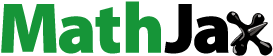
ABSTRACT
Dust from construction field is one of sources for the atmosphere particulate pollution. In this study, a solidifying material, modified polyvinyl alcohol (MPA), is used to control the dust from soil. The controlling efficiency of the MPA is evaluated based on its anti-wind and anti-rain erosion performance under different conditions such as soil types, spraying amount of MPA, slope morphologies (gradients and unevenness), and treated time. Results indicate that the MPA can bind well with the soil particles and it is able to strengthen the integrity of the surface. Soil treated with MPA exhibits good anti-wind effects, and PM10 and PM2.5 in environment do not increase even under a nine-grade wind erosion. Soil treated with MPA can maintain the surface integrity under a rainstorm, and there are no erosion traces and mud after rain erosion. The application of MPA has no limitation in terms of soil type, soil slope, and surface morphology, and thus, it has a wide applied prospect in engineering.
Implications: In order to solve the air pollution problems existing on construction sites in densely populated areas, MPA optimizes a kind of environmental agent to treat, which does not affect the later use of soil. The applicability effect was verified from the perspective of anti-wind and anti-rain erosion doubly. Research results not only can provide data support for the construction management, but also help to promote the civilization and ecological benefit of construction.
It has been reported by the World Health Organization that only less than 1% of Chinese cities satisfy the air quality standard, and air pollutants (such as PM10 and PM2.5) have been the main factors affecting the regional environment (ADB, Citation2012). Dust is one of atmospheric particulate pollutants and it is complex to predict its flow motion (Bhowmick, Raj, and Bandopadhyay Citation2015). In some large and medium-sized cities, the dust from soil contributes to 13%–37% of pollution (Li, Long, and Tie Citation2016), dust from construction sites accounts for 70% to 90% of PM10, and 6% of PM2.5 (Muleski, Cowherd, and Kinsey Citation2005). Such type of dust is usually found in the low-altitude and densely populated urban areas (Long, Li, and Tie Citation2016). Therefore, a cost-effective and efficient control approach that can significantly reduce the dust from the soil/construction site is urgently required.
Currently, greening, sweeping, covering, and water sprinkling are the main solutions for the above problem. Although the application of water sprinkling is easy, it is unsustainable and has a limited effectiveness in arid environments (Hamdanand, Citation2016); covering is only suitable for provisional dispositions, and greening/sweeping needs long-term implementations. Utilizing polymer materials to form a protective shell on soil surfaces can prevent the direct contact of soil with air, which makes it become a prominent strategy in the control of dust arising. However, its applicability mainly depends on the sensitivity of the site environment in which the method will be applied.
In this study, the modified polyvinyl alcohol (MPA) is proposed as the reinforcement material for the construction site to verify its dust-control effect, with the consideration of the following influencing factors: soil types, spraying amount of MPA, slope morphologies (gradients and unevenness), and treated time. The parameters of wind and rain erosion tests are selected based on the wind grade and rain strength in Tianjin, Beijing, and Hebei provinces in the last 50 years. Fluctuations of PM10 and PM2.5 are utilized to evaluate the anti-wind erosion performance, while rain-erosion quantities, surface failures, and particle distributions are utilized to determine the anti-rain erosion performance. The research results can provide reference for dust control and improve the environmental quality of construction site.
Materials and methods
Test materials
Silt, fly ash, cohesive soil, and gravel soil found in construction sites are considered, and their particle size distributions are provided in .
Table 1. Particle size distributions of the soil
MPA is a type of water-soluble polymer material that has a low viscosity (Wang et al. Citation2004; Citation2005). The basic physical and chemical properties are presented in . The application of MPA is convenient, and it only requires dilution of the MPA in water. Note that when MPA is exposed to air, it will form a film of a certain thickness that has excellent anti-dissolution properties (). Once in contact with the soil, it will form a network system under the interaction of bonding, chelation, adsorption, and bridging () (Wenwu et al., Citation2019). Preliminary MPA environmental assessment studies on seed germination and response from pet fish seemed encouraging with no obvious adverse impacts. MPA application to construction field deserves a comprehensive environmental study addressing its potential harm to drinking-water supplies and aquatic lives.
Table 2. Physical and chemical properties of MPA
Test methods
Soil sample preparation
In order to provide the convenience of construction, the spraying amount of MPA is determined according to the soil area (as 0, 0.9, 1.2, and 1.5 kg/m2), and the water demand is determined according to the optimal moisture content of soils (cohesive soil with 20%, silt with 18%, sandy soil with 10%, and gravel soil with l8%) (Herrmann and Bucksch Citation2014). MPA is dissolved into water first, and then the mixed solution is uniformly sprayed on soil surfaces, and finally, the soil sample is air-dried in natural conditions. Simultaneously, the soil only treated by water is utilized as a control sample. The size of soil sample is a length of 600 mm, width of 600 mm, and height of 30 mm.
Wind erosion test
Wind erosion test was conducted on the wind distribution in the recent 50 years in Beijing and Tianjin, and wind grade of nine is chosen for the tests. The process of wind erosion can be seen in . Wind speed is controlled with the help of the air blower, and the trumpet-shaped air outlet and soil sample are set in a sealed cavity. In order to ensure the uniformity of wind erosion, the nozzle adopts horn nozzle, and the size is a length of 1,000 mm, width of 10,000 mm. The air outlet is facing the soil sample with 10 cm distance. The soil sample is placed on the device with an adjustable angle from 0° to 90o. The PM10 and PM2.5 detector is in the downwind direction. The measurement method for PM10 and PM2.5 data is as follows: the environmental value is measured once before the wind erosion experiment, and then the actual value is measured continuously during the wind erosion process.
Reynolds number (Re) is calculated. Construction sites are usually located in urban areas that have a C roughness coefficient (Huang and Wang Citation2010). Based on the wind effect, Re is
where v is the wind speed, m/s, and D is the diameter of the section, m.
The height of a mound in construction sites is 2.5 m, and according to the data for the wind grade in Beijing (Xiong Citation2015), Tianjin, and Hebei, the wind speed is 23 m/s, so that Re is 3.97 × 106.
Re in pipes is (Evans and Morris Citation2017)
where v is average wind speed, m/s; D is diameter of the pipes, m; μ is hydrodynamic viscosity, Pa●s; υ is kinematic viscosity, m2/s; ρ is fluid density, kg/m3; Q is volume flow, m3/s; and A is cross-sectional area, m2.
The air density was 1.205 kg/m3 and hydrodynamic viscosity was 1.81 × 10−5 Pa●s at a temperature of 20°C. The air volume of the centrifugal ventilator (11–62 type) utilized in the test was 10,800 m3/h; therefore, Re is 3.99 × 106, similar to the value for the construction sites.
An anemograph (AR826+) which has a measurement range of 0.3–45 m/s and a measurement accuracy of 0.1 m/s as well as a special testing instrument for PM10 and PM2.5 (CW-HAT200) with a measurement range of 0–999 μg/m3 and a measurement accuracy of 1 μg/m3 are utilized.
Taking the environmental background value as the reference, the variation in PM10 and PM2.5, represented as ΔPM10 and ΔPM2.5, respectively, is analyzed:
PM2.5i or PM10i is the concentration value during the wind-erosion test, and PM2.5o or PM10o is the concentration value for the environment.
Rain erosion test
Rain erosion test is based on the precipitation distribution in the recent 50 years in Beijing and Tianjin, China, and the storm grade of 200 mm/h and rainfall time of 60 min are chosen for the tests. The process of rain erosion can be seen in . An artificial rainfall simulator (HQJY) is used (Lassu et al. Citation2015), where a pump and spray-head are employed to adjust the precipitation intensity and raindrops size, respectively. The spray head is 4 meters above the ground, and a measuring cylinder in the center of the test area is used to monitor rainfall intensity. In the test area (effective rainfall area is a length of 4 m and a width of 4 m), nine groups of spray-head were evenly spaced, and each group had three sizes of spray-head, simulating small, medium, and large raindrops. The control range of raindrop size is from 0.3 mm to 6 mm. Automatic data acquisition is used to control the opening of the spray-head and monitor the precipitation intensity. Two trays are placed under rainfall area, of which the smaller one is filled with soil sample and then kept in the center of the larger tray. There is an outlet on one side of the large tray where the erosion objects can be collected.
The erosion quantity (i.e., the weight of the dry soil washed down by rain erosion, g) is used to assess the anti-rain erosion quantitively, while the erosion traces on the soil surface and morphology distribution of the soil particulate are used as qualitative assessment for the anti-rain erosion effect.
The parameters of spraying amount, soil type, slope, morphology, and treated time in wind and rain erosion tests are listed in .
Table 3. Parameters of influencing factors
Results and discussion
Influence of MPA on the soil structure
shows that significant shrinkage-cracking and peeling occur during the drying process on the soil surface that is treated only with water, and even an entire piece crust separates at the corner. However, there are little small cracks on the surface without peeling after the MPA treatment (), the regular cracks on MPA treated soil occur in the process of sample preparation and compaction. MPA can bond well with the soil particles and then strengthen the soil integrity. Therefore, MPA can effectively solve the problem of shrinkage-cracking and peeling extant in the sample only treated with water.
Figure 5. Surface morphologies distribution of soil treated with water and different spraying amount of MPA
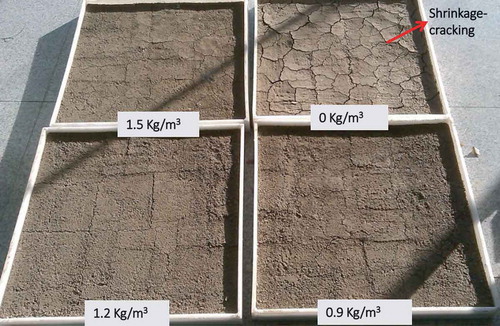
Figure 6. Erosion weight and products as a function of MPA spray amount variability with rain erosion setup shown in
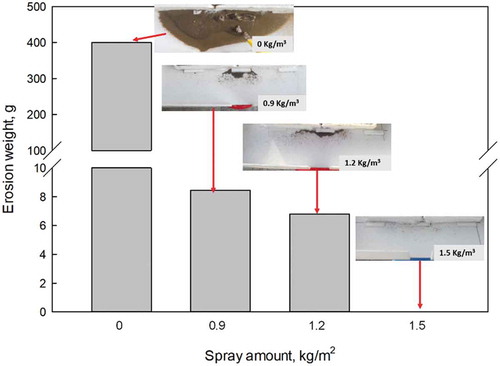
Influence of spraying amount of MPA on anti-rain and anti-wind erosion performances
The soil sample that is only treated with water is easily washed down in rainstorms; within one hour, the erosion weight is 400 g, as shown in . In contrast, for the soil treated with MPA, the erosion weight is low; specifically, it is 8.46 g for a spraying amount of 0.9 kg/m2, accounting for around 1/50 of the erosion weight of the soil treated with water. Furthermore, the erosion weight of the soil treated with MPA is 6.81 g corresponding to 1.2 kg/m2 of spraying amount and 0 g corresponding to 1.5 kg/m2 of spraying amount. Thus, the erosion weight decreases with an increase in the spraying amount, and the soil can avoid the rain erosion damage when the spraying amount is 1.5 kg/m2 ().
Erosion particles of the soil that is treated with water appear in a sludge state. However, the erosion particles from the soil treated with MPA are larger and exist in aggregate states (). The erosion particles of the soil that is treated with MPA originate mainly from the soil corners. This confirms that there is a strong cohesive force between the MPA and the soil particles, which enhances the erosion resistibility of the soil.
Figure 8. Soil erosion particles distribution as the function of rain erosion with setup shown in , and treatment variability (a) with water only and (b) with MPA of 0.9 kg/m2
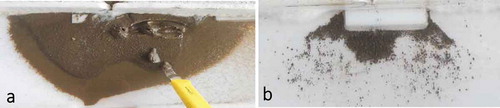
As to the anti-wind erosion performances, the concentration values of PM2.5 and PM10 of the soil treated with MPA are less than those of the soil treated only with water under the nine-grade wind erosion, as depicted in . The PM10 and PM2.5 of the soil treated with water are much larger than the environmental values in the first 10 min of the wind erosions tests, and later, they fluctuate around the environmental values. The environment value is the actual climatic value, and it was measured before the experiment started. The particles located at the shrinkage cracking and peeling areas will be expected to rise again under wind erosion conditions.
Figure 9. Distribution of PM10 and PM2.5 as a function of wind erosion time with setup shown in , and MPA spraying amounts variability
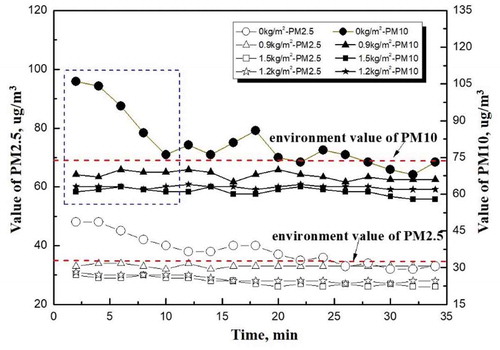
The concentration values of PM10 and PM2.5 from the soil treated with MPA remain stable and are lower than the environmental values under the condition of nine-level wind erosion (). The curve of the soil treated with 0.9 kg/m2 of MPA exhibits a strong fluctuation. The stability of the curve increases with the spraying amount. For the higher spraying amounts of 1.2 and 1.5 kg/m2, the PM10 and PM2.5 curves are similar and stable overall.
Influence of the soil type on anti-rain and anti-wind erosion performances
In term of anti-rain erosion performances, shows that the erosion weights of silt, fly ash, gravel soil, and clay are 5.91, 86.79, 3.69, and 6.81 g, respectively. The anti-rain erosion performances of treated silt, gravel soil, and cohesive soil are excellent, but the erosion weight for fly ash is high. This could be explained by the property of fly ash. Fly ash particles have a multi-pore cellular structure, larger specific surface area, and so, it is easier for fly ash to absorb water. During rainstorms, water infiltrates the underlying soil from the side seams, and then the fly ash loses its bond force with the pan, finally leading to landslide damages. However, an analysis of the surface failure reveals that the integrity of the treated surfaces remains intact when the landslide occurs and there is no obvious erosion trace.
Figure 10. Erosion weights and failures as the function of soil type variability with rain erosion setup shown in
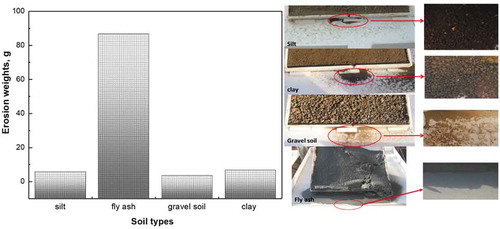
When the particles washed down in the erosion part are compared for different types of soil, it turns out that the particles originating from treated silt and clay are large and loose, with the particle size of the latter being slightly larger than that of the former (). For treated gravel soil, the particles are extremely small and no gravel is washed down. Therefore, the MPA treatment could strengthen the anti-rain erosion performances effectively of different soil type.
As for anti-wind erosion performances, the concentration value of PM10 and PM2.5 of silt, fly ash, cohesive soil, and gravel soil that treated with MPA are lower than the environmental value during wind erosions. Their curves in are stable except for gravel soil; after wind erosion of 12 min, the curve of gravel soil increases suddenly and then immediately decreases. This phenomenon is due to the distinct corner morphology of the gravel soil. Some films were damaged by the wind erosions, causing fine particles in erosion. However, this wind damage was instantaneous as the failure surfaces did not extend, and the other films continued to exhibit anti-wind erosion performances ().
Figure 11. ΔPM10 and ΔPM2.5 (see EquationEqs. 3(3)
(3) and Equation4
(4)
(4) ) as a function of wind erosion time with setup shown in , and soil type variability
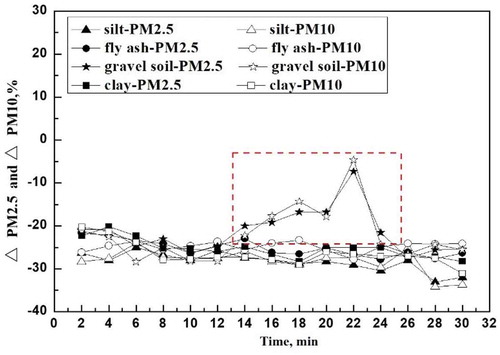
Influence of slopes on anti-rain and anti-wind erosion performances
In term of anti-rain erosion performances, the erosion weight exhibits a gradual increasing trend with increasing slope, as shown in . The erosion weights are 6.81, 9.11, 11.61, and 14.69 g for the slopes of 0°, 10°, 20°, and 30°, respectively. The largest erosion weight of MPA treated soil is only 0.037 times that of the soil treated only with water. Thus, soil slopes have little effect on the treatments and anti-rain erosion performances.
Figure 12. Erosion weights and PM10 and PM2.5 distribution as a function of soil slopes variability with rain and wind erosion setup shown in and , respectively
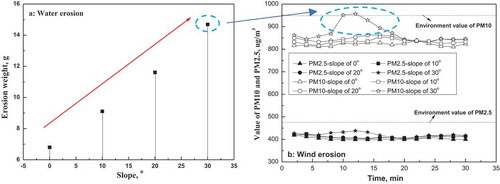
PM10 and PM2.5 of the treated soils with slopes of 20°, 10°, and 0° remain stable and tend to be uniform as shown in . However, a clear fluctuation is seen for the slope of 30°. The reason for this could be explained by the following two aspects: (i) the effective stress areas and wind strengths are higher for steeper slopes, and (ii) the uniformity of the treatment is affected by gravity, causing some weak interfaces. Therefore, it is suggested to increase the spraying amount or spraying times on the weak zones, especially for soils with steeper slopes or edges and corners.
Influence of surface morphologies on anti-rain and anti-wind erosion performances
The erosion weight of the soil that was treated with MPA is 6.81, 5.75, 8.52, and 7.06 g for surfaces that are smooth planes, rough planes, smooth uneven, and rough uneven, respectively (). The erosion weights with these four surfaces were similar, and the erosion weight of the plane soil is 1–2 g less than that of the uneven surface, while the erosion weight of the smooth surface is 1 g more than that of the rough surface.
Figure 13. Erosion weights and failures as a function of soil slope morphology variability with rain erosion setup shown in
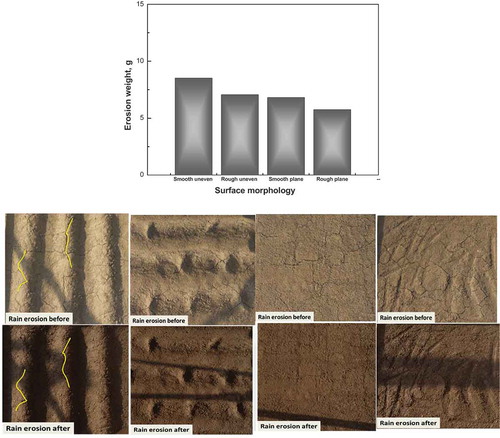
The comparison of surface erosion failures in reveals that there are no obvious erosion traces on the surfaces of the treated soils. Cracks on the surfaces present before the rain erosion do not extend after the rainstorms. In the case of rough surfaces, the irregular depression areas do not form weak interfaces and the surfaces exhibit reasonable anti-rain erosion performances.
Figure 14. ΔPM10 and ΔPM2.5 (see EquationEqs. 3(3)
(3) and Equation4
(4)
(4) ) as a function of soil slope morphologies and density with wind erosion setup shown in Figure 3
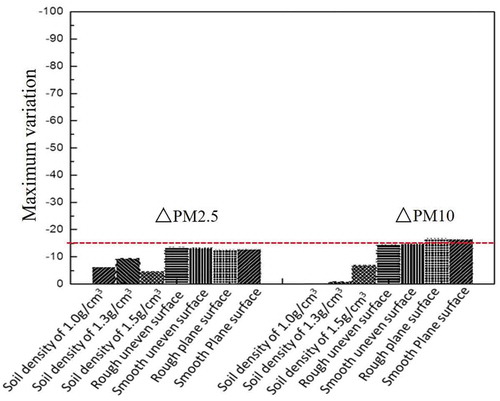
As for anti-wind erosion performances, PM10 and PM2.5 corresponding to different surface morphologies have a minor effect on the anti-wind erosion performances (). Also, all the treated surfaces maintain their integrity without shrinkage-cracking and peeling. The MPA treatment could cover all the rugged and weak interfaces completely, and then connects them to resist the wind erosion.
Therefore, it can be deduced that the treatment with MPA was suitable for any surface morphology, and the roughness of the soil has a negligible effect on the treatment and anti-wind erosion performance.
Conclusion
MPA is in a reasonable anti-dissolution property, and the weight change in the water is within 0.1% of the total mass. MPA binds well with the soil particles and can strengthen the integrity of the soil surfaces. It can effectively solve the problem of shrinkage-cracking extant in water sprinkling.
MPA is suitable for soil treatment. Slopes, morphologies, and type of soil have little impact on the treatment effects. Even when the slope is steep or the surface is rough, MPA can infiltrate the soil and connect with the particles on the surfaces to form wind and rain erosion-resistant protective shell.
MPA is beneficial for treating silt, fly ash, clay, and gravel soil. PM10 and PM2.5 do not increase under the ninth-grade wind erosion, and simultaneously, the soil surface can maintain the integrity under a rainstorm. There are no erosion traces and mud to be observed. Cracks on the surfaces do not extend under wind and rain erosions. Concurrently, the irregular depression areas on the soil surfaces do not form weak interfaces.
In view of the treatment effects and the construction and economy requirements, the optimum spraying amount is identified as 1.2 kg/m2. However, as it is effective on steeper slopes or with numerous gravels, it should be sprayed several times to prevent weak interface.
Acknowledgment
The authors are grateful to the Hebei Research Center of Civil Engineering Technology for its support.
Additional information
Funding
Notes on contributors
Min Li
Min Li is the professor in Hebei University of Technology. Her work revolves around the pollution control and treatment and the improvement of construction environment. The research shed light on the formation mechanism and control technology.
Hongpu Du
Hongpu Du works in the Henan Information Industry Investment Co., Ltd. He mainly engages in the technology achievements transferring of infrastructure intelligent & information, and construction environment.
Lang Zhou
Lang Zhou is pursuing Ph.D. degree in Environmental Engineering Science at University of Florida. His work revolves around the notion of value-added to a readily available waste material. The study would shed light on the mechanisms by which different metal types can be immobilized on a highly heterogeneous sorbent (DWTRs).
Shouxi Chai
Shouxi Chai is the professor in Tianjin Chengjian University. He is a member of the engineering geology committee of the Chinese Geological Society. His work engages in the fields of civil engineering construction.
Min Wang
Min Wang is a master student in Hebei University of Technology. His work revolves around the improvement of construction environment.
References
- ADB (Asian Development Bank). 2012. Toward an environmentally sustainable future: Country environmental analysis of the People’s Republic of China. IDEAS Working Paper Series from RePEc, 224. St Louis: Federal Reserve Bank of St Louis
- Bhowmick, T., K. V. Raj, and S. Bandopadhyay. 2015. Three-dimentional modeling of fugitive dust dispersion in idealized open pit mines. Mining Eng. 67 (10):45–52.
- Evans, M. J., and R. J. Morris. 2017. Chemical agents transported by xylem mass flow propagate variation potentials. Plant J. 91 (6):1029–37. doi:10.1111/tpj.13624.
- Hamdan, N., and E. Kavazanjian. 2016. Enzyme-induced carbonate mineral precipitation for fugitive dust control. Geotechnique 66 (7):546–55. doi:10.1680/jgeot.15.P.168.
- Herrmann, H., and H. Bucksch. 2014. Dictionary geotechnical engineering. Berlin: Springer Berlin Heidelberg.
- Huang, S., and Y. Wang. 2010. New code for seismic design of buildings. Beijing: China Architecture & Building Press.
- Lassu, T., M. Seeger, P. Peters, and S. D. Keesstra. 2015. The wageningen rainfall simulator: Set-up and calibration of an indoor nozzle-type rainfall simulator for soil erosion studies. Land Degrad. Dev. 26 (6):604–12. doi:10.1002/ldr.2360.
- Li, N., X. Long, and X. Tie. 2016. Urban dust in the Guanzhong basin of China, part II: A case study of urban dust pollution using the WRF-Dust model. Sci. Total Environ. 541 (15):1614–24. doi:10.1016/j.scitotenv.2015.10.028.
- Long, L., N. Li, and X. Tie. 2016. Urban dust in the Guanzhong Basin of China, part I: A regional distribution of dust sources retrieved using satellite data. Sci. Total Environ. 15 (January):1603–13. doi:10.1016/j.scitotenv.2015.10.063.
- Muleski, G. E., C. Cowherd, and J. S. Kinsey. 2005. Particulate emission from construction activities. J. Air Waste Manage. Assoc. 55 (6):772–83. doi:10.1080/10473289.2005.10464669.
- Wenwu, C., Z. Qiyong, and L. Hongwei. 2019. Reinforcing effect of relic soil sites penetrated with high polymer martial. Rock Soil Mech. 40 (2):429–435+444.
- Xiong, M. 2015. Climate regionalization and characteristics of surface winds over China in recent 30 years. Plateau Meteorol. 34 (1):39–49.
- Yingmei, W., H. Wenfeng, and C. Wenwu. 2004. Experimental study on resistances to aging of a new polymer solidified material[J]. J. Eng. Geol. 3:318–22.
- Yingmei, W., C. Wenwu, and H. Wenfeng. 2005. Simulation study on resistances to wind erosion of new polymer material in sand fixation. J. Soil Water Conserv. 6:14–16.