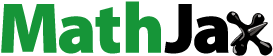
ABSTRACT
The fine particles are considered a significant environmental pollution problem. The wet electrostatic precipitators (ESPs) are now used most widely among the advanced fine particle removal technologies. The rigid glass fiber reinforced plastics (FRP) collectors modified with hole-slotted structures were used to enhance the water film distribution over their surfaces. This paper experimentally investigated the effects of the hole-slot patterns and the operating parameters on the particle removal performance in wet ESPs using the hole-slot-structured collector. The voltage-current characteristics and the energy consumption among the different pattern collectors were discussed. Besides, the effects of the water film on the particle number concentration classification and the effects of the applied voltage and the specific collecting electrode areas (SCA) on the relative collection efficiency (RCE) were investigated and discussed. The experiment results show that the corona current of three hole-slot structured FRP collectors was higher than that of the conventional rigid collectors (CRC) at the same voltage. Using the FRP collectors with circular holes and horizontal slots could decrease the energy consumption and using the FRP collectors with vertical slots increases the energy consumption compared with the CRC when the collection efficiency was lower than 92.5%. The water film could increase the collection efficiency of the fine particles and prevent the fine particles cross through the holes and the slots. The hole-slot-structured FRP collectors were appropriate for the working conditions of high SCA and long residence time. When the water film in the hole and slotted structures was incomplete, the fine particles could pass through the collector plate to form secondary back-mixing.
Implications: The wet ESPs are used most widely among the advanced treatment technologies of the fine particles’ removal. The rigid glass fiber reinforced plastics (FRP) collectors modified with hole-slotted structures were used to enhance the water film distribution over their surfaces. The hole-slot-structured FRP collectors were appropriate for the working conditions of high SCA and long residence time. When the water film in the hole and slotted structures was incomplete, the fine particles could pass through the collector plate to form secondary back-mixing.
Introduction
The fine particles (diameters less than 2.5 μm) emission has been considered a significant pollution problem. The fine particles formed by coal-fired power station boilers in China are one of the main emission sources of fine particles (He et al. Citation2015; Li et al. Citation2018). Large boilers of steelworks and industrial stoves, heating boilers used in the urban heating seasons, and small stoves for daily life are also the main emission sources (Zhao et al. Citation2014). In addition, biomass fuel also produces a large number of particles and gaseous pollutants because of the simple combustion conditions (Just, Rogak, and Kandlikar Citation2013; Shen et al. Citation2012; Ueda, Tomimatsu, and Kagami Citation2001; Yamamoto et al. Citation2018). With characteristics of irregular shape, large specific surface area, and light weight, the fine particles can remain suspended in the air for a long time. Their surfaces are easier to absorb the toxic heavy metals, acidic oxides, organic pollutants, bacteria, and viruses, etc. in the air (Cox et al. Citation2017; Rashdi et al. Citation2015; Sun et al. Citation2015; Zhao et al. Citation2013). And due to their smaller diameter, the fine particles could penetrate the human body’s respiratory defenses system and be deposited in the alveoli through breath which could cause big effect on human health (Cox et al. Citation2017; Tang et al. Citation2016; Tao et al. Citation2014). Besides, the fine particles have important implications on the air visibility (Jung et al. Citation2011; Tang et al. Citation2017; Tian et al. Citation2015) and the ecosystem (Vassura et al. Citation2014). The fine particles are closely related to the large scale and long period of severe haze weather in China, that become environmental pollution problem attracting extensive attention from the whole society (Gao et al. Citation2015; Li et al. Citation2016; Yang et al. Citation2016).
Along with the increasingly stringent dust emission concentration standards for the power plant and industrial stoves (Yuan et al. Citation2017) and the challenge of market competition, using a single traditional fine particles removal technology is difficult to satisfy the current exhaust laws. Hence, it is increasingly urgent to develop the collaborative removal technology (Lee et al. Citation2013) in ways of upgrading the traditional ESPs or developing a new technology of multi-field coupling. At present, the technologies enhancing the removal of PM2.5 include the following methods: agglomeration technology (Xu et al. Citation2009; Zhang and Liao Citation2015), low-low temperature ESPs (Hu et al. Citation2017; Li et al. Citation2017; Shou et al. Citation2016), hybrid ESP/BAG devices (Shi et al. Citation2016), wet ESPs (Zheng et al. Citation2018) and so on. Among the above-advanced treatment technologies to remove the fine particles, the wet ESPs are used most widely (Yu Citation2017).
The wet ESPs technology used the water film to clean the collected dust and that could avoid the reentrainment of dust effectively. Besides, there is no limit to the resistance of the dust. The wet ESPs also have high corona current and obvious effect of particle agglomeration which exhibits obvious technical advantages in fine particles’ removal. Currently the materials of the collectors used basically includes: 316 L stainless steel, dual-phase steel, conductive FRP, carbon, or silicon fiber, organic fiber and so on (Bayless et al. Citation2005; Chang et al. Citation2011; Jiang et al. Citation2014). In general, honeycomb or plate type structure is used to make the collectors surfaces act as the carrier of water film (Ding et al. Citation2015). At present, the rigid collectors still have some problems to improve, such as consuming massive washing water, non-uniform distribution of washing water and shutdown during washing operation, and so on. The poor tensioning and fixation of the fiber collectors could cause themselves deformation and vibration. And then the space between the electrodes could change. That could cause the instability of the electric field. Therefore, the research on modification methods for CRC which can present well-wetting properties with ultra-low water consumption has become a hot topic. That is supposed to solve the traditional problems.
To solve the problem of the non-uniform distribution water film over the collectors’ surfaces, we had tried to use the FRP with hole-slotted structures in the surfaces as the collectors. The hole-slotted structured surfaces were extensively used in industrial-structured packings. The previous studies mainly focused on the spreading patterns of liquid films on their surfaces, the conversion of the spreading patterns, and the effects of the falling film flow on the heat and mass transfer efficiency and so on (Aleksandr et al. Citation2009; McGlamery Citation1988). Recently, the hole-type plates were used as collecting electrodes (Ehara et al. Citation2017) or guidance plates (Wen, Krichtafovitch, and Mamishev Citation2015) in the ESPs in the diesel engines. The perforated plates were used as the collecting electrodes to capture the most dust in a laboratory-scale advanced hybrid particulate collector and the flue gas flow direction was perpendicular to the perforated plates (Tu, Song, and Yao Citation2017). In wet ESPs, the flue gas flow direction was parallel to the collecting electrodes and few reports show the hole-slotted structured surfaces acting as the collectors.
In this study, the effects of the hole-slot patterns and the operating parameters on the particles removal performance in wet ESPs using the hole-slotted structured collectors were investigated at the laboratory scale. The voltage-current characteristics and the energy consumption among the different patterns of collectors were discussed. In addition, the effects of the water film on the particles classification collection efficiency and the effects of the applied voltage and the SCA on the relative collection efficiency were investigated and discussed.
Materials and experimental methodology
Experimental system
A schematic diagram of the experimental system is shown in . The system mainly consisted of the wet ESPs shell, a high-voltage power supply and control system, a water film distribution system, a simulated flue gas system and an induced draught system.
Figure 1. Schematic diagram of the experimental apparatus: 1, wet ESPs shell; 2, DC power; 3, insulation; 4, rotameter; 5, water pump; 6, water tank; 7, water distribution pipe; 8, collecting electrode; 9, discharge electrode; 10, wind shield; 11, heater; 12, aerosol generator; 13, particles feed port; 14, induced draft; 15, regulating valve; 16, export sampling port; 17, inlet sampling port
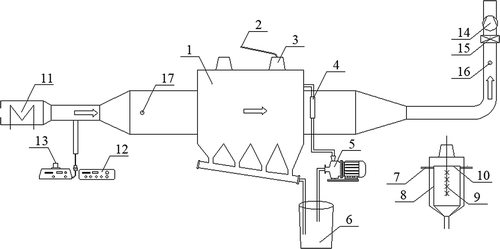
The distance between the discharge and collecting electrodes was 200 mm, each collecting electrode with thickness of 2.5 mm had an area of 750 × 500 mm2, and the total useful length was 2 m. The total collector areas were 3 m2. The hole-slotted collectors were connected to the nylon fabric with insulation sheet and bolts; the water pipe was wrapped in the nylon fabric (See Patent No.: CN201320818783.5 for installation details).
An adjustable DC negative voltage from 0 kV to 70 kV and a maximum current of 10 mA were generated by a high-voltage power supply. The total discharge current and applied voltage could be acquired directly from the display panel of the high-voltage supply. The negative high voltage was applied to the discharge electrodes. As shown in , the discharge electrodes were made of six parallel nichrome-barbed wire with each length of 500 mm.
The water film distribution system supplied continuous water film on the surfaces of the collectors. Then the water containing the collected particles entered the water tank to deposit. After that, the water pump pumped the supernatant circulating water to the water distribution pipe. The rotameter regulated the flow rate range of 0 ~ 160 L/hr.
During the experiments the solid aerosol generator (SAG-410, Topas GmbH, Germany) and an aerosol electrostatic neutralizer (EAN-581, Topas GmbH, Germany) supplied a suitable amount particles from the particles feed port at a predetermined rate. The compressed air carrying the suitable amount particles came into the entrance section before the wet ESPs shell. Then, the flue gas was introduced into the test section by an induced draft. The charged fine particles were transferred to the collecting electrodes and were arrested by the water film under the electric field. The residence time of the fine particles in the test section was controlled by the regulating valve adjusting the flue gas velocity. The residence time was 2.0 ~ 4.0 seconds and the SCA was about 5~20 m2/(m3/sec).
Materials
The CRC used the common Z-type collectors. The hole-slotted collectors were made of glass fiber-reinforced plastics (FRP). The patterns of the holes and slots are shown in . had staggered vertical slots with a width of 3 mm and height of 30 mm which was recorded as VS. The percentage of holes area was 13.55%. has staggered horizontal slots with a width of 30 mm and height of 3 mm which was recorded as HS. The percentage of holes area was 5.76%. has circular holes with a diameter of 8 mm which was recorded as CH. The percentage of holes area was 17.26%.
The test particles were obtained from the ESPs final stage ash hopper of a pulverized coal boiler through the screening by a supersonic wave oscillating screen (625 mesh). The number concentration of the fine particles () were tested with an electrical low-pressure impactor (ELPIVI 4.00, Dekati Ltd., Finland). The number concentration of the fly ash was kept at (4.0 ± 0.2)×105 1/cm3 during the experiments. The particles’ morphology () was measured by the thermal field emission scanning electron microscopy (SUPRA™ 55, Zeiss, Germany). The component of the fine particles tested with the X-ray fluorescence (PW4400, Thermo Scientific, USA) is also shown in .
Experimental methods
The temperature of the flue gas was measured by the type K thermocouples with the test range of −40 ~ 99°C with the measurement error of ±0.1°C. The gas velocity was measured by hot-bulb anemometer (QDF-3, Beijing testing instrument co. Ltd., China) with the test range of 0.05 ~ 30 m/sec with the measurement error of ±4%U. The mass concentration of particles entering and leaving the wet ESPs were monitored by dust meter (3012 H, Laoshan Applied Technology Research Institute, China).
The collection efficiency (η) can be derived as:
where, Cin is the mass concentration of particles entering the wet ESPs (mg/m3) and Cout is the mass concentration leaving the wet ESPs (mg/m3).
The classification collection efficiency of the particles with diameter of di (ηdi) can be derived as:
where, Nin(di) is the number concentration of particles entering the wet ESPs (1/cm3) and Nout(di) is the number concentration leaving the wet ESPs (1/cm3). di is the aerodynamic diameter of the test particles.
Results and discussion
Voltage-current (V-I) characteristics
shows the V–I characteristics of the ESP with wet hole-slotted collectors and dry CRC. The particles inlet mass concentration (Cin) was 70 mg/m3, the treatment time of the particles stayed in the wet ESPs shell was 4 sec and the SCA was 20 m2/(m3/s), the surface flowrate (F) over the hole-slotted collectors was about 40 kg/(h·m2) with the flushing water flow of 60 L/hr, the temperature (T) of the flue gas was 20°C. The applied voltage (V) increased from zero to 70 kV.
As shown in , the V–I curves of the three FRP collectors had similar tendency with exponential relationship between the applied voltage and current. With the increase of the applied voltage, the V–I curves slope of the FRP collectors increased gradually which meant that the higher the voltage increased the faster the current rose. The V–I curve slope of the CRC changed gently. The corona current of three FRP collectors was higher than that of the CRC at the same voltage. The flue gas lading particles entered the electrostatic field, the particles collided with the negative ions and then they were charged and became negative particles. The negative particles moved toward the collecting electrodes under the action of electric field force and deposited on the surface of the collecting electrodes. The electrons passed through the stacking particles to the collecting electrodes and then generated corona current. The existence of liquid film on the surfaces of collecting electrodes increased the corona current using the FRP collectors. Driven by partial pressure of water vapor and ion wind, water molecules diffused into the discharge space, and the density of water vapor molecules increased, which caused the distortion of space charge and field intensity distribution in electrostatic field. The corona onset voltage decreased because of the water film. The collision ionization in the ionization zone produced more positive ions and electrons. The thickness of the ionization zone decreased, the loss of positive ions and photons generated during the ionization process decreased. And the secondary electrons generated by the enhanced ionization caused by the collision on the metal negative discharge electrodes surfaces increased. In addition, the accumulation of negative water ions in the discharge space enhanced the electrostatic field intensity near the wet collecting electrodes (Mazen Citation1985; Wang Citation2013).These two mechanisms enhanced the corona discharge capability of wet electrostatic field.
Using the HS collectors discharged stronger compared with the CH collectors when the applied voltage lower than 40 kV. After the applied voltage excessed 50 kV, the CH collectors discharged strongest. With the increase of flue gas humidity, the number of negative water molecules in discharge space increased. Negative water molecules adsorbed on the surfaces of the discharge electrodes under the action of their surfaces electrostatic field (Macdonald and Barlow Citation1963; Schnur and Grob Citation2009). That could result in the reduction of the corona onset field intensity and the reduction of the electron escape work in the DC electrostatic field. At the same applied voltage, more electrons were excited from the surfaces of the discharge electrodes (Musumeci and Pollack Citation2012) and the corona current rose. The relative humidity of the flue gas in the electrostatic field increased with the increase of the liquid film evaporation rate over the collecting electrodes surfaces. When the applied voltage was lower, the liquid film evaporation rate was weakly affected by the applied voltage. And the flow velocity of flue gas in the electrostatic field was the crucial factor affecting the evaporation rate of the liquid film over the collecting electrodes surfaces. The near wall flow field using the HS collecting electrodes was more turbulent under the influence of the horizontal slots, which promoted the evaporation of liquid film. Therefore, the corona current was higher than that of using the CH collecting electrodes under lower applied voltage. When the applied voltage was high, the evaporation rate of liquid film was strongly influenced by the applied voltage, and the evaporation rate of liquid film increased rapidly with the increase of voltage (Wang Citation2018). The water film-forming rate on the surfaces of the CH collecting electrodes was higher than that on the surfaces of the HS collecting electrodes and that of the VS collecting electrodes was lowest. As a result, the relative humidity of the flue gas inside the electrostatic field using the CH collecting electrodes increased accordingly more. Therefore, the corona current using the CH collecting electrodes was higher than that using the HS collecting electrodes when the applied voltage increased to a certain extent.
Electric power consumption
shows the relationship between collection efficiency of the particles and corona power using the FRP collectors and the CRC. The corona power is the product of corona current and the applied voltage. The VS and HS collectors had similar variation tendency. The variation tendency of the CRC was between the VS and CH collectors when the corona power was lower than 12 W. The variation tendency of the CH collectors changed most. When the corona power was 10 W, the collection efficiency using the CH and HS collectors was higher than the CRC and that using the VS collectors was lower than the CRC. When the corona power was higher than 10 W, the collection efficiency using the FRP collectors was all higher than the CRC. When the corona power was 17.5 W, the collection efficiency using the FRP collectors was higher than the CRC. The results indicated that the particles’ collection efficiency was not only affected by the corona current but also affected by other factors such as the hole-slot patterns, and so on. When the collection efficiency was lower than 92.5%, using the CH and HS collectors could decrease the energy consumption while using the VS collectors increased the energy consumption compared with the CRC. When the collection efficiency was higher than 94.5%, using the FRP collectors decreased the energy consumption compared with the CRC.
Effects of the water film on the collection efficiency
shows the effects of the water film on the particles collection efficiency. The particles inlet mass concentration was 70 mg/m3, the treatment time of the particles stayed in the wet ESPs shell was 4 seconds and the SCA was 20 m2/(m3/s), the applied voltage used was 60kV, the surface flowrate over the hole-slotted collectors was about 40 kg/(h·m2) with the flushing water flow of 60 L/hr. The temperature of the flue gas was 20°C. The following experiments were carried out at room temperature to reduce operating variables and ensure the accuracy of ELPI measurement.
Figure 7. Effects of the water film on the collection efficiency. X1: Particles distribution using FRP collectors with dry and wet surfaces. X2: The collection efficiency of particles using the FRP collectors with wet surfaces coupling voltage. (X = a, b, c) (Cin: 70 mg/m3;SCA: 20 m2/(m3/sec);F:60 L/hr;T: 20 °C;V:60 kV)
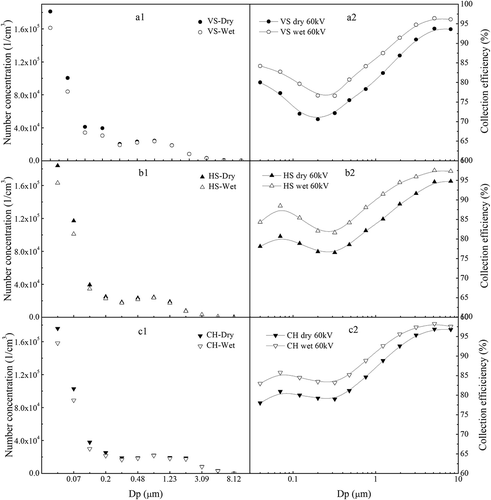
Figure 7X1 shows that the particles number concentration using the hole-slotted FRP collectors with dry and wet surfaces. As shown, the particles with diameters smaller than 0.48 μm decreased evidently than others with water film surface. The fine particles were captured by the water film when they collided with the water film which could reduce the secondary remixing.
Figure 7X2 shows the collection efficiency of particles using the FRP collectors with dry and wet surfaces coupling voltage. As shown, the FRP collectors with water film at the surfaces presented higher collection efficiency than their dry surfaces at same applied voltage. With the increase of particle diameter, the particles’ collection efficiency using the VS collectors first decreased and then increased, while the particles’ collection efficiency using the HS and CH collectors first increased, then decreased, and then increased. The particles’ collection efficiency using the VS collectors with diameter 0.1 ~ 1 μm was relatively low. The particles’ collection efficiency using the HS and CH collectors with diameter 0 ~ 0.04 μm and 0.12 ~ 0.8 μm was relatively low. The particles’ collection efficiency showed a local maximum value when the particle diameter was 0.07 μm. The effect of electrostatic field superimposed with water film on the collection efficiency of fine particles was obvious.
In order to qualitatively analyze the effect of the water film over the collection electrodes on the particles’ collection efficiency using the FRP collectors, the particles’ collection efficiency using the FRP collectors with dry surfaces was set as 100%. The particles’ contrasted collection efficiency is the specific value of the collection efficiency using the FRP collectors with wet and dry surfaces under the same working condition. As shown in , with the increase of the particles diameter, the particles’ contrasted collection efficiency using the VS and HS collectors presented bimodal M-type. The peaks using the VS collectors were located in the diameter of 0.12 μm and 0.76 μm and the peaks using the HS collectors were located in the diameter of 0.07 μm and 1.23 μm. The promotion by the influence of the water film on the smaller particles’ contrasted collection efficiency was more obvious than that on the bigger ones. While the particles’ contrasted collection efficiency using the CH collectors decreased slowly with the increase of the particles diameter. And the particles’ contrasted collection efficiency of using the VS and HS collectors was higher than that of using the CH collectors. The results indicated that the effect of the water film on the promotion of particles’ collection efficiency was finite and that was more obvious to using the VS and HS collectors than using the CH collectors.
Effects of the applied voltage on the collection efficiency
shows the effects of the applied voltage on the particles’ collection efficiency. The particles inlet mass concentration was 70 mg/m3, the treatment time of the particles stayed in the wet ESPs shell was 4 sec and the SCA was 20 m2/(m3/sec), the surface flowrate over the hole-slotted collectors was about 40 kg/(h·m2) with the flushing water flow of 60 L/hr. The temperature of the flue gas was 20°C. The applied voltage used was 40 kV and 60 kV.
Figure 9. Effects of applied voltage on the removal of particles. (Cin: 70 mg/m3;SCA: 20 m2/(m3/s);F: 60 L/hr;T: 20 °C;V: 40, 60 kV)
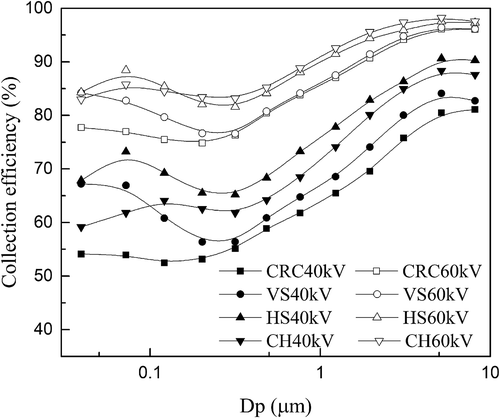
As shown in the figure, the particles’ collection efficiency using the CRC and three FRP collectors all increased when the applied voltage rose from 40 kV to 60 kV. And the collection efficiency using the wet FRP collectors were all higher than the CRC. When the applied voltage was 40 kV, the collection efficiency using the HS collectors was higher than CH collectors, the CH collectors was higher than the VS collectors with the particle’s diameter ≥0.12 μm. When the applied voltage was 60 kV, the collection efficiency using the CH collectors was higher than the HS collectors with the particle’s diameter ≥0.2 μm, the HS collectors was higher than VS collectors.
To compare the collection efficiency between the FRP collectors and the CRC, here we define a parameter that is the relative collection efficiency (RCE). The RCE is the specific value of the collection efficiency using the wet FRP collectors to that of the dry CRC at the same operating conditions. shows that with the increase of particle diameter, the RCE using the VS collectors first decreased, then increased and then decreased. While the HS and CH collectors present double peak phenomenon. Those were different to the wet penetrating polypropylene collectors (Wang et al. Citation2016). The peak of the VS was at the diameter of 1.96 μm and the RCE was higher when the diameter was smaller than 0.2 μm. The first peak of the HS was at the diameter of 0.07 μm, the CH was at the diameter of 0.12 μm, the second peak was all at the diameter of 1.96 μm. The RCE of the wet penetrating polypropylene collectors is higher for the particles with diameter between 0.1 μm and 1.0 μm. Here the RCE for particles with diameter between 0.1 μm and 1.0 μm is just between the two peaks. The RCE with applied voltage 40 kV is higher than that with 60 kV RCE for particles. The results showed that the improvement of particle collection efficiency was more obvious when the voltage was low and the effect of raising the applied voltage on the particles collection efficiency was limited when the applied voltage raised to a certain degree.
Effects of the SCA on the collection efficiency
shows the effect of SCA on particles collection efficiency and RCE. The bottom part presents the collection efficiency curves and the upper part presents the corresponding RCE. The particles inlet mass concentration was 70 mg/m3, the applied voltage used was 60 kV, the surface flowrate over the hole-slotted collectors was about 40 kg/(h·m2) with the flushing water flow of 60 L/hr. The temperature of the flue gas was 20°C. The SCA was 10 and 20 m2/(m3/s).
Figure 11. The effect of SCA on particles collection efficiency and RCE. (a) SCA:20 m2/(m3/s), (b) SCA:10 m2/(m3/s). (Cin: 70 mg/m3;F: 60 L/hr;T: 20 °C;V: 60 kV)
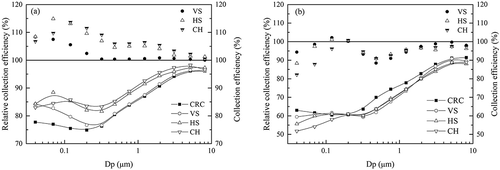
As shown in that the SCA decreased from 20 to 10 m2/(m3/s) the collection efficiency by CRC and FRP collectors all decreased sharply. The particles collection efficiency by the FRP collectors was higher than the CRC when the SCA was 20 m2/(m3/s). When the SCA was 10 m2/(m3/s), the particles collection efficiency by the FRP collectors was almost lower than the CRC. On one hand, the decrease of the SCA led to the reduction of the particle’s residence time in the test apartment. That could result in direct decrease of the particles collection efficiency. On the other hand, the inner test apartment of the wet ESPs was under negative pressure. When the SCA decreased, the flue gas speed increased accordingly, the flue gas turbulence around the hole-slotted surfaces intensified which prevented the particles from reaching the surfaces of the collectors. On the contrary, the CRC had intact surfaces without the former problems. At the same time, the phenomena indicated that the holes and slots of the FRP collectors were not full of water film.
Conclusion
The particles’ removal performance of the FRP collectors modified with hole-slotted structures in wet ESPs was studied. The water film over the hole-slotted structured FRP collectors could increase the collection efficiency of the fine particles and prevent the fine particles cross through the holes and the slots. The hole-slotted structured FRP collectors were appropriate for the working conditions of high SCA and long residence time. When the water film in the hole and slotted structures was incomplete, the fine particles could pass through the collector plate to form secondary back-mixing. When the applied voltage was increased, the collection efficiency of fine particles could only be improved to a certain extent. The performance of the HS collectors was more stable than that of the CH collectors, and the VS collectors had no obvious advantage over the fine particle’s collection.
Disclosure statement
No potential conflict of interest was reported by the authors.
Additional information
Funding
Notes on contributors
Chunyan Xu
Chunyan Xu is a postdoctoral researcher.
Hao Zhang
Hao Zhang is a Doctor Candidate.
Jingcai Chang
Jingcai Chang is a professor at the School of Environmental Science and Engineering, Shandong University, China.
Zhenlei Deng
Zhenlei Deng is an engineer at SanRong Environmental Protection Company, China.
Shan Qing
Shan Qing is a professor at the Faculty of Metallurgical and Energy Engineering, Kunming University of Science and Technology, China.
References
- Aleksandr, P., P. Nikolay, C. Vladimir, and V. Oleg. 2009. Hydrodynamics in falling liquid films on surfaces with complex geometry. Appl. Therm. Eng. 21 (1):207–13.
- Bayless, D. J., L. M. Shi, G. Kremer, B. J. Stuart, J. Reynolds, and J. Caine. 2005. Membrane-based wet electrostatic precipitation. Air Repair. 55 (6):784–91.
- Chang, J. C., Y. Dong, Z. Q. Wang, P. Wang, P. Chen, and C. Y. Ma. 2011. Removal of sulfuric acid aerosol in a wet electrostatic precipitator with single terylene or polypropylene collection electrodes. J. Aerosol. Sci. 42 (8):544–54. doi:10.1016/j.jaerosci.2011.05.006.
- Cox, L. A., (Jr.), X. B. Liu, L. H. Shi, K. Zu, and J. Goodman. 2017. Applying nonparametric methods to analyses of short-term fine particulate matter exposure and hospital admissions for cardiovascular diseases among older adults. Int. J. Environ. Res. Public Health. 14 (9):1051. doi:10.3390/ijerph14091051.
- Ding, Z. J., Z. Y. Yang, L. C. Xiao, Q. B. Zhan, and M. M. Zheng. 2015. Experimental research on performance of corona discharge and dust remove of wire-plate wet electrostatic precipitator. J. Yanshan Univ. 39 (2):177–81.
- Ehara, Y., M. Ohashi, A. Zukeran, K. Kawakami, T. Inui, and Y. Aoki. 2017. Development of hole-type electrostatic precipitator. Int. J. Plasma Environ. Sci. Technol. 11 (1):9–12.
- Gao, J. J., H. Z. Tian, K. Cheng, L. Lu, M. Zheng, S. X. Wang, J. M. Hao, K. Wang, S. B. Hua, and C. Y. Zhu. 2015. The variation of chemical characteristics of PM2.5 and PM10 and formation causes during two haze pollution events in urban Beijing, China. Atmos. Environ. 107 (april):1–8. doi:10.1016/j.atmosenv.2015.02.022.
- He, J. Y., L. Yan, Y. Lei, and X. Y. Wang. 2015. Emission characteristics of particulate matter from coal-fired power plants in china. Res. Environ. Sci. 06 (28):862–68.
- Hu, B., L. Zhang, Y. Yi, F. Luo, and L. J. Yang. 2017. PM2.5 and SO3 collaborative removal in electrostatic precipitator. Powder Technol. 318:484–90. doi:10.1016/j.powtec.2017.06.008.
- Jiang, H. T., S. G. Tian, Y. L. Fu, M. H. Jia, and Y. B. Zhang. 2014. Application of wet electrostatic precipitator in coal-fired power plants. Power Equip. 28:61–64.
- Jung, J., Y. J. Kim, S. G. Aggarwal, and K. Kawamura. 2011. Hygroscopic property of water-soluble organic-enriched aerosols in ulaanbaatar, mongolia during the cold winter of 2007. Atmos. Environ. 45 (16):2722–29. doi:10.1016/j.atmosenv.2011.02.055.
- Just, B., S. Rogak, and M. Kandlikar. 2013. Characterization of ultrafine particulate matter from traditional and improved biomass cookstoves. Environ. Sci. Technol. 47 (7):3506–12. doi:10.1021/es304351p.
- Lee, S. W., T. Herage, R. Dureau, and B. Young. 2013. Measurement of PM_(2.5) and ultra-fine particulate emissions from coal-fired utility boilers. Fuel. 108 (June):60–66. doi:10.1016/j.fuel.2011.11.059.
- Li, J. G., Y. Z. He, H. X. Liu, and H. B. Zhao. 2017. Characteristics of low-low temperature ESP technology and its application in ultra-low emissions for high-ash coal-fueled power plant. Electric Power. 50 (3):28–33.
- Li, L. J., Z. S. Wang, D. W. Zhang, T. Chen, and Y. T. Li. 2016. Analysis of heavy air pollution episodes in Beijing during 2013~2014. China Environ. Sci. 36 (1):27–35.
- Li, X. L., D. B. Zhou, J. X. Duan, W. J. Zhang, J. Z. Li, and J. B. Yan. 2018. Characteristics of particle emission from coal-fired power plants under the ultra low emission standard. Environ. Monit. China 34 (3):45–50.
- Macdonald, J., and A. Barlow. 1963. Work function change on monolayer adsorption. J. Chem. Phys. 39 (2):412–22. doi:10.1063/1.1734263.
- Mazen, A. S. 1985. Positive wire-to-plane coronas as influenced by atmospheric humidity. IEEE Trans. Ind. Appl. IA-21 (1):35–40. doi:10.1109/TIA.1985.349640.
- McGlamery, G. G. 1988. Liquid film transport characteristics of textured metal surfaces. Austin: The University of Texas.
- Musumeci, F., and G. H. Pollack. 2012. Influence of water on the work function of certain metals. Chem. Phys. Lett. 536:65–67. doi:10.1016/j.cplett.2012.03.094.
- Rashdi, S. A., A. A. Arabi, F. M. Howari, and A. Siada. 2015. Distribution of heavy metals in the coastal area of Abu Dhabi in the United Arab emirates. Mar. Pollut. Bull. 97 (1/2):494–98. doi:10.1016/j.marpolbul.2015.05.052.
- Schnur, S., and A. Grob. 2009. Properties of metal–water interfaces studied from first principles. New J. Phys. 11 (12):125003. doi:10.1088/1367-2630/11/12/125003.
- Shen, G. G., S. Y. Wei, W. Wei, Y. Y. Zhang, Y. J. Min, B. Wang, R. Wang, W. Li, H. Z. Shen, and Y. Huang. 2012. Emission factors, size distributions, and emission inventories of carbonaceous particulate matter from residential wood combustion in rural China. Environ. Sci. Technol. 46 (7):4207–14. doi:10.1021/es203957u.
- Shi, W. Z., M. M. Yang, X. H. Zhang, S. Q. Li, and Q. Yao. 2016. Ultra-low emission technical route of coal-fired power plants and the cooperative removal. Proc. Csee. 36 (16):4308–18.
- Shou, C. H., Z. F. Qi, W. Y. Xie, Z. W. Zou, C. H. Liu, M. Li, J. W. Li, X. D. Li, and W. H. Li. 2016. Experimental study on engineering application of particulate matter removal characteristics of low-low temperature electrostatic precipitator. Proceedings of the CSEE. 36 (16):4326–32.
- Sun, L. M., Z. Q. Lin, K. Liao, Z. G. Xi, and D. Y. Wang. 2015. Adverse effects of coal combustion related fine particulate matter (PM2.5) on nematode caenorhabditis elegans. Sci. Total Environ. 512-513:251. doi:10.1016/j.scitotenv.2015.01.058.
- Tang, G. Q., J. Q. Zhang, X. W. Zhu, T. Song, C. Münkel, B. Hu, K. Schäfer, Z. R. Liu, J. K. Zhang, L. L. Wang, et al. 2016. Mixing layer height and its implications for air pollution over Beijing, China. Atmos. Chem. Phys. 16 (4):2459–75. doi:10.5194/acp-16-2459-2016.
- Tang, G. Q., P. S. Zhao, Y. H. Wang, W. K. Gao, M. T. Cheng, J. Y. Xin, X. Li, and Y. S. Wang. 2017. Mortality and air pollution in Beijing: The long-term relationship. Atmos. Environ. 150:(FEB.):238–243. doi:10.1016/j.atmosenv.2016.11.045.
- Tao, Y., Y. M. Liu, S. Q. Mi, and Y. T. Guo. 2014. Atmospheric pollution characteristics of fine particles and their effects on human health. Acta Scientiae Circumstantiate. 34 (3):592–97.
- Tian, P., G. F. Wang, R. J. Zhang, Y. F. Wu, and Y. Peng. 2015. Impacts of aerosol chemical compositions on optical properties in urban Beijing, China. Particuology. 018 (1):155–64. doi:10.1016/j.partic.2014.03.014.
- Tu, G. M., Q. Song, and Q. Yao. 2017. Experimental and numerical study of particle deposition on perforated plates in a hybrid electrostatic filter precipitator. Powder Technol. 321:143–53. doi:10.1016/j.powtec.2017.08.021.
- Ueda, Y., K. Tomimatsu, and M. Kagami. 2001. Development of advanced gas cleaning system for sub-micron particle removal. 8th International Conference on Electrostatic Precipitation, Birmingham.
- Vassura, L., E. Venturini, S. Marchetti, A. Piazzalunga, E. Bernardi, P. Fermo, and F. Passarini. 2014. Markers and influence of open biomass burning on atmospheric particulate size and composition during a major bonfire event. Atmos. Environ. 82:(jan.):218–225. doi:10.1016/j.atmosenv.2013.10.037.
- Wang, X. 2018. Study on mechanism of heat and moisture transfer on electrical field and fine particles. Jinan: Shandong University.
- Wang, X., J. C. Chang, C. Y. Xu, J. Zhang, P. Wang, and C. Y. Ma. 2016. Collection and charging characteristics of particles in an electrostatic precipitator with a wet membrane collecting electrode. J. Electrost. 83:28–34. doi:10.1016/j.elstat.2016.07.007.
- Wang, X. H. 2013. Enhancement mechanism and process of water on particle removal in electrostatic filed. Beijing: Tsinghua University.
- Wen, T.-Y., I. Krichtafovitch, and A. V. Mamishev. 2015. Reduction of aerosol particulates through the use of an electrostatic precipitator with guidance-plate-covered collecting electrodes. J. Aerosol. Sci. 79:40–47. doi:10.1016/j.jaerosci.2014.10.002.
- Xu, F., Z. Y. Luo, B. Wei, L. N. Wang, X. Gao, M. X. Fang, and K. F. Cen. 2009. Electrostatic capture of PM2.5 emitted from coal-fired power plant by pulsed corona discharge combined with DC agglomeration. 11th international conference on electrostatic precipitation, Hangzhou.
- Yamamoto, T., K. Yokoo, A. Kusu, and J. Tatebayashi. 2018. Highly efficient particulate matter removal by a fluidized-bed-type device operated in continuous regeneration mode. Powder Technol. 323:86–94. doi:10.1016/j.powtec.2017.09.035.
- Yang, X. W., Y. Zhou, S. Y. Cheng, G. Wang, and X. Q. Wang. 2016. Characteristics and formation mechanism of a heavy winter air pollution event in Beijing. China. Environ. Sci. 36 (3):679–86.
- Yu, W. J. 2017. Research of application and development for dust ultra-low emission technologies in coal-fired plants. Chem. Ind. Eng. Progr. 36 (b11):428–35.
- Yuan, X. L., M. F. Zhang, Q. S. Wang, Y. T. Wang, and J. Zuo. 2017. Evolution analysis of environmental standards: Effectiveness on air pollutant emissions reduction. J. Clean. Producti. 15 (149):511–20.
- Zhang, W. F., and C. L. Liao. 2015. Research review on agglomeration technologies of submicron particles PM2.5 in China. J. East China Jiaotong Univ. 032 (4):124–30.
- Zhao, H. J., H. Z. Che, X. Y. Zhang, Y. J. Ma, Y. F. Wang, H. Wang, and Y. Q. Wang. 2013. Characteristics of visibility and particulate matter (PM) in an urban area of Northeast China. Atmos. Pollut. Res. 4 (4):427–34. doi:10.5094/APR.2013.049.
- Zhao, S. Z., H. H. Yi, X. L. Tang, F. Y. Gao, and Y. R. Zuo. 2014. Source and harm of air fine particulate matter pollution and control strategies. Sci. Technol. Rev. 32 (33):61–66.
- Zheng, C. H., Y. F. Wang, X. F. Zhang, Z. D. Yang, S. Liu, Y. Guo, Y. Zhang, Y. Wang, and X. Gao. 2018. Current density distribution and optimization of the collection electrodes of a honeycomb wet electrostatic precipitator. RSC Adv. 8 (54):30701–11. doi:10.1039/C8RA04765K.