ABSTRACT
Limitations of the toxicity characteristic leaching procedure (TCLP) for simulating pollutant leaching from wastes disposed of in full-scale landfills are well understood in the waste management profession; the TCLP solution has a lower pH and greater organic acid content than typical landfill leachate. The TCLP serves its intended regulatory objective, however, as long as a conservative estimate of leaching is provided. Here, we examine TCLP’s ability to represent worst-case leaching conditions for monofilled municipal solid waste incineration (MSWI) ash. A critical examination of TCLP’s applicability to MSWI ash is especially relevant, as ash management at MSWI facilities often centers on passing TCLP, regardless of environmental risk posed by the ash or its recyclability. Multiple batch leaching tests were conducted on different MSWI ash streams: mixed ash, fly ash, and different size fractions of bottom ash. Batch-test results were compared with leachate simulating MSWI ash monofills. The TCLP did not consistently provide the most conservative estimate of leaching, supporting the need to consider alternative methodologies in future regulatory development.
Implications: This paper analyzes the existing hazardous waste regulatory testing requirement for municipal solid waste incinerator (MSWI) ash management to evaluate whether the TCLP serves its intended purpose in providing the most conservative estimate of landfilled MSWI ash. The results will serve as guidance and motivation for policy makers and the regulatory community to reevaluate the TCLP’s application for characterizing MSWI ash leaching in certain disposal scenarios and could promote consideration of alternative testing procedures based upon results of this study. This study serves to promote representative and accurate quantification of leaching risk from MSWI ash.
Introduction
The vast majority of the ash produced at U.S. municipal solid waste incineration (MSWI) facilities is disposed of in lined landfills. The type of landfill required depends on whether the ash is characterized as a hazardous waste or not, a determination made using the toxicity characteristic leaching procedure (TCLP) (Bricka, Holmes, and Cullinane Citation1992; Kosson et al. Citation2002; U.S. Environmental Protection Agency [EPA] Citation1980, Citation1986, Citation1991). If one or more of the prescribed elements in the TCLP leachate are measured above the toxicity characteristic (TC) regulatory level, the ash must be managed in accordance with the hazardous waste treatment and disposal requirements of the Resource Conservation and Recovery Act (RCRA) (Bricka, Holmes, and Cullinane Citation1992; EPA Citation1986, Citation1992). As the cost of hazardous waste management is many times that of nonhazardous solid waste, MSWI facility operators go to great lengths to ensure their ash does not fail the TCLP.
The TCLP, modified from its predecessor the extraction procedure toxicity test (EP TOX) in the 1980s (Bricka, Holmes, and Cullinane Citation1992), utilizes an acetic acid solution designed to simulate plausible worst-case leaching conditions that might occur in a landfill containing putrescible waste (Bricka, Holmes, and Cullinane Citation1992; EPA Citation1995). Shortcomings of the TCLP are often highlighted, including its inability to accurately represent many disposal conditions, especially those where decomposing garbage is absent (Hooper et al. Citation1998; EPA Citation1999). Limitations of the TCLP have been acknowledged in several legal and regulatory interpretations. When industry challenged the applicability of the TCLP to determine the TC status of manufactured gas plant (MGP) waste because these wastes were not managed in municipal landfills, the courts ruled in favor of industry, leading the EPA to modify the definition of TC hazardous waste to exclude MGP waste (Kosson et al. Citation2002; EPA Citation1991, Citation1999). In proposed rules for managing lead-based paint (LBP) debris, TCLP results on LBP were used to assess potential lead leachability in municipal waste landfills, but synthetic precipitation leaching procedure (SPLP; similar to the TCLP, but using synthetic rainwater instead of acetic acid) results were used when considering construction and demolition debris (CDD) landfills (Powell et al. Citation2015).
Partly motivated by limitations of the TCLP, the EPA recently promulgated a new suite of leaching procedures, referred to as the Leaching Environmental Assessment Framework (LEAF) (Hattaway, Hardin, and Daniels Citation2013; EPA Citation2013). The use of these new methods has not been integrated into the RCRA regulatory framework, but they have been used to support rulemaking, most notably as a testing procedure in the development of the U.S. coal combustion residual regulations that went into effect in 2015 (Code of Federal Regulations Citation2015). Several potential outcomes for integrating these new leaching procedures into regulations or policy are envisioned. One possibility is that the U.S. hazardous waste regulatory structure will be amended such that alternative leaching procedures will replace the TCLP for some wastes and/or disposal conditions. Another plausible alternative is that the TCLP will remain as a conservative screening method to determine TC status, and that the LEAF methods or other techniques (e.g., SPLP) will serve as tools to evaluate risk for beneficial use determinations of nonhazardous solid waste.
The research presented in this study tests the hypothesis that the TCLP provides a conservative estimate of leaching concentrations for monofilled MSWI ash. Even if TCLP fails to mimic the complexities of pollutant leaching in landfill environments, it still meets its intended regulatory objective as long as it allows for a conservative screening of solid wastes that require additional management as hazardous waste (Bricka, Holmes, and Cullinane Citation1992; EPA Citation1995). An examination of whether the existing TCLP approach provides a conservative leaching estimate is important for many wastes and waste disposal scenarios, but it is especially relevant to MSWI ash. MSWI ash is commonly disposed of in monofills in the absence of decomposing waste. MSWI facility operators place so much emphasis on passing TCLP that they may in fact unintentionally generate an ash with elevated risk.
MSWI ash samples from three different facilities were tested using the TCLP (both fluid 1 and fluid 2) and SPLP. These results are compared with those of EPA LEAF Method 1314, a column test that arguably provides the most reflective laboratory representation of leaching conditions in a MSWI ash monofill. The objective of this research is to assess how well the TCLP serves as a conservative screening tool for MSWI ash and not to explore in detail the chemical mechanisms responsible for leaching differences. The information gained is intended to add to the discussion that will come about if and when future changes to the U.S. hazardous waste regulatory system are debated.
Materials and methods
Facility description and sample collection
Samples of MSWI bottom ash (BA), fly ash (FA), and a mixture of bottom ash and fly ash (mixed ash; MA) were collected from three different MSWI facilities in Florida, USA. MSWI bottom ash samples were collected in two different size fractions from one facility: particle sizes greater than 9.5 mm (BA-GT) and particle sizes less than 9.5 mm (BA-LT), as these size fractions represented two distinct material streams that could potentially be incorporated into different beneficial use applications. The bottom ash samples had been subjected to ferrous and nonferrous metals recovery. A grab sample of mixed ash was collected from a second facility as the ash was being readied for transport to an ash monofill (following quenching, mixing, and ferrous/nonferrous metals recovery). Fly ash from a third facility was directly collected from a MSWI facility’s air pollution control (APC) equipment.
Batch leaching tests
TCLP was performed on the MSWI ash samples in accordance with EPA Method 1311 (EPA Citation2015). The TCLP requires a pretest extraction fluid determination, which is based on the alkalinity of the waste. Extraction fluid 2 contains only acetic acid, whereas fluid 1 contains both acetic acid and sodium hydroxide and is prescribed for wastes exerting less alkalinity. All samples were tested using both TCLP extraction fluids. The SPLP was performed on all samples in accordance with EPA Method 1312, whereby samples were extracted in a synthetic rainwater created with dilutions of sulfuric acid and nitric acid (EPA Citation2015). To supplement discussion of leaching results and to illustrate differences in leaching as a function of pH, EPA Method 1313 was conducted on the BA-LT samples to explore general leaching trends. Method 1313 is a batch leaching test at a liquid-to-solid ratio (LS) of 10 that involves adding acid (nitric acid) or base (potassium hydroxide) until nine prescribed pH values (13.0 ± 0.5, 12.0 ± 0.5, 10.5 ± 0.5, 9.0 ± 0.5, 8.0 ± 0.5, 7.0 ± 0.5, 5.5 ± 0.5, 4.0 ± 0.5, and 2.0 ± 0.5) are reached (EPA Citation2015).
Column leaching
Up-flow percolation column testing was conducted in accordance with EPA Method 1314 (EPA Citation2015). A flow rate of approximately 0.75 LS per day was utilized in all column tests. The ash samples were not size reduced to preserve an as-disposed, undisturbed condition similar to what would exist in a monofill. To accommodate the increase in particle size while remaining consistent with the method and avoiding channeling, the column diameters were scaled based on a ratio of maximum nominal particle size (column diameter = 20× nominal maximum particle size). This resulted in the use of three different diameter columns: 5 cm (MSWI fly ash), 10 cm (MSWI BA <9.5 mm), and 30 cm (MSWI BA >9.5 mm and mixed MSWI ash).
Trace elemental analysis
Trace elemental analysis was conducted for 12 inorganic elements (arsenic, antimony, barium, cadmium, chromium, copper, lead, mercury, nickel, selenium, silver, zinc), using inductively coupled plasma atomic emission spectrometry (ICP-AES) (Thermo-Scientific model ICAP 6000; Waltham, MA, USA) in accordance with EPA Method 6010B (EPA Citation2015). Although it is a metal regulated by RCRA, Hg analysis was not included here, as it requires a separate analytical procedure and Hg is typically not a leaching issue for MSWI ashes (Buchholz and Landsberger Citation1995; Vogg et al. Citation1986). Non-TC elements are included because their leachability using TCLP may be important as part of waste treatment and disposal (discussed in subsequent sections of this paper). Prior to analysis, leachate samples were subjected to an acid digestion in accordance with EPA Method 3010A (EPA Citation2015).
Results and discussion
Comparison of leachate concentrations
All MSWI ash samples were analyzed for the total concentrations of seven TC elements (Ag, As, Ba, Cd, Cr, Pb, and Se) as well as other major inorganic elements (Al, Cu, Ni, Sb, and Zn) contained in the ashes. Total concentrations of the different ashes fell within typical ranges reported in the literature (Roessler et al. Citation2014), with the concentrations in the fly ash being highest for many elements.
Of the seven RCRA TC metals analyzed in the batch test and column test leachates, only Pb and Cd were found to exceed TC limits. These two elements are the most likely to exceed a TC regulatory threshold (Kuo, Lin, and Wey Citation2008; Oehmig et al. Citation2015; Uberol and Shadman Citation1991). Currently in the United States, MSWI facility operators go to great lengths to make certain that their MSWI ash is not hazardous waste. They do this by ensuring that the ash mixture to be disposed of results in a TCLP extraction pH at which both of these metals leach under the TC regulatory level. Thus, the behavior of Pb and Cd from MSWI ash and leachability of these elements as a function of pH are well understood, and much of the discussion herein focuses on these two elements. Of the remaining TC elements tested, Ba and Cr were consistently detected, but well under TC limits. Se was observed in some samples. Ag and As were rarely detected and as such are not included in this analysis.
EPA Method 1314, an up-flow column test, is one of EPA’s new LEAF procedures and arguably the test that provides most reflective laboratory representation of leaching conditions in a MSWI monofill. Column test results showing Pb concentrations for all samples are presented in . A line representing the regulatory level for the toxicity characteristic is presented, and only the FA sample exceeded the TC level of 5.00 mg/L (11.1 mg/L), and only in the first leachate fraction collected.
Figure 1. Pb concentrations from four MSWI ash samples measured during Method 1314 leach testing. Results at each target LS represent the average of two column tests. Graph (a) represents Pb concentrations at each target LS. Graph (b) represents pH at each target LS for four MSWI ash samples. The toxicity characteristic limit for Pb has been displayed as a dashed line at 5 mg/L.
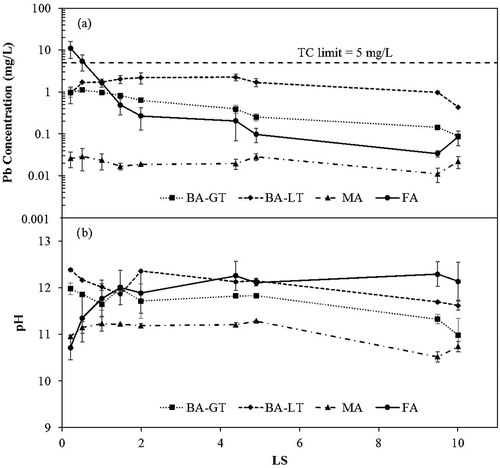
The FA sample, which had the highest total concentration of Pb (2576 mg/kg) (supplementary information, Table S1), displayed the greatest reduction in lead, over 2 orders of magnitude, during the Method 1314 procedure. This behavior is typical of “wash-off” elements that are not limited by solubility (de Groot and Van der Sloot, Citation1992; Van der Sloot Citation1990). The other ash samples exhibited more constant lead concentrations, characteristic of solubility-controlled elements. presents the Method 1314 results for Cd. Only the FA sample showed detectable Cd beyond the first sampling period, and even for this sample Cd leachate concentrations were below detection after a LS of 2.
Figure 2. Cd concentrations from four MSWI ash samples measured during Method 1314 leach testing. Results at each target LS represent the average of two column tests. Graph (a) represents Cd concentrations at each target LS. Graph (b) represents pH at each target LS for four MSWI ash samples. The toxicity characteristic limit for Cd has been displayed as a dashed line at 1 mg/L.
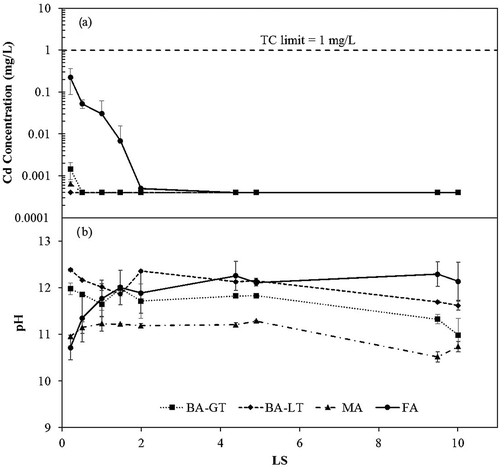
and present a comparison of leachate concentrations (for Pb and Cd, respectively) among the three batch tests and the Method 1314 procedure. SPLP, TCLP fluid 1, and TCLP fluid 2 concentrations correspond to samples on an “as-received” LS of 20 (the moisture content was not factored into the LS of the test), whereas the Method 1314 concentrations were measured in mg/L at nine separate LS points (ranging from 0.2 to 10). The average concentrations of the elements of interest from the column tests were calculated as the summation of element concentration (mg/L) in each collected leachate interval multiplied by the volume of that eluate fraction (mL) and divided by the total volume of nine eluate fractions (mL).
Figure 3. Comparison of Pb concentrations from different extraction solutions in four MSWI ash samples. For SPLP and TCLP, the average of triplicate measurements is presented along with standard deviation as the error bars. For Method 1314, the weighted average concentration is presented, while the error bars represent the minimum and maximum concentrations measured.
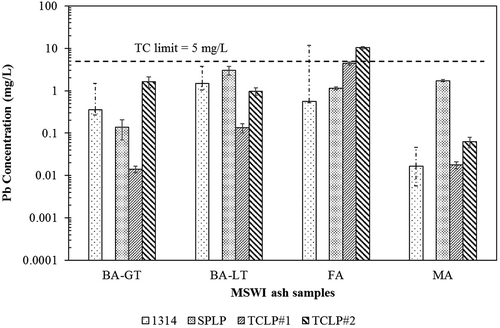
Figure 4. Comparison of Cd concentrations from different extraction solutions in four MSWI ash samples. For SPLP and TCLP, the average of triplicate measurements is presented along with standard deviation as the error bars. For Method 1314, the weighted average concentration is presented, while the error bars represent the minimum and maximum concentrations measured.
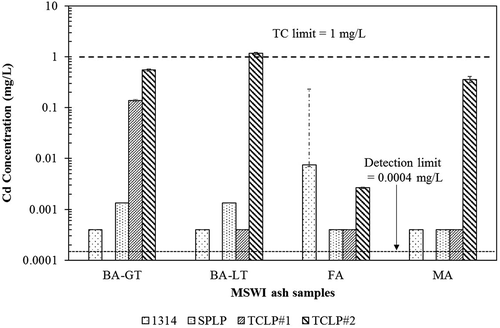
The lead concentrations measured by the four leaching procedures () vary among the same ash sample by over 2 orders of magnitude in some cases. Treating the highest concentration as the most “conservative” from a regulatory perspective, no single procedure consistently provided the most conservative results. In two of the samples (BA-GT and FA), TCLP fluid 2 results in the highest concentration, whereas SPLP results in the highest concentration in the other two (BA-LT and MA). In the BA-GT sample, the method required TCLP fluid was fluid 1, and TCLP fluid 1 resulted in the lowest concentration. In the FA samples, fluid 2 was the required extraction fluid. Thus, for lead, the required TCLP test provided the most conservative result in one of the four samples.
In the case of Cd, TCLP fluid 2 is the most conservative procedure in three of the four samples, but of those samples where fluid 2 was most conservative, it was the required TCLP fluid in only two of these three (BA-LT and MA) (). Cd concentrations ranged by over 3 orders of magnitude among the leaching procedures for individual samples. The one sample (FA) where TCLP fluid 2 was not the most conservative for Cd was, in fact, the only sample where it was most conservative for Pb.
Similar comparison plots are provided in the supplementary information for this paper for Cr, Ba, Se, Sb, Al, Cu, Ni, and Zn (Method 1314 plots are provided as well), as presented in Figure S1–S16, respectively. Results similar to Pb and Cd are observed for the other elements, with wide ranging concentrations, with no single leaching procedure consistently providing the highest concentration.
summarizes the comparison among leaching procedures. The relative order of concentrations is presented for 10 elements for each of the four ash materials. As extractions with both TCLP fluids were performed, the required TCLP as dictated by regulation is underlined. For no element was the prescribed TCLP most conservative for every ash. The prescribed TCLP was most conservative in three of four ashes for Sb and Zn, in two of four ashes for Cd and Ni, and in one of four ashes for Pb and Cu. For the elements Cr, Ba, Se, and Al, the prescribed TCLP never resulted in the greater concentration.
Table 1. Relative leachability comparison of trace metals (Pb, Cr, Ba, Cd, Se, Sb, Al, Cu, Ni, and Zn) for each leaching procedure (TCLP both fluid extractions, SPLP, and EPA Method 1314).
Controlling factors
The results measured for the four ashes examined in this study suggest that the hypothesis of the prescribed TCLP extraction resulting in the most conservative concentration is not true in a majority of cases. Given what is known about inorganic element leachability from ash, particularly the major role of solution pH (Dijkstra, Van der Sloot, and Comans Citation2006; Kosson et al. Citation2002; Quina, Bordado, and Quinta-Ferreira Citation2009; Van der Sloot, Comans, and Hjelmar Citation1996, Citation2001), we examined the results of Pb and Cd in more detail to determine whether the relative leaching among the different tests could be predicted based on extraction pH alone.
EPA Method 1313, another of the LEAF methods, was designed to examine the leaching of elements from waste as a function of pH. As described previously, the MSWI industry has developed a strong understanding of the leaching behavior of Pb and Cd as a function of pH. and present 1313 data combined for multiple MSWI ash samples from the authors’ laboratories to illustrate this well-understood pattern. Included on this plot are 1313 data for the BA-LT sample from this study. The concentration versus pH relationship for Pb follows an amphoteric behavior as evidenced by the U-shaped pattern where Pb concentrations are highest at the lower and higher pH ranges, and lowest in the pH range of 7–9. shows typical 1313 results for Cd, with high leachability at a pH of 5 and below, a dramatic decrease as pH increases from 5 to 7, and low or nondetectable Cd above a pH of 7. Thus, if the final pH of the solution is known, the relative amount (or order) of leaching occurring can be estimated.
Figure 5. Typical pH-dependent leaching relationship for Pb from EPA Method 1313 extractions of two MSWI BA samples from the authors’ laboratories. Data are presented as EPA Method 1313 extract pH versus lead concentration on a log scale. BA-LT samples from this study are denoted by shaded triangles. BA samples from a separate facility are denoted by unshaded circles. Lowest concentrations represent analytical detection limits.
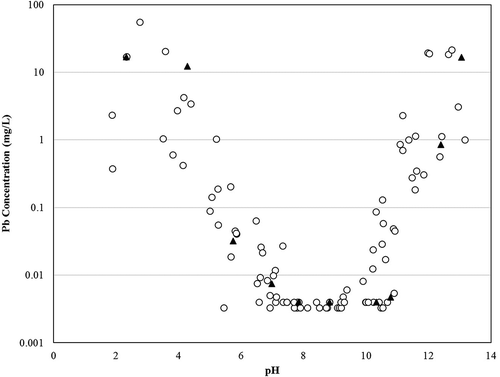
Figure 6. Typical pH-dependent leaching relationship for Cd from EPA Method 1313 extractions of two MSWI BA samples from the authors’ laboratories. Data are presented as EPA Method 1313 extract pH versus Cd concentration on a log scale. BA-LT samples from this study are denoted by shaded triangles. A collection of BA samples from two other facilities are denoted by unshaded circles or shaded diamonds. Lowest concentrations represent analytical detection limits.
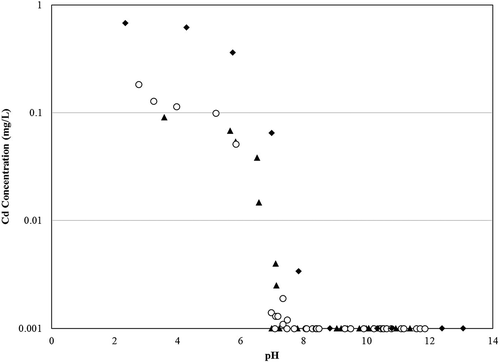
For the most part, the relative amount of leaching among the tests follows the expected pattern based on 1313 leaching trends. The final pH for every test and sample are provided in . As an example, when the final pH of the leaching tests for BA-GT sample are compared with the 1313 plot for Cd (), the predicted order of concentrations (based on extract pH) for the leaching tests (from highest to lowest) was TCLP fluid 2 > TCLP fluid 1 > SPLP ≈ Method 1314; this corresponded to the measured order of leaching tests in . The prediction of the order of conservatism for each leaching procedure for Pb was applied in the same manner as previously done for Cd by comparing the final leachate pH for every test for the BA-LT sample to the 1313 plot for Pb (). The order of the projected leaching procedures was SPLP > Method 1314 > TCLP fluid 1 > TCLP fluid 2. Three of the four orders of leaching tests, from this projection, corresponded to the measured order of leaching tests in .
Table 2. Summary of the final pH of the solutions for SPLP, TCLP fluid 1, TCLP fluid 2, and Method 1314 in ash samples (BA-GT, BA-LT, MA, and FA).
The 1313 relationships do not, however, predict results in all cases. In the case of Pb, two exceptions were observed. Leaching as a result of TCLP fluid tended to be greater than predicted by 1313 at a pH of less than 7. For example, when the final pH from every procedure for the BA-GT sample was compared with that of 1313 relatively for Pb, the relative order of leaching results was Method 1314 ≥ SPLP ≈ TCLP fluid 2 > TCLP fluid 1, but the measured concentrations from TCLP fluid 2 result in the highest Pb concentration (more than SPLP and 1314). A second difference noted for lead was that the TCLP leachability of the fly ash sample was greater than SPLP even though SPLP pH was higher. For example, when comparing the final pH from every leaching procedure for the FA sample with that of 1313 for Pb, the relative order of the predicted leaching was SPLP > TCLP fluid 1 > TCLP fluid 2 > Method 1314.
Implications and conclusion
The TCLP is generally thought in the United States to represent an aggressive and conservative leaching test. The test mimics leachate in a landfill with a lower pH and higher organic acid content than most MSW leachate; for these reasons, it is widely considered an appropriate screening tool to determine which solid wastes are sufficiently risky upon disposal to manage as hazardous. The TCLP has well-recognized concerns, and these limitations have been a major factor in the creation and use of other leaching tests, including the LEAF methodologies. However, despite these concerns, as long as the TCLP functions as a conservative predictor of leaching, the procedure still meets its intended purpose.
This research assessed whether leachate concentrations measured using TCLP represent a conservative leaching concentration when MSWI ash is disposed of in a monofill relative to other protocols. Monofilled MSWI ash provides a meaningful test case, since MSWI facility operators heavily focus on passing the TCLP and produce an ash that may unintentionally leach more in its true mode of disposition. Facility operators often add more lime to their acid-gas scrubbing operations so that the remaining ash falls within a pH range optimized to pass TCLP. Some facilities go so far as to add additional lime to the residuals (e.g., dolomitic lime); this extra lime plays no role in the air pollution control process and is added solely to meet pH targets for passing TCLP.
For many elements, the hypothesis that TCLP was most conservative for monofilled MSWI ash was not true; in some cases, the SPLP or the Method 1314 column procedures provided higher concentrations relative to TCLP. As described above, and has been examined in the body of literature on ash leaching, many factors dictate the magnitude of inorganic chemical leaching, and this varies among chemicals (de Groot and Van der Sloot Citation1992; Ghosh, Mukiibi, and Ela Citation2004; Kosson et al. Citation2002; Tang and Steenari Citation2016; EPA Citation1999; Verbinnen et al. Citation2017; Zhang et al. Citation2008). Although a critical examination of the factors contributing to leaching differences among the tests utilized was not a specific research objective, solution pH expectedly plays one of the most fundamental roles in controlling metal dissolution. When MSWI facility operators increase lime addition in an effort to pass TCLP, they provide pH buffering for acid conditions that will not occur when ash is monofilled; MSWI ash generated at a pH optimized for TCLP leachability may translate to greater leaching under true disposal conditions.
The results here demonstrate that the desire of MSWI facility operators to generate a solid waste classified as nonhazardous per U.S. regulatory definition may in practice create a waste with enhanced risk upon disposal (or reuse). These results support further investigating how tests such as Methods 1313 and Method 1314 can be integrated into the U.S. hazardous waste regulatory system. In the case of MSWI facilities, potential benefits of revised approaches extend beyond potential environmental implications. The use of additional chemicals adds to facility operational costs, and the presence of these additional chemicals may limit some recycling markets for the ash.
Results such as those presented here and in previous studies should provide motivation for the waste management industry and the regulatory community to continue to evaluate the role of TCLP for specific wastes and under certain disposal conditions, and to explore how alternative tests such as the LEAF methodologies can be used for hazardous waste characterization. For wastes that are not co-disposed with other materials (e.g., those disposed of in a monofill), Method 1314 should provide a better estimate of landfill leachate concentrations, whereas Method 1313 can be used to assess future leachability changes as wastes undergo natural weathering. For wastes co-disposed with MSW containing putrescible wastes, more research is needed to assess whether TCLP provides a conservative estimate. Finally, implications of reliance on the TCLP go beyond hazardous waste characterization; in the United States, hazardous waste treatment standards (the land disposal restrictions) for inorganic elements are based upon TCLP concentrations (Code of Federal Regulations Citation2012); treated hazardous waste would not likely be managed with biodegradable wastes and thus not be exposed to the acetic acid conditions of the TCLP.
Supplementary information
The supplementary information section contains leaching test results of seven RCRA elements, pH statistics for Cr, Ba, and Sb, and tabulated MSWI ash total concentration data. This material is available free of charge.
Supplemental Material
Download PDF (764.6 KB)Acknowledgment
The authors thank the MSWI facilities providing materials.
Supplemental data
Supplemental data for this paper can be accessed on the publisher’s website.
Additional information
Funding
Notes on contributors
Vicharana Intrakamhaeng
Vicharana Intrakamhaeng is an environmental scientist at the Pollution Control Department of Bangkok, Thailand, and a doctoral graduate of the University of Florida.
Kyle A. Clavier
Kyle A. Clavier is a graduate research assistant at the University of Florida in Gainesville, Florida
Justin G. Roessler
Justin G. Roessler is a postdoctoral research assistant at the University of Florida in Gainesville, Florida.
Timothy G. Townsend
Timothy G. Townsend is a professor of Environmental Engineering Sciences at the University of Florida in Gainesville, Florida.
References
- Bricka, R. M., T. T. Holmes, and M. J. Cullinane. 1992. A comparative evaluation of two extraction procedures: The TCLP and the EP. U.S. Environmental Protection Agency. Cincinnati, Ohio: Risk Reduction Engineering Laboratory.
- Buchholz, B. A., and S. Landsberger. 1995. Leaching dynamics studies of municipal solid waste incinerator ash. J. Air Waste Manage. Assoc. 45 (8):579–90. doi:10.1080/10473289.1995.10467388.
- Code of Federal Regulations. 2012. Universal treatment standards. Section 268.48, Title 40. doi:10.1094/PDIS-11-11-0999-PDN. Washington, DC: Office of the Federal Register.
- Code of Federal Regulations. 2015. Identification and listing of hazardous waste. Part 261, Title 40. Washington, DC: Office of the Federal Register.
- de Groot, G. J., and H. A. Van der Sloot. 1992. Determination of leaching characteristics of waste materials leading to environmental product certification. Stabilization Solidification Hazard. Radioactive Mixed Wastes 2:149–70. doi:10.1520/STP19548S.
- Dijkstra, J. J., H. A. Van der Sloot, and R. N. J. Comans. 2006. The leaching of major and trace elements from MSWI bottom ash as a function of pH and time. Appl. Geochem. 21 (2):335–51. doi:10.1016/j.apgeochem.2005.11.003.
- Ghosh, A., M. Mukiibi, and W. Ela. 2004. TCLP underestimates leaching of arsenic from solid residuals under landfill conditions. Environ. Sci. Technol. 38:4677–82. doi:10.1021/es030707w.
- Hattaway, J., C. D. Hardin, and J. L. Daniels. 2013. Recommended guidelines for the use and application of the Leaching Environmental Assessment Framework (LEAF) for coal combustion residuals. Paper presented at 2013 World of Coal Ash (WOCA) Conference, Lexington, Kentucky, April 22–25.
- Hooper, K., M. Iskander, G. Sivia, F. Hussein, J. Hsu, M. DeGuzman, Z. Odion, Z. Ilejay, F. Sy, M. Petreas, et al. 1998. Toxicity characteristic leaching procedure fails to extract oxoanion-forming elements that are extract by municipal solid waste leachates. Environ. Sci. Technol. 32 (23):3825–30. doi:10.1021/es980151q.
- Kosson, D. S., H. A. Van der Sloot, F. Sanchez, and A. C. Garrabrants. 2002. An integrated framework for evaluating leaching in waste management and utilization of secondary materials. Environ. Eng. Sci. 19 (3):159–204. doi:10.1089/109287502760079188.
- Kuo, J. H., C. L. Lin, and M. Y. Wey. 2008. Partitioning and emission characteristics of Pb and organics during fluidized bed thermal treatment of Municipal Solid Waste Incineration (MSWI) Fly Ash. Energy Fuels 22 (6):3789–97. doi:10.1021/ef800504q.
- Oehmig, W. N., J. G. Roessler, N. I. Blaisi, and T. G. Townsend. 2015. Contemporary practices and findings essential to the development of effective MSWI ash reuse policy in the United States. Environ. Sci. And Policy. 51:304–12. doi:10.1016/j.envsci.2015.04.024.
- Powell, J. T., P. Jain, J. Smith, T. G. Townsend, and T. M. Tolaymat. 2015. Does disposing of construction and demolition debris in unlined landfills impact groundwater quality? Evidence from 91 landfill sites in Florida. Environ. Sci. Technol. 49 (15):9029–36. doi:10.1021/acs.est.5b01368.
- Quina, M. J., J. C. M. Bordado, and R. M. Quinta-Ferreira. 2009. The influence of pH on the leaching behaviour of inorganic components from municipal solid waste APC residues. Waste Manage. 29 (9):2483–93. doi:10.1016/j.wasman.2009.05.012.
- Roessler, J. G., W. N. Oehmig, N. I. Blaisi, and T. G. Townsend. 2014. Chemical characterization of high-temperature arc gasification slag with a focus on element release in the environment. Environ. Sci. Technol. 48 (14):7781–88. doi:10.1021/es5007803.
- Tang, J., and B. M. Steenari. 2016. Leaching optimization of municipal solid waste incineration ash for resource recovery: A case study of Cu, Zn, Pb, and Cd. Waste Manage. 48:315–22. doi:10.1016/j.wasman.2015.10.003.
- U. S. Environmental Protection Agency. 1980. Hazardous waste management system: Identification and listing of hazardous waste. Fed. Regist. 45: 33084–137.
- U. S. Environmental Protection Agency. 1986. Hazardous waste management system; identification and listing of hazardous waste; notification requirements; reportable quantity adjustments; proposed Rule. Fed. Regist. 51: 21648–93.
- U. S. Environmental Protection Agency. 2015. Test methods for evaluating Solid Waste (SW-846). Washington, DC: U.S. EPA.
- U.S. Environmental Protection Agency. 1991. Leachability phenomena: Recommendations and rationale for analysis of contaminant release by the environmental engineering committee. United States Environmental Protection Agency, Science Advisory Board, EPA-SAB-EEC-92-003, 1–60. Washington, DC: US Environmental Protection Agency.
- U.S. Environmental Protection Agency. 1992. Method 1311: Toxicity characteristic leaching procedure. Washington, DC: US Environmental Protection Agency.
- U.S. Environmental Protection Agency. 1995. Applicability of the toxicity characteristic leaching procedure to mineral processing wastes, 1–3. Washington, DC: U.S. Environmental Protection Agency.
- U.S. Environmental Protection Agency. 1999. Waste leachability: The need for review of current agency procedures. Washington, DC: U. S. Environmental Protection Agency, EPA-SAB-EEC-COM-99-002S, 1–3.
- U.S. Environmental Protection Agency. 2013. Validated methods. Washington, DC: United States Environmental Protection Agency.
- Uberol, M., and F. Shadman. 1991. High temperature removal of cadmium using solid sorbents. Environ. Sci. Technol. 25:1285–89. doi:10.1021/es00019a009.
- Van der Sloot, H. A. 1990. Leaching behaviour of waste and stabilized waste materials; characterization for environmental assessment purposes. Waste Manage. Res. 8 (3):215–28. doi:10.1016/0734-242X(90)90065-U.
- Van der Sloot, H. A., R. N. J. Comans, and O. Hjelmar. 1996. Similarities in the leaching behaviour of trace contaminants from waste, stabilized waste, construction materials and soils. Sci. Total Environ. 178 (1):111–26. doi:10.1016/0048-9697(95)04803-0.
- Van der Sloot, H. A., D. S. Kosson, and O. Hjelmar. 2001. Characteristics, treatment and utilization of residues from municipal waste incineration. Waste Manage. 21 (8):753–65. doi:10.1016/S0956-053X(01)00009-5.
- Verbinnen, B., J. Van Caneghem, P. Billen, and C. Vandecasteele. 2017. Long term leaching behavior of antimony from mswi bottom ash: Influence of mineral additives of organic acids. Waste Biomass Valorization 8 (7):2454–552. doi:10.1007/s12649-016-9796-6.
- Vogg, H., H. Braun, M. Metzger, and J. Schneider. 1986. The specific role of cadmium and mercury in municipal solid waste incineration. Waste Manage. Res. 4:65–74. doi:10.1177/0734242X8600400109.
- Zhang, H., P. J. He, L.-M. Shao, and X.-J. Li. 2008. Leaching behavior of heavy metals from municipal solid waste incineration bottom ash and its geochemical modeling. J. Mater. Cycles Waste Manage. 10 (1):7–13. doi:10.1007/s10163-007-0191-z.