ABSTRACT
The real-time measurement of atmospheric ammonia at municipal solid waste (MSW) landfills and adjacent areas is necessary for landfill management and the health of nearby residence. Continuous, fast, and real-time monitoring of landfill odor gases is a challenge, especially for ammonia. To our knowledge, this was the first study for the characteristics and seasonal variabilities of atmospheric ammonia at a whole landfill using a Mobile White cell Differential Optical Absorption Spectroscopy (MW-DOAS) system, which also simultaneously offers high sensitivity and fast response. Results show that atmospheric ammonia levels at various landfill areas were significantly dependent on the characteristics of areas, such as municipal solid waste-related areas, leachate-related areas, sludge-related areas, and fly ash-related area, the atmospheric ammonia peak or average level at the active leachate pool of the active MSW site was the highest among all areas of the whole landfill, and the ammonia concentrations at the closed MSW landfill sites were low and dependent on the ages. Moreover, it was found that the seasonal variabilities of ammonia concentrations at most of those areas were significantly dependent on the ambient temperature, and ambient temperature variation caused the atmospheric ammonia level at the active leachate pool and active MSW landfill site in the summer survey to raise 3.5 times and 5.58 times than in the winter survey, respectively.
Implications: Continuous, fast, and real-time monitoring ambient ammonia at or nearby a landfill is critical for landfill operators and local EPAs. This study demonstrates that the mobile White cell Differential Optical Absorption Spectroscopy (MW-DOAS) system is an effective tool for real-time monitoring ambient ammonia of a whole landfill. The results in this article provided a guideline to the characteristics and seasonal changes of ambient ammonia at various types of areas of a whole landfill as well as the impact of age to ambient ammonia at the closed landfill areas.
Introduction
Landfill odor gas emissions have been a growing concern for municipal solid waste (MSW) operators and nearby residents (Ding et al. Citation2012; Sarkar, Hobbs, and Longhurst Citation2003; Tian et al. Citation2013). Ammonia and hydrogen sulfide were identified as the two predominate odor compounds emitted from MSW landfills (Ding et al. Citation2012; Fang et al. Citation2015). Atmospheric ammonia plays an important role to form ammonium-containing aerosol through reacting with SO2 and nitrogen oxides (NOx), which is the major fraction of PM2.5 aerosols (Behera and Sharma Citation2010). Furthermore, it is a challenge to effectively respond to odor emissions immediately due to the complexity of odor sources, the low threshold of olfactory sensation, and the lack of proper analytical techniques (Fang et al. Citation2015).
Many researchers have characterized the VOCs (Volatile Organic Compounds) gases in landfill in the last several decades (Chiriac et al. Citation2011; Davoli et al. Citation2003; de Sá Borba et al. Citation2017; Zou et al. Citation2003); however, few studies have been conducted for inorganic odorous gas in MSW landfills, such as ammonia. In particular, the environmental behavior of inorganic odorous gas has not been investigated properly (Ding et al. Citation2012). In current landfill studies, sampling points were very limited for monitoring ammonia at MSW landfills and cannot cover all areas of MSW landfills because of the limitations of current sampling and analytics methods. For example, air samples were collected at six sampling points, including dumping area, leachate treatment plant, administration area, and residence area (Ding et al. Citation2012). Only nine sampling points were selected in a larger MSW landfill and divided into three types as follows: MSW-related area, leachate-related area, and sludge-related area (Fang et al. Citation2012).
It is necessary to understand and monitor the seasonal variations of atmospheric ammonia at various areas of a landfill but despite this almost no research output investigated the relationship between the primary odor concentrations and the seasonal variation in landfill sites (Ding et al. Citation2012). As a result, the impact of seasonal variation to primary inorganic odorous gases in MSW landfill, such as ammonia, is still not clear. Recently, the seasonal variations of odorous compound gas was investigated in a MSW disposal plant, and it was found that the concentration of nitrogenous compound in summer is 7.68 times higher than in winter and 3.1 times higher than in spring (Wu et al. Citation2018). Although the seasonal variations of ammonia were also observed at various sampling points of a landfill using the classic spectrophotometric method, it was reported that the seasonal variation of ammonia concentration was not obvious (Ding et al. Citation2012).
The two main challenges for ammonia detection techniques are that ammonia simultaneously exists in gaseous, particulate, and liquid phases (Dammers et al. Citation2017; Ding et al. Citation2012; Warneck Citation1988), and ammonia is a very “sticky” gas that interacts with materials’ surfaces and causes slow inlet response time (D’Amato et al. Citation2020; Yokelson et al. Citation2003). The widely adopted sampling method for ammonia is still the bulk denuder technique, which causes a low time-resolution and requires further wet-chemical analysis (Keuken et al. Citation1998). Various spectroscopic techniques have been developed to measure common atmospheric ammonia, including chemiluminescence (Breitenbach and Shelef Citation1973), cavity ring down spectrometer (CRDS), quantum cascade laser absorption spectroscopy (QCLAS), open-path differential optical absorption spectroscopy (OP-DOAS) (Wang et al. Citation2015), open-path Fourier transform infrared spectrometer (OP-FTIR) (Galle et al. Citation2000), and portable FTIR. In addition, Chemical Ionization Mass Spectrometer (CIMS), ion mobility spectrometer (IMS), and photo-acoustic spectroscopy (PAS) were adopted to monitor atmospheric ammonia by some researchers. von Bobrutzki et al. evaluated various atmospheric ammonia measurement techniques, including wet chemistry, CRDS, CIMS, IMS, and QCLAS, and pointed out that the response of wet chemistry technique was very slow, the CIMS or QCLAS instrument needs to be calibrated or zeroed every hour in the field, and the IMS instrument was only suitable to low concentration application (von Bobrutzki et al. Citation2010). The response of PAS instrument was significantly delayed (Rom and Zhang Citation2010). The OP-DOAS or OP-FTIR technique is only suitable for static measurement, and the portable FTIR instrument cannot detect low concentration atmospheric ammonia. shows current atmospheric ammonia detection techniques. However, in current landfill atmospheric ammonia studies, only the combination of passive sampler and classical spectrophotometric method or simple colorimetric tubes were adopted to monitor atmospheric ammonia. Furthermore, most of those analytic techniques were adopted only for atmospheric ammonia static or spot monitoring applications.
Table 1. Current atmospheric ammonia studies
A large landfill might occupy more than 10 km2 and needs an effective real-time monitoring technique. For monitoring atmospheric ammonia of a large landfill, the potential solution should have the capabilities of fast response, high sensitivity, and mobile application. The goals of this study were to (1) investigate the characteristics of atmospheric ammonia at various areas of a whole large landfill, rather than limited sampling points; (2) evaluate the relationship between atmospheric ammonia’s seasonal variabilities and ambient temperature; (3) identify the relationship between the age of closed MSW landfill site and atmospheric ammonia.
Experiments
Mobile sampling and measurement in Laogang landfill
Shanghai Laogang MSW Landfill is the largest MSW landfill in China (Feng et al. Citation2017), which occupies more than 15 km2, is located at the south of Pudong international airport and approximately 60 km from Shanghai city center. Laogang landfill consists of four MSW landfill sites, a hybrid landfill site, two leachate treatment plants, and an energy recycle center. The active MSW IV site within Laogang landfill is the largest landfill site in China in terms of capacity and daily intake, and its overall capacity is 80 million cubic meters. It disposes of 7,600 tons MSW daily and accepts residues from external incineration plants and integrated waste treatment plants (Fang et al. Citation2012). The leachate treatment plants accept leachate from the Laogang MSW landfill I, II, III, and IV sites as well as the hybrid landfill site. The hybrid landfill site and energy recycle center are located at the southeast corner of Laogang landfill.
Two mobile measurements were implemented over the whole Laogang landfill in the winter and summer of 2018. The winter survey was implemented on 08/02/2018 and the summer survey was implemented on 30/07/2018. The whole mobile measurement route in Laogao landfill was 23.8 km within 70 minutes and covered most of the areas in Laogang landfill, including MSW landfill phase I site (MSW I), MSW landfill phase II site (MSW II), MSW landfill phase III site (MSW III), MSW landfill phase IV site (MSW IV), leachate treatment plant A (LTA), leachate treatment plant B (LTB), leachate pool A (LPA), leachate pool B (LPB), leachate pool C (LPC), sludge leachate pool A (SLA), sludge tipping site A (STA), hybrid MSW area (HMSW), and fly ash area (FA). The LTA is at the north of MSW IV, and the LTB is at the northeast of MSW IV. The LPA is related to the leachate treatment of the LTA, the LPB is to collect leachate of active MSW IV, and the LPC is to collect leachate in the closed MSW I. The average movement speed of the vehicle was 20.4 km per hour. Data mapping software combined the measurement results and the corresponding location information from global positioning system (GPS) to generate the geographic concentration map of atmospheric ammonia.
The wind direction affects the spread of landfill gas emission. The Pudong airport is located at 6.5 km north of Laogang landfill. The wind data of Pudong airport was used to select an effective route for screening landfill atmospheric ammonia. In the winter survey, the wind direction was from the north, and the wind speed was 4–5 meters per second between 5:54 am to 7:04 am (UTC time), the atmosphere pressure was 1,024 mbar, and the ambient temperature was 6°C. The shapes of MSW-related, leachate-related, or sludge-related areas, are rectangles, and the west or south boundaries of those areas are parallel. Since the wind comes from the north, the west or south boundaries of MSW-related, leachate-related, or sludge-related areas were chosen to evaluate ammonia emission and air quality. The angle between the west boundary of any area and the direction of wind was about 30°, and the angle between the south boundary of any area and the direction of wind was about 60°. In other words, the angle between the practical measurement route and wind direction was 30° or 60°. In the summer survey, the angle is the same since the wind direction or practical measurement route was the same as in the winter survey.
Instrument configuration
The fast screening survey solution was implemented with a Mobile White cell Differential Optical Absorption Spectroscopy (MW-DOAS) system (DV3000, DUVAS Technologies), which was installed in a vehicle. A polytetrafluoroethylene (PTFE) sampling tube with a 2-inch diameter was adopted to collect ambient air samples in real time. The volume of internal optical sampling cell of the DV3000 is two liters, and the sampling air flow was two liters per second. Measurements were taken each second, and no concentration averaging was implemented. In other words, the air sampling, spectrum analysis and mobile measurement results respond to ambient air in real time. The temperature, air pressure, and humidity sensors were installed inside the optical cell. The GPS sensor was integrated with that analytical instrument to provide the real-time global position location information. shows a schematic of the MW-DOAS system.
Spectral analysis
The spectrum signals of ammonia (NH3) in ambient air was analyzed in real time using the principle of DOAS (Platt and Stutz Citation2008) since ammonia has absorption peaks within the ultraviolet wavelength range. The solving wavelength range of 200–300 nm was chosen to solve raw spectra. The differential absorption signature of raw spectrum was extracted, and the solving result was generated each second.
Results
) shows the internal structure of Laogang landfill and the wind directions in the winter and summer surveys, and ) shows the screening route and the ambient ammonia concentrations at Laogang landfill in the winter survey on a Google satellite map. Over the whole screening route, ambient ammonia levels at each screening position in the winter survey were represented by various colors. The hot spots of ambient ammonia were clearly showed in the Google satellite map.
Figure 2. The survey of atmospheric ammonia at Laogang landfill: (a) the internal areas map of Laogang landfill and wind directions in the winter and summer survey and (b) the atmospheric ammonia concentrations at Laogang landfill in the winter survey (Map data: Google, DigitalGlobe)
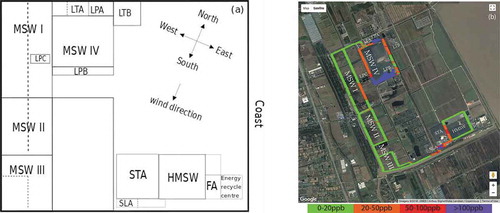
Atmospheric ammonia at MSW landfill areas
To evaluate atmospheric ammonia of MSW areas with similar conditions and avoid the impact of ammonia emissions from leachate-related areas, measurements at the west boundaries of four MSW landfill sites were selected. The mobile measurement routes covered the west boundary of the MSW I, MSW II, MSW III, and MSW IV. The areas of MSW I, MSW II, and MSW III sites are 1.60 km2, 1.00 km2, and 0.838 km2, respectively, and the total length from the north boundary of MSW I to the south boundary of MSW III is about 4 km (Su Citation2005). The length and width of MSW IV are 1,055 m and 765 m (Su Citation2005). The averages of atmospheric ammonia concentrations at various MSW landfill sites, MSW I, MSW II, MSW III, and MSW IV, in the winter survey are showed in .
Table 2. The averages of atmospheric ammonia levels at various areas in the winter or summer survey
Atmospheric ammonia at the leachate-related areas
,,,–) are the raw real-time mobile measurement results of five leachate-related areas in the winter survey, where each x-axis represents various raw sampling positions of those leachate-related areas along the vehicle’s movement routes. Each raw sampling position is corresponding to a measurement result each second. ) and show the atmospheric ammonia levels along the south boundary of LPA and LPB, and the related peak concentrations were 441 ppb and 1,138 ppb, respectively. ) shows the atmospheric ammonia levels along the southeast boundary of the LPC, and its ammonia peak concentration was 370 ppb. ) and show the atmospheric ammonia levels along the west boundary of LTA and LTB, and the related peak concentrations were 78.89 ppb and 52.06 ppb, respectively. also shows the averages of atmospheric ammonia concentrations at the various leachate-related areas, LPA, LPB, LPC, LTA, and LTB, in the winter survey.
Atmospheric ammonia at the hybrid landfill site
The hybrid landfill phase I site consists of three independent areas from east to west: fly ash area, hybrid MSW area, and sludge area (Tang Citation2016; Zhou, Lan, and Zhao Citation2015). Each area has four units from south to north (Tang Citation2016), including tipping subareas and leachate pools. The FA is for tipping the processed fly ash which is generated from the energy recycle center after the waste incineration process, and the sludge area is for tipping preprocessed sludge from external sites (Tang Citation2016). The SLA and STA are located in the sludge area.
(–) show the raw real-time mobile measurement results at the sludge, hybrid MSW and fly ash areas in the winter survey and each x-axis represents various sampling positions of those three areas along the vehicle’s movement routes. and show the atmospheric ammonia concentrations along the south boundaries of SLA and STA, and the peak concentrations were 240 ppb and 121 ppb, respectively. ) shows the atmospheric ammonia concentrations along the west boundary of HMSW, and the peak concentration was 68 ppb. ) shows the atmospheric ammonia along the west boundary of FA and its peak concentration was 19.19 ppb. also shows the average of atmospheric ammonia levels at the areas of the hybrid landfill site, SLA, STA, HMSW, and FA, in the winter survey.
Discussion
Impact of ages and status of MSW-related areas
The winter survey results show that the ammonia concentrations at various MSW landfill sites were related to their ages and status. The west boundaries of all four MSW landfill sites are parallel and toward to the northwest and the angles between the wind direction and the west boundaries of all four MSW landfill sites were the same. The averages of atmospheric ammonia concentrations at the closed MSW I, II, and III sites were 6.19 ppb, 7.68 ppb, 14.26 ppb, and 14.11 ppb in the winter survey, respectively. The MSW I, II, and III sites had been in operation since 1991, 1996, and 2000 (Su Citation2005) and were gradually closed from 2009 to 2013 and covered by high density Polyethylene (HDPE) liner and soil. The average atmospheric ammonia level at the active MSW landfill area, MSW IV, was 34 ppb. The MSW IV area has been operated since 2005 and is still active now, and most of it is also covered by the HDPE liner, except its operating block.
) or shows the comparison of the atmospheric ammonia concentrations at MSW areas in the winter or summer survey. The size of raw sampling and measurement data of each MSW area was different because the lengths of those four MSW areas are different. To compare the atmospheric ammonia concentration trends of various MSW areas at the same time, each x-axis represents 20 sampling positions of an MSW area, which were uniformly selected from raw measurement results of that MSW area. shows that the trend of atmospheric ammonia concentrations at the oldest and closed MSW landfill site, MSW I, was lower than those of the young and closed MSW II and MSW III, and the closed MSW II or III areas’ atmospheric ammonia trend was lower than that of the active MSW IV area. ) clearly shows that there is a direct correlation between the atmospheric ammonia concentrations at various MSW areas and their ages or status.
Figure 4. Impact of MSW ages and status: (a) comparison of the atmospheric ammonia concentrations at MSW areas in the winter survey, and (b) comparison of the atmospheric ammonia concentrations at MSW areas in the summer survey (the x axis represents 20 uniformly chosen sampling locations at various MSW-related areas)
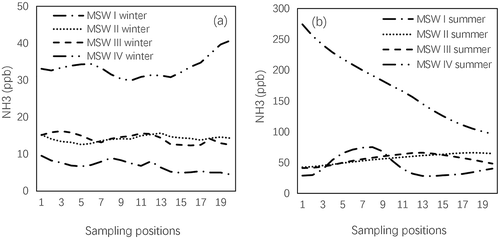
) also shows the relations among the atmospheric ammonia concentration trends at various MSW areas in the summer survey are similar to those in the winter survey, with the exception of MSW I where the summer ammonia concentration readings at the MSW I were disturbed since a part of MSW I was uncovered by the HDPE liner and soil. Parker et al. divided wastes into young, middle-aged, and old refuse according to aerobic condition, and anaerobic condition, and mentioned that the landfill gas emission of a closed MSW landfill is related to the age of waste and the stage of waster degradation (Parker, Dottridge, and Kelly Citation2002). This is consistent with the finding in this study.
The atmospheric ammonia concentrations along the screening route of the closed MSW landfill areas, MSW I, MSW II, and MSW III, were relatively stable in the winter survey, and there was no obvious hot spot of atmospheric ammonia concentrations along those closed MSW landfill areas in the winter survey. Wang et al. reported that atmospheric ammonia concentrations in Shanghai urban, rural, and industry areas were typically 6.2 ppb, 12.4 ppb, and 17.6 ppb, respectively (Wang et al. Citation2015). The averages of ammonia concentrations at the closed MSW I, II, and III areas in this study were close to their research finding. In other words, the winter atmospheric ammonia levels at the closed MSW landfill sites were similar to other urban or rural areas in Shanghai, and those closed MSW landfill sites have been controlled well.
Impact of the status of leachate-related areas
In the winter survey, the identified hot spots of atmospheric ammonia concentrations show that the atmospheric ammonia levels at various leachate-related areas correspond to the characteristics of those areas. The LPB, the active leachate pool within the active MSW landfill area, caused the hot spot of atmospheric ammonia level at the LPB to be 1,138 ppb. The LPB was used to collect and store leachate generated from the active MSW landfill site, MSW IV. This level was the highest among all areas of the whole Laogang landfill in the winter survey and was higher compared with the leachate pools (LPA, LTA, LTB, and LPC) of leachate treatment plants or the closed landfill site.
Furthermore, the winter survey shows that the leachate pool in the closed MSW landfill site also emitted a higher atmospheric ammonia concentration compared with the leachate pool of the leachate treatment plant. Leachate collected in the leachate pools of the closed or active MSW landfills was drained into the leachate treatment plant and its leachate pool, such as LTA and LPA. The LPC is used to collect leachate generated from the closed MSW I, and its atmospheric ammonia peak concentration was 441 ppb. The LPA is for the leachate treatment of LTA, and its ammonia peak concentration was 370 ppb. The atmospheric ammonia peak levels at the leachate treatment plant of LTA and LTB were 78.89 ppb and 52.06 ppb in the winter survey, respectively, which was the lowest in all leachate-related areas demonstrating that it is effectively controlled. This study shows that the anaerobic condition at the closed MSW landfill can reduce the ammonia of collected leachate, and the leachate treatment plant can further reduce ammonia after the leachate treatment process.
Impact of the characteristics of hybrid areas
The hybrid areas consist of a sludge area, a MSW area, and a fly ash area. In the winter survey, it was found that the atmospheric ammonia concentrations at those areas were also related to the characteristics of those areas. The sludge was transported to the sludge discharge area first and was processed at the sludge disposal workplace area to dry it by adding chemical drying agent; then, the sludge was finally put into the sludge tipping site (Fang et al. Citation2012). The atmospheric ammonia concentration at the SLA was about two times higher compared with the STA. The potential reason could be that the SLA is related to the sludge discharge area and generation of leachate, but the STA is related to sludge tipping area and covered with the HDPE liner.
The atmospheric ammonia concentration at the fly ash area was very low which means that ammonia was significantly reduced after the waste incineration process at the nearby energy recycle center. In addition, the HWSW area was also active. Although there was a hot spot of atmospheric ammonia concentrations at the HMSW’s west boundary (HMSW West) of 68 ppb, the average was 35.94 ppb, similar to the average of atmospheric ammonia concentrations at the active MSW IV.
Seasonal variations of atmospheric ammonia at MSW areas
This is the first investigation of the seasonal variability of atmospheric ammonia concentrations at all various areas over a whole large MSW landfill. The summer mobile measurement was implemented on July 30, 2018. The ambient temperature was 35°C, the atmospheric pressure was 1,003 mbar, the wind direction was the north, and the wind speed was 6 mph. The summer survey was implemented with the same instrument, the similar atmospheric pressure and movement speed, the same wind direction, and sampling locations as the winter survey. The ambient temperature difference between the winter and summer mobile measurement was 29°C.
–) show the season variation of atmospheric ammonia concentrations of MSW I, MSW II, MSW III, or MSW IV, and each x-axis represents the corresponding 20 sampling positions, which were uniformly chosen from raw sampling positions of each MSW area in the winter or summer survey. The raw sampling and measurement data’s size of each MSW area in the winter and summer survey were not exactly the same because the mobile measurement speed was slightly various. Those uniformly chosen 20 sampling positions of each MSW area can help to demonstrate the seasonal variability of atmospheric ammonia concentration trends of MSW areas. ) shows the comparison of averages of atmospheric ammonia concentrations at those MSW areas in the winter and summery survey.
Figure 5. Seasonal variations of atmospheric ammonia concentrations at (a) MSW I, (b) MSW II, (c) MSW III, and (d) MSW IV (the x axis represents 20 uniformly chosen sampling locations at various MSW-related areas); (e) seasonal variation of averages of atmospheric ammonia concentrations at various MSW areas
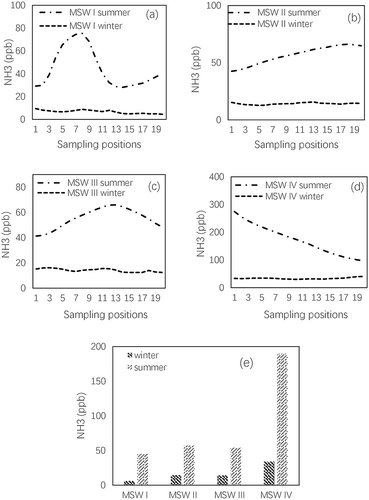
It was found that the averages of summer atmospheric ammonia levels at the closed MSW landfill sites and the active MSW landfill site, MSW I, MSW II, MSW III, and MSW IV, were 7.38, 3.99, 3.82, and 5.58 times higher than in winter, respectively. Those results indicate that the seasonal variability of atmospheric ammonia concentrations at the active MSW landfill site is more dependent on ambient temperature than the closed MSW landfill sites. It was also found that the absolute seasonal variability of ammonia emission concentration at the active MSW site, MSW IV, was more significant than the closed MSW sites, MSW I, MSW II, and MSW III.
Seasonal variations of atmospheric ammonia at leachate-related and hybird areas
–) show that the seasonal variations of ammonia concentrations at the LPA, LPB, the hybrid MSW south (HMSWS), and FA area, and each x-axis represents 20 sampling positions, which were uniformly chosen from raw sampling positions. It was clear that the atmospheric ammonia concentration at all various sites of Laogang landfill in the summer survey is higher than in the winter survey. Especially the ammonia peak concentration at the active leachate pool of the active MSW site, LPB, was 3,990 ppb in the summer survey and 3.5 times higher than in the winter survey. However, the season variability of leachate treatment pool in the leachate treatment plant or the leachate storage pool of closed MSW site is relative smaller, such as LPA. The ammonia peak levels at the LPA in the summer survey were 1.25 times higher than in the winter survey. No obvious and independent hot spot of atmospheric ammonia concentrations at the LPC, LTA or LTB was identified in the summer survey. The potential reasons might be that the LPC is very close to the LPB, the LTA or LTB is very close to the LPA, and the atmospheric ammonia of the LPB or LPA spreads to the LPC, LTA, or LTB.
Figure 6. Seasonal variations of ambient ammonia concentration at (a) LPA, (b) LPB, (c) HMSWS, and (d) FA (the x axis represents 20 uniformly chosen sampling locations at various leachate-related or hybrid areas). Seasonal variations of ambient ammonia peak concentrations at (e) LPA and LPB, and seasonal variations of averages of ambient ammonia concentrations at (f) HMSW and FA
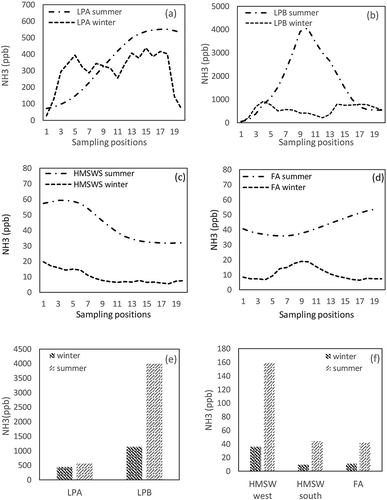
) shows the seasonal variations of atmospheric ammonia peak concentrations at the LPA and LPB, and ) shows the seasonal variations of averages of atmospheric ammonia concentrations at the HMSW and FA. The seasonal variability of atmospheric ammonia concentration at the west or south boundary of hybrid MSW site in the summer survey was 4.42 or 4.58 times higher than in the winter survey. Even the ammonia concentration at the flying ash area was 3.88 times than in the winter survey.
This study shows that ambient temperature is a key factor of seasonal variabilities of atmospheric ammonia concentrations at the Laogang MSW landfill. In addition, the seasonal variabilities of atmospheric ammonia concentrations at various areas of the Laogang MSW landfill also depend on the waste types and the statues of those areas, such as closed or active MSW areas, active or storage leachate areas. The seasonal variability of ambient ammonia levels at the active MSW landfill site was more significant than at the closed MSW landfill sites, and the seasonal variability of ambient ammonia levels at the active leachate pool was more significant than at the leachate treatment pool or leachate storage pool.
The heterogeneity of MSW and clogging of the drainage layer significantly affect the distribution of leachate (Yang, Xu, and Chai Citation2019). The inner source water and rainfall are two important sources of landfill leachate (Dang et al. Citation2020). High leachate level causes landfill instability and landslides (Yang et al. Citation2016). Therefore, Laogang landfill’s operator should pay attention to the clogging of drainage and manage the internal source water and rainfall to reduce leachate and maintain the landfill stability. In addition, classification can effectively help the recovery and utilization of a landfill and reduce the amount of MSW (Yang, Xu, and Chai Citation2018).
Linearity of the MW-DOAS system
Since the ammonia emission levels at the LPA, LPB, and LPC in the winter or summer survey were above the quoted detection range of the MW-DOAS system, an investigation into the linear response of ammonia at high concentrations was conducted. Various ammonia levels between 0 and 5 ppm were introduced into the sample cell of the MW-DOAS system to evaluate the mobile measurement results in the winter and summer surveys. shows the NH3 linearity testing result of mobile DOAS system in the investigation. It was found that the high ammonia levels at the LPA, LPB, and LPC were still within the reasonable linear measurement range of MW-DOAS system.
Comparison between MW-DOAS and standard AQM technique
To validate the atmospheric ammonia measurement accuracy of the MW-DOAS, a comparison test was implemented immediately after the first mobile measurement in winter at a nearby air quality monitoring (AQM) station, located at the south boundary of Laogang landfill. The AQM station uses a standard ThermoFisher Scientific 17i instrument to monitor local atmospheric ammonia, and the results were compared with the MW-DOAS system at the same time and location. The ThermoFisher Scientific 17i instrument is based on chemiluminescence technology and uses the chemical reaction to convert NH3 and NO2 to NO in a 750°C chamber; then it measures a characteristic luminescence related to the concentration variation of NO and calculates the atmospheric ammonia concentration. shows the measurement results of the MW- DOAS system and that standard ammonia AQM instrument at low atmospheric ammonia concentration.
Table 3. The performance comparison of ammonia detection techniques
One standard AQM station consists of various fixed AQM instruments, a zero air generator, an air flow blender and an air conditioner. The cost of one standard AQM station is generally more than a MW-DOAS system. One or more standard AQM stations are not enough to real-time monitor odor pollutants at all areas of a large landfill. However, a MW-DOAS system can real-time monitor most of common air or odor pollutants at all areas of a whole large landfill, except carbon monoxide and carbon dioxide. Therefore, the economic feasibility of MW-DOAS is much better than that of a standard AQM station.
Further research considerations
The fast screening of atmospheric ammonia over a whole landfill can be achieved using the MW-DOAS system. Hot spots of landfill atmospheric ammonia can be identified through the combination of atmospheric ammonia levels from the MW-DOAS system and wind direction information. The fast movement of the vehicle and the MW-DOAS support fast screening of air samples; however, vehicle speed and variation of wind direction might affect the stability of air sampling. Therefore, the vehicle speed should be stable and limited to 20 miles per hour. The distance between sampling positions and fugitive sources also affects the measurement results, and the vehicle route should be close to fugitive sources. Moreover, more experiments and data are needed to model the quantitative impact of MSW age, wind, and ambient temperature to ambient ammonia concentration within a landfill. This will help to predict the ambient ammonia in a landfill.
Conclusion
The continuous, fast, and real-time monitoring of atmospheric ammonia in the whole Laogang landfill was implemented using a mobile active DOAS. The atmospheric ammonia concentrations were quantitatively measured, and the hot spots of atmospheric ammonia were effectively identified at various landfill areas, such as the MSW-related, leachate-related, sludge-related, and the fly ash-related areas. The mobile sweep survey revealed that the active MSW site, the active leachate pool, and the leachate treatment pool are the main ammonia sources in the Laogang landfill, and the active leachate pool in the active MSW site caused the highest atmospheric ammonia level among all areas. The MW-DOAS is an effective solution for the sweep survey of atmospheric ammonia over a whole MSW landfill from ppb level to ppm level.
The atmospheric ammonia levels at those various types of areas in the Laogang landfill were significantly different and were related to the characteristics of those areas. In particular, atmospheric ammonia levels at various MSW landfill sites were related to the status of those MSW landfill sites: active MSW site or closed MSW site. Furthermore, the ages of the closed MSW landfill sites has a significant impact on the atmospheric ammonia, where it was found that the later closed MSW landfill site has a much higher atmospheric ammonia level than the earlier closed MSW landfill site. The atmospheric ammonia levels at all closed MSW landfill sites were relatively low and stable.
The significant seasonal variabilities of atmospheric ammonia were dependent on the ambient temperature at MSW-related, leachate-related, and fly ash-related areas, except the leachate treatment pool of the leachate treatment plant. The atmospheric ammonia levels at the active leachate pool and active MSW site in the summer survey were 3.5 times and 5.58 times higher than in the winter survey, respectively. For the closed MSW sites with various ages, the ammonia levels in the summer survey varied between 3.82 and 7.38 times higher than in the winter survey. The absolute seasonal variability of atmospheric ammonia concentration at the active MSW landfill site was more significant than at the closed MSW landfill sites, and the absolute seasonal variability of atmospheric ammonia level at the active leachate pool was more significant than at the leachate treatment pool or leachate storage pool.
Disclosure statement
The authors declare no conflict of interest.
Additional information
Notes on contributors
Xuewen Yi
Xuewen Yi is a Ph.D. student at School of Environmental and Chemical Engineering, Shanghai University.
Zhiyong Zhang
Zhiyong Zhang is a senior engineer at DUVAS Technologies.
Phillipa Smith
Phillipa Smith is the CTO at DUVAS Technologies.
References
- Ampollini, L., E. F. Katz, S. Bourne, Y. Tian, A. Novoselac, A. H. Goldstein, G. Lucic, M. S. Waring, and P. F. DeCarlo. 2019. Observations and contributions of real-time indoor ammonia concentrations during HOMEChem. Environ. Sci. Technol. 53: 8591−8598.
- Behera, S. N., and M. Sharma. 2010. Investigating the potential role of ammonia in ion chemistry of fine particulate matter formation for an urban environment. Sci. Total Environ. 408 (17):3569–75. doi:10.1016/j.scitotenv.2010.04.017.
- Breitenbach, L. P., and M. Shelef. 1973. Development of a method for the analysis of NO2 and NH3 by NO− measuring instruments. J. Air Pollut. Control Assoc. 23 (2):128–31. doi:10.1080/00022470.1973.10469752.
- Chiriac, R., J. D. A. Morais, J. Carre, R. Bayard, J. M. Chovelon, and R. Gourdon. 2011. Study of the VOC emissions from a municipal solid waste storage pilot-scale cell: Comparison with biogases from municipal waste landfill site. Waste Manag. 31 (11):2294–301. doi:10.1016/j.wasman.2011.06.009.
- D’Amato, F., S. Viciani, A. Montori, A. Lapini, I. Fraboulet, and J. Poulleau. 2020. Optical detection of ammonia inside a stack: Comparison of different techniques. Measurement 159:1–9. doi:10.1016/j.measurement.2020.107746.
- Dammers, E., M. Schaap, M. Haaima, M. Palm, R. J. Wichink Kruit, H. Volten, A. Hensen, D. Swart, and J. W. Erisman. 2017. Measuring atmospheric ammonia with remote sensing campaign: Part 1 – characterisation of vertical ammonia concentration profile in the centre of The Netherlands. Atmos. Environ. 169:97–112. doi:10.1016/j.atmosenv.2017.08.067.
- Dang, M., J. Chai, Z. Xu, Y. Qin, J. Cao, and F. Liu. 2020. Soil water characteristic curve test and saturated-unsaturated seepage analysis in Jiangcungou municipal solid waste landfill, China. Eng. Geol. 264. doi:10.1016/j.enggeo.2019.105374.
- Davoli, E., M. L. Gangai, L. Morselli, and D. Tonelli. 2003. Characterisation of odorants emissions from landfills by SPME and GC/MS. Chemosphere 51 (5):357–68. doi:10.1016/S0045-6535(02)00845-7.
- de Sá Borba, P. F., E. M. Martins, E. Ritter, and S. M. Corrêa. 2017. BTEX emissions from the largest landfill in operation in Rio de Janeiro, Brazil. Bull. Environ. Contam. Toxicol. 98 (5):624–31. doi:10.1007/s00128-017-2050-5.
- Ding, Y., C. Cai, B. Hu, Y. Xu, X. Zheng, Y. Chen, and W. Wu. 2012. Characterization and control of odorous gases at a landfill site: A case study in Hangzhou, China. Waste Manag. 32 (2):317–26. doi:10.1016/j.wasman.2011.07.016.
- Erisman, J. W., Otjes, R., Hensen, A., Jongejan, P., van den Bulk, P., Khlystov, A., Moels, H., and Slanina, S. 2001. Instrument development and application in studies and monitoring of ambient ammonia. Atmos. Environ. 35:1913–1922 doi:10.1016/S1352-2310(00)00544-6.
- Fang, J., F. Lü, H. Zhang, L. Shao, and P. He. 2015. Odor compounds released from different zones of two adjacent waste treatment facilities: Interactive influence and source identification. J. Air Waste Manage. Assoc. 65 (12):1446–55. doi:10.1080/10962247.2015.1104401.
- Fang, J., N. Yang, D. Cen, L. Shao, and P. He. 2012. Odor compounds from different sources of landfill: Characterization and source identification. Waste Manag. 32 (7):1401–10. doi:10.1016/j.wasman.2012.02.013.
- Feng, S.-J., K.-W. Gao, Y.-X. Chen, Y. Li, L. M. Zhang, and H. X. Chen. 2017. Geotechnical properties of municipal solid waste at Laogang Landfill, China. Waste Manag. 63:354–65. doi:10.1016/j.wasman.2016.09.016.
- Galle, B., L. Klemedtsson, B. Bergqvist, M. Ferm, K. Törnqvist, D. W. T. Griffith, N.-O. Jensen, and F. Hansen. 2000. Measurements of ammonia emissions from spreading of manure using gradient FTIR Techniques. Atmos. Environ. 34 (28):4907–15. doi:10.1016/S1352-2310(00)00220-X.
- He, P., N. Yang, H. Gu, H. Zhang, and L. Shao. 2011. N2O and NH3 emissions from a bioreactor landfill operated under limited aerobic degradation conditions. J. Environ. Sci. 23 (6):1011–1019.
- Keuken, M. P., C. A. M. Schoonebeek, A. van Wensveen-louter, and J. Slanina. 1998. Simultaneous sampling of NH3, HNO3, HC1, SO2 and H2O2 in ambient air by a wet Annular Denuder system. Atmos. Environ. 22 (11):2541–48. doi:10.1016/0004-6981(88)90486-6.
- Norman, M., Hansel, A., and Wisthaler, A. 2007. O2+ as reagent ion in the PTR-MS instrument: Detection of gas-phase ammonia. Intern. J. Mass Spectrom. 265:382–287. doi:10.1016/j.ijms.2007.06.010.
- Parker, T., J. Dottridge, and S. Kelly. 2002. Investigation of the composition and emissions of trace components in landfill gas. Bristol: UK Environment Agency.
- Platt, U., and J. Stutz. 2008. Differential optical absorption spectroscopy: principles and applications. Berlin, German: Springer.
- Rom, H. B., and G.-Q. Zhang. 2010. Time delay for aerial ammonia concentration measurements in Livestock buildings. Sensors 10:4634–42. doi:10.3390/s100504634.
- Sarkar, U., S. E. Hobbs, and P. Longhurst. 2003. Dispersion of odour: A case study with a municipal solid waste landfill site in North London, United Kingdom. J. Environ. Manage. 68 (2):153–60. doi:10.1016/S0301-4797(03)00060-4.
- Su, D. 2005. Operation and management in Laogang refuse Landfill. Shanghai, China: Tongji University.
- Suarez-Bertoa, R., P. Mendoza-Villafuerte, F. Riccobono, M. Vojtisek, M. Pechout, A. Perujo, and C. Astorga. 2017. On-road measurement of NH3 emissions from gasoline and diesel passenger cars during real world driving conditions. Atmos. Environ. 166:488–497.
- Tang, J. 2016. Key process analysis and assessment of Shanghai Laogang comprehensive landfill. Environ. Sanit. Eng. 24 (3):25–27.
- Tian, H., J. Gao, J. Hao, L. Lu, C. Zhu, and P. Qiu. 2013. Atmospheric pollution problems and control proposals associated with solid waste management in China: A review. J. Hazard. Mater. 252–253:142–54. doi:10.1016/j.jhazmat.2013.02.013.
- von Bobrutzki, K., C. F. Braban, D. Famulari, S. K. Jones, T. Blackall, T. E. L. Smith, M. Blom, et al. 2010. Field inter-comparison of eleven atmospheric ammonia measurement techniques. Atmos. Meas. Tech. 3:91–112. doi:10.5194/amt-3-91-2010.
- Wang, S., J. Nan, C. Shi, Q. Fu, S. Gao, D. Wang, H. Cui, A. Saiz-Lopez, and B. Zhou. 2015. Atmospheric ammonia and its impacts on regional air quality over the megacity of Shanghai, China. Sci. Rep. 5:15842. doi:10.1038/srep15842.
- Warneck, P. 1988. Chemistry of the natural atmosphere. New York: Academic Press.
- Wu, C., S. Liu, M. Shu, C. Qu, J. Liu, M. Piringer, and G. Schauber. 2018. Seasonal variations in odorous compounds emission from a municipal solid waste disposal plant. Chem. Eng. Trans. 68:85–90.
- Yang, R., Z. Xu, and J. Chai. 2018. A review of characteristics of landfilled municipal solid waste in several countries: Physical composition, unit weight, and permeability coefficient. Polish J. Environ. Stud. 27 (6):2425–35. doi:10.15244/pjoes/81089.
- Yang, R., Z. Xu, and J. Chai. 2019. Seepage analysis of a multilayer waste slope considering the spatial and temporal domains of permeability. Adv. Civ. Eng. 2019. doi:10.1155/2019/3689097.
- Yang, R., Z. Xu, J. Chai, Y. Qin, and Y. Li. 2016. Permeability test and slope stability analysis of municipal solid waste (MSW) in Jiangcungou Landfill, Shaanxi, China. J. Air Waste Manage. Assoc. 66 (7):655–62. doi:10.1080/10962247.2015.1093038.
- Yokelson, R. J., T. J. Christian, I. T. Bertschi, and W. M. Hao. 2003. Evaluation of adsorption effects on measurements of ammonia, acetic acid and methanol. J. Geophys. Res. 108 (D20):4649. doi:10.1029/2003JD003549.
- Zhao, M., S. Wang, J. Tan, Y. Hua, D. Wu, and J. Hao. 2016. Variation of Urban Atmospheric Ammonia Pollution and Its Relation with PM2.5 Chemical Property in Winter of Beijing, China. Aerosol Air Qual. Res. 16:1378–1389.
- Zhou, H., S. Lan, and Y. Zhao. 2015. Engineering application of dried sludge single landfill. Environ. Sanit. Eng. 23 (5):68–70.
- Zou, S. C., S. C. Lee, C. Y. Chan, K. F. Hu, X. M. Wang, L. Y. Chan, and Z. X. Zhang. 2003. Characterization of ambient volatile organic compounds at a landfill site in Guangzhou, South China. Chemosphere 51 (9):1015–22. doi:10.1016/S0045-6535(03)00004-3.