ABSTRACT
An ethylene/air inverse diffusion flame (IDF) burner was employed to generate a stable flame, and selenium was introduced into the combustion flame in vapor phase under different air-fuel ratio (A/F) with SO2 additive. At different height above burner (HAB) along the flame edge, selenium of different speciation (gaseous selenium and particulate selenium) was sampled via the U.S. EPA method 29, and the samples were determined by hydride generation atomic fluorescence spectrometry (HG-AFS), in order to study the mechanism of transformation and enrichment behavior of selenium during the combustion process. The results showed that selenium presented in vapor phase, crossing the flame into air, which means gaseous phase is the main form of selenium during combustion process. Both gaseous selenium and particulate selenium increased with elevated temperature from 820K to 1650K, suggesting that higher temperature is beneficial to the release of selenium. Low concentration of sulfur dioxide would increase the concentration of particulate selenium and gaseous selenium, and accelerate the release of selenium.
Implications: The enrichment behavior of selenium and its transformation in combustion flame were studied. The results showed that gaseous selenium is found in higher quantity in compared to particulate selenium during combustion. Higher temperature and air–fuel ratio will cause an increase in the formation of selenium. While the presence of sulfur dioxide in a range of 0–200 ppm will promote the release of selenium, higher sulfur dioxide level in a range of 200–350 ppm will have a reverse effect.
Introduction
Emissions from coal-fired power plants, including particles, sulfur oxides, nitrogen oxides, carbon dioxide, and volatile organic pollutants, pose an adverse threat to human health and environment. In addition, 11 trace elements, namely, As, Be, Cd, Co, Cr, Hg, Mn, Ni, Pb, Sb and Se emitted from coal-fired power plants are among the 189 hazardous air pollutants (HAPs) identified in the 1990 Clean Air Act Amendments (CAAA) (U.S. Environmental Protection Agency Citation2003).
The research paper focuses mainly on selenium emissions. The coal-fired combustion process is the world’s largest anthropogenic source of selenium (Nriagu and Pacyna Citation1988). Selenium is mostly discharged directly in gaseous form. It is later adsorbed and enriched in fine fly ash particulates. Its biological accumulation causes detrimental effects to human health and the ecological environment. It causes respiratory-tract disease, cardiovascular disease, and even cancer (Bilos et al. Citation2001; Finkelman et al. Citation2002; Jarup Citation2003; Marilena and Elias Citation2008).
Although there are many studies on selenium in coal-fired combustion, most of them are about the selenium extracted from ashes, such as the distribution of selenium in fly ash, the concentration of selenium in fine particles of submicrometer size, and the leaching characteristics and migration of selenium in coal and combustion products (Codling and Wright Citation1998; Galbreath et al. Citation2000; Karuppiah and Gupta Citation1997; Senior et al. Citation2000). Very little is known about the transformation behavior and enrichment characteristics of selenium in the combustion flame.
Soot is a kind of fine particles generated during the incomplete combustion of fuels. A gas burner is an experimental apparatus that is widely used to study the mechanism, the transformation, and the characteristics of soot in combustion flames. There are currently two types of gas burners: normal diffusion flame (NDF) burner and inverse diffusion flame (IDF) burner (Lu, Ren, and Cao Citation2016). In this research, an ethylene/air IDF burner was employed to generate soot in a stable combustion flame, and selenium was introduced into the flame in the vapor phase under different combustion conditions; then its different speciation was sampled using U.S. EPA Method 29, and determined by hydride generation atomic fluorescence spectrometry (HG-AFS), in order to study the mechanism of transformation and enrichment behavior of selenium during the combustion process. In addition, as a main air pollutant in flue gas, sulfur dioxide was also added in this experimental study to investigate its effect on transformation and enrichment of selenium in the combustion flame.
Experimental
Experimental setup
The experimental setup schematic is shown in . It consists of a combustion system, a selenium vapor generation system, a product sampling system, and a sample digestion and determination system.
Figure 1. Experimental system schematic. 1. Nitrogen gas; 2. Ethylene; 3.Air; 4.Sulfur dioxide; 5.Selenium solution; 6.Micro peristaltic pump; 7.Rotor flow meter; 8.Quartz U-tube; 9. Thermostatic oil bath 10. IDF burner; 11.Air flow uniformplate; 12.Exhaust filter; 13.Quartz fiber filter; 14. Two-dimensional lift platform; 15.1 Absorption bottle (empty); 16.2 Absorption bottle (5% HNO3 + 10% H2O2); 17.3 Absorption bottle (silicone); 18.Ice bath; 19.Vacuum pump.
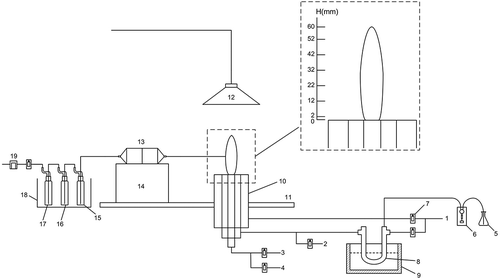
The IDF burner has been described in detail elsewhere (Blevins et al. Citation2002; Lu and Weng Citation2011). The burner consists of three concentric brass tubes: an inner tube (11 mm ID) to provide air and SO2 additive, an intermediate tube (35 mm ID) to supply ethylene and selenium vapor, and an outer tube (73 mm ID) to be used as a shield for the nitrogen stream to maintain the flame in stable combustion. The lower end of the burner was designed as a ladder structure, where the longest inner cylinder was supplied with air, and the outer two layer cylinders were gradually shortened. A fume hood was built above the burner and an exhaust filter was arranged to clean the gas emission.
Soot samples were taken at different positions using a sampling probe with 1-mm-ID capillary tip. The sampling points were set 5.5 mm away from the flame centerline along the flame edge (namely, at the outer edge of the inner tube), and relative height above burner (HAB) at 2, 12, 22, 32, 42, 52, and 60 mm, separately. The flow rates of air, nitrogen, and nitrogen carrier gas were kept at 1.5 L/min, 10 L/min, and 0.4 L/min respectively. The flow rate of ethylene was obtained at different air–fuel (A/F) ratios of 0.6, 0.8, and 1.0. After 20 min of sampling, the filters were weighted and the soot samples were analyzed. The A/F ratio was kept at 0.6, while the sulfur dioxide (SO2) was introduced into the air inlet in different concentrations to study the effect on release of selenium during combustion.
Selenium vapor generation system
A selenium vapor generation system was built to introduce selenium into the IDF burner for combustion. The system consists of a quartz U-shaped tube, an oil bath, selenium solution, a micro peristaltic pump, carrier gas, and other components. The quartz U-shaped tube was placed in the constant-temperature oil bath for heating to generate selenium vapor. In each combustion experiment, the selenium solution (~30 mL) was injected into the sealed quartz U-tube using a micro peristaltic pump. The injection rate was approximately 1.5 mL/min. The selenium standard solution (16.7%) was prepared according to the national standard solution (GBW (E) 080215, 100 μg/mL), which was gradually diluted to 0.1 μg/mL for selenium solution. The selenium solution in the U-shaped tube was released as a selenium vapor when the oil bath was heated to 230°C. With a certain rate of nitrogen as carrier gas, the selenium vapor was carried to the burner, and ethylene was mixed with it before flowing into the middle part of the burner, so as to achieve the combustion of selenium in the IDF burner.
Hydride generation atomic fluorescence spectrometry
HG-AFS has been widely used in the analysis of trace elements and was used to measure the samples at different heights in the flame (Chen, Yuan, and Wang Citation2012; Wu Citation2004). The carrier liquid for AFS consisted of HCl and KBH4, which can react to generate hydrogen. Se(IV) in the solution reacts with hydrogen to form H2Se. H2Se was carried into the atomizer by argon for atomization. Considering that HG-AFS cannot detect Se(VI) directly, Se(VI) has to be reduced to Se(IV) first and then be detected. Under the irradiation of a selenium hollow cathode lamp, the selenium atoms in the ground state are excited to the high energy state. During deactivation and returning to the ground state, they will emit fluorescence with its characteristic wavelength, resulting in a peak-like signal with its fluorescence intensity in the sample. The proportion of selenium is directly proportional to the selenium standard solution, and the selenium content in the sample solution can be calculated (NSPRC, Citation2010; Tang Citation2013).
Results and discussion
Effect of temperature and A/F ratio on the release behavior of particulate matter
To further confirm the effect of changes in oxidizing atmosphere, the air–fuel ratio (A/F), on the transformation behavior and enrichment characteristics of selenium, the A/F ratio was adjusted from 0.6 to 1.0. shows the relations among the flame temperature, the A/F ratio, and the concentration of soot particles in the flame. Under the same A/F ratio the particle concentration increased while the flame temperature decreased from 1650ºC (at 12 mm HAB) to 820ºC (at 42 mm HAB). It is apparent that a high temperature is feasible for complete combustion of hydrocarbon fuels, and reduces soot particle emissions. Since the ethylene cracking reaction occurs in the lower part of the flame, the rearranged atoms combine to form a large number of particles with small size, and the larger condensed particles that could be captured easily have not been generated. However, it is anticipated that the soot particles experience a condensation process with the increase of the HAB, so the soot particles grow and the volume of the particles gradually increases. Therefore, it appears that more particles can be trapped by the filter.
The effect of the A/F ratio upon the release of particulate was further examined. For the same flame height, the particulate emission decreased with an increase in the A/F ratio from 0.6 to 1.0. The reduction in the concentration of particulates was greatly dependent on the difference of the A/F ratio. The concentration of particulates decreased with an increase in the flame temperature. Consequently, the increase of combustion temperature and A/F ratio can effectively reduce the emission of soot particle in the combustion process.
Distribution characteristics of particulate selenium and gaseous selenium under different A/F ratios
In this paper, particulate selenium (Se(P)) refers to selenium enriched in the soot particles on the quartz fiber filter membrane, and gaseous selenium (Se(G)) refers to the part of selenium absorbed by the absorbing liquid. It can be seen from that the concentration of Se(P) in the flame is negatively correlated with the height of flame. In order to study the distribution characteristics of Se(P) and Se(G), three different A/F ratios were tested. The concentrations of Se(P) and Se(G) in the flame captured under different A/F ratios are shown in . Under three A/F ratio conditions, the total amount released of Se(G) especially in the middle part of the flame was very much greater than that of the Se(P), indicating that the Se(G) is the main form for the migration and transformation process in the flame combustion.
Table 1. The release of particulate selenium and gaseous selenium in different A/F ratios.
The concentration of Se(P) obtained under each A/F ratio is shown in . It shows that Se(P) for A/F ratio of 0.6 tended to be lower than those for A/F ratio of either 0.8 or 1.0. In addition, as the flame temperature became higher, the concentration of Se(P) increased, suggesting that more Se(P) can be generated at a higher temperature.
The migration and transformation of selenium vary greatly at different A/F ratios. The law of release is obvious. shows the concentration of Se versus HAB at different A/F ratios. The temperature decreases with an increase of the HAB. With the A/F ratio increasing from 0.6 to 1.0, the concentration of Se increases slightly. The rate of selenium generated in the flame is mostly determined by the temperature and A/F ratio. The former is decided by the HAB and the latter is related to the flow rate of ethylene and air used. In general, higher flame temperatures and higher A/F ratio can lead to an increase in the rate of selenium generated in the flame. This is because the higher flame temperature not only accelerates the evaporation rate of selenium, but also contributes to the complete combustion of the fuel, while the higher A/F ratio enhances the oxidizing additive of the combustion process. Meanwhile, this mostly like improves the combustion efficiency of the fuel, so that the reaction between the soot particles become more intense, which results in the information of small particle size but large specific surface area of fine particles (Lu and Shi Citation2013; Xu et al. Citation2005), thereby increasing the selenium vapor enrichment capacity.
Influence of temperature on the transformation and enrichment characteristics of selenium
The flame temperature and concentration distribution of selenium were investigated at the A/F ratio of 0.6 (see ). The Se(G) only presented in the lower part of the flame (the high temperature combustion zone). This indicates that higher temperature is conducive to generating the Se(G). As the flame temperature rose, the concentration of Se(P) increased; however, this trend was not as obvious as that for Se(G). This explains from the side that the effect of temperature on the Se(G) generating is more significant.
Effect of sulfur dioxide on selenium migration and transformation
This experiment was implemented under the condition of A/F ratio set to 0.6, and particle samples were collected at three different concentrations of SO2 introduced: 0 ppm, 200 ppm, and 350 ppm separately. The results are shown in . shows that under the same SO2 concentration, the release of particulate matter in flame was negatively correlated with the flame temperature, indicating that the hydrocarbon fuel was higher under high temperature conditions. The property of being easy to burn completely was not affected by SO2 concentration.
For the concentration of selenium in the flame, compared with the 200 ppm sulfur dioxide condition, the concentration of Se(P) decreased drastically under the condition of 350 ppm, and none of the Se(P) was trapped at 12 and 22 mm HAB. Under both the conditions of 200 ppm and 350 ppm sulfur dioxide introduced, the concentration of Se(G) presented higher values, especially in the middle part of the flame (HAB = 12 mm, HAB = 22 mm), but more Se(G) was generated in the middle flame while less Se(G) presented in the upper flame (HAB = 32 mm, HAB = 42 mm) under the condition of sulfur dioxide at 350 ppm. The total selenium concentration showed higher values under the condition of 200 ppm sulfur dioxide than those of values for 350 ppm sulfur dioxide along the flame. The results are shown in .
Conclusion
The enrichment behavior of Se in particulate matter and its transformation in a combustion flame was studied, and the results confirmed the following findings:
Se(G) was formed in higher concentrations than Se(P) during combustion.
The concentration of soot particles in the flame decreased, while the generating rate of both Se(P) and Se(G) increased with an increase in A/F ratio.
Sulfur dioxide addition has an effect on the release of selenium in the flame. Generally, total selenium concentration showed higher values under the condition of 200 ppm sulfur dioxide than those for 350 ppm sulfur dioxide along the flame.
Additional information
Funding
Notes on contributors
Jianyi Lu
Jianyi Lu is a professor and Jingwen Lu, Xudan Ren, and Shuwei Zhang are master’s degree candidates at the School of Environmental Science and Engineering, North China Electric Power University, Baoding city, Hebei Province, People’s Republic of China.
References
- Bilos, C., J. C. Colombo, C. N. Skorupka, and M. J. Rodringuez Presa. 2001. Sources, distribution and variability of airborne trace metals in La Plata City area, Argentina. Environ. Pollut. 111 (1):149–158. doi:10.1016/S0269-7491(99)00328-0.
- Blevins, L. G., R. A. Fletcher, B. A. Benner, E. B. Steel, and G. W. Mulholland. 2002. The existence of young soot in the exhaust of inverse diffusion flames. Proc. Combust. Inst. 29 (2):2325–2333. doi:10.1016/S1540-7489(02)80283-8.
- Chen, D. G., Y. X. Yuan, and H. Y. Wang. 2012. Determination of selenium and tellurium in ultra-hard lead alloys by hydride generation atomic fluorescence spectrometry (HG-AFS) [in Chinese]. Chin. J. Inorg. Anal. Chem. 2:38–40+64.
- Codling, E. E., and R. J. Wright. 1998. Plant uptake of selenium, arsenic and molybdenum from soil treated with coal combustion byproducts. Fresenius Environ. Bull. 7 (1/2):118–125.
- Finkelman, R. B., W. Orem, V. Castranova, C. A. Tatu, H. E. Belkin, B. S. Zheng, H. E. Lerch, S. Vmaharaj, and A. L. Bates. 2002. Health impacts of coal and coal use, possible solutions. Int. J. Coal Geol. 50:425–443. doi:10.1016/S0166-5162(02)00125-8.
- Galbreath, K. C., D. L. Toman, C. J. Zygarlicke, and J. H. Pavlish. 2000. Trace element partitioning and transformations during combustion of bituminous and subbituminous U.S. Energy Fuels 14:1265–1279. doi:10.1021/ef000105n.
- Jarup, L. 2003. Hazards of heavy metal contamination. Br. Med. Bull. 68 (486):167–182.
- Karuppiah, M., and G. Gupta. 1997. Toxicity of and metals in coal combustion ash leachate. J. Hazard. Mater. 56 (1):53–58. doi:10.1016/S0304-3894(97)00034-4.
- Lu, J. Y., X. D. Ren, and L. Y. Cao. 2016. Studies on characteristics and formation of soot nanoparticles in an ethylene/air inverse diffusion flame. J. Energy Eng. 142 (3):1–8. doi:10.1061/(ASCE)EY.1943-7897.0000305.
- Lu, J. Y., and X. B. Shi. 2013. Physicochemical properties and formation mechanism of soot during biomass burning [in Chinese]. J. Fuel Chem. Technol. 10:1184–1190.
- Lu, J. Y., and Q. L. Weng. 2011. Distribution of characteristics of gas temperature and soot fraction volume in ethylene/air inverse diffusion flame [in Chinese]. Acta Chimica Sinica 69 (8):1011–1016.
- Marilena, K., and C. Elias. 2008. Human health effects of air pollution. Environ. Pollut. 151 (6):362–367. doi:10.1016/j.envpol.2007.06.012.
- National Standards of the People’s Repubilc of China, GB 5009, 93-2010. 2010. National food safety standards-determination of selenium in foods [in Chinese], 3.
- Nriagu, J. O., and J. M. Pacyna. 1988. Quantitative assessment of world-wide contamination of air, water and soil by trace elements. Nature 333 (6169):134–139. doi:10.1038/333134a0.
- Senior, C. L., T. Zeng, J. Che, M. R. Ames, A. F. Sarofim, I. Olmez, F. E. Huggins, N. Shah, G. P. Huffman, A. Kolker, et al. 2000. Distribution of trace elements in selected pulverized coals as a function of particle size and density. Fuel Process. Technol. 63 (2):215–241. doi:10.1016/S0378-3820(99)00098-3.
- Tang, Q. 2013. Determination of copper, manganese, selenium by atomic fluoresecence spectrometry [in Chinese]. Nanning: Guangxi University.
- U.S. Environmental Protection Agency. 2003. EPA’s fourth external review draft of air quality criteria for particulate matter. USA: BiblioGov.
- Wu, C. 2004. Determination of cadmium in solid by hydride generation atomic fluorescence spectrometry [in Chinese]. Spectrosc. Spectral Anal. 23 (5):990–992.
- Xu, W. D., R. S. Zeng, D. N. Ye, and X. Querol. 2005. Distribution and environmental impacts of selenium in wastes of coal from a power plant [in Chinese]. Environ. Sci. 2:64–68.