ABSTRACT
The association between particulate pollution and cardiovascular morbidity and mortality is well established. While the cardiovascular effects of nationally regulated criteria pollutants (e.g., fine particulate matter [PM2.5] and nitrogen dioxide) have been well documented, there are fewer studies on particulate pollutants that are more specific for traffic, such as black carbon (BC) and particle number (PN). In this paper, we synthesized studies conducted in the Greater Boston Area on cardiovascular health effects of traffic exposure, specifically defined by BC or PN exposure or proximity to major roadways. Large cohort studies demonstrate that exposure to traffic-related particles adversely affect cardiac autonomic function, increase systemic cytokine-mediated inflammation and pro-thrombotic activity, and elevate the risk of hypertension and ischemic stroke. Key patterns emerged when directly comparing studies with overlapping exposure metrics and population cohorts. Most notably, cardiovascular risk estimates of PN and BC exposures were larger in magnitude or more often statistically significant compared to those of PM2.5 exposures. Across multiple exposure metrics (e.g., short-term vs. long-term; observed vs. modeled) and different population cohorts (e.g., elderly, individuals with co-morbidities, young healthy individuals), there is compelling evidence that BC and PN represent traffic-related particles that are especially harmful to cardiovascular health. Further research is needed to validate these findings in other geographic locations, characterize exposure errors associated with using monitored and modeled traffic pollutant levels, and elucidate pathophysiological mechanisms underlying the cardiovascular effects of traffic-related particulate pollutants.
Implications: Traffic emissions are an important source of particles harmful to cardiovascular health. Traffic-related particles, specifically BC and PN, adversely affect cardiac autonomic function, increase systemic inflammation and thrombotic activity, elevate BP, and increase the risk of ischemic stroke. There is evidence that BC and PN are associated with greater cardiovascular risk compared to PM2.5. Further research is needed to elucidate other health effects of traffic-related particles and assess the feasibility of regulating BC and PN or their regional and local sources.
Introduction
The health effects of air pollution exposure range widely and include adverse pulmonary, cardiovascular, neonatal, and neurological outcomes (Dockery et al., Citation1993; Fleisch et al., Citation2015; Franklin, Zeka, and Schwartz, Citation2007; Kioumourtzoglou et al., Citation2016; Pope et al., Citation2002; Rice et al., Citation2015; Wilker et al., Citation2015). Since 1999, investigators at the Harvard Environmental Protection Agency (EPA) Center have conducted research to characterize air pollution exposures and sources, assess health effects at the molecular and physiological level, and identify factors that modify these health effects. In the past 15 years, traffic emissions have been identified as an important source of particle exposure that is associated with many adverse cardiovascular outcomes (Brook et al. Citation2010).
Traffic is a major source of both gaseous and particulate pollutants. Traffic-related particles with an aerodynamic diameter size da ≤ 2.5 μm (fine particles, PM2.5) are of significant public health concern, as they penetrate to the deep alveolar regions of the lungs and have been linked to numerous adverse health effects (Dockery et al. Citation1993; Hoek et al. Citation2002; Künzli et al. Citation2000; Laden et al. Citation2006; Lepeule et al. Citation2012; Seaton et al. Citation1995). As such, PM2.5 is among the criteria pollutants regulated by the U.S. EPA. Fine particles are formed as a result of fossil fuel combustion by motor vehicles and stationary sources such as power plants. As black carbon (BC) is a combustion by-product, BC may be a more precise proxy for traffic-related particles than PM2.5 (Laden et al. Citation2000a).
To assess the health effects of traffic pollutants, recent Harvard EPA Center studies have primarily utilized BC, particle number (PN), and proximity to major roadways as markers of traffic exposure. PN represents mostly particles with an aerodynamic diameter of less than 100 nm, or ultrafine particles (UFPs), which are predominantly freshly generated combustion particles. In comparison, BC particles are a mix of freshly generated UFPs (da<0.1 μm) and aged traffic particles (mostly in the accumulation mode; 0.1< da<1.0 μm) in the Boston metropolitan area (Kang, Koutrakis, and Suh Citation2010). BC is a marker of local and regional traffic pollutants and measured as the light-absorbing component of particulate matter. Distance to major roadways is a proxy for long-term exposure to traffic-related air pollution as well as acute exposures, given that individuals with high long-term residential traffic exposures are also more likely to have high acute exposures. In addition, distance to roadways has been shown to be a good proxy for outdoor concentrations of traffic-related air pollutants (Brauer et al. Citation2003; Hochadel et al. Citation2006; Hoek et al. Citation2001).
Investigators outside of the Harvard EPA Center have also assessed the effect of traffic-related pollution on cardiovascular health. However, many of these studies use less specific measures of traffic pollution, including PM10, ozone, carbon monoxide, nitrogen oxides, and sulfur dioxide (Bilenko et al. Citation2015; Foraster et al. Citation2014; Korek et al. Citation2015; Lanki et al. Citation2015; Shields et al. Citation2013; Sørensen et al. Citation2012). While these pollutants are of significant concern for public health, non-traffic sources also contribute substantially to these pollutants. In comparison, BC, PN, and proximity to major roadways represent more specific proxies for traffic particles. As such, we focus on comparing select studies that utilize these specific markers of traffic exposure in order to highlight salient findings on the effect of traffic-related particulate pollutants on cardiovascular health.
Studies assessing source-specific effects of particulate pollutants have demonstrated that traffic-related particles are associated with cardiovascular morbidity and mortality (Janssen et al. Citation2002; Laden et al. Citation2000b; Lanki et al. Citation2006). In fact, particles from traffic emissions were shown to be more strongly associated with cardiovascular mortality than particles from coal combustion in six U.S. cities (Laden et al. Citation2000b). In addition, the effect of PM10 exposure on the risk of cardiovascular hospital admissions has been found to vary with the proportion of traffic particles (Janssen et al. Citation2002). Therefore, it is important to elucidate the effects of primary traffic particles, such as BC and PN, on cardiovascular health.
Cardiovascular morbidity and mortality have been consistently associated with particulate pollutant exposure (Brook Citation2008; Brook et al. Citation2010; Miller et al. Citation2007; Pope et al. Citation2004). Mechanisms underlying these associations include systemic inflammation, oxidative stress, hypercoagulability, vascular dysfunction, atherosclerosis, and autonomic dysfunction. Studies from the Harvard EPA Center have demonstrated the impact of traffic-related air pollutants on these physiologic measures and associated cardiovascular outcomes. A broad literature search reveals that a significant proportion of large cohort studies utilizing traffic-specific markers such as BC and PN have been conducted by Harvard investigators. In this paper, we synthesize findings from large New England-based epidemiological studies that investigated the impact of traffic-related particle exposures on cardiovascular mortality, hypertension, ischemic stroke, heart rate variability, and molecular markers of endothelial dysfunction, inflammation, and thrombosis.
Cardiovascular mortality
Long-term exposure to particulate air pollution increases risk of cardiovascular mortality in the general population (Di et al. Citation2017; Dockery et al. Citation1993; Miller et al. Citation2007; Pope et al. Citation2002). Residential proximity to a major roadway has been utilized as a surrogate for long-term traffic exposure to demonstrate associations with all-cause mortality (Finkelstein, Jerrett, and Sears Citation2004; Hoek et al. Citation2002), cardiopulmonary mortality (Gehring et al. Citation2006; Hoek et al. Citation2002), stroke mortality (Maheswaran and Elliott Citation2003), sudden cardiac death (Hart et al. Citation2014), and fatal coronary heart disease (Hart et al. Citation2014). In addition, living near a major road has been associated with myocardial infarction (Hart et al. Citation2013), hypertension (Kingsley et al. Citation2015), impaired conduit artery and microvascular function (Wilker et al. Citation2014), vascular end-organ damage (Van Hee et al. Citation2009), atherosclerosis (Hoffmann et al. Citation2007), and elevated C-reactive protein (CRP) levels (Lanki et al. Citation2015).
Long-term traffic exposures are more strongly associated with fatal cardiovascular outcomes and post-discharge mortality compared to nonfatal events (Hart et al. Citation2014; Rosenlund et al. Citation2006, Citation2008; von Klot et al. Citation2009). The link between particulate pollution and cardiac death may be explained by cardiac electrical instability (Zanobetti et al. Citation2009), as cardiac repolarization abnormalities may increase the risk of ventricular arrhythmia and sudden death. In addition, PM2.5 and BC exposures have been associated with ventricular ectopy (Zanobetti et al. Citation2014) and ventricular fibrillation (Dockery et al. Citation2005), which both increase the risk of cardiovascular mortality.
The Harvard EPA Center investigators were among the first to assess the mortality risks of residential proximity to high-traffic roadways for survivors of heart failure (Medina-Ramón et al. Citation2008), ischemic stroke (Wilker et al. Citation2013), and acute myocardial infarction (AMI) (Rosenbloom et al. Citation2012). These studies reported high post-discharge mortality rates of 76% (1,055 out of 1,389 within 5 years) for heart failure, 56% (950 out of 1,683 within a median of 4.6 years) for ischemic stroke, and 30% (1,071 out of 3,547 within 10 years) for AMI. In all three studies, living closer to a high-traffic road was associated with higher post-discharge mortality risk (). Living within 100 m of a major road was associated with a 30% (95% confidence interval [CI]: 13%, 49%), 20% (95% CI: 1%, 43%), and 27% (95% CI: 1%, 60%) increased risk of mortality after acute heart failure, ischemic stroke, and AMI, respectively. In contrast, mortality risks among individuals living within greater distance-to-road categories (e.g., 100–200 m or 200–400 m) were not statistically significant. Of note, these studies differed in follow-up time, reference distance group, and definition of high-traffic road as summarized in .
Table 1. Description of roadway proximity studies assessing cardiovascular mortality risk.
Figure 1. Post-hospitalization mortality risk of roadway proximity among cardiovascular patients. Risk estimates are derived from Medina-Ramón et al. (Citation2008) (post-heart failure), Wilker et al. (Citation2013) (post-ischemic stroke), and Rosenbloom et al. (Citation2012) (post-acute MI).
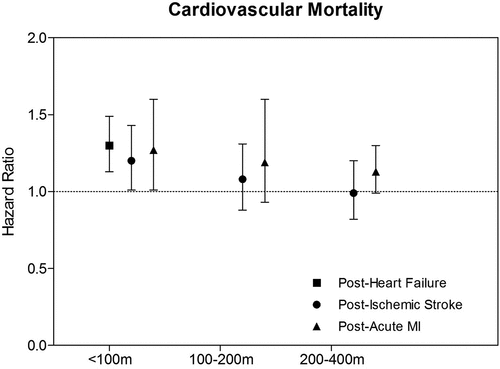
Roadway proximity is representative of both chronic and acute traffic pollutant exposures, as individuals with high chronic residential exposures to traffic-related pollutants are more likely to also have high acute exposures. However, there are important limitations of roadway proximity as a surrogate for traffic pollutant exposure. First, it is not possible to disentangle the impact of other aspects of near-roadway exposure, such as traffic noise, and estimate their relative contribution to cardiovascular risk. Second, without traffic volume data, it is assumed that exposures are similar within a given roadway classification, leading to non-differential exposure classification errors and underestimation of the associated risk. Third, socioeconomic status is a potential confounder given its association with both roadway proximity as well as survival after a serious cardiac event (Kaplan and Keil Citation1993). Lastly, additional exposure misclassification occurs without information on the amount of time spent at home and other home characteristics (e.g., soundproofing, topography, wind speed, and orientation relative to prevailing winds and to roadways). Despite these limitations, distance to roadway has been shown to be a good proxy for traffic-related air pollutant concentrations (Hoek et al. Citation2001; Janssen et al. Citation2002). A recent analysis of speciated PM2.5 data from over 650 indoor samples from 340 homes in the Greater Boston Area illustrated that indoor traffic-related particles increased with roadway proximity, demonstrating roadway proximity as a good proxy for personal exposure to traffic pollutants (Huang et al. Citation2018).
In summary, living closer to a major roadway is associated with an increased risk of mortality in patients with underlying cardiovascular disease. There are several mechanisms mediating the association between traffic pollutant exposure and mortality among cardiovascular patients, including cardiac autonomic dysfunction, atherosclerosis, inflammation, and oxidative stress. The following sections discuss the effects of traffic exposure on these cardiovascular outcomes in greater detail.
Heart rate variability
The relationship between air pollution exposure and adverse cardiovascular effects is thought to be mediated in part by autonomic cardiac dysfunction. Heart rate variability (HRV) is under the control of the autonomic nervous system and a reduction in HRV has been associated with myocardial infarction (Tsuji et al. Citation1996), ischemic events (Kop et al. Citation2001), atrial fibrillation (Bettoni and Zimmermann Citation2002), and sudden death (Odemuyiwa et al. Citation1991). Many studies have demonstrated the association between air pollution exposure and reduced HRV (Adam et al. Citation2014; Baja et al. Citation2013; Gold et al. Citation2000; Park et al. Citation2007, Citation2005; Pieters et al. Citation2012; Pope et al. Citation1999). Certain individuals appear to have greater susceptibility, including the elderly (Schwartz et al. Citation2005), those with pre-existing cardiovascular disease or diabetes (Zanobetti et al. Citation2010), and those with reduced anti-oxidative defenses (Chahine et al. Citation2007).
HRV is a physiological measure for cardiac autonomic tone that describes the variation of the time interval between heart beats, and there are a number of HRV indices that have been utilized by air pollution epidemiologists. HRV indices can be broadly divided into those that primarily indicate parasympathetic activity (e.g., high frequency [HF], root mean squared differences between adjacent RR intervals [r-MSSD], proportion of adjacent normal-to-normal heartbeat intervals differing by more than 50 ms [PNN50]), and those that predominantly reflect sympathetic activity (e.g., total power [TPow], standard deviation of normal-to-normal intervals [SDNN], low frequency [LF]). The predominance of sympathetic and/or diminished parasympathetic activity is believed to drive the association between HRV and adverse cardiovascular outcomes.
Harvard EPA Center studies have demonstrated a greater reduction in HRV associated with BC exposure compared to PM2.5 exposure among cardiovascular patients and elderly individuals. Zanobetti et al. (Citation2010) studied the effect of PM2.5 and BC exposure on HRV in 46 patients who were recently hospitalized in Boston for coronary artery disease, and lived within 40 km of the Harvard central monitoring site (). This study found that decreases in r-MSSD and HF–-measures of parasympathetic tone–-were associated with both PM2.5 and BC for exposure windows ranging from the current hour to the 5-day moving average. Notably, the effect sizes were larger for BC compared to PM2.5 exposure: a decrease in HF by 16.4% (95% CI: −20.7%, −11.8%) was associated with an interquartile range (IQR) increase in 5-day average BC, compared to a decrease of 6.9% (95% CI: −10.5%, −3.1%) for an IQR increase in 5-day average PM2.5. A panel study of 28 elderly Boston residents by Schwartz et al. (Citation2005) also reported that BC exposure had the largest association with HRV reduction among PM2.5 and other air pollutants (including secondary PM, ozone [O3], sulfur dioxide [SO2], nitrogen dioxide [NO2], and carbon monoxide [CO]). illustrates that associations between HRV reduction and BC were more often statistically significant and larger in magnitude than those with PM2.5 in both Zanobetti et al. (Citation2010) and Schwartz et al. (Citation2005).
Figure 2. Effect of traffic exposure on heart rate variability (HRV). Risk estimates reported per interquartile range (IQR) increase in PM2.5 or BC.
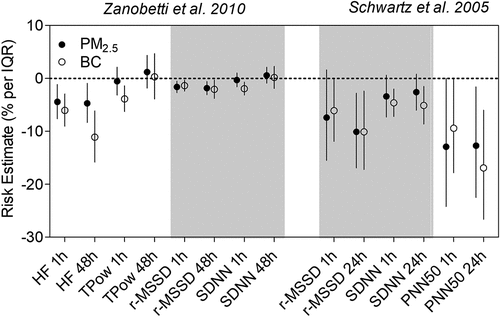
Harvard investigators also conducted a personal exposure study of 44 seniors in suburban St. Louis, MO and consistently found larger associations for BC compared to PM2.5 across all the HRV measures, which included SDNN, r-MSSD, PNN50, HF, and others (Adar et al. Citation2007). Together, these studies suggest that BC exposures may have greater effects on reducing HRV than PM2.5 exposure. Of note, in areas where coal-burning non-traffic sources are the predominant source of air pollution (e.g., Steubenville, OH), PM2.5 and sulfate (a non-traffic coal burning PM component) were equally strongly associated with HRV, reflecting important cardiovascular risks of non-traffic sources of air pollutant exposure (Luttmann-Gibson et al. Citation2014, Citation2006).
In contrast to the aforementioned studies, variable results have been reported in Harvard EPA Center studies on the larger, Normative Aging Study (NAS) cohort (Bell, Rose, and Damon Citation1966, Citation1972). Short-term exposure to BC was not associated with any of the HRV measures in a cross-sectional study (Park et al. Citation2005), but was associated with an increase in LF/HF (a measure of sympathetic tone) in a longitudinal analysis (Baja et al. Citation2013). Another study utilized residential exposures derived from spatiotemporal models and found an association between medium-term (28- and 56-day moving average) BC exposure and a reduced HF, but not with short-term BC exposure (Mordukhovich et al. Citation2015). Therefore, differences in study design (e.g., cross-sectional vs. longitudinal analysis) and exposure characterization (e.g., central monitor vs. spatiotemporal modeling, short- vs. medium-term) may explain variability in results within the same cohort.
Many studies outside of the Harvard EPA Center have illustrated the adverse effects of BC exposure on HRV not only among individuals with cardiovascular disease (Huang et al. Citation2012), diabetes (Sun et al. Citation2015), high body mass index (BMI ≥25 kg/m2) (Dong et al. Citation2018), and chronic obstructive pulmonary disease (COPD) (Pan et al. Citation2018b), but also among healthy individuals (Cole-Hunter et al. Citation2015; Pan et al. Citation2018a). There is also evidence of the adverse effects of increased PN levels on HRV among diabetic patients (Sun et al. Citation2015) as well as healthy individuals (Weichenthal et al. Citation2011). Therefore, traffic-related pollutant exposure is associated with cardiac autonomic dysfunction among not only individuals with existing co-morbidities but also healthy adults.
In summary, exposures to traffic-related particles adversely affect cardiac autonomic function. Short-, medium-, and long-term exposures to PM2.5 and BC are associated with decreased HRV, particularly those that represent parasympathetic tone (e.g., HF, rMSSD, and SDNN). As reduced HRV is a known predictor of poor cardiac outcomes, it is important to identify traffic exposure risks among susceptible populations.
Inflammatory response
Inhalation of particulate pollutants elicits a systemic inflammatory response that is initiated by cytokines released by lung cells engulfing particles that deposit on the lung surface. These cytokines stimulate the bone marrow to produce white blood cells and the liver to produce acute-phase proteins (e.g., CRP, fibrinogen) and coagulation factors. Inflammatory responses promote thrombosis and contribute to and accelerate the progression of atherosclerosis (Libby, Ridker, and Hansson Citation2009; Suwa and Hogg Citation2002), subsequently increasing the risk of complications such as myocardial infarction and stroke. As such, many studies have examined the effect of traffic exposure on inflammatory markers known to be risk factors for cardiovascular disease.
Fibrinogen
As a thrombogenic factor, fibrinogen is an important determinant of blood viscosity and an independent risk factor for cardiovascular disease (Lip Citation1995). Harvard investigators have found associations between BC and PN exposure and increased fibrinogen levels in the NAS cohort (, ) (Bind et al. Citation2012, Citation2014; Zeka et al. Citation2006). In contrast, associations between PM2.5 exposures and fibrinogen were mostly non-significant. Bind et al. (Citation2014) found greater increases in fibrinogen among individuals with genetic susceptibility to oxidative stress, endothelial dysfunction, and metal processing dysfunction. These increases were associated with exposures to PN and BC but not PM2.5. Conversely, outside of the Harvard EPA Center, a Multi-Ethnic Study of Atherosclerosis cohort study of over 6,800 individuals from 6 U.S. cities (Baltimore, MD; Chicago, IL; Winston-Salem, NC; Los Angeles, CA; New York, NY; and St. Paul, MN) have reported increases in fibrinogen with PM2.5 exposures but not BC (Hajat et al. Citation2015).
Table 2. Summary of fibrinogen risk estimates associated with PM2.5, BC, and PN exposure.
Figure 3. Effect of traffic exposure on inflammatory markers–-C reactive protein (CRP) and fibrinogen. Risk estimates reported per 1 μg/m3 increase in BC, 10 μg/m3 increase in PM2.5, and 15,000 particles/cm3 increase in PNC.
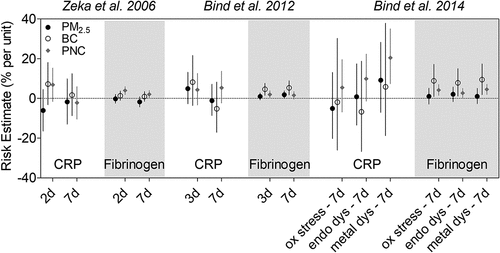
Of note, majority of studies outside of the Harvard EPA Center have not found consistent associations between particles and fibrinogen, with some reporting negative (Panasevich et al. Citation2009; Steinvil et al. Citation2008), positive (Ghio et al. Citation2003; Liao et al. Citation2005), or null associations (Baccarelli et al. Citation2007; Rückerl et al. Citation2007, Citation2014). In the Greater Boston Area, the Framingham Offspring and Third Generation cohort study of nearly 4,000 participants found a negative association between BC and fibrinogen levels (Li et al. Citation2017). Authors concluded this could possibly be due to residual or unmeasured confounding. Therefore, while NAS cohort studies support the relationship between traffic exposure and increased fibrinogen, other studies show mixed results and evaluation of additional inflammatory markers are needed.
C-reactive protein (CPR)
C-reactive protein (CRP) is an acute-phase protein that rises in the presence of inflammation. The aforementioned studies also measured CRP levels and reported weaker associations between traffic exposure and CRP compared to fibrinogen. This is most likely due to larger variability in CRP measurements reported by many studies. Most notably, in a repeated measures analysis by Harvard investigators, elderly men in the NAS cohort with high genetic susceptibility for metal processing dysfunction had increases in CRP level with greater PN exposure, but not with BC or PM2.5 exposure (Bind et al. Citation2014). Consistent with these findings, a subsequent quantile analysis of the same cohort showed that among participants with CRP levels > 2 mg/L (i.e., 60th percentile), an IQR increase in 28-day average PN was associated with an increase in CRP, while no associations were found with BC or PM2.5 (Bind et al. Citation2016). Therefore, PN exposure may confer greater cardiovascular risk than PM2.5 exposure.
Unlike PN, associations between BC exposure and CRP have not been found in NAS cohort studies. However, a Harvard EPA Center study on 88 elderly men with COPD in Eastern Massachusetts found a 12% higher CRP level (95% CI: 2, 23%) per IQR increase in 7-day average indoor BC (Garshick et al. Citation2018). Furthermore, a large study on nearly 4,000 participants in the Framingham Offspring and Third Generation cohorts reported a 5.8% higher CRP level (95% CI: 0.5, 11.4) in association with a 0.5 µg/m3 higher 5-day moving average BC, although associations with other moving averages (1- to 7-days) were null (Li et al. Citation2017).
Outside of the Harvard EPA Center, a study conducted in a Los Angeles cohort of 29 elderly subjects with a history of coronary artery disease found significant associations between CRP and same-day BC and PN exposure, but not for PM2.5 (Delfino et al. Citation2008). In addition, this study compared associations with components of indoor PM and found more robust associations with PM of outdoor origin (e.g., PN, elemental carbon), supporting that traffic-related particles and freshly generated particles trigger inflammatory responses. A follow-up study with a larger cohort (60 elderly subjects with history of coronary artery disease living in Los Angeles) found that CRP was elevated with same-day PN exposure but not BC or PM2.5 exposure (Delfino et al. Citation2009). This finding may reflect differences in the particle size distributions of PN and BC. PN is composed of nanoparticles and ultrafine particles, which differ in aerodynamic properties. For instance, particles 6–12 nm are more sensitive to air stagnation than larger particles 50–100 nm, as evidenced by higher concentrations of nanoparticles near highways in cooler months in Los Angeles (Zhu et al. Citation2004). This adds to the evidence that PN exposure may be more strongly associated with adverse cardiovascular outcomes than PM2.5 exposures. This is especially notable given the relatively higher exposure error of PN due to its considerable spatiotemporal variability.
ICAM-1 and VCAM-1
ICAM-1 and VCAM-1 are proteins found on cell surfaces that mediate the recruitment of white blood cells during an inflammatory response. The soluble form of ICAM-1 and VCAM-1 in the plasma are measured in studies. While ICAM-1 and VCAM-1 are both involved in recruiting white blood cells to sites of inflammation, increased plasma ICAM-1 levels may indicate a general inflammatory state and upregulation in non-endothelial cells, whereas VCAM-1 may have a more restricted distribution within the vascular system (Pradhan, Rifai, and Ridker Citation2002).
Among Harvard EPA Center studies, ICAM-1 and VCAM-1 were found to be associated with BC and PN exposure in the NAS cohort () (Bind et al. Citation2012; Madrigano et al. Citation2010). In addition to NAS cohort studies, Garshick et al. (Citation2018) assessed a smaller cohort of 88 COPD patients and found no association between BC and VCAM-1, whereas O’Neil et al. (Citation2007) found a positive association in a cohort of 92 diabetic patients. Like fibrinogen, there was evidence that VCAM-1 was more strongly associated with BC and PN than PM2.5 (). In Madrigano et al. (Citation2010), increased VCAM-1 levels were associated with BC but not PM2.5. In O’Neil et al. (Citation2007), both BC and PM2.5 exposures were associated with increases in VCAM-1, but BC exposure was linked to greater increases in VCAM-1 levels than PM2.5 exposure. In addition, PN exposure was more strongly associated with increases in VCAM-1 than PM2.5 and BC exposures in Bind et al. (Citation2012). Given that exposure errors for PN are generally larger than for PM2.5 due to greater spatial variability, a greater PN risk estimate despite a less precise exposure measure suggests that the true association between VCAM-1 and PN is likely even greater in magnitude.
Table 3. Summary of VCAM-1 risk estimates associated with PM2.5, BC, and PN exposure.
Figure 4. Effect of traffic exposure on inflammatory markers ICAM and VCAM. Risk estimates reported per 1 μg/m3 increase in BC, 10 μg/m3 increase in PM2.5, and 15,000 particles/cm3 increase in PNC.
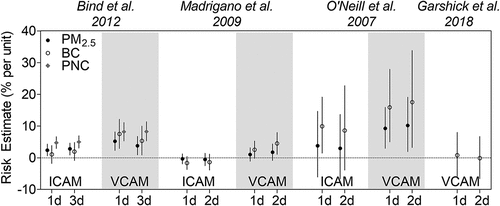
Both Bind et al. (Citation2012) and Alexeeff et al. (Citation2011) investigated the NAS cohort during similar time periods (1999–2008 vs. 2000–2009), but Alexeeff et al. utilized a spatiotemporal land use regression model that was previously developed and validated for estimating daily BC concentrations for each participant’s address (Gryparis et al. Citation2007). Briefly, this model integrated daily monitored values from 82 different monitoring sites in the Greater Boston Area and predicted BC levels using land use measures (e.g., cumulative traffic density, population density, distance to major roadways), geographic information system location (e.g., latitude, longitude), meteorological factors (e.g., apparent temperature, wind speed, planetary boundary layer height), and other characteristics (e.g., day of week, day of season). For a 1 µg/m3 increase in a 28-day average BC, modeled BC concentrations yielded a 5.1% increase in ICAM-1 (95% CI: 0.74, 9.6%) whereas measured BC values yielded a higher risk estimate of 8.8% increase in ICAM-1 (95% CI: 5.3, 12.2%) (, ). Similarly, increases in VCAM-1 levels were lower with the modeled compared to measured BC concentrations.
Table 4. Risk estimates derived from measured versus modeled BC concentrations (risk estimates reported per 1 µg/m3 increase in BC).
Figure 5. Effect of BC exposure on ICAM and VCAM using land use regression models. Risk estimates reported per 1 μg/m3 increase in BC.
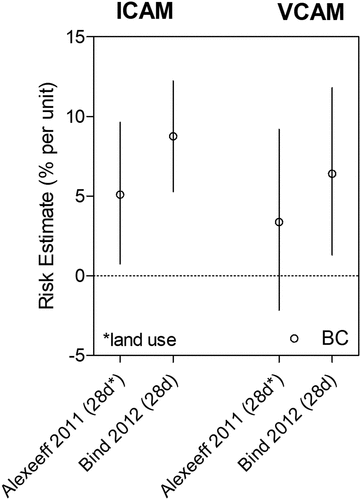
Using a validated land use regression model allows investigators to address individual-level differences in exposures rather than classifying exposures based on measurements at the nearest monitor. A validation study illustrated that 1) the predicted BC estimates are highly correlated with actual exposure measurements from 30 additional monitoring locations in Boston separate from the 82 monitoring stations used to fit the land use model, and that 2) actual exposure measurements are much more closely correlated with the predicted BC measurements than exposure estimates based on a central-site monitor (Gryparis et al. Citation2007). Therefore, risk estimates derived from our land use regression model may reflect a more precise health risk estimate, particularly for pollutants like BC that are more spatially variable.
Outside of the Harvard EPA Center, very few studies have assessed the association of BC and PN exposure with ICAM-1 and VCAM-1. The aforementioned Multi-Ethnic Study of Atherosclerosis cohort study found no association between ICAM-1 with PM2.5 or BC in 2,865 participants in 6 U.S. cities (Hajat et al. Citation2015). While this study included a large number of samples, an important limitation was that an annual average BC concentration was used due to limited availability of daily central monitoring data on BC. A recent personal exposure study conducted among 53 traffic police officers in Kathmandu, Nepal showed no association between PM2.5 or BC with ICAM-1, VCAM-1, or CRP (Shakya et al. Citation2019). This study was limited by sample size and short study duration (i.e., 5–6 days per participant). Therefore, the Harvard EPA Center studies uniquely provide valuable insights into the association of BC and PN exposure with increases in ICAM-1 and VCAM-1 levels.
Other inflammatory markers
In addition to fibrinogen, CRP, ICAM-1, and VCAM-1, studies have assessed a host of other markers of inflammation and thrombosis (e.g., interleukin (IL)-1β, IL-6, IL-8, tumor necrosis factor (TNF)-α, sedimentation rate, white blood cell (WBC) count, sTNF-RII, von Willebrand Factor, endothelin-1b and, vascular endothelial growth factor (VEGF) (Fang et al. Citation2012; Garshick et al. Citation2018; Liu et al. Citation2009; Wittkopp et al. Citation2013). Other studies have explored the effect of traffic pollutant exposures on novel biomarkers of oxidative stress, illustrating evidence of decreased activity of in erythrocyte antioxidant enzymes (e.g., glutathione peroxidase-1 and superoxide dismutase) (Delfino et al. Citation2009) and increases in lipid peroxidation and oxidative DNA damage (Grady et al. Citation2018). In aggregate, these studies suggest that systemic responses–-in particular systemic inflammation and pro-thrombotic activity–-mediate the relationship between traffic pollutant exposure and cardiovascular disease. Several Harvard EPA Center studies have also found an association between BC and ST-segment depression (Chuang et al. Citation2008; Gold et al. Citation2005), which suggests that particle exposure may induce low-grade myocardial inflammation and repolarization changes. Together, these studies provide evidence that traffic pollutants increase cardiovascular disease risk through an inter-related process of inflammation and endothelial dysfunction.
Blood pressure
Air pollution exposure induces autonomic imbalance, oxidative stress, and systemic inflammation. These, in turn, promote endothelial dysfunction (Madrigano et al. Citation2010), impaired vascular reactivity (Wilker et al. Citation2014), and hypertension (Brook et al. Citation2009). Studies have reported associations between elevated BP and short-term PM2.5 and BC exposure among elderly subjects (Delfino et al. Citation2010; Liu et al. Citation2009; Zanobetti et al. Citation2004a), healthy individuals (Kubesch et al. Citation2015; Louwies et al. Citation2015), and newborns (van Rossem et al. Citation2015). Residential proximity to major roadways have also been shown to be associated with hypertension among postmenopausal women (Kirwa et al. Citation2014). However, other studies have found null or inconsistent links between traffic pollutants and blood pressure (Ebelt, Wilson, and Brauer Citation2005; Fuks et al. Citation2014; Ibald-Mulli et al. Citation2004). Human chamber inhalation studies have demonstrated PM2.5-induced increases in BP, particularly in high-traffic areas (Brook et al. Citation2009). Personal exposure studies on indoor and outdoor cycling have also reported an association between short-term (2 hour) ultrafine particle exposure and increases in systolic BP and adverse effects on micro-vascular function among healthy individuals (Kubesch et al. Citation2015; Weichenthal, Hatzopoulou, and Goldberg Citation2014). In addition, a year-long particle filter intervention has been shown to lower systolic BP in a Taiwanese cohort (Chuang et al. Citation2017). Furthermore, PM2.5, BC, and PN have been found to be associated with a higher baseline pulse amplitude, suggesting an adverse effect on vascular tone (Ljungman et al. Citation2014).
A number of Harvard EPA Center studies have investigated the association between traffic pollutants and BP in the NAS cohort (, ). These NAS studies consistently reported increases in both systolic and diastolic BP associated with BC exposure (Bind et al. Citation2016; Mordukhovich et al. Citation2009; Schwartz et al. Citation2012; Wilker et al. Citation2010; Zhong et al. Citation2016). In Mordukhovich et al. (Citation2009), an elevation in BP was observed with BC exposure but not PM2.5 in the NAS cohort (n = 461, 1999–2007). Wilker et al. (Citation2010) studied a larger NAS cohort (n = 789, 1995–2008) and reported even greater BP increases associated with BC exposure. Furthermore, Bind et al. (Citation2016) found larger elevations in systolic BP with BC exposure compared to PM2.5 exposure, particularly among participants with higher baseline systolic BP. For instance, among participants with systolic BP > 155 mmHg (i.e., 90th percentile), an IQR increase in BC exposure was associated with a higher systolic BP by 7.2 mmHg (95% CI: 5.5, 8.8) compared to 3.6 mmHg (95% CI: 1.6, 5.7) for PM2.5 exposure. This finding adds to the evidence that BC exposure may have greater consequences to cardiovascular health than PM2.5 exposure.
Table 5. Summary of blood pressure risk estimates associated with PM2.5, BC, and PN exposure.
Figure 6. Effect of traffic exposure on systolic and diastolic blood pressure. Risk estimates reported per 1 μg/m3 increase in BC and 10 μg/m3 increase in PM2.5.
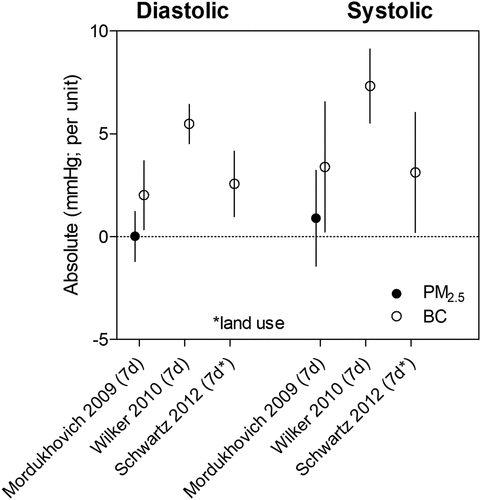
Schwartz et al. (Citation2012) also studied the NAS cohort (n = 853, 1996–2008), but employed a spatiotemporal land use regression model to estimate daily BC concentrations. Modeled BC concentrations in Schwartz et al. (Citation2012) yielded a lower risk estimate than Wilker et al. (Citation2010), which utilized monitored BC concentrations. A 1 μg/m3 increase in 7-day average BC was associated with an increase in diastolic BP by 5.5 mmHg (95% CI: 4.5, 6.4 mmHg) in Wilker et al. (Citation2010) compared to 2.6 mmHg (95% CI: 0.98, 4.2 mmHg) in Schwartz et al. (Citation2012). Similar patterns between modeled and monitored BC concentrations were observed with risk estimates of systolic BP increases. The utilization of land use regression models is an attempt to reduce exposure error as exposure misclassification often leads to an underestimation of the health effects of air pollution (Zeger et al. Citation2000). A slightly lower risk estimate with modeled compared to measured BC concentrations is consistent with the pattern of findings with the risk estimates for ICAM-1 and VCAM-1 levels (). In addition, Wellenius et al. (Citation2012) explicitly compared and reported similar but slightly lower risk estimates for ischemic stroke derived from modeled compared to observed BC concentrations. Further studies comparing risk estimates using both BC measurements from central monitoring sites and modeled BC concentrations are needed.
Other Boston-based Harvard Center studies have found increases in BP with both BC and PM2.5 exposures among cardiac rehabilitation patients (Zanobetti et al. Citation2004b) and individuals with type 2 diabetes (Hoffmann et al. Citation2012). Outside of the Harvard EPA Center, another Boston-based traffic exposure study assessed the effect of same-day PM2.5, BC, and PN on BP among a cohort of 220 individuals who primarily reside near highways (Chung et al. Citation2015). This study found increases in diastolic BP associated with PN exposure but not with PM2.5 or BC. In conjunction with the previously discussed robust effect of PN exposure on fibrinogen and CRP levels, this result provides additional evidence for the harmful effect of PN on cardiovascular health. The null associations with BC and PM2.5 in Chung et al. (Citation2015) may be due to differences in exposure window (e.g., same-day vs. 7-day) and study cohort, as susceptible populations (e.g., patients with history of cardiovascular disease, diabetic patients, elderly) may be at greater risk of BP increases with BC and PM2.5 exposures. A recent study on the same Boston-based cohort found that the association between PN and BP were stronger among non-Hispanic white participants and among diabetics (Corlin et al. Citation2018).
In summary, associations between BP elevation and PM2.5 and BC exposures were observed across different Boston-based cohorts in different time periods, using both monitored air pollution measurements and spatiotemporal model predicted values. In addition, there is evidence that BC and PN exposures pose greater risk of BP increases than PM2.5 exposure.
Ischemic stroke
There is extensive literature on the adverse effect of both short-term (days to weeks) and long-term (months to years) exposure to air pollution and stroke (Ljungman and Mittleman Citation2014). A Harvard EPA Center study using a hybrid satellite remote sensing and land use regression approach to estimate particle exposure in New England found that both acute and chronic PM2.5 exposures were associated with stroke (Kloog et al. Citation2012). Another study geocoded the addresses of all deaths in Massachusetts between 1995 and 2002, and found that an IQR increase in BC was associated with 2.3% (95% CI, 1.2, 3.4%) increase in daily deaths, with an even larger effect on stroke mortality risk (4.4%; 95% CI, −0.2, 9.3%) (Maynard et al. Citation2007).
Among Harvard EPA Center studies, Wellenius et al. (Citation2012) and Mostofsky et al. (Citation2012) are directly comparable, large cohort studies (1,705 and 1,060 patients, respectively) that found an elevated risk of ischemic stroke with exposure to PM2.5 as well as BC (). Both studies utilized a time-stratified case crossover design and included patients who were hospitalized at the Beth Israel Deaconess Medical Center (BIDMC) with neurologist-confirmed ischemic stroke. Mostofsky et al. 2012 additionally demonstrated that the effect of BC exposure was independent of the PM2.5 effect. In that study, a second model adjusted for PM2.5 in estimating the BC exposure risk, and a third model assessed the effect of residual BC mass that is not correlated with PM2.5 concentrations. These risk estimates were similar to those estimated by the single-pollutant model, illustrating the BC effect was independent of PM2.5 exposure. In addition, BC risk estimates were slightly larger than PM2.5 risk estimates.
Figure 7. Estimated effects of traffic exposure on ischemic stroke risk. Risk estimates reported per IQR increase in BC and PM2.5.
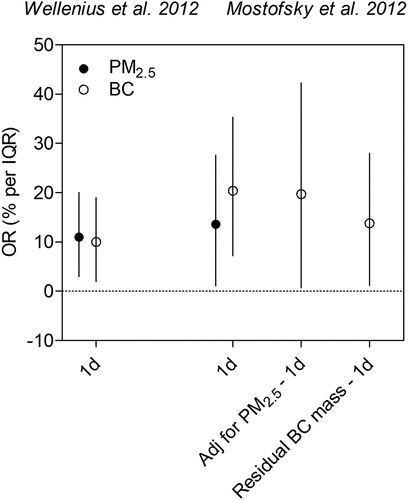
Outside of the Harvard EPA Center, epidemiological studies on the association between stroke and exposure to BC or PN are sparse. A recent large time-stratified case-crossover study (n = 2,742) based in Barcelona, Spain reported an increased risk of ischemic stroke risk among patients with large-artery atherosclerosis to be associated with BC exposure, but not with PM2.5 (Vivanco-Hidalgo et al. Citation2018). In summary, exposure to traffic-related pollutants is associated with an increased risk of ischemic stroke. There is evidence that the effect of BC is independent of the effect of PM2.5 exposure, and that the risk is greater with BC exposure than with PM2.5 exposure.
Conclusion
The adverse effect of air pollution on cardiovascular health is well-established and supported by traffic studies from the Harvard EPA Center. Specific markers of traffic exposure (e.g., roadway proximity, BC, PN) are associated with a number of adverse cardiovascular outcomes, including cardiac autonomic dysfunction, systemic inflammation and thrombosis, elevated BP, and ischemic stroke. Studies both within and outside of the Harvard EPA Center suggest that BC and PN exposures are more strongly associated with cardiovascular outcomes than PM2.5 exposures. There is epidemiological evidence that such associations may be physiologically mediated by inflammatory responses as well as microvascular and autonomic dysfunction; however, further toxicology and targeted animal and controlled human studies elucidating the underlying pathophysiological mechanism are needed. In addition, monitored BC and PN measurements as estimates of personal traffic exposure and the magnitude of exposure errors need further characterization. Strong associations between PN and inflammatory markers (e.g., VCAM-1, CRP) have been observed despite the wide variability in PN measurements–-and subsequently larger exposure errors–-suggesting that the true association may be even greater in magnitude. In addition, the use of land use models may yield a more precise health risk estimate especially for more spatially variable pollutants. More studies directly comparing risk estimates derived from monitored and modeled measurements of particulate pollutants are needed in order to characterize the influence of exposure errors on study results. Further research is necessary to support generalizability of our findings to populations with greater diversity in race, socioeconomic status, geographic location, age, and health status. With the wealth of evidence that traffic emissions as measured by BC and PN are particularly harmful to human health, controlling their local and regional sources is important for protecting public health.
Abbreviations
BC | = | black carbon |
BP | = | blood pressure |
BIDMC | = | Beth Israel Deaconess Medical Center |
CO | = | carbon monoxide |
CRP | = | C-reactive protein |
EPA | = | Environmental Protection Agency |
ICAM-1 | = | intercellular adhesion molecule-1 |
MOBILIZE | = | Maintenance of Balance, Independent Living, Intellect, and Zest in the Elderly |
NO2 | = | nitrogen dioxide |
NAS | = | Normative Aging Study |
PM2.5 | = | fine particulate matter |
PN | = | particle number |
UFP | = | ultrafine particle |
VCAM-1 | = | vascular cell adhesion molecule-1 |
Acknowledgment
This publication was made possible by the National Institute of Environmental Health Sciences grants P01-ES009825, P30-ES000002, R01-ES015172-01, R01-ES024332, R01-ES019853, R01-ES022657-01A1, R00-ES015774, and K23-ES026204 and US EPA grants RD-83479801, RD-83587201, and RD-83241601. Its contents are solely the responsibility of the grantee and do not necessarily represent the official views of the U.S. EPA. Further, U.S. EPA does not endorse the purchase of any commercial products or services mentioned in the publication. The Veterans Administration’s Normative Aging Study is supported by the Cooperative Studies Program/Epidemiology Research and Information Centers of the U.S. Department of Veterans Affairs and is a component of the Massachusetts Veterans Epidemiology Research and Information Center (MAVERIC), Boston, MA. Research reported in this publication was also supported by the John Harvard Distinguished Science Fellows Program within the FAS Division of Science of Harvard University and by the Office of the Director, National Institutes of Health under Award Number DP5OD021412. The content is solely the responsibility of the authors and does not necessarily represent the official views of the National Institutes of Health, the U.S. Department of Veterans Affairs, or the United States Government.
Additional information
Funding
Notes on contributors
Iny Jhun
Iny Jhun and Jina J. Kim are graduates of the Harvard T. H. Chan School of Public Health in Boston, MA.
Iny Jhun and Bennet Cho are students at Harvard Medical School in Boston, MA.
Jina Kim
Iny Jhun and Jina J. Kim are graduates of the Harvard T. H. Chan School of Public Health in Boston, MA.
Bennet Cho
Iny Jhun and Bennet Cho are students at Harvard Medical School in Boston, MA.
Diane R. Gold
Diane R. Gold, Joel Schwartz, Brent A. Coull, Murray Mittleman, Francesca Dominici, and Petros Koutrakis are research professors at the Harvard T. H. Chan School of Public Health in Boston, MA.
Diane R. Gold is also a professor of medicine at Harvard Medical School and affiliated with the Brigham and Women’s Hospital in Boston, MA.
Antonella Zanobetti
Antonella Zanobetti is a principal research scientist at the Harvard T. H. Chan School of Public Health in Boston, MA.
Mary B. Rice
Mary B. Rice and Elissa H. Wilker are assistant professors of medicine at the Harvard Medical School and affiliated with the Beth Israel Deaconess Medical Center in Boston, MA.
Murray A. Mittleman
Murray Mittleman is also an associate professor of medicine at Harvard Medical School and affiliated with the Beth Israel Deaconess Medical Center in Boston, MA.
Eric Garshick
Eric Garshick is a professor medicine at the Harvard Medical School and affiliated with the Veterans Affairs Boston Healthcare System in Boston, MA
Pantel Vokonas
Pantel S. Vokonas is a professor of preventive medicine and epidemiology at the Boston University School of Medicine and affiliated with the Veterans Affairs Boston Healthcare System in Boston, MA.
Marie-Abele Bind
Marie-Abele Bind is a research associate at the Department of Statistics and John Harvard Distinguished Science Fellow, Division of Science at Harvard University in Cambridge, MA.
Elissa H. Wilker
Elissa H. Wilker is also an adjunct assistant professor at the Harvard T. H. Chan School of Public Health in Boston, MA and affiliated with Sanofi Genzyme in Cambridge, MA.
Helen Suh
Helen Suh is a professor in the Department of Civil and Environmental Engineering at Tufts University in Medford, MA.
References
- Adam, M., M. Imboden, E. Boes, E. Schaffner, N. Künzli, H. C. Phuleria, F. Kronenberg, J.-M. Gaspoz, D. Carballo, N. Probst-Hensch, et al. 2014. Modifying effect of a common polymorphism in the interleukin-6 promoter on the relationship between long-term exposure to traffic-related particulate matter and heart rate variability. PLoS ONE 9:e104978. doi:10.1371/journal.pone.0104978.
- Adar, S. D., D. R. Gold, B. A. Coull, J. Schwartz, P. H. Stone, and H. Suh. 2007. Focused exposures to airborne traffic particles and heart rate variability in the elderly. Epidemiology 18:95–103. doi:10.1097/01.ede.0000249409.81050.46.
- Alexeeff, S. E., B. A. Coull, A. Gryparis, H. Suh, D. Sparrow, P. S. Vokonas, and J. Schwartz. 2011. Medium-term exposure to traffic-related air pollution and markers of inflammation and endothelial function. Environ. Health Perspect. 119:481–6. doi:10.1289/ehp.1002560
- Baccarelli, A., A. Zanobetti, I. Martinelli, P. Grillo, L. Hou, S. Giacomini, M. Bonzini, G. Lanzani, P. M. Mannucci, P. A. Bertazzi, et al. 2007. Effects of exposure to air pollution on blood coagulation. J. Thromb. Haemost. 5:252–60. doi:10.1111/j.1538-7836.2007.02300.x.
- Baja, E. S., J. D. Schwartz, B. A. Coull, G. A. Wellenius, P. S. Vokonas, and H. H. Suh. 2013. Structural equation modeling of parasympathetic and sympathetic response to traffic air pollution in a repeated measures study. Environ. Heal. 12:81. doi:10.1186/1476-069X-12-81.
- Bell, B., C. L. Rose, and A. Damon. 1966. The veterans administration longitudinal study of healthy aging. Gerontologist 6:179–84. doi:10.1093/geront/6.4.179.
- Bell, B., C. L. Rose, and A. Damon. 1972. The normative aging study: An Interdisciplinary and longitudinal study of health and aging. Aging Hum. Dev. 3:5–17. doi:10.2190/GGVP-XLB5-PC3N-EF0G.
- Bettoni, M., and M. Zimmermann. 2002. Autonomic tone variations before the onset of paroxysmal atrial fibrillation. Circulation 105:2753–59. doi:10.1161/01.CIR.0000018443.44005.D8.
- Bilenko, N., L. van Rossem, B. Brunekreef, R. Beelen, M. Eeftens, G. Hoek, B. Neal, G. S. Hillis, N. Rafter, A. Tonkin, et al. 2015. Traffic-related air pollution and noise and children’s blood pressure: Results from the PIAMA birth cohort study. Eur. J. Prev. Cardiol. 22:4–12. doi:10.1177/2047487313505821.
- Bind, M.-A., A. Baccarelli, A. Zanobetti, L. Tarantini, H. Suh, P. Vokonas, and J. Schwartz. 2012. Air pollution and markers of coagulation, inflammation, and endothelial function: Associations and epigene-environment interactions in an elderly cohort. Epidemiology 23:332–40. doi:10.1097/EDE.0b013e31824523f0.
- Bind, M.-A., A. Peters, P. Koutrakis, B. Coull, P. Vokonas, and J. Schwartz. 2016. Quantile regression analysis of the distributional effects of air pollution on blood pressure, heart rate variability, blood lipids, and biomarkers of inflammation in Elderly American Men: The normative aging study. Environ. Health Perspect. 124:1189–98. doi:10.1289/ehp.1510044.
- Bind, M.-A., B. Coull, H. Suh, R. Wright, A. Baccarelli, P. Vokonas, J. Schwartz, and H. Ten Cate. 2014. A novel genetic score approach using instruments to investigate interactions between pathways and environment: Application to air pollution. PLoS ONE 9:e96000. doi:10.1371/journal.pone.0096000.
- Brauer, M., G. Hoek, P. van Vliet, K. Meliefste, P. Fischer, U. Gehring, J. Heinrich, J. Cyrys, T. Bellander, M. Lewne, et al. 2003. Estimating long-term average particulate air pollution concentrations: Application of traffic indicators and geographic information systems. Epidemiology 14:228–39. doi:10.1097/01.EDE.0000041910.49046.9B.
- Brook, R. D. 2008. Cardiovascular effects of air pollution. Clin. Sci. 115:175–87. doi:10.1042/CS20070444.
- Brook, R. D., B. Urch, J. T. Dvonch, R. L. Bard, M. Speck, G. Keeler, M. Morishita, F. J. Marsik, A. S. Kamal, N. Kaciroti, et al. 2009. Insights into the mechanisms and mediators of the effects of air pollution exposure on blood pressure and vascular function in healthy humans. Hypertension 54:659–67. doi:10.1161/HYPERTENSIONAHA.109.130237.
- Brook, R. D., S. Rajagopalan, C. A. Pope, J. R. Brook, A. Bhatnagar, A. V. Diez-Roux, F. Holguin, Y. Hong, R. V. Luepker, M. A. Mittleman, et al. 2010. Particulate matter air pollution and cardiovascular disease: An update to the scientific statement from the American Heart Association. Circulation 121:2331–78. doi:10.1161/CIR.0b013e3181dbece1.
- Chahine, T., A. Baccarelli, A. Litonjua, R. O. Wright, H. Suh, D. R. Gold, D. Sparrow, P. Vokonas, and J. Schwartz. 2007. Particulate air pollution, oxidative stress genes, and heart rate variability in an elderly cohort. Environ. Health Perspect. 115:1617–22. doi:10.1289/ehp.10318.
- Chuang, H.-C., K.-F. Ho, L.-Y. Lin, T.-Y. Chang, G.-B. Hong, C.-M. Ma, I.-J. Liu, and K.-J. Chuang. 2017. Long-term indoor air conditioner filtration and cardiovascular health: A randomized crossover intervention study. Environ. Int. 106:91–96. doi:10.1016/j.envint.2017.06.008.
- Chuang, K. J., B. A. Coull, A. Zanobetti, H. Suh, J. Schwartz, P. H. Stone, A. Litonjua, F. E. Speizer, and D. R. Gold. 2008. Particulate air pollution as a risk factor for ST-segment depression in patients with coronary artery disease. Circulation 118:1314–20. doi:10.1161/CIRCULATIONAHA.108.765669.
- Chung M., D. D., Wang, A. M., Rizzo AM, D. Gachette, M., Delnord, R., Parambi, C. M. Kang, and D. Brugge. 2015. Association of PNC, BC, and PM2.5 measured at a central monitoring site with blood pressure in a predominantly near highway population. Int. J. Environ. Res. Public Health 12:2765–80. doi:10.3390/ijerph120302765.
- Cole-Hunter, T., S. Weichenthal, N. Kubesch, M. Foraster, G. Carrasco-Turigas, L. Bouso, D. Martínez, D. Westerdahl, A. de Nazelle, and M. Nieuwenhuijsen. 2015. Impact of traffic-related air pollution on acute changes in cardiac autonomic modulation during rest and physical activity: A cross-over study. J. Expo. Sci. Environ. Epidemiol. 26:133–40. doi:10.1038/jes.2015.66.
- Corlin L, Ball S, Woodin M, Patton A, Lane K, Durant J, and D. Brugge. 2018. Relationship of time-activity-adjusted particle number concentration with blood pressure. Int. J. Environ. Res. Public Health 15:2036. doi:10.3390/ijerph15092036.
- Delfino, R. J., N. Staimer, T. Tjoa, A. Polidori, M. Arhami, D. L. Gillen, M. T. Kleinman, N. D. Vaziri, J. Longhurst, F. Zaldivar, et al. 2008. Circulating biomarkers of inflammation, antioxidant activity, and platelet activation are associated with primary combustion aerosols in subjects with coronary artery disease. Environ. Health Perspect. 116:898–906. doi:10.1289/ehp.11189.
- Delfino, R. J., N. Staimer, T. Tjoa, D. L. Gillen, A. Polidori, M. Arhami, M. T. Kleinman, N. D. Vaziri, J. Longhurst, and C. Sioutas. 2009. Air pollution exposures and circulating biomarkers of effect in a susceptible population: Clues to potential causal component mixtures and mechanisms. Environ. Health Perspect. 117:1232–38. doi:10.1289/ehp.0800194.
- Delfino RJ, Tjoa T, Gillen DL, Staimer N, Polidori A, Arhami M, L. Jamner, C. Sioutas, and J. Longhurst. 2010. Traffic-related air pollution and blood pressure in elderly subjects with coronary artery disease. Epidemiology 21:396–404. doi:10.1097/EDE.0b013e3181d5e19b.
- Di, Q., Y. Wang, A. Zanobetti, Y. Wang, P. Koutrakis, C. Choirat, F. Dominici, and J. D. Schwartz. 2017. Air pollution and mortality in the medicare population. N. Engl. J. Med. 376:2513–22. doi:10.1056/NEJMoa1702747.
- Dockery, D. W., C. A. Pope, X. Xu, J. D. Spengler, J. H. Ware, M. E. Fay, B. G. Ferris, and F. E. Speizer. 1993. An association between air pollution and mortality in six U.S. Cities. N. Engl. J. Med. 329:1753–59. doi:10.1056/NEJM199312093292401.
- Dockery, D. W., H. Luttmann-Gibson, D. Q. Rich, M. S. Link, M. A. Mittleman, D. R. Gold, P. Koutrakis, J. D. Schwartz, and R. L. Verrier. 2005. Association of air pollution with increased incidence of ventricular tachyarrhythmias recorded by implanted cardioverter defibrillators. Environ. Health Perspect. 113:670–74. doi:10.1289/ehp.7767.
- Dong, W., L. Pan, H. Li, M. R. Miller, M. Loh, S. Wu, J. Xu, X. Yang, J. Shan, Y. Chen, et al. 2018. Association of size-fractionated indoor particulate matter and black carbon with heart rate variability in healthy elderly women in Beijing. Indoor Air 28:373–82. doi:10.1111/ina.12449.
- Ebelt, S. T., W. E. Wilson, and M. Brauer. 2005. Exposure to ambient and nonambient components of particulate matter: A comparison of health effects. Epidemiology 16:396–405. doi:10.1097/01.ede.0000158918.57071.3e.
- Fang SC, Mehta AJ, Alexeeff SE, Gryparis A, Coull B, Vokonas P, D. C. Christiani, and J. Schwartz. 2012. Residential black carbon exposure and circulating markers of systemic inflammation in elderly males: The normative aging study. Environ. Health Perspect. 120:674–80. doi:10.1289/ehp.1103982.
- Finkelstein, M. M., M. Jerrett, and M. R. Sears. 2004. Traffic air pollution and mortality rate advancement periods. Am. J. Epidemiol. 160:173–77. doi:10.1093/aje/kwh181.
- Fleisch, A. F., S. L. Rifas-Shiman, P. Koutrakis, J. D. Schwartz, I. Kloog, S. Melly, B. A. Coull, A. Zanobetti, M. W. Gillman, D. R. Gold, et al. 2015. Prenatal exposure to traffic pollution: Associations with reduced fetal growth and rapid infant weight gain. Epidemiology 26:43–50. doi:10.1097/EDE.0000000000000203.
- Foraster, M., X. Basagaña, I. Aguilera, M. Rivera, D. Agis, L. Bouso, A. Deltell, J. Marrugat, R. Ramos, J. Sunyer, et al. 2014. Association of long-term exposure to traffic-related air pollution with blood pressure and hypertension in an adult population-based cohort in Spain (the REGICOR study). Environ. Health Perspect. 122:404–11. doi:10.1289/ehp.1306497.
- Franklin, M., A. Zeka, and J. Schwartz. 2007. Association between PM2.5 and all-cause and specific-cause mortality in 27 US communities. J. Expo. Sci. Environ. Epidemiol. 17:279–87. doi:10.1038/sj.jes.7500530.
- Fuks, K. B., G. Weinmayr, M. Foraster, J. Dratva, R. Hampel, D. Houthuijs, B. Oftedal, A. Oudin, S. Panasevich, J. Penell, et al. 2014. Arterial blood pressure and long-term exposure to traffic-related air pollution: An analysis in the European Study of Cohorts for Air Pollution Effects (ESCAPE). Environ. Health Perspect. 122:896–905. doi:10.1289/ehp.1307725.
- Garshick, E., S. T. Grady, J. E. Hart, B. A. Coull, J. D. Schwartz, F. Laden, M. L. Moy, and P. Koutrakis. 2018. Indoor black carbon and biomarkers of systemic inflammation and endothelial activation in COPD patients. Environ. Res. 165:358–64. doi:10.1016/j.envres.2018.05.010.
- Gehring U, Heinrich J, Krämer U, Grote V, Hochadel M, Sugiri D, M. Kraft, K. Rauchfuss, H. G. Eberwein, and H-E. Wichmann. 2006. Long-term exposure to ambient air pollution and cardiopulmonary mortality in women. Epidemiology 17:545–51. doi:10.1097/01.ede.0000224541.38258.87.
- Ghio, A. J., A. Hall, M. A. Bassett, W. E. Cascio, and R. B. Devlin. 2003. Exposure to concentrated ambient air particles alters hematologic indices in humans. Inhal. Toxicol. 15:1465–78. doi:10.1080/08958370390249111.
- Gold, D. R., A. A. Litonjua, A. Zanobetti, B. A. Coull, J. Schwartz, G. MacCallum, R. L. Verrier, B. D. Nearing, M. J. Canner, H. Suh, et al. 2005. Air pollution and ST-segment depression in elderly subjects. Environ. Health Perspect. 113:883–87. doi:10.1289/ehp.7737.
- Gold DR, Litonjua A, Schwartz J, Lovett E, Larson A, Nearing B, G. Allen, M. Verrier, R. Cherry, and R. Verrier. 2000. Ambient pollution and heart rate variability. Circulation 101:1267–73.
- Grady ST, Koutrakis P, Hart JE, Coull BA, Schwartz J, Laden F, J. J. Zhang, J. Gong, M. L. Moy, E. Garshick. 2018. Indoor black carbon of outdoor origin and oxidative stress biomarkers in patients with chronic obstructive pulmonary disease. Environ. Int. 115:188–95. doi:10.1016/j.envint.2018.02.040.
- Gryparis, A., B. A. Coull, J. Schwartz, and H. H. Suh. 2007. Semiparametric latent variable regression models for spatiotemporal modelling of mobile source particles in the greater Boston area. J. R. Stat. Soc. Ser. C (Applied Stat. 56:183–209. doi:10.1111/j.1467-9876.2007.00573.x.
- Hajat, A., M. Allison, A. V. Diez-Roux, N. S. Jenny, N. W. Jorgensen, A. A. Szpiro, S. Vedal, and J. D. Kaufman. 2015. Long-term exposure to air pollution and markers of inflammation, coagulation, and endothelial activation. Epidemiology 26:310–20. doi:10.1097/EDE.0000000000000267.
- Hart, J. E., E. B. Rimm, K. M. Rexrode, and F. Laden. 2013. Changes in traffic exposure and the risk of incident myocardial infarction and all-cause mortality. Epidemiology 24:734–42. doi:10.1097/EDE.0b013e31829d5dae.
- Hart, J. E., S. E. Chiuve, F. Laden, and C. M. Albert. 2014. Roadway proximity and risk of sudden cardiac death in women. Circulation 130:1474–82. doi:10.1161/CIRCULATIONAHA.114.011489.
- Hochadel, M., J. Heinrich, U. Gehring, V. Morgenstern, T. Kuhlbusch, E. Link, H.-E. Wichmann, and U. Krämer. 2006. Predicting long-term average concentrations of traffic-related air pollutants using GIS-based information. Atmos. Environ. 40:542–53. doi:10.1016/j.atmosenv.2005.09.067.
- Hoek, G., B. Brunekreef, S. Goldbohm, P. Fischer, and P. A. van Den Brandt. 2002. Association between mortality and indicators of traffic-related air pollution in the Netherlands: A cohort study. Lancet 360:1203–09. doi:10.1016/S0140-6736(02)11280-3.
- Hoek, G., P. Fischer, P. Van Den Brandt, S. Goldbohm, and B. Brunekreef. 2001. Estimation of long-term average exposure to outdoor air pollution for a cohort study on mortality. J. Expo. Anal. Environ. Epidemiol. 11:459–69. doi:10.1038/sj.jea.7500189.
- Hoffmann, B., H. Luttmann-Gibson, A. Cohen, A. Zanobetti, and S. C. De. 2012. Opposing effects of particle pollution, ozone, and ambient temperature on arterial blood pressure. Environ Health Perspect. 120:241–47.
- Hoffmann, B., S. Moebus, S. Möhlenkamp, A. Stang, N. Lehmann, N. Dragano, A. Schmermund, M. Memmesheimer, K. Mann, R. Erbel, et al. 2007. Residential exposure to traffic is associated with coronary atherosclerosis. Circulation 116:489–96. doi:10.1161/CIRCULATIONAHA.107.693622.
- Huang, S., J. Lawrence, C.-M. Kang, J. Li, M. Martins, P. Vokonas, D. R. Gold, J. Schwartz, B. A. Coull, and P. Koutrakis. 2018. Road proximity influences indoor exposures to ambient fine particle mass and components. Environ. Pollut. 243:978–87. doi:10.1016/j.envpol.2018.09.046.
- Huang, W., T. Zhu, X. Pan, M. Hu, S.-E. Lu, Y. Lin, T. Wang, Y. Zhang, and X. Tang. 2012. Air pollution and autonomic and vascular dysfunction in patients with cardiovascular disease: Interactions of systemic inflammation, overweight, and gender. Am. J. Epidemiol. 176:117–26. doi:10.1093/aje/kwr511.
- Ibald-Mulli A, Timonen KL, Peters A, Heinrich J, Wölke G, Lanki T, G. Buzorius, W. G. Kreyling, J. de Hartog, G. Hoek, and H. M. ten Brink, et al. 2004. Effects of particulate air pollution on blood pressure and heart rate in subjects with cardiovascular disease: A multicenter approach. Environ. Health Perspect. 112:369–77. doi:10.1289/ehp.6523.
- Janssen, N. A. H., J. Schwartz, A. Zanobetti, and H. H. Suh. 2002. Air conditioning and source-specific particles as modifiers of the effect of PM(10) on hospital admissions for heart and lung disease. Environ. Health Perspect. 110:43–49. doi:10.1289/ehp.02110s5883.
- Kang, C.-M., P. Koutrakis, and H. H. Suh. 2010. Hourly measurements of fine particulate sulfate and carbon aerosols at the Harvard-U.S. Environmental Protection Agency supersite in Boston. J. Air Waste Manage. Assoc. 60:1327–34. doi:10.3155/1047-3289.60.11.1327.
- Kaplan, G. A., and J. E. Keil. 1993. Socioeconomic factors and cardiovascular disease: A review of the literature. Circulation 88:1973–98.
- Kingsley, S. L., M. N. Eliot, E. A. Whitsel, Y. Wang, B. A. Coull, L. Hou, H. G. Margolis, K. L. Margolis, L. Mu, W.-C.-C. Wu, et al. 2015. Residential proximity to major roadways and incident hypertension in post-menopausal women. Environ. Res. 142:522–28. doi:10.1016/j.envres.2015.08.002.
- Kioumourtzoglou M-A, Schwartz JD, Weisskopf MG, Melly SJ, Wang Y, Dominici F, and A. Zanobetti. 2016. Long-term PM2.5 exposure and neurological hospital admissions in the Northeastern United States. Environ. Health Perspect. 124:23–29. doi:10.1289/ehp.1408973.
- Kirwa K, Eliot MN, Wang Y, Adams MA, Morgan CG, Kerr J, G. J. Norman, C. B. Eaton, M. A. Allison, and G. A. Wellenius. 2014. Residential proximity to major roadways and prevalent hypertension among postmenopausal women: Results from the Women’s Health Initiative San Diego Cohort. J. Am. Heart Assoc. 3:e000727. doi:10.1161/JAHA.113.000727.
- Kloog, I., B. A. Coull, A. Zanobetti, P. Koutrakis, and J. D. Schwartz. 2012. Acute and chronic effects of particles on hospital admissions in New-England. PLoS ONE 7:e34664. doi:10.1371/journal.pone.0034664.
- Kop, W. J., R. J. Verdino, J. S. Gottdiener, S. T. O’Leary, C. N. Bairey Merz, and D. S. Krantz. 2001. Changes in heart rate and heart rate variability before ambulatory ischemic events(1). J. Am. Coll. Cardiol. 38:742–49. doi:10.1016/S0735-1097(01)01451-6.
- Korek, M. J., T. D. Bellander, T. Lind, M. Bottai, K. M. Eneroth, B. Caracciolo, U. H. de Faire, L. Fratiglioni, A. Hilding, K. Leander, et al. 2015. Traffic-related air pollution exposure and incidence of stroke in four cohorts from Stockholm. J. Expo. Sci. Environ. Epidemiol 25:517–23. doi:10.1038/jes.2015.22.
- Kubesch, N., A. De Nazelle, S. Guerra, D. Westerdahl, D. Martinez, L. Bouso, B. Neal, G. S. Hillis, N. Rafter, A. Tonkin, et al. 2015. Arterial blood pressure responses to short-term exposure to low and high traffic-related air pollution with and without moderate physical activity. Eur. J. Prev. Cardiol. 22:548–57. doi:10.1177/2047487314555602.
- Künzli, N., R. Kaiser, S. Medina, M. Studnicka, O. Chanel, P. Filliger, M. Herry, F. Horak, V. Puybonnieux-Texier, P. Quénel, et al. 2000. Public-health impact of outdoor and traffic-related air pollution: A European assessment. Lancet (London, England) 356:795–801. doi:10.1016/S0140-6736(00)02653-2.
- Laden, F., J. Schwartz, F. E. Speizer, and D. W. Dockery. 2006. Reduction in fine particulate air pollution and mortality: Extended follow-up of the Harvard Six Cities study. Am. J. Respir. Crit. Care Med. 173:667–72. doi:10.1164/rccm.200503-443OC.
- Laden, F., L. M. Neas, D. W. Dockery, and J. Schwartz. 2000a. Association of fine particulate matter from different sources with daily mortality in six U.S. cities. Environ. Health Perspect. 108:941–47. doi:10.1289/ehp.00108941.
- Laden, F., L. M. Neas, D. W. Dockery, and J. Schwartz. 2000b. Association of fine particulate matter from different sources with daily mortality in six U.S. cities. Environ. Health Perspect. 108:941–47. doi:10.1289/ehp.00108941.
- Lanki, T., J. J. de Hartog, J. Heinrich, G. Hoek, N. A. H. Janssen, A. Peters, M. Stölzel, K. L. Timonen, M. Vallius, E. Vanninen, et al. 2006. Can we identify sources of fine particles responsible for exercise-induced ischemia on days with elevated air pollution? The ULTRA study. Environ. Health Perspect. 114:655–60. doi:10.1289/ehp.8578.
- Lanki, T., R. Hampel, P. Tiittanen, S. Andrich, R. Beelen, B. Brunekreef, J. Dratva, U. De Faire, K. B. Fuks, B. Hoffmann, et al. 2015. Air pollution from road traffic and systemic inflammation in adults: A Cross-sectional analysis in the European ESCAPE Project. Environ. Health Perspect. 123:785–91. doi:10.1289/ehp.1408224.
- Lepeule, J., F. Laden, D. Dockery, and J. Schwartz. 2012. Chronic exposure to fine particles and mortality: An extended follow-up of the Harvard Six Cities study from 1974 to 2009. Environ. Health Perspect. 120:965–70. doi:10.1289/ehp.1104660.
- Li, W., K. S. Dorans, E. H. Wilker, M. B. Rice, P. L. Ljungman, J. D. Schwartz, B. A. Coull, P. Koutrakis, D. R. Gold, J. F. Keaney, et al. 2017. Short-term exposure to ambient air pollution and biomarkers of systemic inflammation: the framingham heart study. Arterioscler. Thromb. Vasc. Biol. 37:1793–800. doi:10.1161/ATVBAHA.117.309799.
- Liao, D., G. Heiss, V. M. Chinchilli, Y. Duan, A. R. Folsom, H.-M. Lin, and V. Salomaa. 2005. Association of criteria pollutants with plasma hemostatic/inflammatory markers: A population-based study. J. Expo. Sci. Environ. Epidemiol. 15:319–28. doi:10.1038/sj.jea.7500408.
- Libby, P., P. M. Ridker, and G. K. Hansson. 2009. Inflammation in Atherosclerosis. J. Am. Coll. Cardiol. 54:2129–38. doi:10.1016/j.jacc.2009.09.009.
- Lip, G. Y. 1995. Fibrinogen and cardiovascular disorders. J. Assoc. Physicians 88:155–65.
- Liu L, Ruddy T, Dalipaj M, Poon R, Szyszkowicz M, You H, R. E. Dales, A. J. Wheeler. 2009. Effects of indoor, outdoor, and personal exposure to particulate air pollution on cardiovascular physiology and systemic mediators in seniors. J. Occup. Environ. Med. 51:1088–98. doi:10.1097/JOM.0b013e3181b35144.
- Ljungman, P. L., E. H. Wilker, M. B. Rice, J. Schwartz, D. R. Gold, P. Koutrakis, J. A. Vita, G. F. Mitchell, R. S. Vasan, E. J. Benjamin, et al. 2014. Short-term exposure to air pollution and digital vascular function. Am. J. Epidemiol. 180:482–89. doi:10.1093/aje/kwu161.
- Ljungman, P. L., and M. A. Mittleman. 2014. Ambient air pollution and stroke. Stroke 45:3734–41. doi:10.1161/STROKEAHA.114.003130.
- Louwies, T., T. Nawrot, B. Cox, E. Dons, J. Penders, E. Provost, L. I. Panis, and P. De Boever. 2015. Blood pressure changes in association with black carbon exposure in a panel of healthy adults are independent of retinal microcirculation. Environ. Int. 75:81–86. doi:10.1016/j.envint.2014.11.006.
- Luttmann-Gibson, H., H. H. Suh, B. A. Coull, D. W. Dockery, S. E. Sarnat, J. Schwartz, P. H. Stone, and D. R. Gold. 2006. Short-term effects of air pollution on heart rate variability in senior adults in Steubenville, Ohio. J. Occup. Environ. Med. 48:780–88. doi:10.1097/01.jom.0000229781.27181.7d.
- Luttmann-Gibson, H., S. E. Sarnat, H. H. Suh, B. A. Coull, J. Schwartz, A. Zanobetti, and D. R. Gold. 2014. Short-term effects of air pollution on oxygen saturation in a cohort of senior adults in Steubenville, Ohio. J. Occup. Environ. Med. 56:149–54. doi:10.1097/JOM.0000000000000089.
- Madrigano, J., A. Baccarelli, R. O. Wright, H. Suh, D. Sparrow, P. S. Vokonas, and J. Schwartz. 2010. Air pollution, obesity, genes and cellular adhesion molecules. Occup. Environ. Med. 67:312–17. doi:10.1136/oem.2009.046193.
- Maheswaran, R., and P. Elliott. 2003. Stroke mortality associated with living near main roads in England and wales: A geographical study. Stroke 34:2776–80. doi:10.1161/01.STR.0000101750.77547.11.
- Maynard, D., B. A. Coull, A. Gryparis, and J. Schwartz. 2007. Mortality risk associated with short-term exposure to traffic particles and sulfates. Environ. Health Perspect. 115:751–55. doi:10.1289/ehp.9537.
- Medina-Ramón, M., R. Goldberg, S. Melly, M. A. Mittleman, and J. Schwartz. 2008. Residential exposure to traffic-related air pollution and survival after heart failure. Environ. Health Perspect 116:481–85. doi:10.1289/ehp.10918.
- Miller KA, Siscovick DS, Sheppard L, Shepherd K, Sullivan JH, Anderson GL, and J. D. Kaufman. 2007. Long-term exposure to air pollution and incidence of cardiovascular events in women. N. Engl. J. Med. 356:447–58. doi:10.1056/NEJMoa054409.
- Mordukhovich, I., B. Coull, I. Kloog, P. Koutrakis, P. Vokonas, and J. Schwartz. 2015. Exposure to sub-chronic and long-term particulate air pollution and heart rate variability in an elderly cohort: The Normative Aging Study. Environ. Health 14:87. doi:10.1186/s12940-015-0074-z.
- Mordukhovich, I., E. Wilker, H. Suh, R. Wright, D. Sparrow, P. S. Vokonas, and J. Schwartz. 2009. Black carbon exposure, oxidative stress genes, and blood pressure in a repeated-measures study. Environ. Health Perspect. 117:1767–72. doi:10.1289/ehp.0900591.
- Mostofsky, E., J. Schwartz, B. A. Coull, P. Koutrakis, G. A. Wellenius, H. H, et al. Suh. 2012. Modeling the association between particle constituents of air pollution and health outcomes. Am. J. Epidemiol. 176:317–326. doi:10.1093/aje/kws018.
- O’Neill, M. S., A. Veves, J. A. Sarnat, A. Zanobetti, D. R. Gold, P. A. Economides, et al. 2007. Air pollution and inflammation in type 2 diabetes: a mechanism for susceptibility. Occup. Environ. Med. 64:373–9. doi:10.1136/oem.2006.030023.
- Odemuyiwa, O., M. Malik, T. Farrell, Y. Bashir, J. Poloniecki, and J. Camm. 1991. Comparison of the predictive characteristics of heart rate variability index and left ventricular ejection fraction for all-cause mortality, arrhythmic events and sudden death after acute myocardial infarction. Am. J. Cardiol. 68:434–39. doi:10.1016/0002-9149(91)90774-F.
- Pan, L., S. Wu, H. Li, J. Xu, W. Dong, J. Shan, X. Yang, Y. Chen, M. Shima, F. Deng, et al. 2018b. The short-term effects of indoor size-fractioned particulate matter and black carbon on cardiac autonomic function in COPD patients. Environ. Int. 112:261–68. doi:10.1016/j.envint.2017.12.037.
- Pan, L., W. Dong, H. Li, M. R. Miller, Y. Chen, M. Loh, S. Wu, J. Xu, X. Yang, M. Shima, et al. 2018a. Association patterns for size-fractioned indoor particulate matter and black carbon and autonomic function differ between patients with chronic obstructive pulmonary disease and their healthy spouses. Environ. Pollut. 236:40–48. doi:10.1016/j.envpol.2018.01.064.
- Panasevich, S., K. Leander, M. Rosenlund, P. Ljungman, T. Bellander, U. de Faire, G. Pershagen, and F. Nyberg. 2009. Associations of long- and short-term air pollution exposure with markers of inflammation and coagulation in a population sample. Occup. Environ. Med. 66:747–53. doi:10.1136/oem.2008.043471.
- Park, S. K., M. S. O’Neill, B. J. B. Stunder, P. S. Vokonas, D. Sparrow, P. Koutrakis, and J. Schwartz. 2007. Source location of air pollution and cardiac autonomic function: Trajectory cluster analysis for exposure assessment. J. Expo. Sci. Environ. Epidemiol. 17:488–97. doi:10.1038/sj.jes.7500552.
- Park, S. K., M. S. O’Neill, P. S. Vokonas, D. Sparrow, and J. Schwartz. 2005. Effects of air pollution on heart rate variability: The VA normative aging study. Environ. Health Perspect. 113:304–09. doi:10.1289/ehp.7447.
- Pieters, N., M. Plusquin, B. Cox, M. Kicinski, J. Vangronsveld, and T. S. Nawrot. 2012. An epidemiological appraisal of the association between heart rate variability and particulate air pollution: A meta-analysis. Heart 98:1127–35. doi:10.1136/heartjnl-2011-301505.
- Pope, C. A., R. L. Verrier, E. G. Lovett, A. C. Larson, M. E. Raizenne, R. E. Kanner, J. Schwartz, G. M. Villegas, D. R. Gold, and D. W. Dockery. 1999. Heart rate variability associated with particulate air pollution. Am. Heart J. 138:890–99. doi:10.1016/S0002-8703(99)70014-1.
- Pope, C. A., R. T. Burnett, G. D. Thurston, M. J. Thun, E. E. Calle, D. Krewski, and J. J. Godleski. 2004. Cardiovascular mortality and long-term exposure to particulate air pollution: Epidemiological evidence of general pathophysiological pathways of disease. Circulation 109:71–77. doi:10.1161/01.CIR.0000108927.80044.7F.
- Pope CA, Burnett RT, Thun MJ, Calle EE, Krewski D, Ito K, and G. D. Thurston. 2002. Lung cancer, cardiopulmonary mortality, and long-term exposure to fine particulate air pollution. JAMA 287:1132–41. doi:10.1001/jama.287.9.1132.
- Pradhan, A. D., N. Rifai, and P. M. Ridker. 2002. Soluble intercellular adhesion molecule-1, soluble vascular adhesion molecule-1, and the development of symptomatic peripheral arterial disease in men. Circulation 106:820–25. doi:10.1161/01.CIR.0000025636.03561.EE.
- Rice, M. B., P. L. Ljungman, E. H. Wilker, K. S. Dorans, D. R. Gold, J. Schwartz, P. Koutrakis, G. R. Washko, G. T. O’Connor, and M. A. Mittleman. 2015. Long-term exposure to traffic emissions and fine particulate matter and lung function decline in the Framingham heart study. Am. J. Respir. Crit. Care Med. 191:656–64. doi:10.1164/rccm.201410-1875OC.
- Rosenbloom, J. I., E. H. Wilker, K. J. Mukamal, J. Schwartz, and M. A. Mittleman. 2012. Residential proximity to major roadway and 10-year all-cause mortality after myocardial infarction. Circulation 125:2197–203. doi:10.1161/CIRCULATIONAHA.111.085811.
- Rosenlund, M., N. Berglind, G. Pershagen, J. Hallqvist, T. Jonson, and T. Bellander. 2006. Long-term exposure to urban air pollution and myocardial infarction. Epidemiology 17:383–90. doi:10.1097/01.ede.0000219722.25569.0f.
- Rosenlund, M., S. Picciotto, F. Forastiere, M. Stafoggia, and C. A. Perucci. 2008. Traffic-related air pollution in relation to incidence and prognosis of coronary heart disease. Epidemiology 19:121–28. doi:10.1097/EDE.0b013e31815c1921.
- Rückerl R, Greven S, Ljungman P, Aalto P, Antoniades C, Bellander T, N. Berglind, C. Chrysohoou, F. Forastiere, and B. Jacquemin. 2007. Air pollution and inflammation (interleukin-6, C-reactive protein, fibrinogen) in myocardial infarction survivors. Environ. Health Perspect. 115:1072–80. doi:10.1289/ehp.10021.
- Rückerl, R., R. Hampel, S. Breitner, J. Cyrys, U. Kraus, J. Carter, L. Dailey, R. B. Devlin, D. Diaz-Sanchez, W. Koenig, et al. 2014. Associations between ambient air pollution and blood markers of inflammation and coagulation/fibrinolysis in susceptible populations. Environ. Int. 70:32–49. doi:10.1016/j.envint.2014.05.013.
- Schwartz J, Litonjua A, Suh H, Verrier M, Zanobetti a, Syring M, Nearing B, R. Verrier, P. Stone, and G. MacCallum, et al. 2005. Traffic related pollution and heart rate variability in a panel of elderly subjects. Thorax 60:455–61. doi:10.1136/thx.2004.024836.
- Schwartz, J., S. E. Alexeeff, I. Mordukhovich, A. Gryparis, P. Vokonas, H. Suh, and B. A. Coull. 2012. Association between long-term exposure to traffic particles and blood pressure in the Veterans Administration Normative Aging Study. Occup. Environ. Med. 69:422–27. doi:10.1136/oemed-2011-100268.
- Seaton, A., W. MacNee, K. Donaldson, and D. Godden. 1995. Particulate air pollution and acute health effects. Lancet (London, England) 345:176–78. doi:10.1016/S0140-6736(95)90173-6.
- Shakya, K. M., R. E. Peltier, Y. Zhang, and B. D. Pandey. 2019. Roadside Exposure and Inflammation Biomarkers among a Cohort of Traffic Police in Kathmandu, Nepal. Int. J. Environ. Res. Public Health 16. doi:10.3390/ijerph16030377.
- Shields KN, Cavallari JM, Hunt MJO, Lazo M, Molina M, Molina L, and F. Holguin. 2013. Traffic-related air pollution exposures and changes in heart rate variability in Mexico City: A panel study. Environ. Health 12:7. doi:10.1186/1476-069X-12-7.
- Sørensen, M., B. Hoffmann, M. Hvidberg, M. Ketzel, S. S. Jensen, Z. J. Andersen, A. Tjønneland, K. Overvad, and O. Raaschou-Nielsen. 2012. Long-term exposure to traffic-related air pollution associated with blood pressure and self-reported hypertension in a Danish cohort. Environ. Health Perspect. 120:418–24. doi:10.1289/ehp.1103631.
- Steinvil, A., L. Kordova-Biezuner, I. Shapira, S. Berliner, and O. Rogowski. 2008. Short-term exposure to air pollution and inflammation-sensitive biomarkers. Environ. Res. 106:51–61. doi:10.1016/j.envres.2007.08.006.
- Sun Y, Song X, Han Y, Ji Y, Gao S, Shang Y, S. E. Lu, T. Zhu, and W. Huang. 2015. Size-fractioned ultrafine particles and black carbon associated with autonomic dysfunction in subjects with diabetes or impaired glucose tolerance in Shanghai, China. Part. Fibre Toxicol. 12:8. doi:10.1186/s12989-015-0084-6.
- Suwa, T., and J. Hogg. 2002. Particulate air pollution induces progression of atherosclerosis. Journal of the American College of Cardiology 39:935–42.
- Tsuji, H., M. G. Larson, F. J. Venditti, E. S. Manders, J. C. Evans, C. L. Feldman, and D. Levy. 1996. Impact of reduced heart rate variability on risk for cardiac events. The Framingham Heart Study. Circulation 94:2850–55. doi:10.1161/01.CIR.94.11.2850.
- Van Hee VC, Adar SD, Szpiro AA, Barr RG, Bluemke DA, Diez Roux A V, E. A. Gill, L. Sheppard, and J. D. Kaufman. 2009. Exposure to traffic and left ventricular mass and function: The multi-ethnic study of atherosclerosis. Am. J. Respir. Crit. Care Med. 179:827–34. doi:10.1164/rccm.200808-1344OC.
- van Rossem, L., S. L. Rifas-Shiman, S. J. Melly, I. Kloog, H. Luttmann-Gibson, A. Zanobetti, B. A. Coull, J. D. Schwartz, M. A. Mittleman, E. Oken, et al. 2015. Prenatal air pollution exposure and newborn blood pressure. Environ. Health Perspect. 123:353–59. doi:10.1289/ehp.1307419.
- Vivanco-Hidalgo, R. M., G. A. Wellenius, X. Basagaña, M. Cirach, A. G. González, P. de Ceballos, A. Zabalza, J. Jiménez-Conde, C. Soriano-Tarraga, E. Giralt-Steinhauer, et al. 2018. Short-term exposure to traffic-related air pollution and ischemic stroke onset in Barcelona, Spain. Environ. Res 162:160–65. doi:10.1016/j.envres.2017.12.024.
- von Klot S, Gryparis A, Tonne C, Yanosky J, Coull BA, Goldberg RJ, D. Lessard, S. J. Melly, H. H. Suh, and J. Schwartz. 2009. Elemental carbon exposure at residence and survival after acute myocardial infarction. Epidemiology 20:547–54. doi:10.1097/EDE.0b013e31819d9501.
- Weichenthal, S., M. Hatzopoulou, and M. S. Goldberg. 2014. Exposure to traffic-related air pollution during physical activity and acute changes in blood pressure, autonomic and micro-vascular function in women: A cross-over study. Part. Fibre Toxicol. 11:70. doi:10.1186/s12989-014-0070-4.
- Weichenthal, S., R. Kulka, A. Dubeau, C. Martin, D. Wang, and R. Dales. 2011. Traffic-related air pollution and acute changes in heart rate variability and respiratory function in urban cyclists. Environ. Health Perspect. 119:1373–78. doi:10.1289/ehp.1003321.
- Wellenius, G. A., C. S. Wilhelm-Benartzi, E. H. Wilker, B. A. Coull, H. H. Suh, P. Koutrakis, et al. 2012. Ambient Particulate Matter and the Response to Orthostatic Challenge in the Elderly. Hypertension 59:558–563. doi:10.1161/hypertensionaha.111.180778.
- Wilker, E. H., A. Baccarelli, H. Suh, P. Vokonas, R. O. Wright, and J. Schwartz. 2010. Black carbon exposures, blood pressure, and interactions with single nucleotide polymorphisms in microRNA processing genes. Environ. Health Perspect. 118:943–48. doi:10.1289/ehp.0901440.
- Wilker, E. H., E. Mostofsky, S.-H. Lue, D. Gold, J. Schwartz, G. A. Wellenius, and M. A. Mittleman. 2013. Residential proximity to high-traffic roadways and poststroke mortality. J. stroke Cerebrovasc. Dis 22:e366–72. doi:10.1016/j.jstrokecerebrovasdis.2013.03.034.
- Wilker, E. H., S. R. Preis, A. S. Beiser, P. A. Wolf, R. Au, I. Kloog, W. Li, J. Schwartz, P. Koutrakis, C. DeCarli, et al. 2015. Long-term exposure to fine particulate matter, residential proximity to major roads and measures of brain structure. Stroke 46:1161–66. doi:10.1161/STROKEAHA.114.008348.
- Wilker EH, Ljungman PL, Rice MB, Kloog I, Schwartz J, Gold DR, P. Koutrakis, J. A. Vita, G. F. Mitchell, R. S. Vasan, et al. 2014. Relation of long-term exposure to air pollution to brachial artery flow-mediated dilation and reactive hyperemia. Am. J. Cardiol. 113:2057–63. doi:10.1016/j.amjcard.2014.03.048.
- Wittkopp, S., N. Staimer, T. Tjoa, D. Gillen, N. Daher, M. Shafer, J. J. Schauer, C. Sioutas, R. J. Delfino, and T. Behrens. 2013. Mitochondrial genetic background modifies the relationship between traffic-related air pollution exposure and systemic biomarkers of inflammation. PLoS ONE 8:e64444. doi:10.1371/journal.pone.0064444.
- Zanobetti A, Coull BA, Gryparis A, Kloog I, Sparrow D, Vokonas PS, R. O. Wright, D. R. Gold, and J. Schwartz. 2014. Associations between arrhythmia episodes and temporally and spatially resolved black carbon and particulate matter in elderly patients. Occup. Environ. Med. 71:201–07. doi:10.1136/oemed-2013-101526.
- Zanobetti, A., D. R. Gold, P. H. Stone, H. H. Suh, J. Schwartz, B. A. Coull, and F. E. Speizer. 2010. Reduction in heart rate variability with traffic and air pollution in patients with coronary artery disease. Environ. Health Perspect 118:324–30. doi:10.1289/ehp.0901003.
- Zanobetti, A., M. J. Canner, P. H. Stone, J. Schwartz, D. Sher, E. Eagan-Bengston, K. A. Gates, L. H. Hartley, H. Suh, and D. R. Gold. 2004a. Ambient pollution and blood pressure in cardiac rehabilitation patients. Circulation 110:2184–89. doi:10.1161/01.CIR.0000143831.33243.D8.
- Zanobetti, A., M. J. Canner, P. H. Stone, J. Schwartz, D. Sher, E. Eagan-Bengston, K. A. Gates, L. H. Hartley, H. Suh, and D. R. Gold. 2004b. Ambient pollution and blood pressure in cardiac rehabilitation patients. Circulation 110:2184–89. doi:10.1161/01.CIR.0000143831.33243.D8.
- Zanobetti, A., P. H. Stone, F. E. Speizer, J. D. Schwartz, B. A. Coull, H. H. Suh, B. D. Nearing, M. A. Mittleman, R. L. Verrier, and D. R. Gold. 2009. T-wave alternans, air pollution and traffic in high-risk subjects. Am. J. Cardiol. 104:665–70. doi:10.1016/j.amjcard.2009.04.046.
- Zeger, S. L., D. Thomas, F. Dominici, J. M. Samet, J. Schwartz, D. Dockery, and A. Cohen. 2000. Exposure measurement error in time-series studies of air pollution: Concepts and consequences. Environ. Health Perspect. 108:419–26. doi:10.1289/ehp.00108419.
- Zeka, A., J. R. Sullivan, P. S. Vokonas, D. Sparrow, and J. Schwartz. 2006. Inflammatory markers and particulate air pollution: Characterizing the pathway to disease. Int. J. Epidemiol. 35:1347–54. doi:10.1093/ije/dyl132.
- Zhong, J., A. Cayir, L. Trevisi, M. Sanchez-Guerra, X. Lin, C. Peng, M.-A. Bind, D. Prada, H. Laue, K. J. M. Brennan, et al. 2016. Traffic-related air pollution, blood pressure, and adaptive response of mitochondrial abundance. Circulation 133:378–87. doi:10.1161/CIRCULATIONAHA.115.018802.
- Zhu, Y., W. C. Hinds, S. Shen, and C. Sioutas. 2004. Seasonal trends of concentration and size distribution of ultrafine particles near major highways in Los Angeles special issue of aerosol science and technology on findings from the fine particulate matter supersites program. Aerosol Sci. Technol. 38:5–13. doi:10.1080/02786820390229156.