively. The synergistic removal efficiency of mercury by different APCDs was verified through the Hg mass balance.
Materials and methods
Site description
Guizhou Province is an important energy exporter in China. In 2017, the output of raw coal in Guizhou was 1.5 billion tons, ranking number 4 in coal resource in the whole of China, and only lower than that in Inner Mongolia, Shanxi, and Shaanxi (Li et al. Citation2012; Qin et al. Citation2017). Most of the local coal in Guizhou can be characterized as anthracite. Based on the distribution characteristics of the coal in Guizhou, seven typical power plants have been selected, which are the QX power plant, DF power plant, FQ power plant, PX power plant, PB power plant, XY power plant, and FE power plant. The layouts of the boiler type, capacity, coal type, and APCDs are summarized in .
Table 1. The basic situation of the power plants.
The selection of power plants takes into account the diversity and representation of installed capacities, combustion mode, and APCDs. The mercury concentration of coal that used in QX, FQ, and XY power plants is basically equal to the average mercury concentration of China, while the concentration of mercury in the PX, PB, DF, and FE power plants is lower than the average value.
Sampling and analysis
The availabilities of mercury sampling and detection methods currently on the market have been investigated thoroughly, and the U.S. EPA Method 30B (Li et al. Citation2018) and Ontario Hydro Method (OHM) (Kellie et al. Citation2004) were chosen for flue gas mercury sampling. The specific sampling process is provided in the support information. By comparing the two sampling methods in the support information, the OHM was used for the testing of the species of mercury in the DF power plant and the QX power plant, and the mercury in the flue gas emitted in other power plants was tested by Method 30B.
Before the test, the sampling units were required to maintain the stability of the system for more than 2 hr and during the test period its output was greater than 85% of the rated load. At the same time, the SCR, air preheater (APH), ESP, and desulfurization system were operated under normal parameters. Boiler load and air volume were stable during the test. In order to ensure the stability of the boiler, soot blowing and slagging operations were stopped during sampling.
The distribution of sampling points in a power plant with SCR+ ESP/FF+ WFGD is illustrated in . During the flue gas sampling, the feed coal and the by-products of APCDs, such as bottom ash, fly ash, and gypsum, were sampled at the same time. The sampling frequency was determined according to the sampling time of the flue gas. Each investigation in the power plant would last 2–3 days, and the sampling of by-products is generally done two or three times per day. The feed coal was sampled from the coal feeder. The bottom ash of boiler was sampled from crape slag of salvaging machine. The fly ash was taken from each bucket of ESP and the desulfurized gypsum was from the FGD dehydration belt (.).
Table 2. The description of the sampling sites.
After the liquid samples were digested, the mercury concentrations were analyzed with the Brooks Rand Model III of cold vapor atomic fluorescence spectrometry (CVAFS) technique in the laboratory. The contents of mercury in the solid samples were analyzed by using the theoretical analysis method in the laboratory using the Lumex RA-915M mercury analyzer and RA-915+ PYRO-915 pyrolysis furnace (LUMEX-MARKETING JSC, Russia), and the solid samples were analyzed according to the relevant standards of China. The main analysis process was to place the sample in a quartz boat and then insert it into the pyrolysis furnace. The released Hg and other volatile compounds were passed though the analysis cell, and mercury concentration data were obtained.
Quality control and quality assurance
The sampling and testing were carried out strictly in accordance with the sampling and analysis standards for mercury in coal-fired flue gas. Due to the low concentration of mercury in flue gas and by-products, the reagents used for OHM sampling were lower Hg blanks. In order to prevent the flue gas from condensing before entering the adsorption bottles, the temperature of the heated probe and the heated area was controlled at 120°C. The sampling rate was maintained at around 8 L/min during a 2-hr sampling period. All absorption bottles were placed in the ice bath to prevent evaporation of the sampling solutions and strengthen flue gas absorption. During the flue gas sampling using Method 30B, two absorbent traps were sampled simultaneously to ensure the accuracy of the experiment. All the traps were well controlled by the USA Ohio Lumex Co., Inc. No target mercury compounds were detected in the sorbent traps before sampling. The analyzer was calibrated as per Method 30B using carbon as calibration substrate. Two calibration curves were generated to analyze the absorbents. KCl trap sections were analyzed at 570°C to 590°C and carbon sections were analyzed at 680°C. In order to adjust the appropriate sampling flow rate and the sampling time, the content of Hg in flue gas absorbed in the traps was measured initially by Lumex R915 M. During the sampling process of Method 30B, the temperature was controlled by the heating line, so that the temperature of the sampler remains the same as that of the flue gas. The sampling time and sampling rate were different depending on the power plant, but most of them were around 30 min and 300 mL/min, respectively. In addition, a discussion of the uncertainty of the two methods is also provided in the supporting information.
New analytical standard curves, parallel samples, and random sampling were conducted to ensure the quality of analyzing. The new analytical standard curve was made by standard substances for soil composition analysis GBW 07405 (GSS-5) (290 ng/g), and the correlation coefficient of standard curve reached more than 99.9%. Due to the existence of replicate samples, the accuracy of the mercury concentrations in the sample was well guaranteed. The final data was averaged based on the data for each sample point. The analyzer was cleaned and the analytical standard curve was reconstructed before analyzing the samples for each power plant.
Results and discussion
Mercury in feed coal
The coal used in the selected power plants can be characterized as anthracite, which has high sulfur and ash, low melting point, and extreme hardness, and the quality of the coal is relatively stable ().
Table 3. Analysis results of coal samples.
As shown in , the Hg content in coal samples ranges from 134.33 ng/g to 231.03 ng/g, with the highest content of mercury in the XY power plant (up to 231.03 ng/g). The mercury content of coal in Guizhou Province was distributed between 20 and 440 ng/g, and the test samples were all within the distribution range of China (Streets et al. Citation2005; Wang, Wenguo Shen, and Ma Citation2000). The mercury contents of the QX power plant, FQ power plant, and XY power plant were basically equal to the average mercury content in Guizhou Province, while the mercury contents in the PX, PB, DF, and FE coal plants were even lower. The content of chlorine in the coal has a great influence on the form of mercury in the flue gas. Adequate free chlorine and a decreasing temperature of flue gas are thermodynamically favorable to oxidize Hg° to Hg2+, assisted by the SCR (Cao et al. Citation2005). However, it can be found from that the chlorine content of the coal used in the seven power plants is relatively low (0.017–0.024%), lower than the average content of chlorine in world coal (0.1%) (Zhang et al. Citation2004). In fact, all coal in China is low-chlorine coal (0.01–0.03%) (Chen Citation2010).
Mercury in flue gas
Mercury is highly volatile, making it different from other trace elements in the process of coal combustion (Xu et al. Citation2003). In the combustion zone of a coal-fired power plant, all the mercury in coal is vaporized as Hg°. In the process of flue gas flowing through each heating surface, the flue gas continues cooling and the mercury in the flue gas undergoes a series of physical and chemical changes (Senior et al. Citation2000; Zhang and Wong Citation2007). Part of the Hg° reacts with other components in the flue gas to form compounds of Hg2+, and some of the Hg° will be adsorbed or condensed on the surface of the residual carbon particles in the fly ash to form HgP, and some of the mercury will remain in gas phase (Withum Citation2004; Yang, Huang, and Wang Citation2012).
During the implementation of the campaign, the flue gas was sampled in DF power plant at sampling sites 1, 2, 4, and 6, respectively. In the QX power plant, sampling of flue gas was conducted at sampling points 3, 5, and 6, respectively. For the rest of the power plants, the concentration of mercury in flue gas after desulfurization was tested (.). The mercury emissions, emission rate, and mass balance were calculated.
Table 4. Mercury concentration in flue gas.
As shown in , the PB power plant used a circulating fluidized-bed boiler for desulfurization and the FQ power plant implements organic amine for desulfurization, while the rest of the power plants were using wet flue gas desulfurization (WFGD). By comparing the mercury concentration in flue gas before and after desulfurization in the QX and FQ power plants, it was found that the mercury concentration in flue gas decreased by 80% and 92% after WFGD, due to the removal of Hg2+ in a scrubber solution since Hg2+ is soluble in water. The mercury concentration of the flue gas discharged from the SCR+ ESP+ WFGD in the XY power plant was the highest, reaching 6.72 μg/Nm3, which was 11 times that in the DF power plant and 9 times that of the PX power plant. The DF and PX power plants used the ESP/FF for dust removal; their removal efficiencies of mercury were relatively high, which benefits from high dust removal efficiency of ESP/FF. The mercury content of coal in the XY power plant was 1.7 times higher than that of the DF power plant, and 1.45 times that of the PX power plant, which made the XY power plant have the highest mercury concentration in flue gas. The Hg concentration after APCDs ranged from 0.60 µg/Nm3 to 6.72 µg/Nm3. The mercury concentration emission to the atmosphere was below the 30 μg/Nm3 specified in GB13223-2011(in China).
Mercury in the by-products of coal combustion and APCDs
The mercury contents in the coal, fly ash, bottom slag, and gypsum or sulfuric acid were sampled and tested (.).
Table 5. Mercury in combustion products of each power plant.
Results showed that mercury was redistributed in the by-products of coal combustion and APCDs. The concentration of mercury in the bottom slag was only about 1 ng/g and the proportion of mercury in the bottom ash to the total mercury in the by-products of coal combustion and APCDs was less than 1%. This result agreed well with the conclusions drawn by other researchers (Tang et al. Citation2016). According to the mass balance calculation, it is found that almost all mercury in coal was released into the flue gas under the condition of high-temperature combustion. The proportion of mercury in the fly ash that was removed by the precipitator was relatively high. The average mercury content in the fly ash accounted for up to 59.49% of total mercury as the by-products of coal combustion and APCDs, indicating that the mercury in the flue gas can be highly enriched in fly ash and then can be removed by a precipitator. That is, mercury synergistic capture by the dust removal equipment was significant. The average mercury content in the gypsum was 32.74% of the total mercury content in the by-products of coal combustion and APCDs. The average mercury content in the flue gas was 13.50% of the total mercury in the products of coal combustion and APCDs, and only 5% of mercury in coal was left in the flue gas after APCDs. The concentration of mercury in sulfuric acid produced by organic amine desulfurization in FQ power plant was 50.07 μg/L, accounting for 48.98% of the total mercury.
Our results showed that mercury enriched in the fly ash and gypsum was much more (.). In China, the reutilization rate of desulfurization gypsum and fly ash was 87.13% and 74%, respectively (China Environment Yearbook, Citation2011; Meng and Wang Citation2012). In the process of reutilization, the re-release of mercury enriched in by-products was related to the existing species of mercury and temperature of process. (D Lee et al. Citation2009; Noel, Biswas, and Giammar Citation2007; Zou et al. Citation2016). During the reutilization of by-products, the mercury levels emitted to air, released to soil, and stored in products including cement, gypsum wallboard, and fly ash brick were 19.94%, 35.01%, and 45.46%, respectively(Hui et al. Citation2015). Hence, the releasable mercury in the by-products APCDs shouldn’t be neglected.
Mercury speciation in flue gas
To explore the effects of common APCDs on the removal of mercury species, the concentrations of mercury species in flue gas were measured at different sampling points in the DF power plant and QX power plant.
The DF power plant was a typical coal-fired power plant with SCR+ ESP/FF+ WFGD devices. Mercury in flue gas was emitted to the atmosphere dominantly in the form of Hg° and the concentration of Hg° was 0.49 μg/Nm3, accounting for 81.66% of all Hg species in emitted flue gas, while the concentrations of Hg2+ and HgP were much lower than that of Hg°. The concentration of Hg2+ was 0.10 μg/Nm3, accounting for 16.67% of total mercury, and the concentration of HgP was 0.005 μg/Nm3, accounting for 0.75% of total mercury in the flue gas. These results were far lower than that of the Hg emission limitation of 30 μg/Nm3 specified by GB13223-2011 in China. It can be concluded that the mercury in the flue gas, especially Hg2+ and HgP, was removed efficiently from flue gas by SCR+ ESP/FF+ WFGD devices. According to the other data we summarized, before SCR and ESP/FF the mercury in flue gas was mainly in the form of HgP, accounting for 57.62% and 86.94%, respectively. After SCR, the proportion of Hg° to HgT in flue gas decreased from 23.39% to 3.9%. This was due to the SCR system having a certain oxidation effect on the conversion of Hg° to Hg2+ and HgP (Durnford et al. Citation2010; Withum Citation2004). After ESP/FF, 99.94% of the HgP in the flue gas was removed, with Hg° and Hg2+ accounting for 42.85% and 58.44%, respectively. This implied that ESP/FF played an important role in the control of HgP in flue gas. The mercury removal efficiency of WFGD depends largely on the amount of Hg2+ appearing at the inlet of the scrubber (Yueyang et al. Citation2014). The concentration of mercury was only 0.6 μg/Nm3 after flue gas passing through the WFGD and the concentration of Hg2+ decreased by 66.67% compared to the flue gas before WFGD, while the effect of removing Hg° and HgP was negligible. The overall Hg removal efficiency of APCDs in the DF power plant was 97.56%.
Taking the QX power plant as an example, the mercury in the flue gas at the outlet of WFGD was mainly in the form of Hg°, accounting for 78.46% of all mercury speciation in the flue gas, while the concentrations of Hg2+ and Hg° were relatively low, accounting for 20.76% and 0.76%, respectively. The concentration of mercury in the flue gas was 1.30 μg/Nm3. Even so, the concentration of mercury in the flue gas emitted from the QX power plant still met the emission limitation of 30 μg/Nm3 specified by GB 132223–2011 in China. For the flue gas before ESP/FF, the mercury mainly existed in the form of HgP, accounting for 62.14% of total mercury. The concentrations of Hg° and Hg2+ in the flue gas were relatively low, accounting for 22.01% and 15.85%, respectively. After flue gas passed through the ESP/FF, almost all the HgP (99.45%) was removed, and the main forms of mercury in the flue gas were Hg° and Hg2+, accounting for 50.23% and 48.55%, respectively. Hg2+ in the flue gas was removed by the WFGD device. In the QX power plant, the WFGD device removed 91.48% of Hg2+ in the flue gas. The overall Hg removal efficiency of SCR+ ESP/FF+ WFGD devices in the QX power plant was 94.40%.
Through the investigation in the DF and QX power plants, almost all the mercury was removed by APCDs in the power plants, with only a small fraction of mercury (about 5%) in coal combustion flue gas was emitted into the atmosphere. Among the three speciations of mercury in the flue gas, the Hg2+ is water-soluble, which contributed to the high Hg removal efficiency of the WFGD (Withum Citation2004), while the mercury enriched on the particles (HgP) is easily removed by the precipitator. Therefore, the combination of precipitator and WFGD played an important role of co-beneficial Hg control in the flue gas after coal combustion.
Mercury mass balance of each coal-fired power plant
In order to examine the accuracy and reliability of the final data, mercury mass balance had been calculated with the sampling and analytical campaigns in . The consumption of coal and the discharge of bottom ash, fly ash, and gypsum of each studied power plant are shown in .
Table 6. The overall mass flow rate of each stream of each power plant.
Mercury emissions from boiler and flue gas control devices include bottom ash emissions after coal combustion (Hgbottom ash), fly ash emissions after ESP or FDC or ESP/FF (Hgfly ash), gypsum emissions after a WFGD system (Hggypsum), and cleaned flue gas emission to the atmosphere (Hgflue gas). The method of establishing mass balance is shown in the following equation:
The characteristics of mercury and the emission factors of mercury in the DF power plant, which installed an SCR+ ESP/FF+ WFGD system for flue gas treatment, can be deduced. During coal combustion, the mercury and its compounds in coal at high temperature undergo a series of reactions, and then almost all the mercury went into the flue gas in the form of Hg°; only 0.01% of the mercury in the coal was remained in the bottom ash. After the flue gas passed through the SCR and air preheater, the Hg° was oxidized to Hg2+ or adsorbed by high-concentration fly ash in the flue gas, resulting in a significant increase in Hg2+ and HgP. When flue gas passed though ESP/FF and WFGD, 81.88% of mercury in coal was removed by ESP/FF and the mercury removed by WFGD accounted for 13.31%. Finally, a very small fraction of mercury (4.7%) in coal was discharged into the atmosphere. The mercury discharged into the atmosphere consists of 81.67% of Hg as Hg°, 16.67% of Hg as Hg2+, and 0.83% of Hg as HgP. The overall mercury removal efficiency of APCDs in the DF power plant was 97.56%. During the coal combustion, the variation trend of the mercury in the QX, PX, and XY power plants was the same as for the DF, because they have the same flue gas treatment system. The overall mercury removal efficiency values of APCDs in the QX, PX, and XY power plants were 95.75%, 96.63%, and 83.40% respectively ( and ).
In the FQ power plant, almost all of the mercury in the coal was redistributed to the by-products of SCR+ ESP+ ammonia desulfurization devices. The overall mercury removal efficiency of APCDs in the FQ power plant was reached 96.05%. In the process of flue gas passing through SCR+ ESP+ ammonia desulfurization devices, 45.98% of mercury was enriched in fly ash, which was then trapped by ESP, and the sulfuric acid from the ammonia desulfurization device enriches a certain amount of mercury, accounting for 48.98% of mercury in the by-products of coal combustion and APCDs. Finally, a small fraction of mercury (4%) in the coal was released into the atmosphere ( and ).
A circulating fluidized-bed combustion technique was used in the PB power plant, which installed selective non-catalytic reduction (SNCR)+ ESP/FF for flue gas treatment. With the flue gas outflowed from the furnace, followed by ESP/FF, mercury in the flue gas was mainly enriched in fly ash (62.94%), which was discharged to the environment or as a raw material of the construction industry. Eventually, a fraction of the Hg (33.02%) in coal finally was emitted to the atmosphere with flue gas through the chimney. The overall mercury removal efficiency of APCDs was 66.96% ( and ).
The fates and discharge factors of Hg with ESP+ WFGD devices in the FE power plant were obvious: 78.35% of Hg in coal was released to the by-products of combustion and APCDs, including 34.33% enriched in fly ash, 42.35% transferred in gypsum, and 24.00% of Hg in coal was emitted to the atmosphere, contributing to the global cycling ( and ).
For the seven power plants in the study, the values of mercury mass balance were in the range of 72.87–109.67% (). Generally speaking, for field monitoring of trace heavy metals such as mercury, a final mass balance value is acceptable from 70% to 130% (Goodarzi Citation2004; Yokoyama et al. Citation2000). The data for mercury mass balance calculated in the study showed that our sampling method, sampling equipment, and analytical method were credible and achieved certain accuracy.
Through the analysis of the mercury distribution in the combustion and flue gas treatment process in the seven power plants ( and ), it can be deduced that the precipitator and FGD in coal-fired power plants can synergistic capture Hg in flue gas efficiently. According to our results already described and this discussion, mercury emitted directly to the atmosphere with flue gas was much less than that of Hg enriched in fly ash and Hg transferred to the desulfurization gypsum. Our results clearly showed that the mercury in the flue gas accounted for only 5–34% of the mercury in the by-products of combustion and APCDs. The maximum discharge source of Hg for power plants was fly ash and gypsum, instead of Hg emitted with flue gas through the chimney into the atmosphere. From the perspective of power generation, fly ash and gypsum were waste material, which can be fully utilized. Some of the utilization processes, especially high-temperature procedures, can lead to all Hg re-emissions and emit a considerable amount of Hg emissions to the atmosphere. Further researches should focus on these secondary Hg pollution problems.
Conclusion
The mercury concentration in the flue gas and the mercury contents in the by-products of coal combustion and APCDs had been measured from coal-fired power plants in Guizhou, southwest China. The mercury in the flue gas discharged from coal-fired power plants existed mainly in the form of Hg°. The APCDs installed in the power plants play a vital role in synergistic removal of mercury from the flue gas. The overall mercury removal efficiency of the APCDs installed in the studied power plants varied from 66.69% to 97.56% and the mercury mass balance varied from 72.87% to 109.67% during the sampling time.
Through the analysis of the mercury content in the by-products of coal combustion and APCDs in seven power plants, the precipitator showed superior ability to remove Hgp from flue gas. The removal efficiency of Hg2+ by WFGD was significantly higher than that of the other two forms of mercury. Mercury emitted directly to the atmosphere with flue gas accounted for only 5–34% of the mercury in the by-products of combustion and APCDs. The maximum discharge source of Hg for power plants was via fly ash and gypsum, instead of Hg emitted with flue gas through the chimney into the atmosphere. In the future, the control of mercury pollution from coal-fired power plants should pay more attention to the release of mercury during the reutilization of APCDs by-products.
Supplemental Material
Download MS Word (354.5 KB)Supplementary data
Supplementary data for this paper can be accessed on the publisher’s website.
Additional information
Funding
References
- Bai, X., H. Ding, J. Lian, D. Ma, X. Yang, N. Sun, W. Xue, and Y. Chang. 2017a. Coal production in China: Past, present, and future projections. Int. Geol. Rev 60 (5-6):1–13.
- Bai, X., H. Ding, J. Lian, D. Ma, X. Yang, N. Sun, W. Xue, and Y. Chang. 2017b. Coal production in China: Past, present, and future projections. Int. Geol. Rev 60 (5-6):1–13.
- Burmistrz, P., K. Kogut, M. Marczak, and J. Zwoździak. 2016. Lignites and subbituminous coals combustion in Polish power plants as a source of anthropogenic mercury emission. Fuel Process. Technol. 152:250–258. doi:10.1016/j.fuproc.2016.06.011.
- Cao, Y., Y. Duan, S. Kellie, L. Li, W. Xu, J. T. R. And, W. P. Pan, P. C. And, A. K. Mehta, and R. Carty. 2005. Impact of coal chlorine on mercury speciation and emission from a 100-MW utility boiler with cold-side electrostatic precipitators and low-NOx burners. Energy Fuels 19 (3):842–854. doi:10.1021/ef034107u.
- Chen, L. 2010. Study on environmental geochemistry of chlorine in Chinese coals. Nancahng Univ.
- China Environment Yearbook Association. 2011. Production, disposal and utilization of industrial solid waste by region. China environmental yearbook22,611-615.
- Durnford, D., A. Dastoor, D. Figuerasnieto, and A. Ryjkov. 2010. Long range transport of mercury to the Arctic and across Canada. Atmos. Chem. Phys. 10 (13):6063–6086. doi:10.5194/acp-10-6063-2010.
- Feng, X., J. Sommar, O. Lindqvist, and Y. Hong. 2002. Occurrence, emissions and deposition of mercury during coal combustion in the Province Guizhou, China. Water Air Soil Pollut. 139 (1):311–324. doi:10.1023/A:1015846605651.
- Galbreath, K. C., and C. J. Zygarlicke. 2000. Mercury transformations in coal combustion flue gas. Fuel Process. Technol. 65 (99):289–310. doi:10.1016/S0378-3820(99)00102-2.
- Goodarzi, F. 2004. Speciation and mass-balance of mercury from pulverized coal fired power plants burning western Canadian subbituminous coals. J. Environ. Monit. 6 (10):792–798. doi:10.1039/b401827c.
- Hui, M. L., Z. Lei, Z. G. Wang, and S. X. Wang. 2015. The mercury mass flow and emissions of coal-fired power plants in China. China Environ. Sci. 35 (8):2241–2250.
- Jiang, G. B., J. B. Shi, and X. B. Feng. 2006. Mercury pollution in China. Environ. Sci. Technol. 40 (12):3672–3678. doi:10.1021/es062707c.
- Kellie, S., Y. Duan, Y. Cao, P. Chu, A. Mehta, R. Carty, K. Liu, W. P. Pan, and J. T. Riley. 2004. Mercury emissions from a 100-MW wall-fired boiler as measured by semicontinuous mercury monitor and Ontario Hydro Method. Fuel Process. Technol. 85 (6–7):487–499. doi:10.1016/j.fuproc.2003.11.004.
- Kumari, A., B. Kumar, S. Manzoor, and U. Kulshrestha. 2015. Status of atmospheric mercury research in South Asia: A review. Aerosol Air Qual. Res. 2015:3.
- Lee, J. Y., K. Cho, L. Cheng, T. C. Keener, G. Jegadeesan, and S. R. Al-Abed. 2009. Investigation of a mercury speciation technique for flue gas desulfurization materials. Air Repair 59 (8):972–979.
- Li, C., Q. Zhang, N. A. Krotkov, D. G. Streets, K. He, S. C. Tsay, and J. F. Gleason. 2010. Recent large reduction in sulfur dioxide emissions from Chinese power plants observed by the Ozone Monitoring Instrument. Geophys. Res. Lett 37 (8):292–305. doi:10.1029/2010GL042594.
- Li, C., Y. Duan, H. Tang, C. Zhu, Y. Zheng, and T. Huang. 2018. Mercury emissions monitoring in a coal-fired power plant by using the EPA method 30B based on a calcium-based sorbent trap. Fuel 221:171–178. doi:10.1016/j.fuel.2018.02.077.
- Li, Y. 2012. Dynamics of clean coal-fired power generation development in China. Energy Policy 51 (6):138–142. doi:10.1016/j.enpol.2011.06.012.
- Li, Z. G., X. B. Feng, G. H. Li, R. S. Yin, and B. Yu. 2012. Mass balance and isotope characteristics of Mercury in two coal-fired power plants in Guizhou, China. Adv. Mater. Res. 518-523:2576–2579. doi:10.4028/www.scientific.net/AMR.518-523.
- Loewen, M., S. Kang, D. Armstrong, Q. Zhang, A. Gregg Tomy, and F. Wang. 2007. Atmospheric transport of Mercury to the Tibetan Plateau. Environ. Sci. Technol. 41 (22):7632. doi:10.1021/es0710398.
- Meng, Y., and S. Wang. 2012. Study on mercury re-emissions during fly ash utilization (in Chinese). Environ. Sci. 39 (6):2993–2999.
- Noel, J. D., P. Biswas, and D. Giammar. 2007. Evaluation of a sequential extraction process used for determining mercury binding mechanisms to coal combustion byproducts, 57:856–867.
- Pacyna, E. G., J. M. Pacyna, F. Steenhuisen, and S. Wilson. 2006. Global anthropogenic mercury emission inventory for 2000. Atmos. Environ. 40 (22):4048–4063. doi:10.1016/j.atmosenv.2006.03.041.
- Pacyna, J. M., O. Travnikov, F. De Simone, I. M. Hedgecock, K. Sundseth, E. G. Pacyna, F. Steenhuisen, N. Pirrone, J. Munthe, and K. Kindbom. 2016. Current and future levels of mercury atmospheric pollution on a global scale. Atmos. Chem. Phys. 16:1–35. doi:10.5194/acp-16-12495-2016.
- Pavlish, J. H., E. A. Sondreal, M. D. Mann, E. S. Olson, K. C. Galbreath, D. L. Laudal, and S. A. Benson. 2003. Status review of mercury control options for coal-fired power plants. Fuel Process. Technol. 82 (2):89–165. doi:10.1016/S0378-3820(03)00059-6.
- Qin, Y., T. A. Moore, J. Shen, Z. Yang, Y. Shen, and G. Wang. 2017. Resources and geology of coalbed methane in China: A review. Int. Geol. Rev 1:1–36.
- Qiu, G., X. Feng, S. Wang, and L. Shang. 2006. Environmental contamination of mercury from Hg-mining areas in Wuchuan, northeastern Guizhou China. Environ. Pollut. 142 (3):549–558. doi:10.1016/j.envpol.2005.10.015.
- Riget, F., R. Dietz, P. Johansen, and G. Asmund. 2000. Lead, cadmium, mercury and selenium in Greenland marine biota and sediments during AMAP phase 1. Sci. Total Environ. 245 (1–3):3. doi:10.1016/S0048-9697(99)00429-5.
- Seigneur, C., K. Vijayaraghavan, K. Lohman, A. Prakash Karamchandani, and C. Scott. 2004. Global source attribution for mercury deposition in the United States. Environ. Sci. Technol. 38 (2):555–569. doi:10.1021/es034109t.
- Senior, C. L., A. F. Sarofim, T. Zeng, J. J. Helble, and R. Mamani-Paco. 2000. Gas-phase transformations of mercury in coal-fired power plants. Fuel Process. Technol. 63 (2):197–213. doi:10.1016/S0378-3820(99)00097-1.
- Shuxiao, W., and Jiming. 2012. Air quality management in China: Issues,challenges,andoptions. Acta Sci. Circum 24 (1):2–13.
- Shuxiao, W., Z. Xing, J. Carey, and Jiming. 2014. Effectiveness of national air pollution control policies on the air quality in metropolitan areas of China. J. Environ. Sci. 26 (1):13–22. doi:10.1016/S1001-0742(13)60381-2.
- Srivastava, R. K., N. Hutson, B. Martin, F. Princiotta, and J. Staudt. 2006. Control of mercury emissions from coal-fired electric utility boilers. Environ. Sci. Technol. 40 (5):1385. doi:10.1021/es062639u.
- Streets, D. G., J. Hao, Y. Wu, J. Jiang, M. Chan, H. Tian, and X. Feng. 2005. Anthropogenic mercury emissions in China. Atmos. Environ. 39 (40):7789–7806. doi:10.1016/j.atmosenv.2005.08.029.
- Tang, S., L. Wang, X. Feng, Z. Feng, R. Li, H. Fan, and K. Li. 2016. Actual mercury speciation and mercury discharges from coal-fired power plants in Inner Mongolia, Northern China. Fuel 180:194–204. doi:10.1016/j.fuel.2016.04.037.
- Tang, S., X. Feng, J. Qiu, G. Yin, and Z. Yang. 2007. Mercury speciation and emissions from coal combustion in Guiyang, Southwest China. Environ. Res. 105 (2):175–182. doi:10.1016/j.envres.2007.03.008.
- Tao, S., C. Li, X. Fan, G. Zeng, P. Lu, X. Zhang, Q. Wen, W. Zhao, D. Luo, and C. Fan. 2012. Activated coke impregnated with cerium chloride used for elemental mercury removal from simulated flue gas. Chem. Eng. J. 210 (210):547–556. doi:10.1016/j.cej.2012.09.028.
- Temme, C., J. W. Einax, R. Ebinghaus, and W. H. Schroeder. 2003. Measurements of atmospheric mercury species at a coastal site in the Antarctic and over the south Atlantic Ocean during polar summer. Environ. Sci. Technol. 37 (1):22. doi:10.1021/es025884w.
- Tian, H., Y. Wang, Z. Xue, Y. Qu, F. Chai, and J. Hao. 2011. Atmospheric emissions estimation of Hg, As, and Se from coal-fired power plants in China, 2007. Sci. Total Environ. 409 (16):3078–3081. doi:10.1016/j.scitotenv.2011.04.039.
- Wang, Q., A. Wenguo Shen, and Z. Ma. 2000. Estimation of mercury emission from coal combustion in China. China Environmentalence 34 (13):2711–2713.
- Wang, S., J. Xing, B. Zhao, C. Jang, and J. Hao. 2014. Effectiveness of national air pollution control policies on the air quality in metropolitan areas of China. Acta Sci. Circum 26 (1):13–22.
- Wdowin, M., M. Macherzyński, R. Panek, J. Górecki, and W. Franus. 2015. Investigation of the sorption of mercury vapour from exhaust gas by an Ag-X zeolite. Clay. Miner. 50 (1):31–40. doi:10.1180/claymin.2015.050.1.04.
- Wdowin, M., M. M. Wiatros-Motyka, R. Panek, L. A. Stevens, W. Franus, and C. E. Snape. 2014. Experimental study of mercury removal from exhaust gases. Fuel 128 (28):451–457. doi:10.1016/j.fuel.2014.03.041.
- Wiatros-Motyka, M. M., C. G. Sun, L. A. Stevens, and C. E. Snape. 2013. High capacity co-precipitated manganese oxides sorbents for oxidative mercury capture. Fuel 109 (7):559–562. doi:10.1016/j.fuel.2013.03.019.
- Withum, J. A. 2004. EVALUATION OF MERCURY EMISSIONS FROM COAL-FIRED FACILITIES WITH SCR AND FGD SYSTEMS. Off. Scientific Tech. Inf. Tech. Rep. doi:10.2172/888537
- Wu, J., Y. Cao, W. Pan, and W. Pan. 2015. The status of mercury emission from coal combustion power station, Springer Berlin Heidelberg, 19–30.
- Wu, Y., D. G. Streets, S. X. Wang, and J. M. Hao. 2010. Uncertainties in estimating mercury emissions from coal-fired power plants in China. Atmos. Chem. Phys. 9 (6):2937–2946. doi:10.5194/acp-10-2937-2010.
- Wu, Y. L., D. G. Rahmaningrum, Y. C. Lai, L. K. Tu, S. J. Lee, L. C. Wang, and G. P. Chang-Chien. 2012. Mercury emissions from a coal-fired power plant and their impact on the nearby environment. Aerosol Air Qual. Res. 12 (4):643–650. doi:10.4209/aaqr.2012.04.0080.
- Xu, M., Y. Qiao, C. Zheng, L. Li, and J. Liu. 2003. Modeling of homogeneous mercury speciation using detailed chemical kinetics. Combust. Flame 132 (1–2):208–218. doi:10.1016/S0010-2180(02)00438-8.
- Yang, Y., Q. Huang, and Q. Wang. 2012. Ignoring emissions of Hg from coal ash and desulfurized gypsum will lead to ineffective mercury control in coal-fired power plants in China. Environ. Sci. Technol. 46 (6):3058. doi:10.1021/es300786d.
- Yokoyama, T., K. Asakura, H. Matsuda, S. Ito, and N. Noda. 2000. Mercury emissions from a coal-fired power plant in Japan. Sci. Total Environ. 259 (1):97–103.
- Yueyang, X., X. Jianming, W. Hongliang, L. Bing, G. Yiming, and L. Jun. 2014. Research on Mercury collaborative control by conventional pollutants purification facilities of coal-fired power plants. Proc. CSEE 34 (23):3924–3931.
- Zhang, J. Y., C. G. Zheng, D. Y. Ren, C. L. Chou, J. Liu, R. S. Zeng, Z. P. Wang, F. H. Zhao, and Y. T. Ge. 2004. Distribution of potentially hazardous trace elements in coals from Shanxi province, China. Fuel 83 (1):129–135. doi:10.1016/S0016-2361(03)00221-7.
- Zhang, L., and M. H. Wong. 2007. Environmental mercury contamination in China: Sources and impacts ☆. Environ. Int. 33 (1):108–121. doi:10.1016/j.envint.2006.06.022.
- Zhang, L., S. Wang, Y. Meng, and J. Hao. 2012. Influence of Mercury and chlorine content of coal on mercury emissions from coal-fired power plants in China. Environ. Sci. Technol. 46 (11):6385. doi:10.1021/es300286n.
- Zou, R., X. Zeng, G. Luo, Y. Qiu, B. Zhang, Y. Xu, H. Wu, and H. Yao. 2016. Mercury stability of byproducts from wet flue gas desulfurization devices. Fuel 186:215–221. doi:10.1016/j.fuel.2016.08.023.