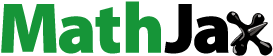
ABSTRACT
Globally, waste electrical and electronic equipment is one of the fastest-growing waste sectors. Mobile phones constitute the major portion of the telecommunication e-waste category. Over the years, waste mobile phones were considered as a potential source of secondary metals. This study aims to determine the physical and chemical composition of the discarded mobile phones and to evaluate its recovery potential. The printed circuit boards from the discarded (waste) mobile phones (MPCB) were collected and samples of different sizes 3 × 3 cm, 2 mm, 1 mm, 500 µm, and 150 µm were obtained after milling and sieving. Elemental composition revealed the presence of base metals, Cu, Zn, Fe, Ni, and Pb, in higher quantities with a significant amount of precious metals Au and Ag. Amount of base metals present in different MPCB size fractions was found in the order 3 × 3 cm > 2 mm > 1 mm > 500 µm >150 µm. The amount of precious metals like Ag and Au was found to be higher in large-sized MPCB fractions. FTIR studies declared the presence of polymers like ABS, PC, and HIPs in MPCB samples. TCLP tests for toxic metals revealed that MPCBs contained high concentrations of cadmium, lead, and mercury highlighting their hazardous potential. The ultimate analysis revealed that NMF has a GCV of 12.34 MJ/kg and a volatile content of 42.25%, which can be a potential source of energy that can be recovered through the gasification or pyrolysis process. Overall, the comprehensive characterization of waste MPCBs will systematically provide a better understanding of e-waste recycling processes for beneficiation purpose and sustainable resource utilization.
Implications: A comprehensive characterization of waste mobile phone printed circuit boards for its elemental composition was performed. Mechanical treatment steps before MPCBs processing increased the exposure of metals resulting in a higher concentration of metals in acid-digested samples. The elemental analysis of MPCBs revealed that MPCBs possessed significant quantities of base and precious metals. The amount of precious metals like Ag and Au was also found in higher ranges in large-sized MPCB fractions, which elucidated fact to be considered in the pre-treatment process for metal recoveries. The high content of base and precious metals in waste mobile phones displayed their economic potential in the market. This new source may compensate for the escalating global demand for gold and silver. Results from the study indicated that MPCBs can serve as an excellent secondary source for various metals and as an efficient alternative fuel.
Introduction
Urban mining of e-waste
Waste electrical and electronic equipment (WEEE) is the complex and fast-growing waste stream that covers a large variety of products (Baldé et al. Citation2015). The amount of WEEE generated in 2016 was 44.7 million metric tonnes (Mt), according to the “Global E-waste Monitor 2017” survey conducted by the United Nations environment program. It is also forecasted to escalate to 52.2 Mt by 2021 (Balde et al. Citation2017). To minimize and solve the global e-waste issues, a sustainable production process must be optimized to recycle and reuse materials from these secondary resources. In line with the concept of the circular economy, landfill mining was followed for the extraction of elements from the e-waste stocks. Such a process of recovering compounds and elements from any anthropogenic wastes from industries and households, i.e., secondary resources is termed urban mining (Cossu and Williams Citation2015; Krook et al. Citation2011).
WEEEs are still considered the backbone of urban mining as they contain relatively high concentrations of many metals, especially copper, nickel, gold, silver, platinum group metals, but also rare earth elements (REE), gallium, germanium, indium, tantalum, etc., including those currently classified as critical raw materials. The most significant advantage of urban mining of e-waste is that the amount of valuable metals recoverable is up to ten times greater than the amount extracted from natural ores (Szamałek and Galos Citation2016). The extraction of minerals from ores involves geological study and survey before mining, which is costly, and the amount of metal extracted cannot be predicted. As an example, the gold recovery from conventional gold mine yields only 1–5 g per ton of ore, whereas urban mining of e-waste may represent 250–350 g per ton of scrap (Owens Citation2013). The efficient reuse of e-waste produced over the years can reduce the demand for primary raw materials and will result in the substitution of the entire product, components, and materials. Recycling of e-waste also boosts the reduction of new material exploitation and supports urban mining, reducing energy consumption, lowering emissions, and reinforcing sustainability standards (Xavier et al. Citation2019).
Metals make up most of the portion of e-waste, which adds more economic value and receives much attention in the recycling process. Metal values in the e-waste are present in their naïve metallic form or as alloys fixed in nonmetallic parts. Metal values present in e-waste are generally classified into base metals, precious metals, and toxic metals (Oguchi, Sakanakura, and Terazono Citation2013). Base metals, precious metals, and REEE are collectively termed as technology metals as they possess both technological and economic potential (Işıldar et al. Citation2018). Most of the minerals have been listed as critical since their supply is much lower than the ever-increasing demand. Also, they are considered to be strategic as they do not have any substitutes (Bakas Citation2016). Precious and vital minerals constitute about 80% of the intrinsic value of the equipment, recycling these strategic elements could contribute to reducing dependence on the natural resources, encouraging recycling sectors, reducing environmental impacts, and handling e-waste management (Nguyen et al. Citation2017). Also, urban mining of e-waste can be seen as a multifaceted solution to social (creating jobs), environmental (reducing environmental impacts and avoiding conventional mining) and economic benefits (making profits).
Circular economy and e-waste
A circular economy (CE) describes an economic system that is based on business models that replace the ‘end-of-life’ concept with reducing, alternatively reusing, recycling, and recovering materials in production and consumption processes. It also aims to accomplish sustainable development, which implies creating environmental quality, economic prosperity, and social equity, to the benefit of current and future generations (Kirchherr, Reike, and Hekkert Citation2017). CE cyclically closes the loop since the linear economy is unsustainable from both material and environmental point of view (Burlakovs et al. Citation2018). Several sustainability steps have been gradually introduced, the CE model has the potential to bring about many amendments and new developments that may significantly combat e-waste management issues. While the conventional linear model does not consider the finiteness of the natural resources, the CE model manages to integrate the finiteness of resources into the model and proposes the reintroduction of elements from secondary resources like e-waste into a regenerative system (Blériot and Johnson Citation2013).
CE model involves the recovery of metals from scraps, which is the real economic value of e-waste, taking into account their mineralogy, particularly the precious metals (Au, Ag, and platinum group of metals) which are the significant drivers of the recycling system and are the basis of a CE. The complexity of WEEE makes it rather challenging to capture everything that has a value from these goods while creating the minimum amount of final waste, which is key to creating a CE and a resource-efficient society (Reuter and van Schaik Citation2016). An effective strategy focussing on improving the technological abilities of electronic waste recyclers, enhancing the repair and reuse of parts, and investing in end-processing technologies for materials recovery must be developed. To be able to do so, we need to understand the composition and how the precious metals, base metals, and platinum group metals behave in the recycling system and how the complex interactions of materials and product design affect their loss from the CE.
End-of-life mobile phones as a source of metals to be recycled
Mobile phones are categorized under small IT and telecommunication equipment, the most widely used item among all the electronic equipment (Robinson Citation2009). According to a report, the world accounts for 7.7 billion mobile phone subscriptions and 4.2 billion active mobile broadband connections (ITU Citation2016). About 80% of the world’s population equipped with mobile phones and cellular connections eventually generates enormous end-of-life (EoL) mobile phones (Arshadi and Mousavi Citation2015; Nnorom and Osibanjo Citation2009). The lower lifetime of mobile phones of about only 9–18 months is also responsible for the enormous generation of EoL mobile phones. It is further driven by the relatively short time replacement period of the users to get new/upgraded devices often and even before it becomes nonfunctional. Thus, obsolete mobile phones were predominant in the small IT equipment waste category of 3.9 Mt generated in 2016 (Balde et al. Citation2017).
These Mobile phones are complicated devices with a complex organization of different components. Most of the mobile phones constitute printed circuit boards (PCB), liquid crystal display (LCD), keypad, battery, and charger. PCBs are mostly constructed using copper and were soldered to the board using adhesives and metal coatings. PCBs are usually made of fiberglass, epoxy resin, and coated with gold plating. They also contain hazardous substances such as arsenic, antimony, beryllium, brominated flame-retardants, cadmium, lead (soldering and connectors) in addition to the base and precious metals (Jakub Citation2014). LCDs made of liquid crystals containing toxic substances like mercury make them hazardous (Işıldar et al. Citation2018). Mobile phone batteries are composed of lithium, cadmium, nickel, zinc, and copper. While the portable phone charger used to recharge the cell is made of copper wires encased in plastics, with tiny amounts of gold, cadmium, and brominated flame-retardants. The plastic casing usually composed of polycarbonates (PC), acrylonitrile butadiene styrene (ABS), and sometimes the combination of both (Nnorom and Osibanjo Citation2009). The other components include the antenna, speaker, and accessories, which may possess a limited range of heavy metals and hazardous materials (Tanskanen Citation2012). About more than 70% of mobile phone components can be recycled, but only about 0.003% of the mass of mobile phones only have been recycled of the total weight of the waste every year globally (Rowley Citation2006). The PCB of a mobile phone comprises about 20–35% of the total weight of a mobile phone (Ghosh et al. Citation2015). It also consists of 30% metals and 70% nonmetals. About 340 g of gold, 3.5 kg of silver, 140 g of palladium, 130 kg of copper were recoverable from per tonne of mobile phone waste (Sohaili, Muniyandi, and Mohamad Citation2012).
PCB is an integral part of mobile phones, which electrically connects and supports the other electronic components of the mobile phone. Different methodologies were followed by various researchers for the quantification of metal values contained in printed circuit boards of mobile phones (Kasper et al. Citation2011; Maragkos, Hahladakis, and Gidarakos Citation2013; Priya and Hait Citation2018; Vats and Singh Citation2015). The composition of the waste mobile phone printed circuit boards (MPCB) is diverse and has a complex structure, varying composition of components. Usually, the structure of MPCB is classified into single-layered, double-layered, or multi-layered based on the construction of conductive layers and laminates (Ghosh et al. Citation2015). Typical composition of MPCB consists of 30% of polymers, 30% of refractory oxides, and 40% of metals (Kasper et al. Citation2011). Most MPCBs are made of FR-4 type epoxy glass, with a copper-clad laminate coating made of epoxy resin, glass fiber, and copper of high purity (Kumar, Holuszko, and Janke Citation2018).
The metallic composition of MPCB varies with the type of mobile phones such as keypad mobiles, smartphones, different models, and different brands. Metal composition of mobile phones is characterized as precious metals (Au and Ag), base metals (Al, Cu, Al, Fe, Ni, Sn, and Zn), and hazardous metals (As, Be, Cd, Hg, In, Pb, and Sb) (Khaliq et al. Citation2014). For different intended purposes, various metals are used in MPCB. A typical MPCB was found to be contained 39.6% of Copper, 3.42% of Nickel, 3.43% of Zinc, 2.09% of Tin, 1.42% of Iron, 1.17% of Lead, 0.31% of Aluminum, 0.06% of Gold, and 0.06% of Silver out of 51% of total metal composition (Kasper et al. Citation2011). Apart from the abundant presence of the base and precious metals, MPCBs also contain a considerable quantity of toxic metals that cannot be ignored. (Maragkos, Hahladakis, and Gidarakos Citation2013) determined the total heavy metal content in each of the mobile phone parts printed circuit boards, plastic housing (PH), and liquid crystal display (LCD). The results revealed that the MPCBs of cellular phones contained higher concentrations of heavy metals, while PH and LCD monitor concentration levels were much lower. The characterization of the nonmetal fraction of MPCBs by (Kumar, Holuszko, and Janke Citation2018) revealed the presence of harmful PBDEs and toxic metals like antimony. Also, the lead leachate concentration was found to be 18 mg/L.
Given the enormous amount of obsolete mobile phones generated, the complexity of MPCBs and high metal content; the physical and chemical characteristic of waste MPCBs is important for prioritizing metals to be recovered and recycled. Though MPCBs are considered as a potential source of valuable metals among WEEE, the precise information on their physical and chemical characteristics is sparsely available in the existing reports (Holgersson et al. Citation2018; Maragkos, Hahladakis, and Gidarakos Citation2013; Oguchi et al. Citation2011). Several studies were conducted on the elemental composition of MPCB metallic portion and very sparse information exist on the characteristics of MPCB nonmetallic portions leading to sheer waste of resources (Maragkos, Hahladakis, and Gidarakos Citation2013; Vats and Singh Citation2015). Hence, this study aims at the comprehensive characterization of metallic and nonmetallic portions of obsolete MPCBs. The variation of metal content among different size MPCB fractions is investigated to suggest the best MPCB fraction size for metal recovery through different recovery techniques including pyrometallurgy, hydrometallurgy, and biohydrometallurgy (Annamalai and Gurumurthy Citation2019; Gurumurthy and Annamalai Citation2019). Also, this study aims to perform a study on their polymer content, metal recovery priority, and economic value to provide useful information for the eco-design of mobile phones to facilitate the separation of materials in the different recovery techniques and to assist policymakers involved in material sustainability and future recycling practices.
Methods
Sample collection
A mixed proportion of old mobile phones of different brands, especially keypad models, was selected for this study. Approximately 5 kg of waste mobile phone scraps were collected from the local mobile phone service centers and repairers at Vellore, Tamil Nadu, India. The collected mobile phones were not in working condition, and some had only the case and motherboard without batteries. The collected samples were stored in plastic bins.
Shredding and segregation
The mobile phones were dismantled to remove all the major components like MPCBs, plastic casing, LCDs, battery, keypad, camera, and connectors. The connectors. Dismantled MPCBs subjected to comminution using stainless scissors to dimensions approximately 3 cm x 3 cm. Further size reduction achieved by hammer milling (Model HM 10, India) and subjected to electrostatic separation (Model ES 1010, India) to isolate metallic fractions (MF) from nonmetallic fractions (NMF). MF with uniform sizes of 3 × 3 cm, 2 mm, 1 mm, 500 µm, and 150 µm were achieved through sieving with sieves of respective mesh sizes. Samples of different sizes were collected and stored in separate bins for further analysis. The metallic composition may vary with the sample size fractions; hence, all the sample size fractions were subject to metal analysis and leaching tests. The nonmetallic portion, which majorly contains the laminates, glass fibers, epoxy polymers, and plastics, was used for carrying out proximate and ultimate analysis.
Acid digestion of MPCB
About 1.0 g ± 0.005 g of MF of different size fractions was weighed added to 250 mL Erlenmeyer flasks. The sample was digested in 50 mL of 1:3 mixture of HNO3:HCl (aqua regia) and refluxed in a round bottom flask for 1 hr to dissolve as much as particles as possible (Kim, Seo, and Roh Citation2018). Then, the sample was allowed to cool and left undisturbed for 48 hr for complete digestion. The digested liquor was transferred to a 100 mL volumetric flask, and the volume was made up to 100 mL using ultra-pure water. The resultant liquid was filtered through a 25 mm Whatman filter paper, 0.45 µm Nylon syringe filter, and then diluted with ultra-pure water to lower concentrations. The aqueous leachate of different size fractions was stored at 4°C. Metal values were analyzed through inductively coupled plasma optical emission spectroscopy (ICP-OES, Perkin Elmer Optima 5300 DV, India) in duplicates. Each MF was quantitatively analyzed for Ag, Al, Au, Fe, Ni, Pb, Pd, and Zn and the total metal content present. The residues collected after filtration were weighed until constant mass and stored in a dry container for qualitative elemental analysis by Scanning Electron Microscopy/Energy Dispersive X-ray spectroscopy (SEM/EDX).
Toxicity characteristic leaching procedure (TCLP)
Leaching characteristic tests were conducted according to US EPA SW-846 method 1311 and compared the metal concentration of extracts with regulatory levels to determine the toxicity level of the as-received MPCB fractions. The samples with values that exceeded the threshold limits were characterized as hazardous waste. The MPCB fractions were extracted with extraction fluids and are a function of the alkalinity of the samples. About 10 g of the MPCB fraction was added to 100 mL of extraction fluid (glacial acetic acid), and the mixture was agitated for 24 hr at room temperature. After that, the mixture was filtered, and the TCLP extracts were collected. The extracts were acidified using 5% Con.HNO3 and stored at 4°C for metal analysis using ICP-OES (US EPA Citation1992). The TCLP extract was analyzed for the indicated heavy metal concentrations and compared with the US EPA regulatory level.
Proximate and ultimate analysis
Proximate and ultimate analyses were performed using the segregated nonmetallic fractions (NMF) of the MPCB fractions. The differential density in deionized water was used to remove light fractions containing mostly fiber, polymers, and ceramic materials from the substantial fraction that contained metals and ceramic layers. The floating lighter portions were removed while the heavier particles were decanted. Lighter NMF was analyzed for its material composition while the proximate, ultimate analysis, and gross calorific value (GCV) were carried out. According to the American standard for testing materials, ASTM D-3173-87 (moisture and fixed carbon) (ASTM Citation1998), ASTM D-3175-89a (volatile material) (ASTM Citation1988b), and ASTM D-3174-93 (ash content) (ASTM Citation1988a), the proximate and ultimate analyses were carried out. Bomb calorimeter was used to determine the calorific value, according to ASTM D-2015 (ASMT Citation1996).
Calculation of intrinsic and total value of metals present in MPCB
The estimation of the market value of metals present in MPCBs is vital to ensure the economic feasibility of the recovery of metal values from the MPCBs. According to the mass fraction of each mineral present in MPCBs, the intrinsic costs were calculated. For this estimation, the metal prices as per the London Metal Exchange on a three-month average scale were considered. Metal concentration values were calculated with their average values according to their respective weight fractions in MPCBs.
Results and discussion
Dismantling of waste mobile phones
Mobile phone consists of primary parts such as the battery, PCB, connectors, keypad, motherboard, and metallic components. The description of the elements of a typical keypad mobile phone of the 2012 model from the samples collected is represented in . The average weight of the mobile phone was 64.1 g, in which motherboard and casing account for the significant weight of 34.09% and 25.89%, respectively. Based on the manufacturer, design, model, and age of mobile phones, the composition and the content of the mobile phones may be varied (Oguchi et al. Citation2011; Paiano, Lagioia, and Cataldo Citation2013).
Table 1. Weight of individual components of a typical mobile phone
Elemental composition of MPCB
The acid-digested samples were diluted before ICP-OES analysis to meet the requirement of the equipment. It is one of the most precise instruments for metal analysis in leached solutions. The instrument calibration is done by using deionized water and standard reference materials for all the metals. The mass of metal dissolved in acid digestion analyzed from ICP-OES observations is calculated as follows:
Hence, the metal content in acid digested MPCB can be expressed as:
where m is the mass of metal dissolved in acid digestion (mg), v is the volume of acid used in acid digestion (mL), c is the concentration of metal analyzed by ICP-OES (mg/L), and w is the mass of MPCB used in acid digestion (g). The metal composition of the acid-digested MPCB samples is shown in . The values obtained were compared with the other results available in the literature as shown in .
Table 2. Elemental composition of different MPCB size fractions analyzed after acid digestion (Results of duplicates with Mean ± SD were reported)
Table 3. Elemental composition of MPCBs (as received) in the present study and values reported by other authors (all values in %)
Metals make up almost about 40% by mass of mobile phone PCBs and the rest composed of plastics, ceramics, and refractory oxides. Metals such as iron, nickel, lead, and zinc constitute the bulk of the mobile phone MPCBs. Precious metals like gold, silver, and palladium were also present in significant quantities. The results also substantiated well by other studies carried out over recent years (Ilyas and Lee Citation2014; Jianfeng et al. Citation2009; Pei et al. Citation2010; Pant et al. Citation2012; Zhu et al. Citation2011). The content of Ag and Au is higher when compared with the MPCB sample reported by Willner (Fornalczyk et al. Citation2013). The differences in metal composition of MPCBs may be due to the different characterization methods followed, analytical methods used, design, make, and model of the mobile phones. Variation in the metal composition was observed for different MPCB sample sizes. The concentration of base metals like aluminum, iron, lead, and zinc was found in the order 3 × 3 cm > 2 mm > 1 mm > 500 µm >150 µm. The amount of precious metals like Ag and Au was also found in higher ranges in large-sized MPCB fractions as depicted in . The results elucidated that the content of metal concentrations in MPCB samples increases with an increase in size. The results can be applied in the pre-treatment process for preparing MPCB samples of the higher metal content and for designing an active recovery technique.
Microstructure and chemical composition of MPCB
SEM micrographs of the MPCB sample of smaller size (150 µm) are shown in for a better understanding of the morphology of MPCB samples. It can be observed that the MPCB sample is heterogeneous with non-uniform sizes, shapes, and textures. It also revealed that particles exist as rods, spheres of fine crystals and flakes on the surfaces. Before acid digestion, the MPCB samples appeared to have a finer and smoother texture. After acid digestion, the surfaces were eroded due to chemical leaching, and the residues formed were seemed to be amorphous. represents the energy-dispersive X-ray spectroscopy (EDX) analysis of the acid-treated and untreated mobile phone MPCBs performed to analyze the surface elemental composition, and the values are presented in . depicts both the acid-treated and untreated MPCB comprised of a large portion of silicon and copper, which are the main components used in MPCB construction. The results also revealed that the metal ratios were increased after acid digestion, which validates that the acid digestion treatment was effective in solubilizing the metal values present in MPCBs.
Table 4. Elemental composition of MPCB sample (150 µm) determined through energy-dispersive X-ray spectroscopy analysis (EDX)
XRD and FTIR observations of MPCB
XRD observations of MPCB samples are shown in . The significant peaks were observed at 2θ = 30° and 60° before acid digestion, and the peaks formed around 30–38° revealed the presence of gold and silver in both treated and untreated MPCB samples. Some peaks were amortized after acid leaching showing the possibility of disruption of crystalline regions. The transfer of metals from the alloy to the solution during acid digestion could be the primary reason. The peaks formed were similar to the observations made in identical MPCB samples handled by (Kim, Seo, and Roh Citation2018; Zazycki et al. Citation2017).
Figure 4. Mineral phases of MPCB (150 µm) sample observed under X-ray diffraction spectrometer (XRD)
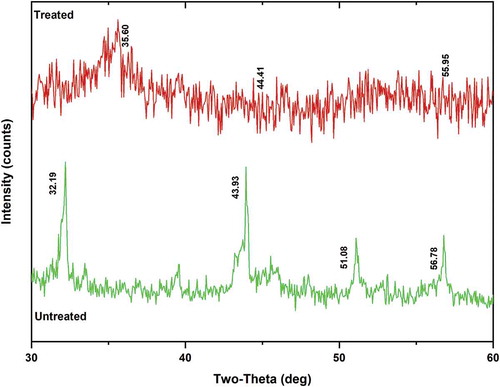
The existence of different kinds of polymers in MPCBs was observed for treated and untreated MPCBs using FTIR, as shown in . The FTIR spectra region of 600–1800 cm−1 represents the spectra of the acid-treated and untreated MPCB sample. The stretching vibrations at 630 cm−1 and 754–798 cm−1 were attributed to aromatic rings C-H. The band in the region between 1111 and 1182 cm−1 was generated by the C-O of esters. The peak emerged at 1234 cm−1 corresponds to the absorption frequency of C-N of amines and amides. The skeleton C = C stretching vibration of the benzene ring was found to have emerged at 1500 cm−1. Finally, the peak at 1728 cm−1 corresponds to C = O of aldehydes and ketones plane bending (Kan et al. Citation2015; Rajagopal et al. Citation2017; Raman et al. Citation2016; Wu and Qiu Citation2015). Previous studies reported that e-waste contained polymers such as fiberglass reinforced plastics (FRP), polyesters (PET, PBT), polyvinyl chloride (PVC), polycarbonates (PC), nylon (polyamide (PA)), acrylonitrile butadiene styrene (ABS), polystyrene (PS), polyethylene (PE), polypropylene (PP), high-impact polystyrene (HIPS), and elastomer (neoprene, SBR, silicone, etc.) (Arshadi, Yaghmaei, and Mousavi Citation2018; Arnold et al. Citation2010; Fontana et al. Citation2019; Kasper Citation2011; Zhan et al. Citation2020). Considering the comparison between the functional groups and peaks, presence of polymers such as poly-butyl acrylate (799 cm−1, 1014 cm−1), ABS (630–754 cm−1), PC (1111–1182 cm−1) and HIPS (1500 cm−1) in MPCBs was affirmed. The results also represented the fact that they are involved in the fabrication of most of the MPCBs (Fontana et al. Citation2019).
In addition to precious metals like gold and silver, MPCBs also possess toxic heavy metals. TCLP tests were carried out, and the metal concentrations in the TCLP extracts of the MPCBs were compared with the United States Environmental Protection Agency (USEPA) standards for hazardous waste. shows the metal concentration contained in the TCLP extract of the collected MPCB sample. Cadmium, mercury, and lead concentrations in the TCLP extract for MPCB samples were higher than the regulatory level set by USEPA for hazardous waste disposal. Hence, the MPCBs must be classified as hazardous waste and should be treated before disposal. The study also stresses the fact that the nonmetallic portions of MPCBs cannot be ignored and must be prioritized in any sustainable recycling process (Guo, Guo, and Xu Citation2009).
Characterization of MPCBs in terms of calorific value
About 60% of heavy fractions and 40% of light fraction from MPCBs were collected after separation by density method using deionized water. Most of the light NMF comprised of polymers, plastics, and glass fibers. The ultimate analysis of the light fraction revealed that it possessed C: 69.34% ± 3.0%, H: 6.2% ± 0.2%, N: 2.6% ± 0.4%, ash-free and dry. depicts the proximate analysis and GCV values of the NMF. It was observed from the results that GCV values were higher for NMF with high volatile and are compared with GCV of other alternative fuels, as shown in .
Table 5. Properties of NMF and various alternative fuels (all in %) (Capablo et al. Citation2009; Chinyama Citation2011; Sharabaroff et al. Citation2017; Zieri and Ismail Citation2018)
Table 6. Gross calorific values of NMF and various alternative fuels (Capablo et al. Citation2009; Chinyama Citation2011; Sharabaroff et al. Citation2017; Zieri and Ismail Citation2018)
The overall elemental composition depicted on an ash-free basis, having a high C-H mass ratio, is almost equivalent to the actual standards for polymers usually used in the MPCBs. These findings also proved the significance of employing mechanical separation of milled MPCB samples. This process also ensured that the NMF has physical characteristics of actual enduring fuel sources. The GCV of this light fraction is 12.34 MJ/kg, which is on par with municipal waste and with a high ash content of 46.54%. The GCV is slightly lower when compared to the mineral coal, which has a GCV of 14 MJ/kg and an ash content of about 59%. One of the critical reasons for not obtaining a representative sample out of NMF is the diversity of shapes and sizes in them. Hence, a highly reliable process is required to achieve efficient material out of MPCBs for energy recovery. However, a trapping system must be installed along the recovery process to arrest the flue gases and hazardous emissions that may occur during gasification and pyrolysis, as suggested by (Font et al. Citation2011; Hall and Williams Citation2007; Molt et al. Citation2009).
Intrinsic and total value of waste mobile phone MPCBs
The concentration of different metals and the total value of waste mobile phone MPCBs were calculated according to the average mass fraction of the MPCBs determined in . The different metals content found in natural mineral ores and the amount of respective metals present in examined MPCBs were shown in . It can be observed that the amount of metals, Ag, Au, Cu, Ni, Pb, and Zn, found in MPCBs is higher than or equal to the content present in respective mineral ores. The estimated intrinsic and total values of the metals are depicted in . The values are calculated with averaged metal concentrations determined in waste mobile phone MPCBs based on the data from London Metal Exchange, 2019, on a three-month average scale. The data revealed the probable generation of revenue through the efficient recovery of metals from waste mobile phone MPCBs. Au constituted for the majority of the intrinsic value of 80.2%, followed by Pd, Cu, Ni, Ag, Pb, Zn, Al, and Fe of intrinsic values 15.21%, 2.63%, 0.98%, 0.73%, 0.1%, 0.03%, and 0.02%, respectively. Au and Pd are the most valuable metals, and their constituents in MPCBs prioritize the recycling of waste MPCBs. Though Cu dominates on a weight basis (202.971 g/kg) in MPCBs, it had occupied a small fraction of the total value of the waste MPCBs. Despite Au and Pd constitute tiny fraction on a weight basis, their large market value 49222.74 USD/kg and 51280.40 USD/kg, respectively, makes them hold 95.41% of the total value of the discarded MPCBs. This finding demonstrates that the total value of the MPCBs majorly depends on the concentration of Au and Pd present in them. From the data, the total value of the MPCBs was estimated to be 49.21 USD/kg. The outcome of the study draws great attention to consider electronic waste as a potential secondary source for various metal values and to develop sustainable processes for their recovery.
Table 7. Comparison of metal content in actual mineral ores and MPCB
Table 8. Estimation of market value of metal concentrations contained in MPCBs
Conclusion
A beneficiation oriented comprehensive characterization of obsolete mobile phone PCBs, which are largely now dismissed as outdated was conducted. The study was performed to determine the elemental composition, polymer content, metal recovery priority, and economic value to provide useful information to facilitate efficient recovery of metals in the different recovery techniques and to assist policymakers involved in material sustainability and future recycling practices.
Metal content makes up to 40% by mass of MPCBs and the rest composed of plastics, ceramics, epoxy, and polymers. Mechanical processing steps before MPCBs treatment increased the exposure of metals resulting in a higher concentration of metals in acid-digested samples. The elemental composition analysis revealed the presence of metals of high quantities Cu (43.19%), Zn (14.25%), Ni (13.44%), Fe (13.9%) and a considerable range of precious metals Ag (0.01%), Au (0.31%), and Pd (0.063%) making them a potential secondary source. Au and Pd are the most valuable metals, and their constituents in MPCBs, respectively, make them hold 95.41%. Cu dominates on a weight basis (202.971 g/kg) in MPCBs with a total value of 2.63% of MPCBs. Since Au has higher economic value than other metal values, its recovery must be prioritized in a recycling process. The total value of the MPCBs was estimated to be 49.21 USD/kg making it an important e-waste where much attention is required. It was also observed that the amount of metals, Ag, Au, Cu, Ni, Pb, and Zn, found in MPCBs is higher than their actual content present in respective mineral ores. This new source may compensate for the escalating global demand for precious metals like Au and Ag and many other base metals. Heterogeneity in elemental composition was observed for different size fractions of acid-digested MPCB samples.
The concentration of base metals was found to be in the order 3 × 3 cm > 2 mm > 1 mm > 500 µm >150 µm. The amount of precious metals like Ag and Au was also found in higher ranges in large-sized MPCB fractions than smaller MPCB sizes, which elucidated the fact to be considered in the pre-treatment process for metal recoveries. TCLP extract analysis revealed the presence of heavy metals like mercury, lead, and cadmium above the USEPA standards. This emphasizes the fact that MPCB is hazardous and must not be disposed of without proper treatment to avoid environmental pollution. FTIR spectra revealed that ABS, HIPs, and PC are the important polymers that exist in the MPCB samples. The ultimate analysis revealed that NMF has a GCV of 12.34 MJ/kg and a volatile content of 42.25%, which can be a potential source of energy that can be recovered through the gasification or pyrolysis process. This study for comprehensive characterization of MPCBs for metal values, polymers, and energy potential will help to develop an analogy between elemental content of waste MPCBs and prioritization of elements for recovery and also to serve as an efficient alternative fuel.
Acknowledgment
The authors thank the China Section of the Air & Waste Management Association for the generous scholarship they received to cover the cost of page charges and make the publication of this paper possible.
Additional information
Notes on contributors
Mohan Annamalai
Christina HoodMohan Annamalai is a Research Scholar at School of Biosciences and Technology, Vellore Institute of Technology, Vellore, Tamil Nadu, India.
Kalaichelvan Gurumurthy
Kalaichelvan Gurumurthy is a Senior Professor at VIT School of Agricultural Innovations and Advanced Learning, Vellore Institute of Technology, Vellore, Tamil Nadu, India.
References
- Annamalai, M., and K. Gurumurthy. 2019. Enhanced bioleaching of copper from circuit boards of computer waste by acidithiobacillus ferrooxidans. Environ. Chem. Lett. 0123456789. doi:https://doi.org/10.1007/s10311-019-00911-y.
- Arnold, J. C., T. Watson, S. Alston, M. Carnie, and C. Glover. 2010. The use of FTIR mapping to assess phase distribution in mixed and recycled WEEE plastics. Polym. Test. 29 (4):459–70. doi:https://doi.org/10.1016/j.polymertesting.2010.02.006.
- Arshadi, M., S. Yaghmaei, and S. M. Mousavi. 2018. Content evaluation of different waste PCBs to enhance basic metals recycling. Resour. Conserv. Recycl. 139 (September):298–306. doi:https://doi.org/10.1016/j.resconrec.2018.08.013.
- Arshadi, M., and S. M. Mousavi. 2015. Multi-Objective optimization of heavy metals bioleaching from discarded mobile phone PCBs: Simultaneous Cu and Ni recovery using acidithiobacillus ferrooxidans. Separation and Purification Technology 147:210–19. doi:https://doi.org/10.1016/j.seppur.2015.04.020.
- ASMT. 1996. ASTM D-2015-Standard test method for gross calorific value of coal and coke by the adiabatic bomb calorimeter. West Conshohocken, PA, USA: ASTM.
- ASTM. 1988a. ASTM D-3174-93 - Standard test method for ash in the analysis sample of coal and coke from coal, Vol. 5. West Conshohocken, PA: Annual Book of ASTM Standards.
- ASTM. 1988b. ASTM D-3175-89a - Standard test method for volatile matter in the analysis sample of coal and coke, Vol. 5. West Conshohocken, PA: Annual Book of ASTM Standards.
- ASTM. 1998. ASTM D 3173-87 - Standard test method for moisture in the analysis sample of coal and coke, Vol. 5. West Conshohocken, PA: Annual Book of ASTM Standards, Section.
- Bakas, I. 2016. Critical metals in discarded electronics: Mapping recycling potentials from selected waste electronics in the Nordic Region. Copenhagen K, Denmark: Nordic Council of Ministers.
- Baldé, C. P., F. Wang, R. Kuehr, and J. Huisman. 2015. The global E-waste monitor 2014. United Nations University, IAS – SCYCLE, Bonn, Germany. Bonn, Germany: United Nations University, IAS – SCYCLE.
- Balde, C. P., V. Forti, V. Gray, R. Kuehr, and P. Stegmann. 2017. The global E-waste monitor 2017. United Nations University. doi:https://doi.org/10.1016/j.proci.2014.05.148.
- Bizzo, W. A., R. A. Figueiredo, and V. F. De Andrade. 2014. Characterization of printed circuit boards for metal and energy recovery after milling and mechanical separation. Materials 7 (6):4555–66. doi:https://doi.org/10.3390/ma7064555.
- Blériot, J., and C. Johnson. 2013. A new dynamic effective business in a circular economy. Isle of Wight, UK: Ellen MacArthur Foundation Publishing.
- Burlakovs, J., Y. Jani, M. Kriipsalu, Z. Vincevica-Gaile, F. Kaczala, G. Celma, R. Ozola, L. Rozina, V. Rudovica, M. Hogland et al. 2018. On the way to ‘zero waste’ management: Recovery potential of elements, including rare earth elements, from fine fraction of waste. J. Clean. Prod. 186:81–90. doi:https://doi.org/10.1016/j.jclepro.2018.03.102.
- Capablo, J., P. A. Jensen, K. H. Pedersen, K. Hjuler, L. Nikolaisen, R. Backman, and F. Frandsen. 2009. Ash properties of alternative biomass. Energy Fuels 23 (4):1965–76.
- Chinyama, M. P. M. 2011. Alternative fuels in cement manufacturing. Altern. Fuel 263–84.
- Cossu, R., and I. D. Williams. 2015. Urban mining: Concepts, terminology, challenges. Waste Manage. 45:1–3. doi:https://doi.org/10.1016/j.wasman.2015.09.040.
- Das, A., A. Vidyadhar, and S. P. Mehrotra. 2009. A novel flowsheet for the recovery of metal values from waste printed circuit boards. Resour. Conserv. Recycl. 53 (8):464–69.
- EPA, US. 1992. Method 1311 Toxicity characteristic leaching procedure (TCLP). In Agency EP, editor. Washington DC, USA1992.
- Font, R., J. Moltó, S. Egea, and J. A. Conesa. 2011. Thermogravimetric kinetic analysis and pollutant evolution during the pyrolysis and combustion of mobile phone case. Chemosphere 85 (3):516–24. doi:https://doi.org/10.1016/j.chemosphere.2011.08.013.
- Fontana, D., M. Pietrantonio, S. Pucciarmati, C. Rao, and F. Forte. 2019. A comprehensive characterization of end-of-life mobile phones for secondary material resources identification. Waste Manage. 99:22–30. doi:https://doi.org/10.1016/j.wasman.2019.08.011.
- Fornalczyk, A., J. Willner, K. Francuz, and J. Cebulski. 2013. E-waste as a source of valuable metals. Arch. Mater. Sci. Eng. 63 (2):87–92.
- Ghosh, B., M. K. Ghosh, P. Parhi, P. S. Mukherjee, and B. K. Mishra. 2015. Waste printed circuit boards recycling: An extensive assessment of current status. J. Clean. Prod. doi:https://doi.org/10.1016/j.jclepro.2015.02.024.
- Guo, J., J. Guo, and Z. Xu. 2009. Recycling of non-metallic fractions from waste printed circuit boards: A review. J. Hazard. Mater. 168 (2–3):567–90. doi:https://doi.org/10.1016/j.jhazmat.2009.02.104.
- Gurumurthy, K., and M. Annamalai. 2019. Microbiological leaching of metals and its recovery from waste electrical and electronic equipment: A review. World Rev. Sci. Technol. Sustainable Dev. 15 (1):1. doi:https://doi.org/10.1504/wrstsd.2019.10019986.
- Hall, W. J., and P. T. Williams. 2007. Separation and recovery of materials from scrap printed circuit boards. Resour. Conserv. Recycl. 51:691–709. doi:https://doi.org/10.1016/j.resconrec.2006.11.010.
- Holgersson, S., B. M. Steenari, M. Björkman, and K. Cullbrand. 2018. Analysis of the metal content of small-size Waste Electric and Electronic Equipment (WEEE) printed circuit boards—Part 1: Internet routers, mobile phones and smartphones. Resour. Conserv. Recycl. 133:300–08. doi:https://doi.org/10.1016/j.resconrec.2017.02.011.
- Ilyas, S., and J.-C. Lee. 2014. Bioleaching of metals from electronic scrap in a stirred tank reactor. Hydrometallurgy 149:50–62. doi:https://doi.org/10.1016/j.hydromet.2014.07.004.
- Işıldar, A., E. R. Rene, E. D. van Hullebusch, and P. N. L. Lens. 2018. Electronic waste as a secondary source of critical metals: Management and recovery technologies. Resour. Conserv. Recycl. 135 (July):296–312. doi:https://doi.org/10.1016/j.resconrec.2017.07.031.
- ITU. 2016. International telecommunication union (2016a). http://www.itu.int/en/ITU-D/Statistics/Documents/publications/misr2016/MISR2016-w4.pdf.
- Jakub, S. 2014. Metals content in printed circuit board waste. Pol. J. Environ. Stud. 23 (6):2365–69.
- Jianfeng, B., W. Jingwei, X. Jinqiu, Z. Mingyuan, G. Jie, and Z. Chenglong. 2009. Microbiological recovering of metals from printed circuit boards by acidithiobacillus ferrooxidans. 2009 IEEE International Symposium on Sustainable Systems and Technology, 1–6. Phoenix, AZ, doi: https://doi.org/10.1109/ISSST.2009.5156716.
- Kan, Y., Q. Yue, J. Kong, B. Gao, and Q. Li. 2015. The application of activated carbon produced from waste Printed Circuit Boards (PCBs) by H 3 PO 4 and steam activation for the removal of malachite green. Chem. Eng. J. 260:541–49. doi:https://doi.org/10.1016/j.cej.2014.09.047.
- Kasper, A. C. 2011. Characterization and recovery of polymers from mobile phone scrap. Waste Manage. Res. 29:714–726. doi:https://doi.org/10.1177/0734242X10391528.
- Kasper, A. C., G. B. T. Berselli, B. D. Freitas, J. A. S. Tenório, A. M. Bernardes, and H. M. Veit. 2011. Printed wiring boards for mobile phones: Characterization and recycling of copper. Waste Manage. 31 (12):2536–45. doi:https://doi.org/10.1016/j.wasman.2011.08.013.
- Khaliq, A., M. A. Rhamdhani, G. Brooks, and S. Masood. 2014. Metal extraction processes for electronic waste and existing industrial routes: A review and australian perspective. Resources 3:152–79. doi:https://doi.org/10.3390/resources3010152.
- Kim, Y., H. Seo, and Y. Roh. 2018. Metal recovery from the mobile phone waste by chemical and biological treatments. Minerals 8 (1). doi: https://doi.org/10.3390/min8010008.
- Kirchherr, J., D. Reike, and M. Hekkert. 2017. Conceptualizing the circular economy: An analysis of 114 definitions. Resour. Conserv. Recycl. 127:221–32.
- Krook, J., A. Carlsson, M. Eklund, P. Frändegård, and N. Svensson. 2011. Urban mining: Hibernating copper stocks in local power grids. J. Clean. Prod. 19 (9–10):1052–56. doi:https://doi.org/10.1016/j.jclepro.2011.01.015.
- Kumar, A., M. E. Holuszko, and T. Janke. 2018. Characterization of the non-metal fraction of the processed waste printed circuit boards. Waste Manage. 75:94–102. doi:https://doi.org/10.1016/j.wasman.2018.02.010.
- Maragkos, K. G., J. N. Hahladakis, and E. Gidarakos. 2013. Qualitative and quantitative determination of heavy metals in waste cellular phones. Waste Manage. 33 (9):1882–89. doi:https://doi.org/10.1016/j.wasman.2013.05.016.
- Molt, J., R. Font, A. Galvez, and J. A. Conesa. 2009. Pyrolysis and combustion of electronic wastes. J. Anal. Appl. Pyrolysis 84 (1):68–78. doi:https://doi.org/10.1016/j.jaap.2008.10.023.
- Nguyen, R. T., L. A. Diaz, D. Devin Imholte, and T. E. Lister. 2017. Economic assessment for recycling critical metals from hard disk drives using a comprehensive recovery process. Jom 69 (9):1546–52. doi:https://doi.org/10.1007/s11837-017-2399-2.
- Nnorom, I. C., and O. Osibanjo. 2009. Toxicity characterization of waste mobile phone plastics. J. Hazard. Mater. 161 (1):183–88. doi:https://doi.org/10.1016/j.jhazmat.2008.03.067.
- Oguchi, M., H. Sakanakura, and A. Terazono. 2013. Toxic metals in WEEE: Characterization and substance flow analysis in waste treatment processes. Sci. Total Environ. 463–464:1124–32. doi:https://doi.org/10.1016/j.scitotenv.2012.07.078.
- Oguchi, M., S. Murakami, H. Sakanakura, A. Kida, and T. Kameya. 2011. A preliminary categorization of end-of-life electrical and electronic equipment as secondary metal resources. Waste Manage. 31 (9–10):2150–60. doi:https://doi.org/10.1016/j.wasman.2011.05.009.
- Oliveira, P. C., M. Cabral, C. A. Nogueira, and F. Margarido. 2010. Printed circuit boards recycling: Characterization of granulometric fractions from shredding process. Mater. Sci. Forum 636–637:1434–39. doi:https://doi.org/10.4028/www.scientific.net/MSF.636-637.1434.
- Owens, B. 2013. Extreme Prospects. Nature 495:S4–6.
- Paiano, A., G. Lagioia, and A. Cataldo. 2013. A critical analysis of the sustainability of mobile phone use. Resour. Conserv. Recycl. 73:162–71. doi:https://doi.org/10.1016/j.resconrec.2013.02.008.
- Pant, D., D. Joshi, M. K. Upreti, and R. K. Kotnala. 2012. Chemical and biological extraction of metals present in E waste: A hybrid technology. Waste Manage. 32 (5):979–90. doi:https://doi.org/10.1016/j.wasman.2011.12.002.
- Pei, D., J. Xu, Q. Zhuang, H.-F. Tse, and M. A. Esteban. 2010. Induced pluripotent stem cell technology in regenerative medicine and biology. Adv. Biochem. Eng. Biotechnol. 123 (July 2015):127–41. doi:https://doi.org/10.1007/10.
- Priya, A., and S. Hait. 2018. Comprehensive characterization of printed circuit boards of various end-of-life electrical and electronic equipment for beneficiation investigation. Waste Manage. 75:103–23. doi:https://doi.org/10.1016/j.wasman.2018.02.014.
- Rajagopal, R. R., R. Rajarao, S. T. Cholake, and V. Sahajwalla. 2017. Sustainable composite panels from non-metallic waste printed circuit boards and automotive plastics. J. Clean. Prod. 144:470–81. doi:https://doi.org/10.1016/j.jclepro.2016.12.139.
- Raman, R., L. S. Aravinda, R. Rajarao, B. Ramachandra, and V. Sahajwalla. 2016. Electrochimica acta activated carbon derived from non-metallic printed circuit board waste for supercapacitor application. Electrochim. Acta 211:488–98. doi:https://doi.org/10.1016/j.electacta.2016.06.077.
- Reuter, M., and A. van Schaik. 2016. Gold – A key enabler of a circular economy. Gold ore processing. Elsevier B.V. doi:https://doi.org/10.1016/b978-0-444-63658-4.00053-0.
- Robinson, B. H. 2009. E-waste: An assessment of global production and environmental impacts. Sci. Total Environ. 408 (2):183–91. doi:https://doi.org/10.1016/j.scitotenv.2009.09.044.
- Rowley, J. 2006. Mobile phone lifecycles. http://gsma.com/publicpolicy/wp-content/uploads/2012/04/environmobilelifecycles.pdf.
- Schuelp, M., C. Hagelueken, R. Kuehr, F. Magalini, C. Maurer, C. Meskers, E. Mueller, and F. Wang. 2009. Recycling from E-waste to resources. United Nations Environment Programme & United Nations University, 120. http://www.unep.org/pdf/Recycling_From_e-waste_to_resources.pdf.
- Sharabaroff, A., D. Bernard, D. Lemarchand, N. Tetreault, C. Thevenet, and A. de Souance. 2017. Increasing the use of alternative fuels at cement plants: International best practice. Washington, DC, USA: World Bank Group.
- Sohaili, J., S. K. Muniyandi, and S. S. Mohamad. 2012. A review on printed circuit boards waste recycling technologies and reuse of recovered nonmetallic materials. Int. J. Sci. Eng. Res. 2 (3):138–44.
- Szamałek, K., and K. Galos. 2016. Metals in Spent Mobile Phones (SMP) – A new challenge for mineral resources management introduction. Gospodarka Surowcami Mineralnymi 32 (4):45–58. doi:https://doi.org/10.1515/gospo-2016-0037.
- Tanskanen, P. 2012. Electronics waste : Recycling of mobile phones, Post-consumer waste recycling and optimal production, Enri Damanhuri, IntechOpen. doi:https://doi.org/10.5772/31530. https://www.intechopen.com/books/post-consumer-waste-recycling-and-optimal-production/electronics-waste-recycling-of-mobile-phones
- Vats, M. C., and S. K. Singh. 2015. Assessment of gold and silver in assorted mobile phone Printed Circuit Boards (PCBs): Original article. Waste Manage. 45 (October). doi: https://doi.org/10.1016/j.wasman.2015.06.002.
- Wu, W., and K. Qiu. 2015. Journal of analytical and applied pyrolysis vacuum co-pyrolysis of Chinese fir sawdust and waste printed circuit boards. Part II: Influence of heating conditions. J. Anal. Appl. Pyrolysis 111:216–23. doi:https://doi.org/10.1016/j.jaap.2014.11.006.
- Xavier, L. H., E. C. Giese, A. C. Ribeiro-Duthie, and F. A. F. Lins. 2019. Sustainability and the circular economy: A theoretical approach focused on e-waste urban mining. Resour. Policy 101467. October 2017. doi:https://doi.org/10.1016/j.resourpol.2019.101467.
- Yamane, L. H., V. T. de Moraes, D. C. R. Espinosa, and J. A. S. Tenório. 2011. Recycling of WEEE: Characterization of spent printed circuit boards from mobile phones and computers. Waste Manage. 31 (12):2553–58. doi:https://doi.org/10.1016/j.wasman.2011.07.006.
- Yoo, J.-M., J. Jeong, K. Yoo, J.-C. Lee, and W. Kim. 2009. Enrichment of the metallic components from waste printed circuit boards by a mechanical separation process using a stamp mill. Waste Manage. 29 (3):1132–37.
- Zazycki, M. A., E. H. Tanabe, D. A. Bertuol, and G. L. Dotto. 2017. Adsorption of valuable metals from leachates of mobile phone wastes using biopolymers and activated carbon. J. Environ. Manage. 188:18–25. doi:https://doi.org/10.1016/j.jenvman.2016.11.078.
- Zhan, L., X. Zhao, Z. Ahmad, and Z. Xu. 2020. Chemosphere leaching behavior of Sb and Br from E-waste Fl ame retardant plastics. Chemosphere 245:125684. doi:https://doi.org/10.1016/j.chemosphere.2019.125684.
- Zhu, N., Y. Xiang, T. Zhang, P. Wu, Z. Dang, P. Li, and J. Wu. 2011. Bioleaching of metal concentrates of waste printed circuit boards by mixed culture of acidophilic bacteria. J. Hazard. Mater. 192 (2):614–19. doi:https://doi.org/10.1016/j.jhazmat.2011.05.062.
- Zieri, W., and I. Ismail. 2018. Alternative fuels from waste products in cement industry. In Handbook of ecomaterials, ed. L. Martínez, O. Kharissova, B. Kharisov, 1–24. Springer, Cham. doi:https://doi.org/10.1007/978-3-319-48281-1_142-1