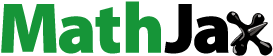
ABSTRACT
The present study deals with the development of a wood assisted fungal system (WAFS) from wood chips using Trametes hirsuta to remove polycyclic aromatic hydrocarbons (PAHs) in BRW. The WAFS exhibited a 1.4-fold higher ligninolytic enzyme production than free fungi in the effluent. Further, to understand PAHs bioremediation by T. hirsuta, biodegradation along with biosorption were studied in model PAHs, phenanthrene (Phe) and benzo (a) pyrene (BaP), in the presence of synthesized rhamnolipids. The WAFS mineralized up to an average of 91.26% Phe and 87.72 % BaP along with biosorption of 12.35% Phe and 18.36 % BaP within 12 days. Thus, the addition of rhamnolipids showed 1.2-fold enhanced biodegradation. However, rhamnolipid concentrations beyond 50 ppm reduced the degradation efficiency of WAFS. Moreover, the degradation capability of total aromatic hydrocarbon (TAH) in biorefinery wastewater by WAFS is 1.8-fold higher than that of free fungi, which confirms the effectiveness of the system.
Implications: Simultaneous application of white-rot fungus along with surfactant into a pollutant environment affects the microenvironment of the fungus and reduces the production of their degradative enzymes. In addition, the requirement of periodical supplement of external nutrient in the real-time matrix for the growth of white rot fungi may trigger competitive growth of indigenous microorganisms. Considering this glitch, the current work utilizes the carpenter waste for the strategical develop a wood assisted fungal system to protect the microenvironment of the fungi in the presence of rhamnolipids and contribute to their survival in real time matrix, with enhanced PAHs degradation efficiency.
Introduction
Polycyclic Aromatic Hydrocarbons (PAHs) are one of the potent environmental pollutants, which are hydrophobic and chemically reactive, making them highly recalcitrant in the environment (Batista-García et al. Citation2017). The major setback in any remediation process is the limitation in the bioavailability of the pollutants on the sub-surface portion of aqueous phase due to the high log Kow values of the PAHs (Rathankumar et al. Citation2020b). Amongst the various remediation techniques, including chemical, physical, and biological methods, utilization of fungi or their secreted ligninolytic enzymes in the presence of surfactant yielded a comparatively higher degradation efficiency. However, Sondhi and his group reported the inhibitory effect of synthetic ionic surfactant (below their critical micelle formation concentration (CMC) values) on laccase activity and showed that the interaction of the surfactant with the enzyme may cause alteration in the physical or enzymatic characteristics, which leads to reduction in the enzyme activity (Sondhi et al. Citation2014). With much extoll on the environmental friendliness and green processing, biosurfactants are recognized as an excellent biodegradable surface-active agent which is an excellent alternative to the conventional surfactants (Rathankumar et al. Citation2019), various reports claimed that the presence of surfactant have a positive effect on the production and activity of ligninolytic enzymes (Ron and Rosenberg Citation2001). However, in either of the cases, it is evident that fungi undergo stress in the presence of synthetic or biosurfactants, which affects the fungi-assisted degradation efficiency. Thus, this demands for the development of unique carrier system to reduce the stress levels in fungi even in the presence of surfactants.
On the other hand, with the increase in the wood consumption, more than 2.6 billion tons of wood waste is contributed from European Union countries, which reflects the enormity of waste generated (FAO Citation2016). White rot fungi have the ability to grow on wood chips, by colonizing not only the surface, but also the interspace of the wood chip (Lu et al. Citation2009). In addition, the presence of the trace amount of nutrients and lignin in the wood enhance the fungal growth and induce the production of ligninolytic enzymes, thereby establishing themselves as suitable self-sustained systems. From our previous studies, we identified that Tramates hirsuta (T. hirsuta) is a suitable mycoremediation tool for the removal of PAHs and phenolic contaminants in biorefinery wastewater (Table S1) (Batista-García et al. Citation2017). Thus, by exploiting the advantages attained from the growth of fungi on wood and to address the effective management of the generated wood waste, the aim of the current study was to present a strategical route for the development of a wood-assisted T. hirsuta system for its application as an active bioremediation tool in the presence of surfactants and the key process parameters were optimized. Subsequently, the effects of the biosurfactant, rhamnolipids, on the biodegradation and biosorption of the model PAHs, phenanthrene and benzo (a) pyrene, were also established. Comprehensively, the present study utilized the optimized process conditions to establish the developed process toward the real time application in the effective bioremediation of PAHs in biorefinery wastewater.
Materials and methods
Chemicals and microorganisms
The standard chemicals Phenanthrene, Benzo (a) pyrene, O-tetraphenol, 16-PAHs along with the substrates 3,4-Dimethoxybenzyl alcohol (veratryl Alcohol), 2,2′-azino-bis (3-ethylbenzothiazoline-6-sulfonic acid) (ABTS), Benzoquinone and 2,5-Dimethoxyphenol (DMP) were purchased from Sigma Aldrich Corporation (St. Louis, MO, United States). The wood chips from carpentry waste utilized in the experiment was procured from the local market of Chennai, India. The effluent used for spiking the PAHs was collected from waste water treatment plant in SRMIST, Kattankulathur, India. All the other chemicals and solvents used in the study were of analytical grade and were procured from Southern India Scientifics Cooperation Private Limited, Chennai, India. T. hirsuta (IBB 450) was procured from Institute of Biochemistry and Biotechnology (IBB), Georgia, United States, and the strain was maintained in GYM (glucose, yeast extract, and malt extract) media at pH 6.0 (Batista-García et al. Citation2017). The rhamnolipids utilized in the study was produced and purified according to our previous report (Rathankumar et al. Citation2019). The biorefinery wastewater utilized in the study was collected from the nearby industrial area of Sherbrooke, QC, Canada.
Wood assisted fungal biosystem
For the development of the wood assisted fungal system (WAFS), wood chips of 0.1–0.2 cm thickness were subjected to pre-treatment by sequentially soaking the wood chips in boiling water (Lu et al. Citation2009). The resultant wood chips were inoculated with T. hirsuta at 28°C till the formation of white mat like structure on the wood chips. The WAFS was tested for its efficiency in the production of ligninolytic enzyme cocktail by incubating the WAFS in 250 mL conical flask containing 100 mL of autoclaved effluent. At regular interval of time, the enzyme activities of the WAFS were measured and were represented with respect to the control (free fungi). The activities of laccase (lac), lignin peroxidase (LiP), manganese peroxidase (MnP), and aryl alcohol oxidase (AAO) were measured spectrophotometrically according to our pervious reporting of Rathankumar et al. (Citation2020), Kumar et al. (Citation2014), Vishnu et al. (Citation2017). The lac activity was measured by estimating the kinetics of rate of oxidation of 1 mM of ABTS at 420 nm. The rate of oxidation of 2 mM of 3,4- dimethoxybenzyl alcohol by AAO was quantified at 310 nm to measure the AAO activity. Similarly, the LiP activity was assayed in the presence of 0.4 mM hydrogen peroxide along with 2 mM of 3,4- dimethoxybenzyl alcohol. The MnP activity was estimated by quantifying the oxidation of 0.1 mM 2,6 dimethoxyphenol in the presence of 0.1 mM hydrogen peroxidase and 0.5 mM manganese sulfate at 310 nm. The unit activity of the enzymes was defined as the amount of enzyme required to bio-transform 1 μmol of their respective substrates in unit time. The enzyme activities were calculated using Beer Lambert’s law using their respective molar absorption coefficient (εLip = 9300 M−1.cm−1, εLac = 3.6 × 104 M−1.cm−1, εMnP = 27,500 M−1.cm−1, and εAAO = 9800 M−1.cm−1).
Degradation and biosorption studies of PAHs by WAFS
For the degradation studies, 50 mL of 50 ppm of each PAHs phenantharene (Phe) and benzo(a)pyrene (BaP) wre dissolved in 5 mL of acetone and made up to 50 mL with the effluent. Each spiked sample was subjected to mild sonication for complete solubilization of the PAHs (Rathankumar et al. Citation2020). The biodegradation efficiency was monitored by introducing 4 g (~106 spore/g) of WAFS in the respective flasks (Lu et al. Citation2009). To study the effect of biosurfactant on degradation of PAHs, varying concentrations of rhamnolipids (50–125 ppm) were introduced into the effluent. The results were compared with three controls consisting of 4 g (~106 spore/g) of free fungi in the presence of rhamnolipids and without rhamnolipids. The concentration of the PAHs in various systems was quantified at regular intervals of 24 h by sacrificing the respective flask every day in order to avoid any error while sampling (Batista-García et al. Citation2017). To understand whether the process is biosorption or biodegradation, heat killed fungal biomass and WAFS were introduced into the media containing PAHs and rhamnolipid (Ma et al. Citation2018). After 72 h incubation under static condition at 30°C, the residual PAHs were quantified.
For PAHs quantification, samples were extracted by adding equal volumes of dichloromethane and subjecting to cleaning using solid phase extraction techniques (C18 cartridge). The extraction efficiency of Phe and BaP were established by using O-teraphenol as a surrogate. The resultant samples were analyzed using gas chromatography (Shimadzu 2025, Japan) fitted fire ionization detector (FID) with HP-5 (30 m × 0.25 mm × 0.25 µm) quartz slender segment column, according to Mandal et al. (Citation2015).
Degradation studies of TAHs present in biorefinery wastewater
Utilizing the optimized concentration of rhamnolipids, degradation studies were performed in biorefinery wastewater using the WAFS and free fungi. Prior to the experiment, the profile of total aromatic hydrocarbons was established using GC-FID (Mandal et al. Citation2015). The degradation efficiency of WAFS was performed by quantifying the relative reduction of the PAHs in the GC-FID chromatogram (Mehrizi et al. Citation2019).
Statistical analysis
All the experiments were preformed in triplicates, and the results were represented as mean average along with standard deviation. The obtained data was statistically analyzed for its significance by one-way analysis of variance (ANOVA) using GraphPad Prism (8.0.1), and the significance of the data was compared by post hoc analysis (Bonferroni test).
Results and discussion
T. hirsuta grown on wood chips was assessed for its ability to produce the key ligninolytic enzymes. The production of the ligninolytic enzymes was confirmed by the ability of 1 mL of the cultured effluent to oxidize the respective substrates. To ensure the accuracy in quantification, all the enzyme activities were measured by recording the rate of the oxidation of the respective substrates over 10 min and the slope obtained by plotting the graph between O.D. and time was utilized for the calculation of the enzyme activities using Beer Lambert’s law (Batista-García et al. Citation2017; Rathankumar et al. Citation2020). The WAFS exhibited a maximum MnP activity of 124.362.53 U/L and was found to be 1.5-fold higher than that of the free fungi in the effluent (), which suggested the presence of sufficient Mn2+ in the WAFS to initiate MnP production (Liew et al. Citation2011). As the wood chips are a rich source of lignin, an enhanced production of laccase by 1.4-fold was noted in the WAFS, which supported the role of lignin in improving the laccase production. Similar to laccase and MnP, LiP and AAO also exhibited high volumetric enzymatic activities. On contrary to the previous reporting on low production of AAO using T. hirsuta (Cilerdzic et al. Citation2011), 170.42 U/L of AAO was obtained in WAFS in the current study (). This supported the role of wood chips as an inducer in the production of ligninolytic enzymes and provided a solid support by maintaining the microenvironment required for the growth of the white rot fungi (Lu et al. Citation2009). The absence of any external nutrient simulation along with limited carbohydrate source was considered to be the major reason for the low production of the ligninolytic enzyme cocktail in comparison with the conventional nutrient broth media of T. hirsuta as reported by Batista-García et al. (Citation2017). Depending on the ligninolytic enzymes activities, seven days of incubation was considered as an optimum incubation time for the maximum production of enzyme cocktail.
Figure 1. Kinetics of (a) laccase (lac) and manganese peroxidase (MnP) (b) Aryl alcohol oxidase (AAO) and Lignin peroxidase (LiP) in free fungi (FF) and Wood assisted fungal system (WAFS) and the effect of various concentrations of rhamnolipids (Rha) on the laccase (lac), manganese peroxidase (MnP), Aryl alcohol oxidase (AAO) and Lignin peroxidase (LiP) production in (c) free fungi (FF) and (d) Wood assisted fungal system (WAFS) at day 7. (*P ≤ 0.05, **P ≤ 0.01 and ***P ≤ 0.001) where P ≤ 0.05 is considered as significant.)
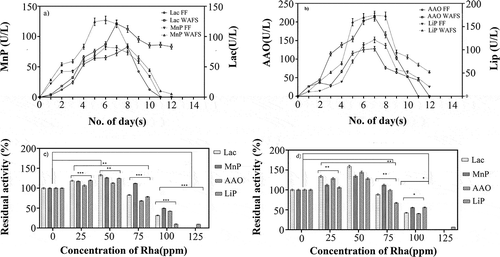
Effect of rhamnolipids on enzyme cocktail production
The addition of rhamnolipids stimulated the enhanced production of laccase, AAO, Lip, and MnP, and the laccase activity was 1.2-fold higher than other enzymes (). The ability of the surfactants to release the enzymes trapped to the mycelium of fungi and to mitigate the inhibitory effect of PAHs on fungal growth were predicted to be the prime reasons for the increase in the activities of these enzymes (Zhou et al. Citation2011). Yamanaka et al. (Citation2008) reported that a minimal concentration of rhamnolipids can prolong the growth phase of fungi leading to enhanced production of these crucial enzymes and also proved to enhance the cell membrane permeability of the fungi, leading to improved secretion of the internal enzymes (Yamanaka et al. Citation2008). However, with the increase in the concentration of rhamnolipids beyond 75 ppm, a gradual reduction in the activity of the enzymes, with no enzyme activity at a concentration of 125 ppm, was noted (), which supported the inhibitory and antimicrobial activity of rhamnolipids with MIC range of 75 to 2,500 ppm (Magalhães and Nitschke Citation2013) The current results can be corelated to pervious reporting of Sha et al. (Citation2012), which claimed the efficiency of 100 ppm rhamnolipids to inhibit 54% of fungal growth.
The enzymes produced in the WAFS may be adsorbed by the wood chip, resulting in the release of only a limited amount of enzymes, which is similar to the mycelial trap in free fungi (Wang et al. Citation2008). However, an increase in the activity of the enzymes cocktail at the concentration of 50 ppm of rhamnolipids was noted (). On the other hand, increase in the bioavailability of lignin in the wood acts as an inducer for ligninolytic enzymes production triggering an enhanced expression of laccase and MnP (Zeng et al. Citation2006). In the present study, it was observed that the production of laccase was approximately 30% higher than LiP, AAO, and MnP. Liew et al. (Citation2011) reported a more reduced production of LiP than MnP and laccase in the presence of wood chip due to their minimal involvement in delignification process, which was presumed to be a major cause for the reduction in LiP activity (Liew et al. Citation2011). Contrarily to the free fungi, at 100 ppm of rhamnolipids, a considerable amount of ligninolytic enzymes was obtained in the WAFS, which supported the defense of fungi to external stress when immobilized on the wood chips. The current study supported the enhanced production of the ligninolytic enzymes in the presence of a considerable concentration of rhamnolipids in the WAFS, compared to previous reporting. In addition, the ability of rhamnolipids as a wetting agent claimed to maintain the microenvironment of the fungi in WAFS, making them a suitable system for industrial use (Zeng et al. Citation2006).
Degradation and biosorption studies of Phenanthrene and Benzo (a) pyrene by WAFS
Based on the degradation profile of Phe and BaP by T. hirsuta, 50 ppm was selected to study the degradation efficiency. Upon spiking the effluent with the respective PAHs, the final concentration of PAHs was found to be Phe- 49.2 ± 0.5 ppm and BaP- 48.5 ± 0.3 ppm in the effluent. Although there are several enzymes that play a vital role in the degradation of hydrophobic organic compounds, the current study emphasized on the role of the key degradative extracellular enzymes, i.e., ligninolytic enzymes based on our previous reporting Rathankumar et al. (Citation2020). In all the strategies, a complete bioremediation of the PAHs was achieved and were considered due to the combinational effect of adsorption and degradation. With respect to time, the rate of degradation of Phe and BaP gradually increased and attained an apparent saturation in the degradation at day 11 and day 9 in free fungi and WAFS, respectively (). The degradation rate of the PAHs was found to be significantly high in free fungi than in WAFS by an average of 1.07-fold, which was assumed to be due to the rapid adsorption of the PAHs by the wood particles (Chen, Yuan, and Liu Citation2011). In addition, the immobilization of the fungus on the wood chips might lead to the decrease in its biomass, thereby resulting in the reduction of ligninolytic enzymes production, which was considered to be one of the reasons for the decrease in the efficiency of WAFS. Moreover, the structural complexity of BaP along with its hydrophobic nature due to the presence of multiple benzene rings makes BaP more persistent to degradation in comparison to Phe, which was clearly reflected in the reduced degradation efficiency of BaP (81.64%) by 1.0-fold with respect to Phe in WAFS. The biodegradation of PAHs by T. hirsuta suggested its ability to utilize the PAHs as its sole carbon source, which agreed with pervious reporting. On relating to the ligninolytic enzymes secretion, a maximum degradation rate of PAHs was achieved when the production of MnP and Lac were high, which suggests the dominant role of MnP followed by Lac in mineralizing the PAHs. On the other hand, LiP along with MnP were reported to aid in ring hydrolysis of hydrophobic organic compounds (Bogan and Lamar Citation1996), which supported the role of these enzymes in the oxidation of PAHs. However, in the current study, by nullifying the action of the intracellular enzymes, adsorption studies were performed, and it was noted that the free fungi had higher adsorption of BaP (12.23%) than Phe (8.25%), which can be correlated to the fact that white rot fungi play a major role in the bioremediation of the hydrophobic organic compounds by biosorption and biodegradation.
Figure 2. Degradation and biosorption kinetics of 50 ppm spiked phenanthrene (Phe) and benzo (a) pyrene (BaP) in effluent by (a) free fungi and (b) Wood assisted fungal system (WAFS)
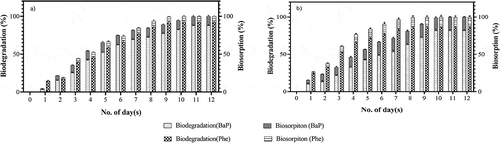
The biosorption of the PAHs were studied by heat killed fungal mycelia and heat killed WASF by assuming that the active role of intracellular enzymes in the degradation of the PAHs was very low. Further, the initial and final protein concentrations were quantified and the flasks with modulating concentration of protein levels were not considered for the biosorption studies with an assumption of the presence or growth of microorganisms which may affect the degradation of PAHs (Chen and Ding Citation2012). It was observed that a maximum of an average of 15% of PAHs was adsorbed in the heat-killed WASF, which was 1.5-fold lower than in heat killed free fungi. Moreover, the sorption effect was more predominant in the case of BaP in both the strategies than Phe due its high hydrophobicity levels (KOW value 6.13) (Rathankumar et al. Citation2020). The heat-killed mycelium contributed to biosorption of more than 12% of BaP and 8% of Phe (). The natural ability of the fungal exopolysaccharide sheath around the mycelium was reported to aid in biosorption of the PAHs (Chen and Ding Citation2012). The abundant presence of the conjugated structures, along with the number of functional groups such as -COO-,O-C = O,-CO-NH, -NH2, and -OH in the fungal mycelium could have acted as active sites for the sorption of PAHs either by electron donor--acceptor interaction or by planar π–π interactions (Gu et al. Citation2015). In case of the WAFS, the porous structure of the wood chip led to an enhanced sorption of 18.34% BaP and 14.36% Phe (). In both the strategies, maximum sorption was noticed on day 4, which was followed by an apparent saturation. Although the presence of lignin was found to enhance the production of the ligninolytic enzymes (Liew et al. Citation2011), rapid adsorption of the enzymes onto the porous surface of the WASF may reduce the degradation rate of the PAHs. A reduction in the overall degradation rate by approximately 1.0-fold in WAFS compared to the free fungi supported the above hypothesis. On the basis of the results obtained, it is was clear that live fungi act as bio-sorbent that sequester PAHs as well as a bioreactor to degrade the PAHs (Chen and Ding Citation2012).
Effect of rhamnolipids on the degradation of PAHs
The presence of surfactants was proven to enhance the degradation abilities of PAHs either by modulating the release of ligninolytic enzymes or by increasing the bioavailability of the PAHs (Das, Yang, and Ma Citation2014). From the previous experiments, the role of mycelium in sorption of Phe and BaP were evident, which limited the rate of degradation. In the presence of rhamnolipids, a gradual reduction in the sorption of Phe with increase in the concentration of rhamnolipids till 50 ppm was observed in both the WAFS and the control (). In spite of the higher log Kow value, a complete desorption of BaP was observed at 75 ppm in both the strategies using free fungi and WAFS, which reflected the effectiveness of rhamnolipids. The ability of the rhamnolipids to trap the hydrophobic organic compounds in their micelle is reported to decrease the adsorption of the PAHs. However, with the increase in the concentration of rhamnolipids beyond 50 ppm, a steady reduction in the degradation rate of the PAHs by approximately 1.3-fold was noted in the free fungi (), confirming the anti-microbial nature of the rhamnolipids (Rathankumar et al. Citation2019). The de-stabilization of the key enzymes or conformational changes in the active site of the enzymes due to the increased surfactant interaction was assumed to reduce the degradation rate at higher concentration of rhamnolipids (Holmberg Citation2018). In addition, a gradual decrease in the cell surface hydrophobicity (CSH) with increase in the rhamnolipids concentration, leading to the reduction in bioavailability of Phe, was considered as another major reason for the reduction in the degradation rate (Zhao, Selvam, and Wong Citation2011). However, in WAFS, the degradation rate of BaP was improved by 10% in comparison with the free fungi and was observed to exhibit a resistance to surfactant of beyond 75 ppm by showing an average of 2-fold increase in the degradation rate (). Even though it was proven that rhamnolipids have limited role in the growth enhancement of white rot fungi, the formation of the protective barrier around the fungi and alleviating the toxicity of the PAHs might aid in improving the initial growth of fungi (Zhou et al. Citation2011) In addition, several works have claimed the possibility of rhamnolipids in enhancing the release of enzymes by increasing the cell membrane permeability, which leads to increase in the yield of the ligninolytic enzymes and indirectly help in enhancing the degradation rate of PAHs (Whang et al. Citation2008).
Figure 3. Degradation and biosorption of 50 ppm spiked phenanthrene (Phe) and benzo (a) pyrene (BaP) in effluent in varying concentrations of rhamnolipids (Rha) during day 7 by (a) free fungi and (b) Wood assisted fungal system (WAFS). (*P ≤ 0.05, **P ≤ 0.01 and ***P ≤ 0.001) where P ≤ 0.05 is considered as significant.)
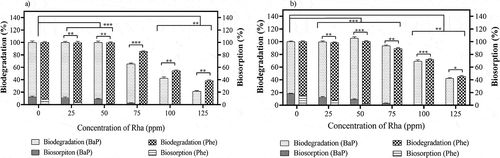
Removal of crude oil using free and WAFS system
The efficiency of the WAFS in the remediation of real time biorefinery wastewaters was studied with the optimized conditions of rhamnolipids assisted degradation. The characteristic of the wastewaters is presented in Table S2. The WAFS exhibited enhanced remediation of the biorefinery wastewater by completely remediating the total aromatic hydrocarbons (TAH) in 10 days, in comparison with the free fungi (). Associating the enhanced lac and MnP production in the WAFS over free fungi justified the enhanced degradation of TAH by 1.5-fold in the WAFS than in the free fungi (Figure S1). The presence of rhamnolipids suggested to enhance the bioavailability of TAH, which was reported to be controlled by the cell hydrophobicity and aqueous dispersion of TAH (Whang et al. Citation2008). Thus, the WAFS was observed to exhibit efficient removal of the TAH from the biorefinery wastewater, which was further enhanced by the presence of optimum concentrations of rhamnolipids.
Conclusion
An integrated WAFS was developed using T. hirsuta incorporated with rhamnolipids for the simple and efficient removal of PAHs. This study deals with biodegradation and myco-remediation of the model PAHs, phenanthrene and benzo (a) pyrene, which provides an effective solution to reduce the momentous issue of pollution caused by recalcitrant chemicals. The role of WAFS was to inculcate reusability, facilitating an additional edge to the entire process. Rhamnolipid, a bio-surfactant, aided in the bio-availability of the PAHs, thus enhancing the removal and in turn enhanced the production of key ligninolytic enzymes. The ability of the WAFS in bioremediation of crude oil proved their effectiveness for large-scale application. This study could eventually contribute toward methods to address PAHs bioremediation from the water bodies which is an emerging concern worldwide.
Supplemental Material
Download MS Word (925.8 KB)Acknowledgment
This work was supported by Department of Science and Technology ---Science and Engineering Research Board (SERB), India [SSY/2019/000462]. The authors would also like to extend their gratitude to Sri Ramaswamy Memorial Institute of Science and Technology, Tamil Nadu, India, for their help and for aiding in facilitating the research.
Supplementary data
Supplemental data for this article can be accessed on the publisher’s website.
Disclosure statement
No potential conflict of interest was reported by the authors.
Additional information
Notes on contributors
Abiram Karanam Rathankumar
Abiram Karanam Rathankumar is a Ph.D. student at the Department of Biotechnology, School of Bioengineering in SRM Institute of Science and Technology, Chennai, India.
Kongkona Saikia
Kongkona Saikia is a Ph.D. student at the Department of Biotechnology, School of Bioengineering in SRM Institute of Science and Technology, Chennai, India.
Senthil Kumar Ponnusamy
Senthil Kumar Ponnusamy is an Associate Professor at Department of Chemical Engineering, SSN College of Engineering, Chennai, India.
María del Rayo Sánchez-Carbente
María del Rayo Sánchez-Carbente is a Professor at Centre of Biotechnological Research (CEIB), Universidad Autónoma del Estado de Morelos, Cuernavaca, México.
Vinoth Kumar Vaidyanathan
Vaidyanathan Vinoth Kumar is an Associate professor at Department of Biotechnology, School of Bioengineering in SRM Institute of Science and Technology, Chennai, India.
References
- Batista-García, R. A., V. V. Kumar, A. Ariste, O. E. Tovar-Herrera, O. Savary, H. Peidro-Guzmán, D. González-Abradelo, S. A. Jackson, A. D. Dobson, and M. Del Rayo Sánchez-Carbente. 2017. Simple screening protocol for identification of potential mycoremediation tools for the elimination of polycyclic aromatic hydrocarbons and phenols from hyperalkalophile industrial effluents. J. Environ. Manage. 198:1–11. doi:10.1016/j.jenvman.2017.05.010.
- Bogan, B. W., and R. T. Lamar. 1996. Polycyclic aromatic hydrocarbon-degrading capabilities of Phanerochaete laevis HHB-1625 and its extracellular ligninolytic enzymes. Appl. Environ. Microbiol. 62 (5):1597–603. doi:10.1128/AEM.62.5.1597-1603.1996.
- Chen, B., and J. Ding. 2012. Biosorption and biodegradation of phenanthrene and pyrene in sterilized and unsterilized soil slurry systems stimulated by Phanerochaete chrysosporium. J. Hazard. Mater. 229:159–69. doi:10.1016/j.jhazmat.2012.05.090.
- Chen, B., M. Yuan, and H. Liu. 2011. Removal of polycyclic aromatic hydrocarbons from aqueous solution using plant residue materials as a biosorbent. J. Hazard. Mater. 188 (1–3):436–42. doi:10.1016/j.jhazmat.2011.01.114.
- Cilerdzic, J., M. Stajic, J. Vukojevic, S. Duletic-Lausevic, and A. Knezevic. 2011. Potential of Trametes hirsuta to produce ligninolytic enzymes during degradation of agricultural residues. Bioresour. Technol. 6:2885–95.
- Das, P., X.-P. Yang, and L. Z. Ma. 2014. Analysis of biosurfactants from industrially viable Pseudomonas strain isolated from crude oil suggests how rhamnolipids congeners affect emulsification property and antimicrobial activity. Front. Microbiol. 5:696. doi:10.3389/fmicb.2014.00696.
- FAO. 2016. 2015 global forest products facts and figures. Rome: FAO–Food and Agriculture Organization of the United Nations.
- Gu, H., X. Luo, H. Wang, L. Wu, J. Wu, and J. Xu. 2015. The characteristics of phenanthrene biosorption by chemically modified biomass of Phanerochaete chrysosporium. Environ. Sci. Pollut. Res. 22:11850–61. doi:10.1007/s11356-015-4451-5.
- Holmberg, K. 2018. Interactions between surfactants and hydrolytic enzymes. Colloids Surf B Biointerfaces 168:169–77. doi:10.1016/j.colsurfb.2017.12.002.
- Kumar, V. V., S. Sivanesan, and H. Cabana. 2014. Magnetic cross-linked laccase aggregates—Bioremediation tool for decolorization of distinct classes of recalcitrant dyes. Sci. Total Environ. 487:830–39. doi:10.1016/j.scitotenv.2014.04.009.
- Liew, C., A. Husaini, H. Hussain, S. Muid, K. Liew, and H. Roslan. 2011. Lignin biodegradation and ligninolytic enzyme studies during biopulping of Acacia mangium wood chips by tropical white rot fungi. World J. Microbiol. Biotechnol. 27:1457–68. doi:10.1007/s11274-010-0598-x.
- Lu, Y., L. Yan, Y. Wang, S. Zhou, J. Fu, and J. Zhang. 2009. Biodegradation of phenolic compounds from coking wastewater by immobilized white rot fungus Phanerochaete chrysosporium. J. Hazar. Mater. 165:1091–97. doi:10.1016/j.jhazmat.2008.10.091.
- Ma, Z., J. Liu, R. P. Dick, H. Li, D. Shen, Y. Gao, M. G. Waigi, and W. Ling. 2018. Rhamnolipid influences biosorption and biodegradation of phenanthrene by phenanthrene-degrading strain Pseudomonas sp. Ph6. Environ. Pollut. 240:359–67. doi:10.1016/j.envpol.2018.04.125.
- Magalhães, L., and M. Nitschke. 2013. Antimicrobial activity of rhamnolipids against Listeria monocytogenes and their synergistic interaction with nisin. Food Control 29:138–42. doi:10.1016/j.foodcont.2012.06.009.
- Mandal, S., N. Khuda, M. Mian, M. Moniruzzaman, N. Nahar, M. Mamun, and M. Shoeb. 2015. Analysis of ground and surface water samples from some area of Dhaka city for polycyclic aromatic hydrocarbons (PAHs). Dhaka Univ. J. Sci. 63:59–60. doi:10.3329/dujs.v63i1.21770.
- Mehrizi, E. A., M. Kermani, M. Farzadkia, A. Esarfili, and M. Ghorbanian. 2019. Study of improvement of bioremediation performance for the degradation of petroleum hydrocarbons in oily sludge by a chemical pretreatment strategy. J. Mater. Cycles Waste 21:1052–1062. doi: 10.1007/s10163-019-00848-y.
- Rathankumar, A. K., K. Saikia, K. T. Nagarajan, V. K. Vaidyanathan, H. Cabana, and V. K. Vaidyanathan. 2020b. Development of efficient and sustainable added value products from municipal biosolids through an industrial feasible process. J. Clean. Prod. (In press). doi: 10.1016/j.jclepro.2020.121749.
- Rathankumar, A. K., K. Saikia, K. Ramachandran, R. A. Batista, H. Cabana, and V. K. Vaidyanathan. 2020. Effect of soil organic matter (SOM) on the degradation of polycyclic aromatic hydrocarbons using Pleurotus dryinus IBB 903-A microcosm study. J. Environ. Manage. 260:110153. doi:10.1016/j.jenvman.2020.110153.
- Rathankumar, A. K., S. SaiLavanyaa, K. Saikia, A. Gururajan, S. Sivanesan, M. Gosselin, V. K. Vaidyanathan, and H. Cabana. 2019. Systemic concocting of cross‐linked enzyme aggregates of Candida antarctica Lipase B (Novozyme 435) for the biomanufacturing of rhamnolipids. J. Surfactants Deterg. 22:477–90. doi:10.1002/jsde.12266.
- Ron, E. Z., and E. Rosenberg. 2001. Natural roles of biosurfactants: Minireview. Environ. Microbiol. 3:229–36. doi:10.1046/j.1462-2920.2001.00190.x.
- Sha, R., L. Jiang, Q. Meng, G. Zhang, and Z. Song. 2012. Producing cell‐free culture broth of rhamnolipids as a cost‐effective fungicide against plant pathogens. J. Basic Microbiol. 52:458–66. doi:10.1002/jobm.201100295.
- Sondhi, S., P. Sharma, S. Saini, N. Puri, and N. Gupta. 2014. Purification and characterization of an extracellular, thermo-alkali-stable, metal tolerant laccase from Bacillus tequilensis SN4. PLoS One 9:e96951. doi:10.1371/journal.pone.0096951.
- Vishnu, D., G. Neeraj, R. Swaroopini, R. Shobana, V. V. Kumar, and H. Cabana. 2017. Synergetic integration of laccase and versatile peroxidase with magnetic silica microspheres towards remediation of biorefinery wastewater. Environ. Sci. Pollut. Res. 24:17993–8009. doi:10.1007/s11356-017-9318-5.
- Wang, P., X. Hu, S. Cook, M. Begonia, K. S. Lee, and H.-M. Hwang. 2008. Effect of culture conditions on the production of ligninolytic enzymes by white rot fungi Phanerochaete chrysosporium (ATCC 20696) and separation of its lignin peroxidase. World J. Microbiol. Biotechnol. 24:2205–12. doi:10.1007/s11274-008-9731-5.
- Whang, L.-M., P.-W. G. Liu, -C.-C. Ma, and -S.-S. Cheng. 2008. Application of biosurfactants, rhamnolipid, and surfactin, for enhanced biodegradation of diesel-contaminated water and soil. J. Hazard. Mater. 151:155–63. doi:10.1016/j.jhazmat.2007.05.063.
- Yamanaka, R., C. F. Soares, D. R. Matheus, and K. M. Machado. 2008. Lignolytic enzymes produced by Trametes villosa CCB176 under different culture conditions. Braz. J. Microbiol. 39:78–84. doi:10.1590/S1517-83822008000100019.
- Zeng, G.-M., J.-G. Shi, X.-Z. Yuan, J. Liu, Z.-B. Zhang, G.-H. Huang, J.-B. Li, B.-D. Xi, and H.-L. Liu. 2006. Effects of Tween 80 and rhamnolipid on the extracellular enzymes of Penicillium simplicissimum isolated from compost. Enzyme Microb. Technol. 39:1451–56. doi:10.1016/j.enzmictec.2006.03.035.
- Zhao, Z., A. Selvam, and J. W.-C. Wong. 2011. Effects of rhamnolipids on cell surface hydrophobicity of PAH degrading bacteria and the biodegradation of phenanthrene. Bioresour. Technol. 102:3999–4007. doi:10.1016/j.biortech.2010.11.088.
- Zhou, M.-F., X.-Z. Yuan, H. Zhong, Z.-F. Liu, H. Li, -L.-L. Jiang, and G.-M. Zeng. 2011. Effect of biosurfactants on laccase production and phenol biodegradation in solid-state fermentation. Appl. Biochem. Biotechnol. 164:103–14. doi:10.1007/s12010-010-9118-6.