ABSTRACT
With the increase in the cognizance toward the wide and abundant lignocellulosic biomass, a great interest has been garnered toward the production of value-added products from the biomass. Hence, by capitalizing the Casuarina equisetifolia biomass, the current work developed a simultaneous pre-treatment and saccharification (SPS) process using transgenic Trichoderma atroviride. The ability of T. atroviride to produce lignolytic and cellulolytic enzymes was enhanced by optimizing key process parameters. Under the optimized conditions, a maximum of 1245.6 U/kg of cellulase and 1203.36 U/kg of xylanase, 183.2 U/kg of laccase along with 392.36 g/kg of fermentable sugars were obtained. On comparing with acid and alkaline pre-treatment methods, the T. atroviride -mediated SPS process exhibited trace formation of fermentative inhibitors, which resulted in a minimal inhibition of Escherichia coli. Overall, the current work implements the biorefinery concept on Casuarina equisetifolia biomass by advocating circular economy.
Implications: Valorization of lignocellulosic waste biomass into value added compound and as biofuel is considered as a promising alternative resource, owing to its availability and low production cost. However, the presence of chemically resistant lignin demands an intensive treatment process, which sometimes leads to the formation of fermentative inhibitors. Casuarina equisetifolia is a deciduous commercial plant, and an average of 125 tonnes/hector of waste is generated annually in India. By considering the demerit of delignification and the wide availability of Casuarina equisetifolia biomass (CB), the current work aimed at the development of a single-pot simultaneous pre-treatment and saccharification (SPS) of CB by transgenic Trichoderma atroviride.
Introduction
Lignocellulosic biomass, a most promising renewable carbon source, covers 60% of earth plant biomass with the availability of 170–200 × 109 tones/day worldwide for long-term solution to energy generation (Láinez et al. Citation2018). The hierarchical self-assembly architecture of the lignocellulosic biomass, with the interaction of lignin with the crystalline cellulose and hemicellulose, leads to the formation of lignin carbohydrates complex (LCC) (Harrison et al. Citation2013). The LCC reduces the bio-availability of cellulose and hemicellulose, thereby necessitating the application of pre-treatment. Generally, pre-treatment is a process that partially/completely disrupts the LCC and enhances the efficiency of biomass hydrolysis (Harrison et al. Citation2013). An ideal pre-treatment process is able to break the interaction among the lignin and other biomolecules, thereby converting the crystalline cellulose to amorphous cellulose by increasing the porosity (Láinez et al. Citation2018). However, the utilization of harsh chemicals and extreme heating process in the pre-treatment approach generally leads to significant drawbacks in achieving an industrially feasible and eco-friendly process.
In this context, by exploiting the delignification properties of the oxidoreductase enzyme, laccase, and peroxidases processes was considered to be a sustainable alternative (Liew et al. Citation2011). However, utilization of the pre-treatment and saccharification processes as separate unit operations is considered to be a time-staking and enzyme intensive process (Dhiman et al. Citation2017; Kumar, Gujjala, and Banerjee Citation2017). As a result, a new process by the concoction of pre-treatment and saccharification into a single pot for the development of a monophasic simultaneous pre-treatment and saccharification (SPS) was introduced. Although several researchers reported the successful utilization of enzymatic SPS method, the problems regarding poor repeatability and efficiency along with higher production cost coupled with lack of commercial industrial equipment make the enzymatic SPS process less popular (Liu and Qu Citation2018).
To counter this problem, the synergistic and concerted action of multienzyme produced by filamentous fungi makes poses as an ideal alternative for the conventional enzymatic SPS. Trichoderma atroviride is a saprophytic fungus, a mycoparasite, and a plant symbiont. In this genus, the synergism among the different kind of cellulases and hemicellulases leads to efficient lignocellulose degradation which has been previously described (Conesa, Seguí, and Fito Citation2018). In the genome of many Trichoderma species, there are reports of genes that codify for phenol oxidases, enzymes with the potential to degrade lignin; however, little is known about their regulation or expression (Cázares-García, Vázquez-Garcidueñas, and Vázquez-Marrufo Citation2013).
Recently we constructed a transgenic Trichoderma atroviride that constitutively expresses a laccase gene from Tramates sanguineus which was capable of removing lignin derived xenobiotic compounds (Balcázar-López et al. Citation2016). The genetic manipulation included isolation of laccase gene from Trametes sanguineus and cloning it in the vector pUE10 followed by transfection into Trichoderma atroviride, at the downstream to blu17 terminator. Further, to enhanced gene expression, a strong promotor pki, isolated from Tremetes reesei, was utilized. Apart from its natural ability to produce cellulase and xylanase, the resultant genetically engineered Trichoderma sp. expressed enhanced laccase production, thereby making them a potential candidate for depolymerization of lignocellulosic biomass. On this front, the current work utilized the widely available lignocellulosic waste biomass in India., i.e.,, Casuarina equisetifolia or Australian pine tree for the first time for the SPS. Thus, the novelty of current study was the optimization of process parameters involved in SPS of C. equisetifolia biomass using transgenic Trichoderma atroviride for the maximum production of fermentable sugars by enhancing the secretion of lignocellulolytic enzyme cocktail. As a subsequent objective, a comparative study with conventional biomass treatment process (acidic and alkaline) was performed to elucidate the applicability of produced reducing sugars.
Materials and methods
Chemicals and microorganisms
All the substrates (≥98% purity) utilized in the experiment were purchased from Sigma-Aldrich (St. Louis, USA). Xylan from beechwood was obtained from Megazyme (Bray, Ireland). All the other solvents and chemicals utilized in the study were of analytical grade and were obtained from Southern India Scientific Corporation Private Limited (Chennai, India). The Casuarina equisetifolia biomass (CB) was collected from nearby agriculture land of SRMIST, Chennai. A transgenic Trichoderma atroviride IMI206040 that constitutively expresses a laccase gene from Tramates sanguineus was maintained in minimal GYM (glucose, yeast extract, malt extract) media at 28°C (Balcázar-López et al. Citation2016).
Quantification of enzymes
The key lignolytic enzyme, laccase, was estimated by quantifying the rate of the oxidation kinetics of 0.45 mM of ABTS spectrophotometrically at 420 nm (Balcázar-López et al. Citation2016; Vishnu et al. Citation2017). On the other hand, the cellulase activity was quantified using the filter paper method according to the International Union of Pure and Applied Chemistry (Ghose Citation1987). The assay was performed by measuring the kinetics of the sugar released from 50 mg of Whatman filter paper over a time of 60 min. Similarly, xylanase activity was quantified using xylan from beechwood as a substrate and measuring the released sugars spectrophotometrically at 540 nm by DNS method (Coward‐Kelly et al. Citation2003). One enzyme unit was defined as the amount of cellulase/Xylanase required to release 1 µM of sugar from their respective substrates in 1 min. The enzyme units were represented in terms of amount of enzyme released, when 1 kg of biomass was used (U/kg).
On the other hand, glucose and xylose were quantified spectrophotometrically as per the standard protocols that are reported previously (Deschatelets and Ernest Citation1986; Sahmetlioglu et al. Citation2006). In brief, glucose was estimated by quantifying the amount of oxidized o-dianisidine formed on reacting with 5 mg of glucose oxidase and peroxidase each, at 540 nm (Sahmetlioglu et al. Citation2006). Similarly, xylose was quantified by measuring the color complex formation on reacting with 2% p-bromoaniline at 520 nm (Deschatelets and Ernest Citation1986). The fermentable sugars were represented as sum of the concentrations of glucose and xylose. The proteins were estimated by Lowry’s method (Peterson Citation1977)
Simultaneous Pre-treatment and Saccharification (SPS)
For the SPS process, 10 gm of the CB, with a particle size of less than 0.2 mm, was taken in a conical flask containing 20 mL of distilled water, to which 10% Trichoderma biomass (106 spore/mL) were introduced and incubated at 28°C. For analysis, the flasks were harvested by adding 10 mL of 0.1 M phosphate buffer pH 7.0 and subjecting to mild sonication at 60 kHz for 30 s, followed by centrifuging at 4500 rpm for 10 min. By using three phase partition technique, the enzymes present in the supernatant utilized for enzyme and sugar analysis.
Depending on the enzyme activities obtained, physico-biological parameters such as pH 4.0–6.0, temperature 25-40°C, incubation time 0–168 h, inoculum size 3–15% and inoculum age 24–96 hr were studied. Based on the optimized results, the amount of the fermentable sugars released from CB was quantified. In addition, the results T. atroviride treated CB were compared with other conventional chemical-based saccharification methods, which includes treatment with 10%, (v/v) sulfuric acid at 135°C (Lenihan et al. Citation2010) and 2% (w/v) sodium hydroxide at 110°C (Lenihan et al. Citation2010).
Toxicity profiling
The formation of fermentative inhibitors during SPS was studied by spectrophotometrically quantifying the growth of Escherichia coli at 600 nm in the presence of various concentrations of the supernatant obtained after SPS treatment. The results were compared with the supernatant obtained from acid and alkali-based saccharification method (Novotný et al. Citation2006).
Results and discussion
Optimization of SPS process parameters
The incubation time is considered as a crucial parameter, which provides information on the growth of fungi, enzyme production, and substrate utilization. In the current study, a maximum of 183.2 U/kg of laccase along with 1245.6 U/kg of cellulase and 1203.36 U/kg of xylanase was achieved in 96 h, which was followed by a significant decline upon further incubation (). The decline in the activity of the enzyme could be due to several factors, that include a decline in the metabolic activity of T. atroviride under stress conditions as a result of essential nutrient depletion (Saini, Aggarwal, and Yadav Citation2017) or other physiological changes resulting in the reduction of the enzyme stability.
Figure 1. Effect of incubation time on the production of enzyme cocktail and fermentable sugars. All the experiments were performed in triplicates and the results were represented as mean average with standard deviation
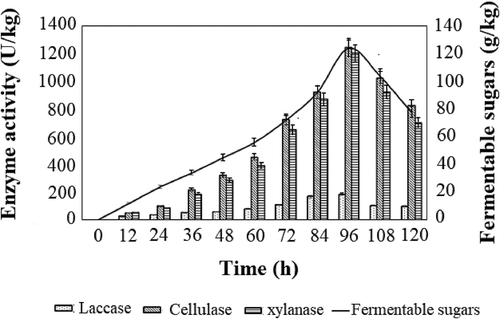
On the other hand, production of cellulase, xylanase, and laccase was reported to be dependent on the temperature and pH for the establishment of a successful biorefinery process. Upon optimization the temperature and pH, a maximum laccase activity of 173.36 U/kg, 1145.9 U/kg of cellulase, and 1025.36 U/kg of xylanase along with 221.12 g/kg of fermentable sugars were obtained at a temperature of 30°C and pH 5.0 (). However, with the increase in the temperature, a gradual reduction in the protein content, with a maximum of 5.2-fold reduction in the protein content with respective to room temperature was obtained, which supported the reduced tolerance of T. atroviride to elevated temperature due to its affects the folding, mobility and compactness of the enzyme structure. Regardless of the incubation temperature, the maximal enzyme activity was obtained at a less acidic pH 4.0– 5.0, and the variation is drastic when compared to the activity at pH 6.0. The increase in the cellulase and xylanase activity by 1.5-fold emphasized the active growth of the T. atroviride at acidic pH. Colussi and coworkers, reported that the modulation on the pH has a direct effect on ionization of key amino acid residues of the hydrophobic enzymes, leading to a reduction in the enzyme activities (Colussi et al. Citation2012).
Table 1. Effect of temperature and pH on enzyme cocktail production of transgenic Trichoderma atroviride.
With respective to inoculum, a maximum enzyme cocktail production was observed when 10% inoculum was utilized (). Saini, Aggarwal, and Yadav (Citation2017) reported that the increase in the levels of the inoculum percentage hinders the balance between nutrient accessibility and biomass due to the clumping of the biomass, which directly or indirectly affects the enzyme production and secretion (Saini, Aggarwal, and Yadav Citation2017). On the other hand, a low level of inoculum delays the time for attaining optimal biomass for the production of enzymes. Similarly, the effect of the inoculum age was found to dictate the synthesis of the enzyme cocktail. In the current study, 48 hr old inoculum age exhibited an average of 1.4-fold increase in the secretion of enzyme cocktail in comparison with 24 hr old culture ().
Figure 2. Effect of (a) inoculum age and (b) inoculum size on the production of enzyme cocktail and fermentable sugars. All the experiments were performed in triplicates and the results were represented as mean average with standard deviation
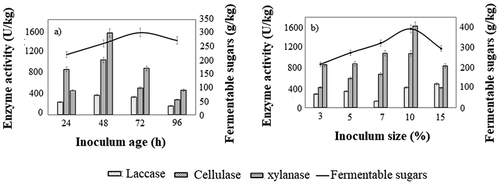
The treatment of the CB under optimized condition yielded a maximum release of 321.54 g/kg of fermentable sugars, with a 30% enhancement in the delignification process in comparison with the initial media conditions (). The SEM results of CB after treatment clearly showed the effectiveness of T. atroviride in the delignification process (Figure S1). In the SEM images, the presence of clear and smooth surface on the untreated biomass represents the presence of lignin. On the other hand, the biomass subjected to SPS showed a rough and highly damaged surface, inferring the degradation of lignin molecules that masks the polysaccharides. Further, the fourier transform infrared (FTIR) analysis clearly showed the changes in the functional groups present in cellulose, hemicellulose, and lignin of biomass sample before and after SPS (Figure S2). The peak at 3287.32 cm-1 represents the OH stretching due to guaiacyl, hydroxyphenyl, and syringyl groups of lignin, which was more prominent in control biomass than SPS treated biomass. The readily visible peak 1034.28 attributes for the presence C-O stretching vibrations of the hemicellulose and cellulose.
Figure 3. (a) Saccharification kinetics and (b) biomass load of Casuarina biomass for simultaneous pre-treatment and saccharification (SPS) process. All the experiments were performed in triplicates and the results were represented as mean average with standard deviation
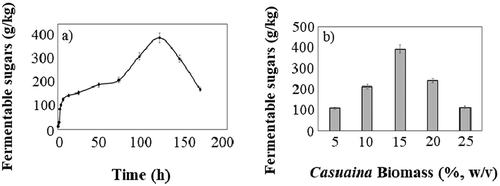
During the delignification process, a gradual reduction in the inhibitory effect of lignin on the cellulolytic enzymes directly increased the accessibility of cellulose to the enzyme cellulase, which could have led to the gradual increase in the yields of the total sugars with respective time (Ghorbani et al. Citation2015). However, after 96 h, a drastic reduction in the yield was observed, which was due to the consumption of the produced fermentable sugars by T. atroviride after exhaustion of external nutrient supplement. Furthermore, for maximizing the yield of fermentable sugars, the biomass to liquid culture was optimized. With increase in the biomass percentage from 5% to 15% (w/v), the fermentable sugars yield increased and reached the maximum at 15% biomass, yielding 392.36 g/kg of fermentable sugars. However, with the further increase in the biomass, an average of threefold reduction in the yield of fermentable sugars was noted with 25% biomass (). A similar result was reported by optimizing the saccharification parameters for bamboo (Kumar, Gujjala, and Banerjee Citation2017). Based on the current results, it was clear that in SPS, laccase along with cellulase and xylanase work together to achieve complete hydrolysis of biomass. The initial attack by the laccase increases the cell wall permeability by depolymerizing the lignin molecule thereby enabling the other enzymes to cause hydrolysis of polysaccharides for the production of reducing sugars (Potumarthi et al. Citation2013).
Toxicity studies
The formation of fermentative inhibitors, having antimicrobial activity, along with fermentable sugars was considered as a general demerit in the conventional saccharification process (Bellido et al. Citation2011). The presence of fermenter inhibitors not only inhibits the microbial growth but also limits further usage of produced sugar. In this context, the SPS process did not exhibit notable toxicity toward E. coli. However, in acid and alkaline pre-treatment, a drastic inhibition of E. coli by 1.4 and 1.3-fold with respective to the control (O.D. 1.85) supports the presence of fermentative inhibitors (). Fermentation inhibitors, such as furfural, 5-hydroxymethy furfural, and levulinic acid, were reported to inhibit the growth, which demand to perform industrially less feasible detoxification process (Kim et al. Citation2013). On the other hand, the ability of Trichoderma sp. to evade the formation of fermentative inhibitors, the degradation capabilities of the produced enzyme cocktail limited the toxicity level, which was a major advantage of the current study. On the other hand, the Trichoderma rich residual biomass can be valorized by utilizing as a bio controlling agent for soil application, for which further studies have to be performed.
Figure 4. Comparative toxicity analysis of SPS with respective conventional acid treatment and alkaline treatment methods on Casuarina biomass. All the experiments were performed in triplicates and the results were represented as mean average with standard deviation
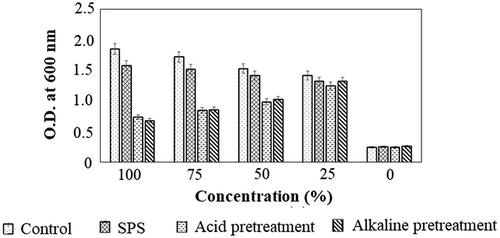
Combining two crucial stage of the biorefinery industries, i.e.,, pretreatment and saccharification in a single step not only reduces the labor but also the energy consumption. On comparing with the conventional pretreatment process, SPS technology is environmentally friendly for its less generation of effluent of water and potentially reduce water requirement. Moreover, the presence of laccase in SPS act as additional advantage in simultaneous detoxification of phenolic and fermentative inhibitor compounds formed during saccharification process and makes the overall process an industrially feasible green process.
Conclusion
From this study, it was evident that SPS is a feasible and effective technique for the enhanced production of fermentable sugars, where 392.36 g/kg was obtained. The ability of transgenic T. atroviride to produce a wide array of enzymes, viz., laccase, cellulase, and xylanase constituted it as the best model fungus for the SPS process. The degree of delignification was found to be highly dependent on the operating parameters and upon optimization, a drastic increase in the production of fermentable sugars was achieved. Further, the formation of fermenter inhibitors during the convention pre-treatment process was significantly reduced in the current study and did not exhibit any toxicity toward the microorganism. Consequently, the current technology is less tedious, eco-friendly, and a single-pot methodology which incurs industrial applicability with further exploration.
Supplemental Material
Download MS Word (658.1 KB)Acknowledgment
Vaidyanathan Vinoth Kumar and Jorge Luis Folch-Mallol would like to thank Indo-Mexican bilateral cooperation project (No. DST/INT/MEXICO/P-13/2016) funded by the Department of Science and Technology, Government of India along with the National Council for Science and Technology, Mexico.
Disclosure statement
No potential conflict of interest was reported by the authors.
Supplemental Material
Supplemental data for this article can be accessed on the publisher’s website
Additional information
Funding
Notes on contributors
Abiram Karanam Rathankumar
Abiram Karanam Rathankumar is a Ph.D. student at the Department of Biotechnology in SRM Institute of Science and Technology, Chennai, India.
Somdutt Ravindran
Somdutt Ravindran is B.Tech student at the Department of Biotechnology in SRM Institute of Science and Technology, Chennai, India.
Kongkona Saikia
Kongkona Saikia is a Ph.D. student at the Department of Biotechnology in SRM Institute of Science and Technology, Chennai, India.
Varshni Arvind
Varshni Arvind is B.Tech student at the Department of Biotechnology in SRM Institute of Science and Technology, Chennai, India.
Ramón Alberto Batista-Garcia
Ramón Alberto Batista-Garcia is a Research professor in Centro de Investigaciones en Dinámica Celular. Universidad Autónoma del Estado de Morelos, Cuernavaca, México.
Jorge Luis Folch-Mallol
Jorge Luis Folch-Mallol is a professor in Centro de Investigaciones en Dinámica Celular. Universidad Autónoma del Estado de Morelos, Cuernavaca, México.
Vaidyanathan Vinoth Kumar
Vaidyanathan Vinoth Kumar is an Associate professor at the Department of Biotechnology in SRM Institute of Science and Technology, Chennai, India.
References
- Balcázar-López, E., L. H. Méndez-Lorenzo, R. A. Batista-García, U. Esquivel-Naranjo, M. Ayala, V. V. Kumar, O. Savary, H. Cabana, A. Herrera-Estrella, and J. L. Folch-Mallol. 2016. Xenobiotic compounds degradation by heterologous expression of a Trametes sanguineus laccase in Trichoderma atroviride. PLoS ONE 11:e0147997. doi:10.1371/journal.pone.0147997.
- Bellido, C., S. Bolado, M. Coca, S. Lucas, G. González-Benito, and M. T. García-Cubero. 2011. Effect of inhibitors formed during wheat straw pretreatment on ethanol fermentation by Pichia stipitis. Bioresour. Technol. 102:10868–74. doi:10.1016/j.biortech.2011.08.128.
- Cázares-García, S. V., M. S. Vázquez-Garcidueñas, and G. Vázquez-Marrufo. 2013. Structural and phylogenetic analysis of laccases from Trichoderma: A bioinformatic approach. PLoS ONE 8:e55295. doi:10.1371/journal.pone.0055295.
- Colussi, F., W. Garcia, F. R. Rosseto, B. L. S. de Mello, M. de Oliveira Neto, and I. Polikarpov. 2012. Effect of pH and temperature on the global compactness, structure, and activity of cellobiohydrolase Cel7A from Trichoderma harzianum. Eur. Biophys. J. 41:89–98. doi:10.1007/s00249-011-0762-8.
- Conesa, C., L. Seguí, and P. Fito. 2018. Hydrolytic performance of Aspergillus niger and Trichoderma reesei cellulases on lignocellulosic industrial pineapple waste intended for bioethanol production. Waste Biomass Valori. 9:1359–68. doi:10.1007/s12649-017-9887-z.
- Coward‐Kelly, G., C. Aiello‐Mazzari, S. Kim, C. Granda, and M. Holtzapple. 2003. Suggested improvements to the standard filter paper assay used to measure cellulase activity. Biotechnol. Bioeng. 82:745–49. doi:10.1002/bit.10620.
- Deschatelets, L., and K. C. Ernest. 1986. A simple pentose assay for biomass conversion studies. Appl. Microbiol. Biotechnol. 24 (5):379–85. doi:10.1007/BF00294594.
- Dhiman, S. S., A. David, N. Shrestha, G. R. Johnson, K. M. Benjamin, V. Gadhamshetty, and R. K. Sani. 2017. Simultaneous hydrolysis and fermentation of unprocessed food waste into ethanol using thermophilic anaerobic bacteria. Bioresour. Technol. 244:733–40. doi:10.1016/j.biortech.2017.07.102.
- Ghorbani, F., M. Karimi, D. Biria, H. Kariminia, and A. Jeihanipour. 2015. Enhancement of fungal delignification of rice straw by Trichoderma viride sp. to improve its saccharification. Biochem. Eng. J. 101:77–84. doi:10.1016/j.bej.2015.05.005.
- Ghose, T. 1987. Measurement of cellulase activities. Pure Appl. Chem. 59:257–68. doi:10.1351/pac198759020257.
- Harrison, M. D., Z. Zhang, K. Shand, I. M. O’Hara, W. O. Doherty, and J. L. Dale. 2013. Effect of pretreatment on saccharification of sugarcane bagasse by complex and simple enzyme mixtures. Bioresour. Technol. 148:105–13. doi:10.1016/j.biortech.2013.08.099.
- Kim, S.-K., D.-H. Park, S. H. Song, Y.-J. Wee, and G.-T. Jeong. 2013. Effect of fermentation inhibitors in the presence and absence of activated charcoal on the growth of Saccharomyces cerevisiae. Bioproc. Biosyst. Eng. 36:659–66. doi:10.1007/s00449-013-0888-4.
- Kumar, S., L. K. Gujjala, and R. Banerjee. 2017. Simultaneous pretreatment and saccharification of bamboo for biobutanol production. Ind. Crops Prod. 101:21–28. doi:10.1016/j.indcrop.2017.02.028.
- Láinez, M., H. A. Ruiz, A. A. Castro-Luna, and S. Martínez-Hernández. 2018. Release of simple sugars from lignocellulosic biomass of Agave salmiana leaves subject to sequential pretreatment and enzymatic saccharification. Biomass Bioenerg. 118:133–40. doi:10.1016/j.biombioe.2018.08.012.
- Lenihan, P., A. Orozco, E. O’neill, M. Ahmad, D. Rooney, and G. Walker. 2010. Dilute acid hydrolysis of lignocellulosic biomass. Chem. Eng. J. 156:395–403. doi:10.1016/j.cej.2009.10.061.
- Liew, C., A. Husaini, H. Hussain, S. Muid, K. Liew, and H. Roslan. 2011. Lignin biodegradation and ligninolytic enzyme studies during biopulping of Acacia mangium wood chips by tropical white rot fungi. World J. Microbiol. Biotechnol. 27:1457–68. doi:10.1007/s11274-010-0598-x.
- Liu, G., and Y. Qu. 2018. Engineering of filamentous fungi for efficient conversion of lignocellulose: Tools, recent advances and prospects. Biotechnol. Adv. 37:519–29. doi:10.1016/j.biotechadv.2018.12.004.
- Novotný, Č., N. Dias, A. Kapanen, K. Malachová, M. Vándrovcová, M. Itävaara, and N. Lima. 2006. Comparative use of bacterial, algal and protozoan tests to study toxicity of azo-and anthraquinone dyes. Chemosphere 63:1436–42. doi:10.1016/j.chemosphere.2005.10.002.
- Peterson, G. L. 1977. A simplification of the protein assay method of Lowry et al. which is more generally applicable. Anal. Biochem. 83:346–56. doi:10.1016/0003-2697(77)90043-4.
- Potumarthi, R., R. R. Baadhe, P. Nayak, and A. Jetty. 2013. Simultaneous pretreatment and sacchariffication of rice husk by Phanerochete chrysosporium for improved production of reducing sugars. Bioresour. Tech. 128:113–17. doi:10.1016/j.biortech.2012.10.030.
- Sahmetlioglu, E., H. Yürük, L. Toppare, I. Cianga, and Y. Yagci. 2006. Immobilization of invertase and glucose oxidase in conducting copolymers of thiophene functionalized poly (vinyl alcohol) with pyrrole. React. Funct. Polym. 66 (3):365–71. doi:10.1016/j.reactfunctpolym.2005.08.009.
- Saini, A., N. K. Aggarwal, and A. Yadav. 2017. Cost-effective cellulase production using Parthenium hysterophorus biomass as an unconventional lignocellulosic substrate. 3 Biotech 7:12. doi:10.1007/s13205-017-0604-1.
- Vishnu, D., G. Neeraj, R. Swaroopini, R. Shobana, V. V. Kumar, and H. Cabana. 2017. Synergetic integration of laccase and versatile peroxidase with magnetic silica microspheres towards remediation of biorefinery wastewater. Environ. Sci. Pollut. Res. 24:17993–8009. doi:10.1007/s11356-017-9318-5.