ABSTRACT
A low-cost air sensor package was used to monitor indoor air quality (IAQ) in a classroom at the Albany Middle School in the San Francisco Bay Area of California. A rapid increase in carbon dioxide (CO2) was observed in the classroom as soon as it is occupied. When the classroom is unoccupied, the CO2 levels decay slowly toward the outdoor background level. All high CO2 concentrations observed inside the classroom, above the outdoor background, was due to exhaling of the occupants. The CO2 concentrations generally exceed the recommended level of 1000 ppb towards the end of the school day. The exceedances and slow decay may suggest that the ventilation rate in this school is not sufficient. The particulate level in the classroom was low until a distant wildfire advected large amount of particulate matter to the San Francisco Bay Area. Very high (10–15 times compared to the background) particle numbers (per m3 of particles with diameter >0.3 µm) were observed in the classroom during the wildfire. These particles were relatively small (0.3–1.0 µm) and the filters (MERV 8) of the ventilation system were unable to filter them out. Therefore, the measurements made by low-cost particle counters can inform the school administrators of adverse IAQ during future wildfire (or other combustion) events. The particle number was independent of the occupation before and during the wildfire suggesting that all observed particles were infiltrated into the classroom from outside. Consistent with previous studies, no appreciable increase in the local ambient CO2 background was observed during this distant wildfire event.
Implications: Low-cost air sensors are effective in monitoring indoor air quality in classrooms. The CO2 levels in classrooms are mainly generated indoors due to exhalation of occupants. Concentration of CO2 generally exceed the recommended level of 1000 ppb towards the end of the school day. In contrast, the particulate matter mostly comes from outdoors and small particles penetrate though the filters normally used at schools. Distant wildfires do not increase the local CO2 background appreciably, but significantly increase the particulate matter concentrations both indoors and outdoors. Further investigations are needed to assure that ventilation rates in classrooms are sufficiently health protective.
Introduction
The number of deaths of people worldwide due to polluted air now surpasses the number of deaths due to tobacco use, AIDS, tuberculosis, or malaria. It also causes a significant amount of non-communicable diseases (Landrigan Citation2017). A part of these deaths/diseases are due to poor indoor air quality (IAQ) which has historically received less attention than the outdoor (ambient) air pollution. Children are more susceptible to air pollution because their lungs are still growing and they inhale more air (per unit body weight) than adults do (American Lung Association Citation2018). A large, long-term study of the effects of chronic air pollution exposures on the health of children living in Southern California not only confirmed that health of children is adversely affected by air pollution but improvements in air quality can be beneficial to children’s health (Gauderman et al. Citation2004, Citation2015). Students spend roughly one-third of their weekdays in school buildings during the regular school year, and the IAQ in schools has now become an important area of scientific inquiry that also has Environmental Justice implications (Grineski and Collins Citation2018).
Albany is a small town (~1.7 square miles and a population of ~20,000) located just north of Berkeley, California (Albany Citation2018) in the San Francisco Bay Area. It has one high school, one middle school, and three elementary schools (AUSD Citation2018). There are no major emissions sources in Albany except for the Interstate 80 that runs along the western border. A group of students formed an Air Quality Club at the Albany High School (AHS) in 2017. They have since built a simple air monitoring package (or a sensor package) using low-cost air sensor for particulate matter, carbon dioxide (CO2), and meteorological parameters (temperature, barometric pressure, and relative humidity). More details of this package are provided in the next section. As their first project, they conducted a short-term (~3 months) air monitoring campaign in one classroom at the Albany Middle School (AMS). Since then they have built more sensor packages and are now monitoring IAQ in additional classrooms with the goal of evaluating the effectiveness of the Heating, Ventilation, and Air Conditioning (HVAC) system.
During the monitoring campaign at AMS, a large (~150,000 acres burnt) wildland fire named Camp Fire (CAL FIRE Citation2018) started on November 8, 2018, in the upper Sacramento Valley of California in a rural area near the town of Paradise. This devastating early-winter fire was finally fully contained thanks to a rainstorm that began on November 21, 2018. Although it was ~200 km north-northeast of Albany, the effects of the fire (visible smoke and a burning odor) were felt throughout the San Francisco Bay Area. While there were numerous wildfires in northern California in the past, this is the first time in decades that the Bay Area was so severely impacted by a distant wildfire. The fire plumes were brought south by the northerly winds prevalent in the upper Sacramento Valley during the winter months, and the Bay Area was a convenient outlet for the plumes to flow into the Pacific Ocean. Thus, the time of the year and the location of the fire made this a rare and prolonged pollution event in the Bay Area.
The monitoring period started well before the onset of the wildfire (November 8, 2018) and, thus we were able to compare and contrast the observations made before and during the wildfire.
It is important to note here that this monitoring campaign augmented the Science, Technology, Engineering, and Mathematics (STEM) education curriculum (Dou et al. Citation2019) at the Albany schools. The STEM education is encouraged by the U.S. Department of Education (https://www.ed.gov/stem). This project was also used to make students aware of the rapidly-growing Community Science efforts (Phillips et al. Citation2019) especially related to air pollution. A near-future goal of this project is to develop a Community Science program in Albany, California with the schools as science hubs.
Technical details of the sensor package
shows the basic sensor package. It consists of a BME280 (temperature, barometric pressure, and relative humidity), a PMS7003 (particulate matter), and an MH-Z19 (CO2) sensors. The sensors were primarily selected based on their low cost and ease of interfacing with a computer: A necessary requirements for novice high-school students. A secondary condition for selection was the widespread use by the community science groups elsewhere.
Figure 1. The sensor package used in this study. The figure shows the sensors as well as their spatial arrangement into a compact package that can be easily moved to a desired location with electricity.
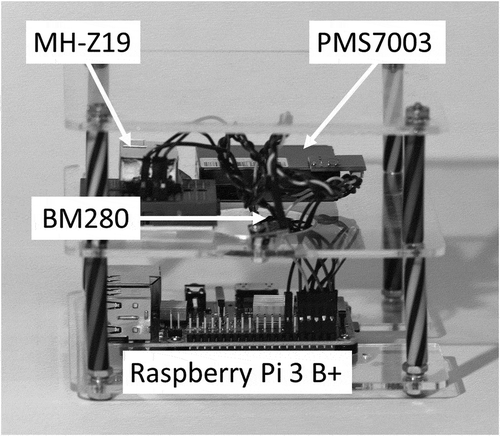
The BME280 is a miniature-integrated sensor package produced by Bosch Sensortec for mobile applications (Bosch Citation2018). This sensor package is readily available from several vendors as breakout boards suitable for our application.
PMS7003 is the latest version of the Plantower particle counter series. The previous version (PMS5003) is used in the Purple Air sensor that is widely used for low-cost indoor and community air monitoring (Purple Air Citation2017). This sensor is also evaluated by the Air Quality Sensor Performance Evaluation Center of the South Coast Air Quality Management District (AQ-SPEC Citation2018). This evaluation is based on the particulate matter mass (which is a criteria pollutant) and not on the particle number (which is not a criteria pollutant in the US, but we use in this paper). However, this sensor is not an approved Federal Equivalent Method (FEM) for particulate matter with aerodynamic diameter less than 10 µm (PM10) or that less than 2.5 µm (PM2.5) measurements (U.S. EPA Citation2016). Therefore, measurements made with this sensor cannot be used in any regulatory application. Also, the algorithm that PMS7003 uses to calculate particulate matter (PM) mass from particle number is not available to the public. Due to this proprietary nature of the calculation, and other reasons described later in this document, we have decided not to use the PM mass measured by PMS7003 in our study. Instead, we use the measured particle number with diameter above 0.3 µm per cubic meter of air. The diameter used here defined with respect to particles interaction with light and may be different from the aerodynamic diameter (Seinfeld and Pandis Citation2006) used in the regulatory context. Since the particulate matter mass calculated with the measured particulate number distribution and AQ-SPEC deems it to be satisfactory, we assume the particulate number distribution to be satisfactory as well.
MH-Z19 measures CO2 using the Non-dispersive infrared (NDIR) spectroscopy. CO2 is not a criteria pollutant under the Federal Clean Air Act, and there are no Federal Reference Method (FRM) or Federal Equivalent Methods (FEM) to measure it (U.S. EPA Citation2018). However, the global background CO2 measurements conducted at the Mauna Loa Observatory in Hawaii uses infrared spectroscopy (NOAA Citation2018). The auto calibration feature of MH-Z19 was used for the measurements in this study, since the school lacks resources for a more careful calibration using standard gases. However, evidence gathered during this study (and described later) indicates that the auto calibration was satisfactory for the purpose of this study.
These low-cost sensors are directly controlled by a Raspberry Pi Version 3 B+ (RPi) computer (Raspberry Pi Citation2018) with the Raspbian Stretch operating system and the Python computer programming language. PRi itself is a versatile low-cost small single-board computer that has a system on chip (SOC). The RPi is powered with a 5 V adapter off the wall power and sensors are powered with RPi’s 5 or 3.3 V output pins in the general purpose input output (GPIO) header. More information on the sensors are provided in . The BME280 interfaces with the RPi using the Inter-Integrated Circuit (I2C) protocol. PMS7003 uses Universal Asynchronous Receiver/Transmitter (UART) connection, and the MH-Z19 was programmed with Pulse Width Modulation (PWM) mainly as a pedagogical tool to expose students to various sensor-computer communication methods/protocols.
Table 1. Information on the sensors used in this study.
Readers interested in building their own versions of the sensor package described in this section are encouraged to directly contact the corresponding author (Ajith Kaduwela, [email protected]) for details and assistance.
Observations and discussion
The sensor package was located in the back corner of a classroom at AMS roughly at the head height of the seated students. This classroom is connected to a central HVAC system that has filters with Minimum Efficiency Reporting Value 8 (MERV 8). We have not measured the efficiency of the HVAC system.
All data from the low-cost sensor package presented here were filtered using the Savitzky-Golay algorithm (Citation1964) to reduce the noise.
shows the CO2 and PM prior to the Camp Fire in the AMS classroom for a typical Monday, Wednesday, and a Saturday. Tuesday, Thursday, and Friday have the same schedule as Monday. Wednesday schedule is different because there is an Advisory period that day and to accommodate that other periods are shortened. Sunday is very similar to Saturday, and the school is not in session during the weekends.
Figure 2. Typical CO2 and PM number concentration at AMS prior to the Camp Fire. (a). Wednesday, October 01, 2018, (b). Wednesday, October 3, 2018, and (c). Saturday, October 6, 2018. In (a) and (b), the vertical-dashed lines are the school period demarcations. The leftmost line represents the beginning of the school (8 a.m.) and the rightmost line the end (2:10 pm on Wednesdays and 3:05 pm other days). The black solid line is for CO2 (ppm), and the black-dashed line is for PM (#/m3 for particles with diameter >0.3 µm). The periods where there are no scheduled classes in this room (including the lunch period) are shaded.
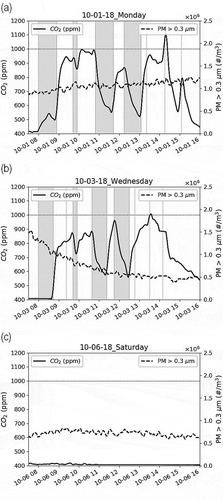
shows concentration variations during school hours prior to the wildfire period. As seen from panels (a) and (b), the CO2 concentrations increase very rapidly (within minutes) with the presence of students and decay slowly towards the background in the absence. The standard practice of the HVAC recirculation of in-school air (return air) predominantly with slow mixing of outside air (with background CO2 concentrations of ~400 ppm) into the school may be one of the reasons for this rapid increase and slow decay. This rise/fall of CO2 in the presence/absence of the occupants and the flat CO2 trace during the weekends (absence of occupants) strongly suggest that the CO2 observed above background during school hours is entirely due to the exhalation of the occupants of the school.
Also, this figure shows that there is no dependence on PM number concentration on occupation during school hours. shows the entire first week of October 2018. This figure shows the hours outside of schooltime.
shows that when the school is not occupied (evening to early morning through the night and during weekends) the CO2 concentrations reach the outdoor background levels. This suggests that the auto calibration of the MH-Z19 is satisfactory and further confirms that CO2 observed above background level is due to exhalation of school occupants.
Also, note in and that a horizontal line is drawn at 1000 ppm of CO2. This is the generally accepted guideline level (ASHRAE Citation1989) for indoor environments. Beyond this level, occupants begin to feel the ill effects of CO2. These may include (Norbäck, Nordström, and Zhao Citation2013) drowsiness, lethargy, stuffiness, and a feel that the air is stale. All these factors can interfere with learning.
The 1000 ppm standard for CO2 is exceeded almost every school day in this classroom towards the end of the day. This may indicate continuous buildup of CO2 indoors as the school day progresses and the HVAC may not be able to successfully mitigate the CO2 build up at the current operational levels.
In contrast, as seen in both and , the PM levels (#/m3 of particles with diameter >0.3 µm) appear to be independent of the presence/absence of occupants. There are no sources of PM in the classroom other than resuspension due to movement of occupants and that does not seem to be a major source. Thus, the small amounts of observed PM in the classroom (in the absence of a wildfire) seem to have come from outside.
The PM concentrations were elevated during the fire-impact days as shown in . Note that both y-axis scales are different between and . Wildfire plumes have severely impacted the Bay Area as seen by satellite images (NASA Citation2019) and, thus the 10–15 times increase in the PM in compared to and , but the CO2 concentrations have also increased some days for a different reason as explained later in this document.
As shown in , the measured particle number (#/m3 with diameter >0.3 µm) was compared with the PM2.5 mass (µg/m3) measured at two regulatory-grade Beta Attenuation Monitors (BAMs) in Berkeley (Aquatic Park, ~3.5 km south-south-west of AHS) and San Pablo (Rumrill Boulevard, ~9 km north-west). This BAM data can be seen near real time at the California Air Resource Board (CARB) mobile site (https://mobile.arb.ca.gov/breathewell/) and can be downloaded from the CARB Air Quality and Meteorological Information System (https://www.arb.ca.gov/aqmis2/aqmis2.php).
Figure 5. Comparison of AMS particle number measurement (solid black line) with BAM readings (black stars and dots) for PM2.5 mass.
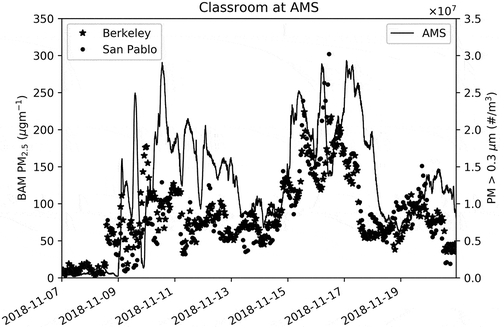
As shown in that the AMS particle number measurement tracks the CARB BAM PM2.5 mass measurement reasonably well. It should be stressed here that comparisons between particle number inside the classroom and the ambient particle mass at BAM monitors in are intended to be qualitative. Note here that we are comparing an indoor particle number for predominantly small particles (~75% in the 0.3–0.5 µm and ~20% in the 0.5–1.0 µm range) that does not contribute appreciably to mass (if one assumes that particles are spherical, then the mass ∝ radius3) with PM2.5 mass.
Another important difference is that the particle number is reported every ~10 sec and BAM readings are hourly. One could in principle calculate the hourly PM mass from the particle number. However, this calculation has several assumptions and the final PM mass calculated is not comparable with the regulatory PM standards. Also, the real-time nature of the measurement is lost in averaging and some class periods are less than an hour. Here, the intention is to show that real-time indoor particle number is a reasonably good indicator of outdoor regulatory PM mass and thus can be used by school administrators in real time, together with other indicators they routinely use, in their decision-making process.
There is a clear shift (delay) of the AMS particle numbers and the BAM PM mass. This may be due to the fact that it takes time for particles to penetrate through the HVAC system in to indoors, and it also takes time to clean particles out of the indoor air through the HVAC. Since we have not measured the efficiency of the HVAC system, we are unable to test this hypothesis at this time.
It is important to stress here that reasonably good agreement shown in is not expected for all pollution events. In some cases, we expect the PMS7003 to register much localized emission scenarios (e.g., idling diesel vehicle, a small local fire, or nearby construction work) that will not register at distant BAMs. Inversely, there could be some emissions events that register at either (or both) BAM and not at AMS.
Now turning to CO2 measurements reported in , as stated previously with reference to , the auto calibration of the MH-Z19 is successful and high CO2 concentrations observed indoors are due to exhalation of occupants. However, the CO2 levels were significantly above the 1000 ppm standard for a long period on November 13, 15, and 20 during the wildfire (). During the same wildfire period, CO2 levels during November 9, 14, and 19 were comparable to non-wildfire days. Also, note that 10–11 was a weekend and 12 was a holiday. The school was closed on 16 due to wildfire impacts and 17–18 was a weekend. As shown in the CO2 levels were near background during non-school hours and holidays. This supports our claim that the wildfire did not cause a noticeable increase in the regional CO2 background far downwind as AMS. The observation that distant wildfires do not increase the regional CO2 appreciably was also reported by Mallia et al. (Citation2015).
During the wildfire impact period, the AMS kept students indoors during the breaks (during which they would have gone outdoors otherwise). This could have caused anincrease in CO2 during this period. It is not clear however the reason for significant increase in CO2 some days and not others. Here, we are merely attempting to understand the CO2 increases somedays, and our effort should not be construed as questioning the decision made by school administrators to keep students indoors during breaks to shield them from wildfire smoke.
Since there was no outdoor CO2 sensor at AMS, the CO2 readings made during the wildfire period by the BErkeley Atmospheric CO2 Network (BEACO2N) (Shusterman et al. Citation2018) were investigated. The BEACO2N data (http://beacon.berkeley.edu/about/) at the Chabot Space & Science Center for this period did not exceed 475 ppm. This further confirms that there was no large outdoor CO2 enhancement due to Camp Fire and the elevated levels that were observed () could have been due to the exhalation of occupants.
The exposure to CO2 above the ASHRAE-recommended level of 1000 ppm can lead to increased school absences (Gaihre et al. Citation2014; Mendell et al. Citation2013), but Gaihre et al. (Citation2014) did not find evidence to support that these elevated levels would affect academic attainments. However, there is evidence that the attentional processes of students (Coley, Greeves, and Saxby Citation2007) and the decision-making performance (Satish et al. Citation2012) are inversely proportional to the CO2 levels. A follow-up to the study by Satish et al. (Citation2012) found that a 15% decline of cognitive ability scores among office workers at 950 ppm and 50% declines at 1400 ppm of CO2 (Allen et al. Citation2016). Yet, another study using different measures of mental ability reported no cognitive decline in a non-industrial setting even at 5000 ppm of CO2. Obviously, further studies in this area are needed given that outdoor CO2 concentrations are steadily increasing and classroom concentrations will continue to increase due to this increasing background.
The buildup of CO2 in classrooms is solely due to inadequate ventilation rates (Coley and Beisteiner Citation2002). California State Standard requires an outdoor air ventilation rate of 7.1 l/s/person (California Energy Commission Citation2016). Increasing classroom ventilation rates to or beyond California standard may increase the learning ability of students (Mendell et al. Citation2016) and decrease the illness-related absences. The additional cost of increased ventilation may be more than offset by attendance-linked funding to schools (Mendell et al. Citation2013).
Conclusion
A low-cost sensor package was used to measure IAQ in a classroom at AMS. As seen in , observations made using the sensor show that the CO2 concentrations in this room to generally exceed the ASHRAE recommended level of 1000 ppm towards the end of the school day. This could be due to the buildup CO2 during the day caused by inadequate ventilation rate of the HVAC system. Together with the low-cost CO2 sensor used in this study (MH-Z19), or any other suitable CO2 sensor, it should be possible to implement a demand-controlled ventilation system for the HVAC. However, safety and insurance requirements for schools may force them to purchase commercially available products. The particle loading is generally very low during the school hours, and the concentrations appear to be independent of the occupation of the classroom. However, during a distant wildfire, the particle number concentrations were much higher (10–15 times) than those during non-wildfire days in the classroom despite the HVAC system with MERV 8 filters. Therefore, we believe that school administrators can use the results of our low-cost particle number counter, together with other indicators they use, to manage IAQ during outdoor PM events. It is important to note that this study has not evaluated the effectiveness of the filter system directly. However, based on the experience of one of the authors at the California Environment Protection Agency (Green) building in Sacramento, temporarily upgrading to MERV 13–16 filter packs during wildfires, possibly with a charcoal pre-filter to eliminate burning smell, may be a potential remedy. We have also seen, consistent with previous studies, that distant wildfires do not increase the local CO2 levels (both indoor and outdoor) appreciably. However, we have seen increased CO2 indoors some wildfire days, but we were unable to find the cause of this increase. The school kept students inside during recesses to minimize their exposure to elevated particulate concentrations outside. We recommend that the indoor air quality impacts of this practice be investigated further in a future study.
Acknowledgment
Building of the sensor packages for this study was funded by a generous grant from the Albany Educational Fund to author Sean Morris. Jeffrey Castle (AHS) assisted with CAD/Laser cutting of the components for the sensor package. Caleb Foster (AHS) provided the photograph in .
Additional information
Funding
Notes on contributors
Ajith P. Kaduwela
Ajith P. Kaduwela ([email protected]) is a Professional Research Scientist at the Air Quality Research Center of the University of California at Davis.
Amal P. Kaduwela
Amal P. Kaduwela, Ely Jrade, and Matthew Brusseau are students at the Albany High School in California.
Sean Morris
Sean Morris ([email protected]) and Valerie Risk ([email protected]) are teachers at the Albany High School in California.
Jennifer Morris
Jennifer Morris ([email protected]) is a teacher at the Albany Middle School in California.
References
- Albany. 2018. Details on the city of Albany in the Alameda County of California. Accessed February 14, 2019. https://www.albanyca.org/our-city/our-community.
- Allen, J. G., P. MacNaughton, U. Satish, S. Santanam, J. Vallarino, and J. D. Spengler. 2016. Associations of cognitive function scores with carbon dioxide, ventilation, and volatile organic compound exposures in office workers: A controlled exposure study of green and conventional office environments. Environ. Health Perspect. 124:805–12. doi:10.1289/ehp.1510037.
- American Lung Association. 2018. Children and Air Pollution. Accessed February 14, 2019. https://www.lung.org/our-initiatives/healthy-air/outdoor/air-pollution/children-and-air-pollution.html.
- AQ-SPEC. 2018. Air quality sensor performance evaluation center of the South Coast air quality management district. Accessed February 14, 2019. http://www.aqmd.gov/aq-spec/evaluations.
- ASHRAE. 1989. Ventilation for acceptable indoor air quality. Atlanta, GA: American Society of Heating, Refrigerating and Air Conditioning Engineers (ASHRAE standard 62-1989).
- AUSD. 2018. Albany unified school district Accessed February 14, 2019. https://www.ausdk12.org/.
- Bosch. 2018. BME280 integrated environmental sensor by Bosch Sensortec Accessed February 14, 2019. https://www.bosch-sensortec.com/bst/products/all_products/bme280.
- CAL FIRE. 2018. California department of forestry and fire protection. Accessed February 14, 2019. http://www.fire.ca.gov/current_incidents/incidentdetails/Index/2277.
- California Energy Commission.2016. For more information of minimum ventilation rates mandated for buildings by the California code of regulations. Accessed February 14, 2019. https://www.energy.ca.gov/title24/2016standards/.
- Coley, D. A., and A. Beisteiner. 2002. Carbon dioxide levels and ventilation rates in schools. Int. J. Vent. 1 (1):45–52. doi:10.1080/14733315.2002.11683621.
- Coley, D. A., R. Greeves, and B. K. Saxby. 2007. The effect of low ventilation rates on the cognitive function of a primary school class. Int. J. Vent. 6 (2):107–12. doi:10.1080/14733315.2007.11683770.
- Dou, R., Z. Hazari, K. Dabney, G. Sonnert, and P. Sadler. 2019. Early informal STEM experiences and STEM identity: The importance of talking science. Sci. Educ. 103 (3):623–37. doi:10.1002/sce.21499.
- Gaihre, S., S. Semple, J. Miller, S. Fielding, and S. Turner. 2014. Classroom carbon dioxide concentration, school attendance, and educational attainment. J. Sch. Health. 84 (9):569–74. doi:10.1111/josh.12183.
- Gauderman, W. J., E. Avol, F. Gilliland, H. Vora, D. Thomas, K. Berhane, R. McConnell, N. Kuenzli, F. Lurmann, E. Rappaport, et al. 2004. The effect of air pollution on lung development from 10 to 18 years of age. N. Engl. J. Med. 351:1057–67. doi:10.1056/NEJMoa040610.
- Gauderman, W. J., R. Urman, E. Avol, K. Berhane, R. McConnell, E. Rapport, R. Chang, F. Lurmann, and F. Gilliland. 2015. Association of improved air quality with lung development in children. N. Engl. J. Med. 372:905–13. doi:10.1056/NEJMoa1414123.
- Grineski, S. E., and T. W. Collins. 2018. Geographic and social disparities in exposure to air neurotoxicants at U.S. public schools. Environ. Res. 161:580–87. doi:10.1016/j.envres.2017.11.047.
- Landrigan, P. J. 2017. Air pollution and health. Lancet 2 (1):e1–e55. doi:10.1016/S2468-2667(16)30023-8.
- Mallia, D. V., J. C. Lin, S. Urbanski, J. Ehleringer, and T. Nehrkorn. 2015. Impacts of upwind wildfire emissions on CO, CO2, and PM2.5 concentrations in Salt Lake City, Utah. J. Geophys. Res. - Atom. 120 (1):147–66. doi:10.1002/2014JD022472.
- Mendell, M. J., E. A. Eliseeva, M. M. Davies, and A. Lobscheid. 2016. Do classroom ventilation rates in California elementary schools influence standardized test scores? Results from a prospective study. Indoor Air 26 (4):546–57. doi:10.1111/ina.12241.
- Mendell, M. J., E. A. Eliseeva, M. M. Davies, M. Spears, A. Lobscheid, W. J. Fisk, and M. G. Apte. 2013. Association of classroom ventilation with reduced illness absence: A prospective study in California elementary schools. Indoor Air 23:515–28. doi:10.1111/ina.12042.
- NASA. 2019. California Shrouded in Smoke from the Ongoing Camp Fire. Accessed May 8, 2019. https://www.nasa.gov/image-feature/goddard/2018/california-shrouded-in-smoke-from-the-ongoing-camp-fire.
- NOAA. 2018. How we measure background CO2 levels on Mauna Loa. Accessed February 14, 2019. https://www.esrl.noaa.gov/gmd/ccgg/about/co2_measurements.html.
- Norbäck, D., K. Nordström, and Z. Zhao. 2013. Carbon dioxide (CO2) demand-controlled ventilation in university computer classrooms and possible effects on headache, fatigue and perceived indoor environment: An intervention study. Int. Arch. Occup. Environ. Health 86 (2):199–209. doi:10.1007/s00420-012-0756-6.
- Phillips, T. B., H. L. Ballard, B. V. Lewenstein, and R. Bonney. 2019. Engagement in science through citizen science: Moving beyond data collection. Sci. Educ. 103 (3):665–90. doi:10.1002/sce.21501.
- Purple Air. 2017. PurpleAir: Air Quality Monitoring. Accessed February 14, 2019. https://www.purpleair.com/.
- Raspberry Pi. 2018. Accessed February 14, 2019. https://www.raspberrypi.org/products/raspberry-pi-3-model-b-plus/.
- Satish, U., M. J. Mendell, K. Shekhar, T. Hotchi, D. Sullivan, S. Streufert, and W. J. Fisk. 2012. Is CO2 an indoor pollutant? Direct effects of low-to-moderate CO2 Concentrations on human decision-making performance. Environ. Health Perspect. 120 (12):1671–77. doi:10.1289/ehp.1104789.
- Savitzky, A., and M. J. E. Golay. 1964. Smoothing and differentiation of data by simplified least squares procedures. Anal. Chem. 36 (8):1627–39. doi:10.1021/ac60214a047.
- Seinfeld, J. H., and S. N. Pandis. 2006. Atmospheric chemistry and physics: From air pollution to climate change. Hoboken, NJ: J. Wiley.
- Shusterman, A. A., J. Kim, K. J. Lieschke, C. Newman, P. J. Wooldridge, and R. C. Cohen. 2018. Observing local CO2 sources using low-cost, near-surface urban monitors. Atom. Phys. Chem. 18 (18):13773–85. doi:10.5194/acp-18-13773-2018.
- U.S. EPA. 2016. Reference and equivalent methods used to measure National Ambient Air Quality Standards (NAAQS) criteria air pollutants - Volume I. https://cfpub.epa.gov/si/si_public_record_report.cfm?Lab=NERL&dirEntryId=321491 (accessed February 14, 2019).
- U.S. EPA. 2018. Accessed February 14, 2019. https://www.epa.gov/criteria-air-pollutants/naaqs-table.