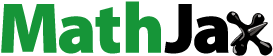
ABSTRACT
Disposal of spent catalyst in an economical and green way has become a great concern for industrial production. We developed a process including acid leaching, solvent extraction and stripping in order to recycle spent catalyst. In this study, we conducted selective recovery of molybdenum through focus on finding an optimized extraction and stripping process by comparing different extractants and stripping agents. To separate molybdenum from other metals efficiently and figure out the mechanism of extraction process, the five different extractants of methyl trioctyl ammonium chloride, tri-n-octylamine, tris (2-ethylhexyl) amine, bis (2-ethylhexyl) phosphate, and tributyl phosphate with different functional groups were examined; the extraction ability and extraction mechanism of these five extractants were systematically studied under the same system for the first time. It was found that more than 98% of the molybdenum could be extracted with an organic phase consisting of tri-n-octylamine or methyl trioctyl ammonium chloride under the optimal conditions. The result indicated that the tri-n-octylamine and methyl trioctyl ammonium chloride possess excellent molybdenum extraction ability, the extraction capacity of the rest extractants was in the order of bis (2-ethylhexyl) phosphate > tris (2-ethylhexyl) amine > tributyl phosphate. In the stripping process, NH4OH, NaOH, and H2SO4 were chosen as stripping agent to strip the molybdenum from the loaded tri-n-octylamine organic phase. The stripping ability of the three studied stripping agents was in the order NaOH > NH4OH > H2SO4. The Fourier transform infrared (FTIR) spectra showed that the structure of the tri-n-octylamine organic phase was stable during the extraction and stripping process. Results showed that molybdenum could be highly and efficiently recovered by optimized extraction and stripping process.
Implications: A series of different extractants and stripping agent have been systematically studied in order to compare their extraction and stripping ability under the same system. Based on the obtained results, an optimized extraction and stripping process was proposed to recycle molybdenum from spent catalyst efficiently. It is possible to dispose spent catalysts in an economic and environmental way by this developed metal recovery process.
Introduction
Catalysts have been widely used in the chemical, petroleum, and environment protection industry for their special ability to promote the reaction rate. Especially in petroleum refining production, large amounts of catalysts are required to transfer the crude oil into clean petroleum products (Hu et al. Citation2017; Yang et al. Citation2019). During the catalytic processing, these catalysts will get contaminated and become deactivated spent catalysts. These spent catalysts have been categorized as hazardous waste; potential environmental problems may be posed by accumulation or simple landfill treatment of the spent catalysts (Banda et al. Citation2013; Zheng et al. Citation2019). In the meantime, the spent catalyst of the petroleum refining industry contains a large amount of valuable metals such as molybdenum, which is a strategic metal with special properties widely used in many fields. But due to rapidly growing demand and ceaseless exploitation of molybdenum ores, it becomes more important to recover molybdenum from diverse resources, and the spent catalysts can be one of the major source of molybdenum (Akcil et al. Citation2015; Zhang et al. Citation2019). On account of on-going environmental concerns and resource utilization, it is critical to propose a method to recover the valuable metals from spent catalysts in an economical and environmental way.
During the past decade, a number of pyrometallurgical and hydrometallurgical processes have been developed and reported for the recovery of molybdenum from different kinds of molybdenum resources. It is known that hydrometallurgical processes exhibit significant advantages such as low energy consumption and low cost for the treatment over pyrometallurgical processes. Among the hydrometallurgical processes, solvent extraction was considered as an efficient method to recover molybdenum, it is widely used in practical production due to its simple operation, low cost, good selectivity, recyclability of extraction phase, and high extraction capacity (Meckes et al. Citation1997; Shah, Phadke, and Kocher Citation1995; Wu et al. Citation2012).
In recent years, a number of extractants have been reported to extract molybdenum; these extractants can be divided into four categories: amine extractants, phosphorus extractants, chelate extractants, as well as ionic liquids (Onghena et al. Citation2017a; Rout, Wellens, and Binnemans Citation2014). Amine extractants, including primary (Li et al. Citation2018; Ning, Cao, and Zhang Citation2010; Ning et al. Citation2014), secondary (Iatsenko et al. Citation2001), and tertiary (Morı́S, Dı́Ez, and Coca Citation1999; Parhi et al. Citation2011; Yang et al. Citation2016) amines, as well as quaternary ammonium salts (Rout et al. Citation2017; Wejman-Gibas et al. Citation2016), have been widely used for solvent extraction. Some phosphorus extractants such as bis (2-ethylhexyl) phosphate (Ojo and Ajayi Citation2013; Quijada-Maldonado, Torres, and Romero Citation2016; Yin et al. Citation2014), Cyanex923 (Batchu et al. Citation2017), and PC-88A (Xia et al. Citation2015) have been applied in many industrial fields. Some researchers have studied the extraction of molybdenum with chelate extractants such as LIX 63(Zeng and Cheng Citation2010), LIX 84-I (Park, Kim, and Parhi Citation2010), and LIX 622 N (Lasheen et al. Citation2014) and obtained many positive results. The molybdenum extraction ability is mainly affected by the complexation capability of extractants and the extraction condition. Figure out the differences of molybdenum extraction behavior of different extractants is one of the key parameters to find an optimized molybdenum recovery process. But most researches only used single extractant in various conditions.
In this case, the comparative extraction behavior of molybdenum with three different amine extractants and two phosphorus extractants was investigated under the same conditions in this study. The initial aqueous pH, extractant concentrations as well as extraction time were investigated to figure out the optimal extraction process. To realize highly efficient recovery of molybdenum, three different stripping agents were systematicaly and comparatively studied to find an efficient route to strip molybdenum from the loaded organic phase.
Experimental section
Leaching process
In this study, we used spent hydrodesulfurization (HDS) catalyst (Mo/Co-Al2O3) for the leaching process. The chemical composition of the spent HDS catalyst is presented in . Besides a high Mo content, the spent catalyst also contains impurities such as S, Si, and Fe. To recover the valuable metals, the spent catalyst was firstly calcined at a Muffle Furnace at 500 °C for 1 hour to remove impurities, the leaching efficiency of valuable metals can be greatly improved after calcination process. Then the calcined spent catalyst was leached with 2 mol/L sulfuric acid at 80 °C for 30 min in a round bottom flask. Heating and stirring were provided by a temperature controlled mantle heater and a stirrer motor, respectively. The concentration of metal ions was analyzed by ICP-OES, the results of leaching process presented in .
Table 1. Chemical composition of spent catalyst.
Table 2. Results of the leaching process.
Experimental materials
For solvent extraction study, methyl trioctyl ammonium chloride (Aliquat 336), tri-n-octylamine (Alamine 336), tris (2-ethylhexyl) amine (TEHA), bis (2-ethylhexyl) phosphate (DEHPA), and tributyl phosphate (TBP) were supplied by Aladdin Co., and used as received. Kerosene and n-decanol were supplied by Aladdin Co., and used as diluent and modifier for amine extractants, respectively. For stripping study, NaOH, NH4OH, and H2SO4 were supplied by Sinopharm Chemical Reagent Co., and used as stripping agents.
Experimental methods
The solvent extraction and stripping experiments were performed by equilibrating the organic and aqueous phases (20 ml each) with magnetic paddle and agitator in a 100 mL beaker at 30 ± 1°C. The mixture was separated with a separating funnel. The concentration of metal ions was analyzed by ICP-OES. The metal content in the loaded organic phase was estimated by mass balance, and the distribution ratio (D) was calculated as follows:
C1 = metal concentration in the organic phase.
C2 = metal concentration in the raffinate.
The extraction efficiency (E%) was calculated as follows:
The stripping efficiency (S%) was calculated as follows:
C3 = metal concentration in the stripped solution.
C4 = metal concentration in the loaded organic phase.
Results and discussion
Effect of aqueous solution pH
As it is crucial to figure out the relationship between the initial aqueous pH and extraction efficiency of molybdenum, the experiments were performed at an initial aqueous pH ranging from 0.5 to 3.0. The spent catalyst was leached with sulfuric acid, and the pH of the actual leach liquor was 0.3. It is noteworthy that the pH of leach liquor was adjusted with ammonia. Alamin336, Aliquat336, DEHPA, TEHA, TBP concentrations were maintained at 0.05 mol/L, and the reaction time was kept at 10 min, at an organic to aqueous volume ratio O/A = 1 and 5% (v/v) n-decanol added to the amine extractans as a modifier. The extraction efficiency of molybdenum is shown in . As can be seen, the extraction efficiency of molybdenum with Alamin336, Aliquat336, TEHA increased from 87.8%, 80.1%, and 4.2% to 98.4%, 98.3%, and 26.7%, respectively, with the increase of pH from 0.5 to 1. When the pH increased to 3, the extraction efficiency of molybdenum with Alamin336, Aliquat336, and TEHA decreased to 36.7%, 83.5%, and 1%, respectively; these results are consistent with former research (Padhan and Sarangi Citation2014). The extraction efficiency of molybdenum with DEHPA and TBP had no obvious change due to the low concentration of extractants. The reason of this phenomenon is that the molybdenum species of MoO42- possesses a strong binding ability with amine extractants (Parhi et al. Citation2011), and molybdenum species of MoO22+ becomes the main species at pH lower than 1 (Olazabal et al. Citation1992); the extraction efficiency of molybdenum drop as the pH increase to 3 because of the formation of other molybdenum species (Lasheen et al. Citation2014). The extraction efficiency with amine extractants at various pH have a similar tendency, the extraction stoichiometry of molybdenum in sulfuric acid solution with tertiary amine (Alamine 336 and TEHA) occur in two steps; it can be described as in EquationEquations (4)(4)
(4) and (Equation5
(5)
(5) ) (Zeng and Cheng Citation2009):
Figure 1. Effect of initial pH on molybdenum extraction behavior with different extractants under the same extractants concentration, extraction time, and O/A ratio.
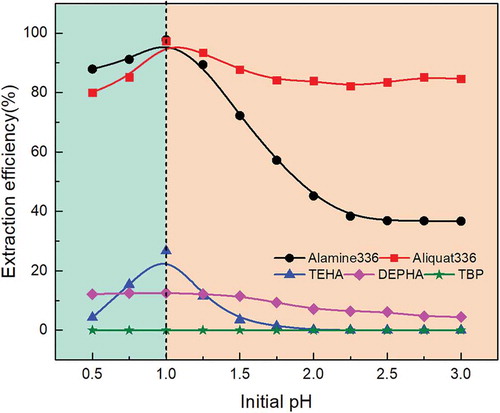
The extraction stoichiometry of molybdenum with Aliquat 336 can be described as in EquationEquation (6)(6)
(6) (Cheema et al. Citation2018):
The phosphorus extractants can be used to extract MoO22+ when the aqueous pH lower is than 1 (Zeng and Cheng Citation2009); the extraction stoichiometry of molybdenum with DEHPA can be described as in EquationEquation (7(7)
(7) ):
The extraction stoichiometry with TBP can be described as in EquationEquation (8(8)
(8) ):
The efficient separation of molybdenum was realized though the formation of a complex which can easily be dissolved in the organic phase, while other metals still remain in the aqueous phase. In this case, to maintain high extraction level of molybdenum, an initial aqueous pH of 1 was used in subsequent experiments. It is noteworthy that the co-extraction of cobalt and aluminum was nil within the entire pH range studied in , which indicated that cobalt and aluminum can not be extracted by these extractants in this pH range.
Effect of extractants concentration
As the extractant concentration is a critical condition of the molybdenum extraction process, experiments were performed at various concentrations of Aliquat 336, Alamine 336, DEHPA, TEHA, and TBP; the reaction time was kept at 10 min, the O/A ratio at 1/1, and the initial aqueous pH was 1. As shown in , by increasing the concentration of Aliquat 336 and Alamine 336 from 0.01 mol/L to 0.1 mol/L, the molybdenum extraction efficiency increased from 48.5% to 98.3% and 36.2% to 98.4%, respectively. When the Aliquat 336 and Alamine 336 concentration increased to 0.05 mol/L, the extraction efficiency of molybdenum approached 98%. By increasing the TEHA concentration from 0.01 mol/L to 0.3 mol/L, the molybdenum extraction efficiency sharply reached to 87.5% and kept invariant with the increase of TEHA concentration to 0.5mol/L. For phosphorus extractants, the extraction efficiency was 98.2% when the DEHPA concentration increased to 0.5mol/L. Furthermore, the molybdenum extraction ability by TBP was not as strong as previous extactants; when the TBP concentration increased to 3.5mol/L, the extraction efficiency nearly reached 85%. shows that the molybdenum extraction ability by Aliquat 336 and Alamine 336 reached the maximum value at a concentration of 0.05 mol/L, whereas the maximum extraction efficiency was reached at 0.3 mol/L, 0.5mol/L, and 3.5mol/L for TEHA, DEHPA, and TBP, respectively.
Figure 2. Effect of extractants concentration on molybdenum extraction behavior with different extractants under the same initial pH, extraction time, and O/A ratio.
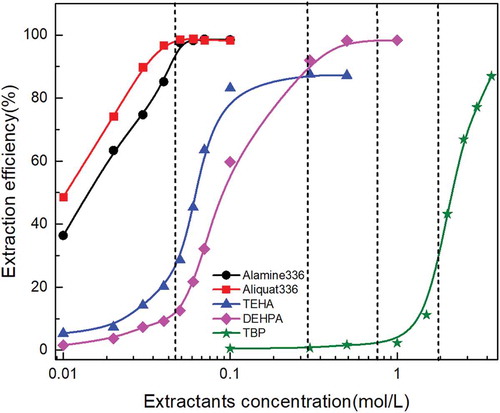
The extraction reactions of molybdenum with the studied extractants was described in EquationEquations (5)(5)
(5) , (Equation6
(6)
(6) ), (Equation7
(7)
(7) ), and (Equation8
(8)
(8) ). So, the equilibrium constant Kex can expressed as follows:
Taking the logarithm of EquationEquations (9)(9)
(9) , (Equation10
(10)
(10) ), (Equation11
(11)
(11) ), and (12) and rearrangement, the following equations relating the logarithm of equilibrium constant with the logarithm of extractant can be obtained:
The term Log Kex is constant at constant temperature, acidity, and ionic strength. Therefore, the only variable would be the concentration of extractant. In order to examine the mole number of extractant participated in the extraction reaction, a graph of log D vs. log [extractant] was plotted in . The slope values of each linear plot were shown in ; the slope value of Alamine 336, Aliquat 336, DEHPA, and TEHA was close to 2. The obtained slope value proved the suggested reaction in EquationEquation (4)(4)
(4) . It confirmed that two moles of these extractants reacted with each extracted metal ion and form a extraction complex. The slope value of TBP close to 3 was obtained, and it explained why more TBP was needed to reach a high extraction efficiency comparing to other extractants.
Effect of extraction time
The extraction kinetics with 0.05 mol/L Alamin 336, 0.05 mol/L Aliquat 336, 0.5mol/L DEHPA, 0.3 mol/L TEHA, as well as 3.5mol/L TBP were studied at initial aqueous pH of 1 and O/A ratio of 1:1. As can be seen in , all these extractants display a same extraction kinetics performance of molybdenum, the extraction of molybdenum with these extractants was fast and reached the equilibrium within 3 min, and the molybdenum extraction efficiency obtained to be maximum with increasing reaction time to 10 min; this was in accord with the former studies about the effect of reaction time (Parhi et al. Citation2011). The follow-up experiment was conducted with amine extractant Alamine 336 due to its superior extraction ability.
Extraction distribution isotherm of molybdenum with Alamine 336
The extraction distribution isotherm shows the change of molybdenum concentration in organic phase and aqueous phase. In this article, molybdenum extraction distribution isotherm was obtained through different O/A ratio with 0.05 mol/L Alamine 336 in kerosene and 5%(v/v) n-decanol at initial aqueous pH of 1, extraction time of 5 min, and the McCabe–Thiele diagram was plotted to investigate the theoretical number of stages for maximum molybdenum extraction in . It shows that two theoretical stages in counter-current are needed for quantitative extraction of molybdenum at O/A ratio of 1:1.
Figure 5. McCabe–Thiele plot for molybdenum extraction with 0.05 mol/L Alamine 336 at initial aqueous pH of 1 and extraction time of 5 min.
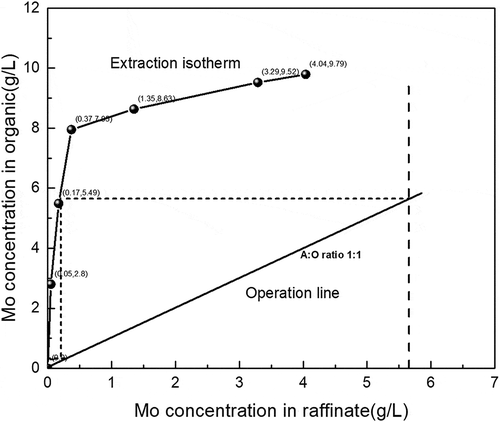
Three stages counter current simulation (CCS) study was conducted with 0.05 mol/L Alamine336 in kerosene and 5%(v/v) n-decanol, O/A ratio of 1:1, and equilibrium pH 1, the result being showed in . From the results of the CCS study, the extraction efficiencies of second stage were determined to be 99.5%. The final loaded organic was analyzed to contain 5.62 g/L molybdenum with no cobalt and aluminum. Then, a sufficient quantity of molybdenum loaded organic was generated to carry out the stripping studies.
Table 3. Results of the two-stage counter current extraction of molybdenum.
Effect of stripping agent concentration
The stripping of molybdenum from the loaded organic phase is an important process in the recovery of molybdenum. The stripping agent such as inorganic acids or inorganic bases are mainly used to destroy the structure of the extraction complex in the organic phase and form a molybdenum compound that is soluble in water. In the present study, inorganic bases NH4OH, NaOH and inorganic acids H2SO4 were chosen to strip molybdenum from the loaded Alamine 336 organic phase. Stripping studies were performed by increasing the concentration of NH4OH from 0.05 mol/L to 0.5mol/L, NaOH from 0.05 mol/L to 0.3 mol/L, and H2SO4 from 0.2 mol/L to 5 mol/L at O/A ratio of 1:1, for 10 min. As showed in , the stripping efficiency of molybdenum increased with the increasing of NH4OH concentration from 0.05 mol/L to 0.2 mol/L and NaOH concentration from 0.05 mol/L to 0.15 mol/L; the molybdenum stripping efficiency remained constant when NH4OH concentration and NaOH concentration were beyond 0.2 mol/L and 0.15 mol/L, respectively. The stripping efficiency of molybdenum from the loaded Alamine 336 increased from 2.8% to 88.2% with the increasing H2SO4 concentration from 0.2 mol/L to 3.5 mol/L and remained constant at 88.2% when more H2SO4 concentration was used. In this study, a maximum of 99.3% and 99.5% molybdenum stripping efficiency was achieved using 0.2 mol/L of NH4OH and 0.15 mol/L of NaOH, respectively. In an alkaline solution, mononuclear anionic species of molybdenum are predominant. The reason why NH4OH and NaOH have better stripping ability of molybdenum is that mononuclear anionic species of molybdenum are predominant in alkaline solution (Zeng and Cheng Citation2010), and these species are easier to form a soluble compound. It indicated that NaOH shows a better stripping ability as a stripping agent.
Effect of stripping time
shows the effect of stripping time on stripping efficiency of molybdenum with 0.2 mol/L NH4OH, 0.15 mol/L NaOH and 3.5 mol/L H2SO4 at O/A ratio of 1:1. It is seen in that the molybdenum stripping efficiency with NH4OH, NaOH, and H2SO4 increased from 26.1%, 54.6%, and 7.5% to 99.3%, 99.5%, and 88.2% with increasing reaction time from 0 to 2 min, while the stripping efficiency of the three stripping agents remained constant when the reaction time increased to 10 min, and afterwards the stripping rate was constant with the increasing of reaction time.
Stripping distribution isotherm of molybdenum
The stripping distribution isotherm of molybdenum is shown in . In the stripping process, the stripping studies were conducted with 0.15 mol/L NaOH at different O/A ratios. The obtained molybdenum stripping distribution isotherm illustrated that two theoretical counter current stages are needed for quantitative stripping of molybdenum at O/A ratio of 3:1.
Figure 8. McCabe–Thiele plot for molybdenum stripping with 0.15 mol/L NaOH and 4 min stripping time.
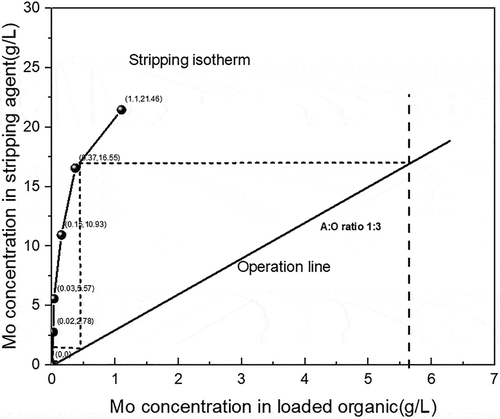
A two-stage CCS stripping study was conducted with 0.15 mol/L NaOH in the mentioned conditions, the results being showed in . From the results of the CCS study, the extraction efficiencies of the second stage were determined to be 99.4%, the stripped solution contained 16.75 g/L of molybdenum indicating the effective enrichment was achieved.
Table 4. Results of the two-stage counter current stripping of molybdenum.
Fourier transform infrared (FTIR) spectra analysis of extraction and stripping process
In order to study the mechanism of the extraction and stripping process, the Fourier transform infrared (FTIR) spectra of fresh organic phase (a), molybdenum loaded organic phase (b), and organic phase after stripping (c) were measured, respectively, all the organic phases consisting of Alamine 336 in kerosene and n-decanol as modifier. It can be seen from that the characteristic peaks of the fresh organic phase are almost identical to the organic phase after stripping and the same peak including the stretching vibration of C–H (≈2800 cm−1) and C-N (≈1350 cm−1) appear in all three organic phases. Furthermore, the peak at 929 cm−1 shows in loaded organic phase (b) is due to Mo-O stretching vibrations in the polyoxomolybdate which are extracted by Alamine 336 (Bal et al. Citation2004). This result verified the molybdenum species and extraction mechanism proposed before, indicating that the complete stripping of molybdenum is obtained, and the structure of Alamine 336 organic phase was stable during the extraction and stripping process, which could be regenerated and achieve recycling extraction.
Conclusion
The selective recovery of molybdenum from the spent catalyst with various extractants has been investigated. The effect of initial aqueous pH, extractants concentration, reaction time, extraction distribution isotherm, and stripping agent concentrations was examined. The results indicated as follows:
All the extractans show better extraction ability of molybdenum at initial aqueous pH of 1, and amine extractants show a better extraction ability than phosphorus extractants; especially for Aliquat 336 and Alamine 336, the extraction efficiency reached above 98% under suitable conditions. In the stripping process, NaOH shows a superior stripping ability, and 0.15 mol/L of NaOH was enough to strip almost all the molybdenum from the loaded organic phase.
The extraction and stripping ability of different extractants and stripping agent have been well compared in this study. Based on the obtained results, an optimized process consist of extraction with Alamine 336 and stripping with NaOH to achieve highly efficient recovery of molybdenum from spent catalyst has been proposed.
Disclosure statement
No potential conflict of interest was reported by the authors.
Additional information
Funding
Notes on contributors
Menglei Zhang
Menglei Zhang is a Ph.D. candidate at State Key Laboratory of Clean Energy Utilization, State Environmental Protection Center for Coal-Fired Air Pollution Control, Zhejiang University, Hangzhou, China.
Hao Song
Hao Song is a Ph.D. at State Key Laboratory of Clean Energy Utilization, State Environmental Protection Center for Coal-Fired Air Pollution Control, Zhejiang University, Hangzhou, China.
Chenghang Zheng
Chenghang Zheng is a professor at State Key Laboratory of Clean Energy Utilization, State Environmental Protection Center for Coal-Fired Air Pollution Control, Zhejiang University, Hangzhou, China.
Zhenglong Lin
Zhenglong Lin is a graduate student at State Key Laboratory of Clean Energy Utilization, State Environmental Protection Center for Coal-Fired Air Pollution Control, Zhejiang University, Hangzhou, China.
Yi Liu
Yi Liu is a graduate student at State Key Laboratory of Clean Energy Utilization, State Environmental Protection Center for Coal-Fired Air Pollution Control, Zhejiang University, Hangzhou, China.
Weihong Wu
Weihong Wu is an associate professor at State Key Laboratory of Clean Energy Utilization, State Environmental Protection Center for Coal-Fired Air Pollution Control, Zhejiang University, Hangzhou, China.
Xiang Gao
Xiang Gao is a professor at State Key Laboratory of Clean Energy Utilization, State Environmental Protection Center for Coal-Fired Air Pollution Control, Zhejiang University, Hangzhou, China.
References
- Akcil, A., F. Vegliò, F. Ferella, M. D. Okudan, and A. Tuncuk. 2015. A review of metal recovery from spent petroleum catalysts and ash. Waste Manage. 45 (Urban Mining):420–33. doi:10.1016/j.wasman.2015.07.007.
- Bal, Y., K. E. Bal, G. Cote, and A. Lallam. 2004. Characterization of the solid third phases that precipitate from the organic solutions of Aliquat? 336 after extraction of molybdenum(VI) and vanadium(V). Hydrometallurgy 75 (1–4):123–34.
- Banda, R., T. H. Nguyen, S. H. Sohn, and S. L. Man. 2013. Recovery of valuable metals and regeneration of acid from the leaching solution of spent HDS catalysts by solvent extraction. Hydrometallurgy 133 (2):161–67. doi:10.1016/j.hydromet.2013.01.006.
- Batchu, N. K., T. Vander Hoogerstraete, D. Banerjee, and K. Binnemans. 2017. Separation of rare-earth ions from ethylene glycol (+LiCl) solutions by non-aqueous solvent extraction with Cyanex 923. RSC Adv. 7 (72):45351–62. doi:10.1039/C7RA09144C.
- Cheema, H. A., S. Ilyas, S. Masud, M. A. Muhsan, I. Mahmood, and J.-C. Lee. 2018. Selective recovery of rhenium from molybdenite flue-dust leach liquor using solvent extraction with TBP. Sep. Purif. Tech. 191 (116–121):1383–5866. doi:10.1016/j.seppur.2017.09.021.
- Hu, W., Y. Zhang, S. Liu, C. Zheng, X. Gao, I. Nova, and E. Tronconi. 2017. Improvement in activity and alkali resistance of a novel V-Ce(SO 4) 2/Ti catalyst for selective catalytic reduction of NO with NH 3. Appl Catal B 206 (Complete):449–60. doi:10.1016/j.apcatb.2017.01.036.
- Iatsenko, G. N., A. A. Palant, V. A. Petrova, and R. K. Tagirov. 2001. Solvent extraction of molybdenum (VI), tungsten (VI) and rhenium (VII) by diisododecylamine from leach liquors. Hydrometallurgy 60 (1):1–5. doi:10.1016/S0304-386X(00)00123-7.
- Lasheen, T. A., M. E. Ibrahim, H. B. Hassib, and A. S. Helal. 2014. Recovery of molybdenum from uranium bearing solution by solvent extraction with 5-Nonylsalicylaldoxime. Hydrometallurgy 146 (3):175–82. doi:10.1016/j.hydromet.2014.03.011.
- Li, Z., G. Zhang, W. Guan, L. Zeng, L. Xiao, L. Qinggang, Z. Cao, and L. Xiuyuan. 2018. Separation of tungsten from molybdate using solvent extraction with primary amine N1923. Hydrometallurgy 175:203–07. doi:10.1016/j.hydromet.2017.10.018.
- Meckes, M. C., J. Tillman, L. Drees, and E. Saylor. 1997. Removal of PCBs from a contaminated soil using CF-systems solvent extraction process. J. Air Waste Manage. Assoc. 47 (10):1119–24. doi:10.1080/10473289.1997.10464404.
- Morı́S, M. A., F. V. Dı́Ez, and J. Coca. 1999. Solvent extraction of molybdenum and tungsten by Alamine 336 and DEHPA in a rotating disc contactor. Sepa. Purif. Tech. 17 (3):173–79. doi:10.1016/S1383-5866(99)00022-2.
- Ning, P., H. Cao, and Y. Zhang. 2010. Selective extraction and deep removal of tungsten from sodium molybdate solution by primary amine N1923. Sepa. Purif. Tech. 70 (1):27–33. doi:10.1016/j.seppur.2009.08.006.
- Ning, P., X. Lin, H. Cao, and Y. Zhang. 2014. Selective extraction and deep separation of V(V) and Cr(VI) in the leaching solution of chromium-bearing vanadium slag with primary amine LK-N21. Sepa. Purif. Tech. 137 (nov):109–15. doi:10.1016/j.seppur.2014.08.033.
- Ojo, J. O., and O. O. Ajayi. 2013. Mechanism of the extraction of Molybdenum (VI) from diluted HCl and HNO3 solutions with di(2-ethylhexyl)phosphoric acid. Int. J. Biolog. Chem. Sci. 7 (3):1370. doi:10.4314/ijbcs.v7i3.42.
- Olazabal, M. A., M. M. Orive, L. A. Fernã¡Ndez, and J. M. Madariaga. 1992. Selective extraction of vanadium (V) from solutions containing molybdenum (VI) by ammonium salts dissolved in toluene. Solvent Extr. Ion Exch. 10 (4):623–35. doi:10.1080/07366299208918125.
- Onghena, B., S. Valgaeren, T. Vander Hoogerstraete, and K. Binnemans. 2017a. Cobalt (II)/nickel (II) separation from sulfate media by solvent extraction with an undiluted quaternary phosphonium ionic liquid. RSC Adv. 7 (57):35992–99. doi:10.1039/C7RA04753C.
- Padhan, E., and K. Sarangi. 2014. Separation of molybdenum and cobalt from spent catalyst using Cyanex 272 and Cyanex 301. Int. J. Miner. Process. 127 (2):52–61. doi:10.1016/j.minpro.2014.01.003.
- Parhi, P. K., K. H. Park, H. I. Kim, and J. T. Park. 2011. Recovery of molybdenum from the sea nodule leach liquor by solvent extraction using Alamine 304-I. Hydrometallurgy 105 (3):195–200. doi:10.1016/j.hydromet.2010.09.004.
- Park, K.-H., H.-I. Kim, and P. K. Parhi. 2010. Recovery of molybdenum from spent catalyst leach solutions by solvent extraction with LIX 84-I. Sep. Purif. Tech. 74 (3):294–299 1383-5866. doi:10.1016/j.seppur.2010.06.018.
- Quijada-Maldonado, E., M. J. Torres, and J. Romero. 2016. Solvent extraction of Molybdenum (VI) from aqueous solution using ionic liquids as diluents. Sepa. Purif. Tech. 200:200–06.
- Rout, A., S. Wellens, and K. Binnemans. 2014. Separation of rare earths and nickel by solvent extraction with two mutually immiscible ionic liquids. RSC Adv. 4 (11):5753–58. doi:10.1039/c3ra46261g.
- Rout, P. C., G. K. Mishra, B. Padh, K. R. Suresh, and B. Ramachandra Reddy. 2017. Solvent extraction separation of molybdenum as thio-molybdate complex from alkaline tungsten leach liquor of spent HDS catalyst–A pilot study. Hydrometallurgy 174 (140–146):0304–386X. doi:10.1016/j.hydromet.2017.10.002.
- Shah, D. B., A. V. Phadke, and W. M. Kocher. 1995. Lead removal from foundry waste by solvent extraction. J. Air Waste Manage. Assoc. 45 (3):150–55. doi:10.1080/10473289.1995.10467354.
- Wejman-Gibas, K., K. Wieszczycka, A. Wojciechowska, K. Ochromowicz, and P. Pohl. 2016. Extraction of molybdenum(VI) from sulfate media by 3-pyridineketoxime and its quaternary salts. Sepa. Purif. Tech. 158:71–79. doi:10.1016/j.seppur.2015.11.037.
- Wu, J., C. Wei, X. Li, S. Wang, M. Wang, and C. Li. 2012. Selective extraction of Mo using Cyanex-272 and tributyl phosphate from low grade Ni–Mo ore leach liquor. Sepa. Purif. Tech. 99 (41):120–26. doi:10.1016/j.seppur.2012.08.007.
- Xia, Y., L. Xiao, C. Xiao, and L. Zeng. 2015. Direct solvent extraction of molybdenum(VI) from sulfuric acid leach solutions using PC-88A. Hydrometallurgy 158:114–18. doi:10.1016/j.hydromet.2015.10.016.
- Yang, X., Y. Zhang, S. Bao, and C. Shen. 2016. Separation and recovery of vanadium from a sulfuric-acid leaching solution of stone coal by solvent extraction using trialkylamine. Sepa. Purif. Tech. 164:49–55. doi:10.1016/j.seppur.2016.03.021.
- Yang, Z., J. Peidong, Q. Li, Y. Jiang, C. Zheng, Y. Wang, X. Gao, and R. Lin. 2019. Comprehensive understanding of SO3 effects on synergies among air pollution control devices in ultra-low emission power plants burning high-sulfur coal. J. Clean. Prod. 239. doi:10.1016/j.jclepro.2019.118096.
- Yin, S. H., S. W. Li, W. Y. Wu, X. Bian, J. H. Peng, and L. B. Zhang. 2014. Extraction and separation of Ce(III) and Pr(III) in the system containing two complexing agents with di-(2-ethylhexyl) phosphoric acid. RSC Adv 4 (104):59997–60001. doi:10.1039/C4RA10143J.
- Zeng, L., and C. Y. Cheng. 2009. A literature review of the recovery of molybdenum and vanadium from spent hydrodesulphurisation catalysts: Part II: Separation and purification. Hydrometallurgy 98 (1):10–20. doi:10.1016/j.hydromet.2009.03.012.
- Zeng, L., and C. Y. Cheng. 2010. Recovery of molybdenum and vanadium from synthetic sulphuric acid leach solutions of spent hydrodesulphurisation catalysts using solvent extraction. Hydrometallurgy 101 (3):141–47. doi:10.1016/j.hydromet.2009.12.008.
- Zhang, S., S. Liu, X. Zhu, Y. Yang, W. Hu, H. Zhao, R. Qu, C. Zheng, and X. Gao. 2019. Low temperature catalytic oxidation of propane over cobalt-cerium spinel oxides catalysts. Appl. Surf. Sci. 479:1132–40. doi:10.1016/j.apsusc.2019.02.118.
- Zheng, C., L. Xiao, R. Qu, S. Liu, Q. Xin, P. Ji, H. Song, W. Wu, and X. Gao. 2019. Numerical simulation of selective catalytic reduction of NO and SO2 oxidation in monolith catalyst. Chem. Eng. J. 361:874–84. doi:10.1016/j.cej.2018.12.150.