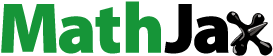
ABSTRACT
The efficiency of the composting process for tomato residues and chicken manure was estimated after monitoring of the rotary composting system. Physicochemical parameters and Compost Quality Index were evaluated. The tomato residues (leaves, stems, and some green and damaged fruits) were collected, cut into small pieces, moistened approximately (60–65%), and mixed with 20% chicken manure then distributed into three rotary drum bioreactors. The obtained results showed that, the temperature above 50°C was maintained for more than two days. Carbon: nitrogen (C:N) ratio was reduced from 30:1 to 19.13:1. The pH value ranged between 7 and 8.80 during the composting process, while the electrical conductivity (EC) ranged between 2.67 to 4.53 dS/m. Both compost quality parameters (Dewar and germination index) and (Solvita-CO2 and Solvita-NH3) indicated that, the final compost is stable and mature.
Implications: The idea of this research revolves around assessing the maturity and stability of the compost resulting from mixing tomato residues with chicken manure, using a rotary drum bioreactor which is characterized by reducing the time of the active phase to several hours or days instead of weeks or months. Several tests related to the maturity and stability of mixture have been used to judge its quality. Also, many parameters related to this topic were monitored and discussed with many previous researches to determine the importance of benefiting from mixing the different wastes together and obtaining a good fertilizer ready for application as an agricultural substrate or a soil conditioner.
Introduction
Organic waste generation is increasing worldwide and strategies must be developed and optimized for their environmental impact and cost. It is an urgent task to develop high efficient, inexpensive and environmentally friendly conversion technologies (Wang, Zeng, and Cao Citation2018). Molecules of biomaterials are promising materials, with low toxicity, good biodegradability, and environment friendliness (Zheng, Olayinka, and Bin Citation2018). One of the most widely used technologies is composting (Togun, Akanbi, and Adediran Citation2004). It has a high capacity for transforming various organic wastes into a valuable product useful for agricultural purposes (Jindo et al. Citation2012), as horticultural growth media to improve the soil structure by increasing soil organic matter and soil fertility (Gao et al. Citation2010; Togun, Akanbi, and Adediran Citation2004).
The rotary drum bioireactor is one of the promising devolved composting systems which have been established worldwide as having positive advanced features (Vuorinen and Saharinen Citation1997). It offers aeration, agitation and mixing of the compost to produce a reliable and uniform end product (Kalamdhad and Kazmi Citation2009).
Poultry plays a major role in developing countries, as is production relatively inexpensive and widely available (Windhorst Citation2008). The uncontrolled decomposition of chicken manure releases NH3, N2O, and CH4 in the atmosphere, posing many threats to the environment. Also, these wastes can contaminate soil and water if applied periodically as a direct organic soil amendment. Kulcu (Citation2014) investigated that, the optimum mixing ratio that can be applied in the fertilization process of greenhouse tomato residues, wheat straw and dairy manure for fertilization is 60% greenhouse tomato waste, 30% dairy manure and 10% wheat straw. Although the addition of mature compost to agricultural soil has several advantages, immature compost addition creates a suitable environment for anaerobic microbes because microbes deplete oxygen in the root area and continue to digest the organic materials available anaerobically (Butler et al. Citation2001; Mathur et al. Citation1993). Consequently, some intermediated toxic compounds are produced, such as hydrogen sulfide (H2S) and nitrite (NO2), which can limit the plant growth or adversely affect seed germination (Butler et al. Citation2001; Cambardella, Richard, and Russell Citation2003). Therefore, compost stability and maturity are often determined before any recommendation is made not only to assess the quality of the compost product, but also indirectly measuring the efficacy of the compost technology itself (Arnold and McCumber Citation2015). Also, successful composting process requires the monitoring of influential factors and control the composting progress such as compost temperature, moisture content, aeration C:N ratio, pH, and EC, where reflects the level of salinity in a compost product (Gao et al. Citation2010). Therefore, the aim of this study is to investigate the evolution of some physico-chemical, biological parameters and assess the maturity and stability of the composted tomato residues mixed with chicken manure using a rotary drum bioreactor.
Materials and methods
Collection of plant residues
Greenhouse-tomato, fresh plant residues (stems, leaves, and some green and damaged fruits) were collected from various farms of Riyadh city, Saudi Arabia. Preceding the composting, tomato residues were obtained at an average moisture content (MC) of approximately 90%. Then they were spread out on the ground to dry for three days before being chopped using a gas motor-powered shredder (Model FYS-76 Shredder, China Mainland) to promote better aeration and moisture control. In order to enhance the microbial degradation process, the residues were grinded to reach a suitable particle size (≈ 1–2 cm). The grinded residues were left on the floor for an additional two consecutive days for more drying (MC 15%), than tightly bagged and transported to the Biology Lab at the Educational Farm, King Saud University camps. For making compost tomato plant residue (80%) was mixed with 20% chicken manure, as a nitrogen source, and MC of the mixture was adjusted to be about 60%. The main chemical characteristics of the raw materials used in this study are listed in .
Table 1. Chemical characteristics of the raw materials used in the study (mean ± SD, n = 3)
Composting was performed in three identical pilot-scale rotary drum bioreactors (200 L each) at the Educational Farm, Agric. Eng. Dept., King Saud University. Each bioreactor was consisted of a steel barrel (), designed to provide space for 50 kg (wet bases) of mixture plus 25% of the volume as head space. The barrel has an inner diameter of 58.5 cm, length of 91.4 cm, wall thickness of 0.09 cm and having a door of 50 cm × 40.5 cm that was made for loading, unloading, sampling, and cleaning. The outer surfaces of each bioreactor were insulated with a layer of 2.5 cm-thick glass wool. Each bioreactor rotated horizontally around a fixed axis (i.e., steel tube, 5 cm outer diameter) at 3 rpm by using a 0.25 HP MEZ electric motor (model no. 220–380-3, Zhejiang, China). The perimeter of the fixed tube in each bioreactor included on-line holes distributed longitudinally in the upper surface of the tube for measuring the compost temperature, and on the lower surface for aeration. The air was provided continuously from an air compressor to the central fixed tube, which the barrel rotated around, at the chosen airflow. The regulated air, then passed through a flow meter to control and measure the volume of the air entering each bioreactor. The flow meter has a scale that can control the air volume at 0.001 to 0.025 m3/min. Thermocouples (Copper-constantan-type T-Cole Parmer, Chicago, IL, USA) were used to measure the temperature inside and outside each bioreactor. The MC of the mixture was adjusted to about 60% at the beginning of the composting process, and C:N ratio at ≈ 30:1. After that, the adjusted mixture was transferred to three bioreactors (filled up to 75% of the total volume).
Stability and maturity measurement
Physical and chemical analyses of composted materials
Representative samples of mixture were taken every 2 and 8 days (during the active and curing stage respectively (6 days active stage and 30 days curing stage) for analyzing and measuring the following parameters: composts temperature, MC, pH, EC, total organic matter (TOM), and C:N ratio throughout the composting time. The temperature of the compost was measured every 10 min using three thermocouple sensors inserted inside each bioreactor, connected to a data logger; the average of the three bioreactors represented the evolution of compost temperature. The gravimetric method (BIS Citation1982) was used to determine the moisture content by drying the compost sample (at 105°C for 24 h) and weighing the loss. The pH was measured by using a Jenway digital pH meter, model 3510 (United Kingdom). EC was measured by filtering the compost sample through Whatman filter paper No. 42 using a digital conductivity meter. Organic carbon (OC) and organic matter (OM) contents were calculated as follows: OC (%) = OM (%)/1.8, OM (%) =100-Ash (%) (Abdullah and Chin Citation2010). Losses of organic matter (OM) and total nitrogen (N) were calculated from the initial (X1) and final (X2) ash contents and the initial (N1) and final (N2) concentrations, according to the following equations (Paredes et al. Citation2000):
The content of ash was determined as the difference between the dry matter content and the gravimetric loss-on-ignition produced by ashing of the previously dried samples, at 550°C for 8 hrs. (EN 13,039 1999). Total nitrogen (%) was determined by the Kjeldahl digestion method (EN 13,654–1 2001).
California compost quality index protocol
Stability and maturity of compost cannot be described by a single parameter; at least, two or more specific tests will provide the highest level of confirmation. Generally, the evaluation of compost quality is done according to a procedure summarized by the California Compost Quality Index (Buchanan et al. Citation2001), where final product can be described as very mature, mature and immaturecomposts according to the reading of their maturity index. This is done specifically by three levels of decision as shown in .
Solvita maturity test
For Solvita test, moisture content of the samples was adequate as determined by a squeeze test. The sample carefully fills the Solvita jar to the fill line. The samples were incubated in 200 ml containers with a Solvita reactor for a 4-hour incubation period at 20–25°C following the manufacturer’s instructions (WERL-2018, Guide to Solvita Testing for Compost Maturity Index). Then, the extent of color change was measured using DCR (Digital CO2 & NH3 Color Reader, Solvita®) and the maturity index (MI) was calculated as described in the manufacturer’s protocol.
Dewar (self-heating) test
For Dewar test (WEL Citation2009), the samples were stored at 4°C until use. Each sample was kept in a flask for six days, then the maximum temperature and the corresponding room temperature were recorded, and consequently, the temperature difference was estimated for each sample.
Germination percentage and germination index
Germination index (GI) was determined using an adaptation method described by Zucconi et al. (Citation1981b). Ten grams of screened sample was mechanically shaken with 100 ml of distilled water for one hour in the incubator shaker (IKA® KS 4000i control, Germany/Deutschland). The suspension was mixed for 15 min at 3000 rpm, and the supernatant was filtered through a Whatman filter paper No. 6. Ten seeds of white radish (Raphanus sativus) were placed on filter paper Whatman No. 1 in sterile Petri dishes (10 cm diameter). The seeds were scattered with optimum distance between them in each dish. About 4.0 ml (divided into two intervals) of the tomato composts extract was pipetted into a Petri dish. 4 ml of distilled water (added on two intervals) was used as a control and the aqueous extract was also sterilized through filtration at 0.45 µm pore size. The test was run in three replicates. Then the Petri dishes were covered with the lids and incubated at 25°C in the dark inside the incubator (Binder, USA) for 5 days. At the end of the 5th day, the percentage of seed germination in the tomato composts extract was compared with that of the distilled water control. The radish seeds were examined for germination, where germination was sure as any protrusion through the seed coat (Bazrafshan et al. Citation2016). Treatments were evaluated by counting the number of germinated seeds and measuring the length of the roots (Gao et al. Citation2010). The percentage of GI for evaluating phytotoxicity was calculated based on relative seed germination (SG) and root elongation (RE) was calculated according to (Zucconi et al. Citation1981b) as follows:
Statistical analyses
Statistical analysis was performed to compare variations in compost properties using SAS ver. 9.0 (SAS Institute, Cary, NC, USA). Data are presented as means (SEM) and analyzed by one-way analysis of variance (ANOVA), with the least significant difference (LSD) test at p_0.05.
Results and discussion
Temperature of the mixture
The temperature of the mixture started to increase rapidly after the creation of composting conditions and reached 53°C on the first 2 days, and reached its maximum value (63°C) after 66 hours of operation due to the faster breakdown of the available organic matter (OM) and nitrogenous compounds through microbial reactions (Petric, Helic, and Avdic Citation2012). Starting at ambient temperature, the compost can reach 40°C – 60°C in less than two days depending on the composition and environmental conditions (Young, Rekha, and Arun Citation2005). Then, it continued over 53°C for more than two successive days (). This high temperature is important during the composting process, as theoretically, they could help destroy many pathogenic micro-organisms, weed seeds and weed propagules, thereby reducing the risk to human, animal and plant health (Brinton and Droffher Citation1994). The cooling stage started when the compost temperature started to decrease below 55°C after 80 hours. At the end of the cooling stage, temperature fluctuations became limited and temperatures remained largely constant until the end of the experiment. This is the final stage of the composting process and is called the curing or maturation stage; it takes a long time to produce humus and stable organic end product.
The level of microbial heat generation due to tomato residue and chicken manure degradation was reduced obviously after the temperature reached 63°C. Hence, this temperature may have surpassed the optimum conditions for the thermophilic microorganisms and, as a result, microbial growth and possibly microbial activity declined, resulting in decreased bioreactor temperature (Ghaly, Alkoaik, and Snow Citation2006). Several researchers have tried to define the optimal temperature for composting. Mac-Gregor et al. (Citation1981) and Bach et al. (Citation1987) indicated that, the temperature optimum for biological degradation during the composting process in the range of 52–60°C. Leton and Stentiford (Citation1990) proposed the optimum operating temperatures in the range of 45–55°C with an initial period above 55°C to sanitize materials. Also, it was recorded by Nakasaki, Shoda, and Kubota (Citation1985) that, the optimal temperature for composting process as a whole is from 35°C to 55°C. Therefore, generally speaking, moderate is favored rather than extreme thermophilic temperatures during composting (Mac-Gregor et al. Citation1981). These results in agreement with the recommendations of the Guidelines for compost quality (Canadian Council of Ministers of the Environment Citation2005). As the OM became more stabilized, the microbial activity and the OM decomposition rate decreased, and the temperature in the compost gradually dropped, reaching 27°C (after 120 hours) owing to a reduction in bioavailable nutrients (Gao et al. Citation2010). Generally, decreasing temperature in the later stages of the composting process points to the stabilization of decomposed organic portions into compost (Manohara and Belagali Citation2014).
Moisture content
The optimum MC of the compost is a vital factor for the microbial decomposition of organic waste. MC remained approximately between 60 and 63% during the active period of composting, which considers the optimum range of microbial activity (Cabañas-Vargas et al. Citation2005; Haug Citation1993), while decreased to reach 57.5% during the curing period. In general, the optimum MC in an aerobic composting process is around 40–60%, which is favored during the composting process. The initial MC above 60% is proper for an acceptable composting (Elcik, Zoungrana, and Bekaraki Citation2016). However, excessive MC leads to a lower rate of oxygen, resulting in anaerobic conditions, which decrease OM degradation rate as well as promotes bad odor formation owing to the release of volatile compounds such as carbon and hydrogen sulfide (Manohara and Belagali Citation2014).
pH
During the active stage, pH value was about 7 in the first two days, and decreased less than 7 due to the production of organic acids and high level of CO2 derived from the intense fermentation carbohydrates (Estevez-Schwarz et al. Citation2012). Afterward, the pH began to rise and reached 9.5 in the thermophilic period. This increase results from the release of ammonia due to the start of the proteolytic process (De Nobili and Petussi Citation1988). It decreased thereafter at curing stage to reach 8.8 at the end of the composting process due to the formation of NH3 (Bazrafshan et al. Citation2016; Gao et al. Citation2010; Liao et al. Citation1996) and mineralization of organic nitrogen (Bustamante et al. Citation2008; Wong, Mak, and Chan Citation2001). This finding is in accordance with the recent study by Elcik, Zoungrana, and Bekaraki (Citation2016) who reported that the pH increases over time as a result of microbial activity that caused the production of ammonia during ammonification and mineralization of organic nitrogen. Young, Rekha, and Arun (Citation2005) indicated that, the pH of the compost has been observed to vary with time, showing an initial drop and then increasing to between 8.0 and 9.0, finally leveling off between 7.0 and 8.9. During the curing stage, the pH remained in the alkaline range may due to the mineralization of organic N that leads to NH3 emission and depletion (Bazrafshan et al. Citation2016). Generally the pH tends to be alkaline in a mature compost (Song, Li, and Jia Citation2014; Young, Rekha, and Arun Citation2005). As long as the compost stays aerobic, the buffering has been proved sufficiently within the compost to allow the pH to stabilize at an alkaline level (Young, Rekha, and Arun Citation2005).
Electrical conductivity (EC)
Electrical conductivity (EC) is one of the most important physical parameters identifying conductance of liquid solutions. It is an indicator of soluble salt content, where it reflects the level of salinity in a compost product which suggests the possibility of phytotoxic or phyto-inhibitory effects (Gao et al. Citation2010). High levels of salt in a compost can result in a reduction in crop yields when salt concentrations near the root zone accumulate to a level at which the root can no longer extract sufficient water from the soil-compost solution. So it is very important to measure the EC in compost because, high salt levels may injure plants, with seedlings and transplants being most susceptible to injury (Sullivan et al. Citation2018). On the other hand, more soluble salts are soluble nutrients, so compost with a high salt concentration may be a good source of nutrients when applied at a low rate (Sullivan et al. Citation2018). An acceptable EC for compost used in field situations depends on soil EC prior to application, the compost application rate, depth of compost in corporation (tillage), soil texture, and irrigation water management (Sullivan et al. Citation2018). EC during the composting process ranged from 2.67 to 4.53 dS/m. The increasing in EC in the curing stage might be due to the net loss of weight and release of soluble salts through decomposition activity and degradation of organic matter during the composting process (Avnimelech et al. Citation1996; Bazrafshan et al. Citation2016; Singh and Kalamdhad Citation2013). As well it can be considered due to the increase in mineral cation concentration that it is not attenuated by salt leaching or by binding to stable organic complex and majority due to loss of organic matter (Francou, Poitrenaud, and Houot Citation2005; Gao et al. Citation2010). Some other studies believe that, because the mature compost is dark black due to its high carbon content and the carbonaceous materials are conductive materials. This result in agreement with Wang et al. (Citation2019a) when they reported that, the ultra-black materials with excellent performance are carbon materials with high EC, and when these materials are heated, their temperature rises, causing changes in their electrical resistance (Chen et al. Citation2020; Zheng, Olayinka, and Bin Citation2018). Also, Wang et al. (Citation2019b) reported that, graphene or carbon based materials have unique properties, such as high EC and superior mechanical flexibility. While, Zhou, Feng, and Chia-Yun (Citation2019), reported that, the EC may increase due to the addition of some nanomaterials or decrease the resistivity by adding some chemical elements to the substrate (Rui et al. Citation2019). As well, the result of this study is in agreement with Hemidat et al. (Citation2018) and Khater (Citation2015) who reported that the EC varied from 2.58 to 4.14 dS/m, and Brinton (Citation2000) who reported that EC varied from 2.0 to 4.0 for growing media. Irvan et al. (Citation2018), reported that, EC increased to reach 7 dS/m at day 20 before to decrease and become constant at 4.72 dS/m at day 40.
For commercial vegetable crop production, the obtained EC result of tomato compost is suitable, where most of the commercial tomato and cucumber cultivars are sensitive to moderate salinity levels up to 2.5 dS/m, without significant reduction of yield (Abu-Zinada Citation2015; Singh, Sastry, and Singh Citation2012). On the other hand, higher EC values might reveal the degree of compost salinity, indicating that its possible phytotoxicity influences the growth of vegetable plants if applied to the soil (Madan et al. Citation2012).
Carbon to nitrogen (C:N) ratio
The C:N ratio is used to describe the organic waste decomposition and compost quality with respect to OM and N cycling. Several investigations indicated numerous ideal C:N ratios ranging from 12:1 to 25:1 for compost quality (Estevez-Schwarz et al. Citation2012; Tiquia Citation2010). For compost with a high C:N ratio (more than 40), the microorganisms take much time to break down waste for N deficiency, reducing composting performance (Escobar and Solarte Citation2015), while compost with a low C:N ratio causes ammonium toxicity (Bazrafshan et al. Citation2016). In the present study, the C:N ratio of mixture decreased through the active stage from 30.01 to 28.22. While, in the curing stage it decreased gradually to reach 19.13 at the end of composting period. According to Singh et al. (Citation2009), C:N ratio of the final compost within 10–15 represents the stabilized compost and the C:N ratio between 15 and 20 is commonly accepted (Kayhanian and Tchobanoglous Citation1993). While, C:N ratio less than 20 represents matured compost (Heerden et al. Citation2002), which was achieved in the present study. According to Bernal et al. (Citation1998); Chefetz et al. (Citation1998); and Bazrafshan et al. (Citation2016), the reduction in the C:N ratio during the composting process of the mixture was attributed to the transformation of carbon to CO2, followed by a decrease in organic acid concentration and an increase in the N content per unit material. Generally, the C:N ratio of compost should be about 20 to avoid N immobilization and to simplify the release of mineral N for crop use when the compost is applied to the soil (Allison Citation1973). Compost characteristics of the studied parameters during composting stages are shown in .
Table 2. Compost characteristics during active and curing stages (mean ± SD, n = 3)
Solvita testing guide
The result of the Solvita test of the composted sample of the mixture was 7.23 out of 8 for CO2, and 4.17 out of 5 for NH3. The maturity index (MI = 7) is calculated by reading both probes and determining the interrelation of the two indicators according to the manufacturer’s protocol. According to WERL (Citation2018) and Buchanan et al. (Citation2001), the interpretation of the Solvita test result represents that the compost is very mature with low ammonia. More details of the Solvita test are shown in .
Dewar self-heating test
The Dewar or self-heating test is a valuable method for testing the maturity of compost (Estevez-Schwarz et al. Citation2012). The results of Dewar flask temperature verses time () showed that, there were small differences between the temperatures inside and outside of the Dewar flask during the 6 days, ranged between 1.2 to 1.6°C (˂ 10°C). These results indicated that the compost product is finished and mature (WEL Citation2009).
Table 3. Result of Dewar or self-heating test of the final compost product (mean ± SD, n = 3)
Germination percentage and germination index
Germination index (GI) is one of the sensitive parameters for evaluating the toxicity and the degree of compost maturity (Tiquia, Tam, and Hodgkiss Citation1996; Zucconi et al. Citation1981b). The germination percentage of white radish seeds (Raphanus sativus) in the control and in the compost runs during the 5 days are presented in . Most of the seeds had germinated in the control and in the compost runs, particularly over the test period. The relative reduction in germination seeds during the earlier germination periods might be owing to the release of some concentrations of ammonia, which influences the seed zygote’s sensitivity during its earlier germination period (Asgharipour and Sirousmehr Citation2012; Ells, McSay, and Workman Citation1991; Wong Citation1985). To assess the response of white radish seeds to the toxicity of the compost extract, a GI based on relative seed germination and the relative root elongation index was used (Tiquia, Tam, and Hodgkiss Citation1996). They reported that, GI is a sensitive indicator of compost maturity and phytotoxicity, where GI values lower than 50% indicate high phytotoxicity, values between 50 and 80% point to moderate phytotoxicity, and values more than 80% indicate nonappearance of phytotoxicity. However, phytotoxicity may occur with stable or mature composts due to substances which are not removed in the composting process, like heavy metals, and persistent herbicides (Research report Citation2005). The GI value of white radish seeds, which germinated on the tomato compost at the end of the germination period, is 74.23% (), indicated that, the tested sample of the compost is nottotally free of phytotoxicity but low phytotoxicity is present. This result is in agreement with the earlier findings obtained by the Zucconi research group (Zucconi et al. Citation1981a, Citation1981b; Zucconi, Monaco, and Forte Citation1985) as well as the later findings of Emino and Warman (Citation2004), Barral and Paradelo (Citation2011) and Estevez-Schwarz et al. (Citation2012).
Table 4. Radish seeds germinated during the germination period
Table 5. Quality indicators results of the compost
The summary results of the final compost quality indicators are shown in . It is clear that the C:N ratio 19.13:1 meets the first judgment decider of compost quality as stated by Buchanan et al. (Citation2001). Moisture content (MC) is also in the optimum range for microbes. The second decider of compost quality is the stability test (Dewar). The result of Dewar test indicated that the compost is a finished and mature (max. temperature ˂ 10), while the third decider is the maturity test (GI). The result of the GI test indicated that moderate phytotoxicity is present. According to the compost quality index protocol, tested compost quality is “Good”. A similar conclusion is also reached using the results of Solvita tests. Stability test (Solvita-CO2) and maturity test (Solvita-NH3) showed that the final compost is mature. Therefore, our recommendation is to extend the maturation period to increase the quality of the final compost.
Conclusion
The final results showed that the maximum temperature of the compost reaches 63°C by the 5th day and it continued at over 50°C for more than two days. The C: N ratio decreased from 30:1 to 19.13:1 at the end of the composting time. The pH value ranged between 7.0 to 8.8, while EC ranged between 2.67 and 4.53 dS/m. The Solvita tests for CO2 and NH3 were 7.23 and 4.17 respectively. The Dewar test temperature ranged between 1.2–1.6°C. The presence of a small amount of ammonia inhibited seed germination and root elongation (GI = 74.23%). The physiochemical and both compost quality parameters (Dewar and GI) and (Solvita-CO2 and Solvita-NH3) were indicated that, the final compost is mature and has low phytotoxicity. Therefore, according to the results obtained in this study, the final compost product is stable and mature and needs a further maturation period to become ready for application as an agricultural substrate or a soil conditioner.
Acknowledgment
The authors extend their appreciation to the Deanship of Scientific Research at King Saud University for funding the work through the research group project No. RGP-VPP-134.
Disclosure statement
No potential conflict of interest was reported by the authors.
Additional information
Funding
Notes on contributors
Mohamed A. Rashwan
Mohamed A. Rashwan, Assistant Professor, Department of Agricultural Engineering, College of Food and Agricultural Sciences, King Saud University, Saudi Arabia. Associate Professor, Department of Agriculture and Biosystems Engineering, Faculty of Agriculture, Alexandria University, Egypt. I did postdoctoral studies at a University of Alexandria, I hold a doctorate from Kagoshima University, Japan, in 2005 in the field of agricultural engineering. I am interested in working in environmental engineering fields such as compost production and greenhouse cooling. I also have an interest in solar energy and its uses in agriculture. I supervise a number of Master’s theses. I have published and judged many research papers and authored many books in the field of agricultural engineering.
Fahad Naser Alkoaik
Fahad Nasser Alkoaik, Professor and head of department of Agricultural Engineering, College of Food and Agriculture Sciences, King Saud University. The Main Investigator of a project titled “Design and Feasibility Study of Farm Scale Composting Unit for the Sustainable Utilization of Horticultural Wastes in the Production of Alternative Growth Substrates for Vegetables in Greenhouses.” Supervising of a number of Master’s and Doctoral Theses. Publishing and judging many research papers and authoring many books in the field of agricultural engineering. Attending many conferences at home and abroad.
Hesham Abdel-Razzak Saleh
Hesham Abdel-Razzak Saleh, Professor of Vegetable Crops Department, Faculty of Agriculture, Alexandria University, Egypt. Ph.D. in Agricultural Sciences (Dept. of Biotechnology and Biochemistry, 2002), Faculty of Agriculture, Miyazaki Univ., Miyazaki, Japan. He worked for 7 years at The College of Food and Agriculture Sciences, King Saud University, Vegetable Crops Department. Co-Investigator of project under National Science, Technology and Innovation Plan, King Saud University, Kingdom of Saudi Arabia. Supervising of a number of Master’s and Doctoral Theses. Publishing and judging many research papers and authoring many books in the field of agricultural engineering.
Ronnel Blanqueza Fulleros
Ronnel Blanqueza Fulleros and Mansour Nagy Ibrahim work as a technician in the laboratory, where they make various laboratory experiments and measurements and help in writing scientific papers.
References
- Abdullah, N., and N. Chin. 2010. Simplex-centroid mixture formulation for optimized composting of kitchen waste. Bioresour. Technol. 101 (21):8205–10.
- Abu-Zinada, I. 2015. Effect of salinity levels and application stage on cucumber and soil under greenhouse condition. Int. J. Agric. Crop Sci. 8 (1):73–80.
- Allison, F. 1973. Soil organic matter and its role in crop production. Amsterdam: Elsevier Scientific Publ. Company.
- Arnold, P., and M. McCumber. 2015. Determining the most effective method of measuring compost maturity. Canada: Cecily Flemming, Scientist, Biosolids and Soils Standards, Ecological Standards Section, Standards Development Branch, Ontario Ministry of the Environment Project published by The Ontario Ministry of Environment & RRFB Nova Scotia.
- Asgharipour, M., and A. Sirousmehr. 2012. Comparison of three techniques for estimating phytotoxicity in municipal solid waste compost. Annu. Biol. Res. 3 (2):1094–101.
- Avnimelech, Y., M. Bruner, I. Ezrony, R. Sela, and M. Kochba. 1996. Stability indexes for municipal solid waste compost. Compost Sci. Util. 4 (2):13–20.
- Bach, P., K. Nakasaki, M. Shoda, and H. Kubota. 1987. Thermal balance in composting operations. J. Ferment. Technol. 65 (2):199–209.
- Barral, M., and R. Paradelo. 2011. A review on the use of phytotoxicity as a compost quality indicator. Dyn. Soil Dyn. Plant 5 (2):36–44.
- Bazrafshan, E., A. Zarei, K. Mostafapour, N. Poormollae, S. Mahmoodi, and M. Zazouli. 2016. Maturity and stability evaluation of composted municipal solid wastes. Health Scope 5 (1):1–9.
- Bernal, M. P., M. Paredes, M. Sánchez-Monedero, and J. Cegarra. 1998. Maturity and stability parameters of composts prepared with a wide range of organic wastes. Bioresour. Technol. 63:91–99.
- BIS. 1982. Methods for analysis of solid wastes (excluding industrial solid wastes). New Delhi, India: Indian Standards Institution.
- Brinton, W. F. 2000. Compost quality standards & guidelines, final report. Canada: Woods End R Laboratory, Inc., published by The Ontario Ministry of Environment & RRFB Nova Scotia.
- Brinton, W. F., and M. W. Droffher. 1994. Microbial approaches to characterization of composting processes. Compost Sci. Util. 2:12–17.
- Buchanan, M., W. Brinton, F. Shields, J. West, and W. Thompson. 2001. California compost maturity index. Manuscript report to CIWMB https://woodsend.com/wp-content/uploads/2019/09/Compost-Maturity-Index_CA-Compost-Quality-Council_Buchanan_Brinton_2001.pdf
- Bustamante, M., C. Paredes, F. Marhuenda-Egea, A. Espinosa, M. Bernal, and R. Moral. 2008. Co-composting of distillery wastes with animal manures: Carbon and nitrogen transformations in the evaluation of compost stability. Chemosphere 72:551–57.
- Butler, T., L. Sikora, P. Teeinhilber, and L. Douglass. 2001. Compost age and sample storage effects on maturity indicators of biosolids compost. J. Environ. Qual. 30:2141–48.
- Cabañas-Vargas, D., M. Sánchez-Monedero, S. Urpilainen, A. Kamilaki, and E. Stentiford. 2005. Assessing the stability and maturity of compost at large-scale plants. Ingeniería 9-2:25–30.
- Cambardella, C., T. Richard, and A. Russell. 2003. Compost mineralization in soil as a function of composting process conditions. J. Soil Biol. 39:117–127.
- Canadian Council of Ministers of the Environment (CCME). 2005. Guidelines for compost quality PN 1340. Part 1. Ottawa, ON: Environment Canada.
- Chefetz, B., Y. Chen, Y. Hadar, and P. G. Hatcher. 1998. Characterization of dissolved organic matter extracted from composted municipal solid waste. Soil Sci. Soc. American J. 62 (2):326–32.
- Chen, J., Z. Yutian, G. Zhanhu, and N. Albert. 2020. Recent progress on thermo-electrical properties of conductive polymer composites and their application in temperature sensors. Engineered Science 12:13–22.
- De Nobili, M., and F. Petussi. 1988. Humification index as evaluation of the stabilization degree during compost. J. Ferment. Technol. 66:557–83.
- Elcik, H., A. Zoungrana, and N. Bekaraki. 2016. Laboratory-scale investigation of aerobic compost ability of municipal solid waste in Istanbul. Sigma J. Eng. & Nat. Sci. 34 (2):211–20.
- Ells, J., A. McSay, and S. M. Workman. 1991. Toxic effects of manure, alfalfa and ammonia on emergence and growth of cucumber seedlings. Hortic. Sci. 26:380–83.
- Emino, E., and P. Warman. 2004. Biological assay for compost quality. Compost Sci. Util. 12:342–48.
- Escobar, N., and V. Solarte. 2015. Microbial diversity associated with organic fertilizer obtained by composting of agricultural waste. Int. J. Biosci. Biochem. Bioinf. 5 (2):70–79.
- Estevez-Schwarz, I., S. Seoana-Labandeira, A. Nunez-Delgado, and E. Lopez-Mosquera. 2012. Production and characterization of compost made from garden and other waste. Pol. J. Environ. Stud. 21 (4):855–64.
- Francou, C., M. Poitrenaud, and S. Houot. 2005. Stabilization of organic matter during composting: Influence of process and feedstocks. Compost Sci. Util. 13:72–83.
- Gao, M., F. Liang, B. Yub, and L. Yang. 2010. Evaluation of stability and maturity during forced-aeration composting of chicken manure and sawdust at different C/N ratios. Chemosphere 78:614–19.
- Ghaly, A., F. Alkoaik, and A. Snow. 2006. Thermal balance of in-vessel composting of tomato plant residues. Can. Biosyst. Eng. 48: 6.1–6.11.
- Haug, R. 1993. The practical handbook of compost engineering, 377–78. Boca Raton, Florida: Lewis publishers.
- Heerden, I. V., C. Cronje, S. H. Swart, and J. M. Kotze. 2002. Microbial, chemical and physical aspects of citrus waste composting. Bioresour. Technol. 81:71–76.
- Hemidat, S., M. Jaar, A. Nassour, and M. Nelles. 2018. Monitoring of composting process parameters: A case study in Jordan. Waste Biomass Valorization 9:2257–74.
- Irvan, T. H., B. Trisakti, F. Batubara, and H. Daimon. 2018. Composting of empty fruit bunches in the tower composter effect of air intake holes. IOP Conf. Series: Mat. Sci. Eng. 309 (2018):012066. doi:10.1088/1757-899X/309/1/012066.
- Jindo, K., K. Suto, K. Matsumoto, C. Garcia, T. Sonoki, and M. A. Sancchez-Monedero. 2012. Chemical and biochemical characterization of biochar-blended composts prepared from poultry manure. Bioresour. Technol. 110:396–404.
- Kalamdhad, A., and A. Kazmi. 2009. Effect of turning frequency on compost stability and some chemical characteristics in a rotary drum computer. Chemosphere 74:1327–34.
- Kayhanian, M., and G. Tchobanoglous. 1993. Characteristics of humus produced from anaerobio composting of the biodegradble organic fraction of municipal solid waste. Environ. Technol. 14 (9):815–29.
- Khater, E. S. G. 2015. Some physical and chemical properties of compost. Int. J. Waste Resour. 5:172. doi:10.4172/2252-5211.1000172.
- Kulcu, R. 2014. Communication composting of greenhouse tomato plant residues, wheat straw, and separated dairy manure, and the effect of free air space on the process. Pol. J. Environ. Stud. 23 (4):1341–46.
- Leton, T. G., and E. I. Stentiford. 1990. Control of aeration in static pile composting. Waste Manage. Res. 8:299–306.
- Liao, P., L. Jones, A. Lau, S. Walkemeyer, B. Egan, and N. Holbek. 1996. Composting of fish wastes in a full-scale in-vessel system. Bioresour. Technol. 59:163–68.
- Mac-Gregor, S., F. Miller, K. Psarianos, and M. Finstein. 1981. Composting process control based on interaction between microbial heat output and temperature. Appl. Environ. Microbiol. 41:1321–30.
- Madan, S., A. Bhatia, A. Rajpal, and A. Kazmi. 2012. Maturity assessment of rotary drum and windrow composts in terms of germination index and enzymatic activities. Int. J. Appl. Sci. Eng. Res. 1 (3):415–26.
- Manohara, B., and S. Belagali. 2014. Characterization of essential nutrients and heavy metals during municipal solid waste composting. Int. J. Innov. Res. Sci. Eng. Technol. 3 (2):9664–72.
- Mathur, S., G. Owen, H. Dinel, and M. Schnitzer. 1993. Determination of compost bio-maturity. J. Biol. Agric. Hortic. 10:65–85.
- Nakasaki, K., M. Shoda, and H. Kubota. 1985. Effect of temperature on composting of sewage sludge. Appl. Environ. Microbiol. 50 (6):1526–30.
- Paredes, C., A. Roig, M. P. Bernal, M. A. Sa´nchez-Monedero, and J. Cegarra. 2000. Evolution of organic matter and nitrogen during composting of olive mill wastewater with solid organic wastes. Biol. Fertil. Soils 32:222–27.
- Petric, I., A. Helic, and E. Avdic. 2012. Evolution of process parameters and determination of kinetics for co-composting of organic fraction of municipal solid waste with poultry manure. Bioresour. Technol. 117:107–16.
- Research report, 2005. Assessment of options and requirements for stability and maturity testing of composts, statistical report on test methods. by Phil Wallace, Enviros Ltd. Published by: The Waste and Resources Action Programme, The Old Academy, 21 Horse fair, Banbury, Oxon OX16 0AH, Issue 2 – www.wrap.org.uk
- Rui, L., L. Wei, L. Mengting, H. Qinyu, W. Yinzhen, Z. Qiuqiang, and W. Teng. 2019. Structural, morphological, optical and electrical properties of Cu-doped PBs nanofilms. ES Mater. Manuf. 4:38–44. doi:10.30919/esmm5f217.
- Singh, J., and A. Kalamdhad. 2013. Assessment of bioavailability and leachability of heavy metals during rotary drum composting of green waste. Ecol. Eng. 52:56–69.
- Singh, J., D. Sastry, and V. Singh. 2012. Effects of salinity on tomato during seed germination stage. Physiol. Mol. Biol. Plants 18 (1):45–50.
- Singh, Y. K., A. S. Kalamdhad, M. Ali, and A. A. Kazmi. 2009. Maturation of primary stabilized compost from rotary drum composter. Resour. Conserv. Recycl. 53:386–92.
- Song, C. H., M. X. Li, and X. Jia. 2014. Comparison of bacterial community structure and dynamics during the thermophilic composting of different types of solid wastes: Anaerobic digestion residue, pig manure and chicken manure. Microb. Biotechnol. 7:424–33.
- Sullivan, D. M., A. I. Bary, R. O. Miller, and L. J. Brewer. 2018. Interpreting compost analyses.file:///C:/Users/mrashwan.c/Downloads/Sullivanetal.2018.InterpretingcompostanalysesOSUpublicationEM921710262018%20(1).pdf
- Tiquia, S., N. Tam, and I. Hodgkiss. 1996. Effects of composting on phytotoxicity of spent pig-manure sawdust litter. Environ. Pollut. 93:249–56.
- Tiquia, S. M. 2010. Reduction of compost phytotoxicity during the process of decomposition. Chemosphere 79 (5):506–12.
- Togun, A., W. Akanbi, and J. A. Adediran. 2004. Growth, nutrient uptake and yield of tomato in response to different plant residue composts. Food Agric. Environ. 2 (1):310–16.
- Vuorinen, A., and M. Saharinen. 1997. Evolution of microbiological and chemical parameters during manure and straw co-composting in a drum composting system. Agric. Ecosys. & Envir. 66:19–29.
- Wang, H., Z. Chen, Z. Bin, Z. Zhihua, S. Jun, and D. Ai. 2019a. Ultra-black carbon silica core-shell aerogels with controllable electrical conductivities. Adv. Compos. Hybrid Mater. 2:743–52. doi:10.1007/s42114-019-00123-6.
- Wang, J., L. Yuyan, F. Zhimin, W. Wu, W. Bin, and G. Zhanhu. 2019b. Ink-based 3D printing technologies for graphene-based materials: A review. Adv. Compos. Hybrid Mater. 2:1–33. doi:10.1007/s42114-018-0067-9.
- Wang, X., X. Zeng, and D. Cao. 2018. Biomass-derived nitrogen-doped porous carbons (NPC) and NPC/polyaniline composites as high performance super capacitor materials. Eng. Sci. 1:55–63.
- WEL. 2009. Woods end research laboratory, Inc. Dewar Self-heating Test. https://www.yumpu.com/en/document/view/41989225/dewar-self-heating-test-update-woods-end-laboratories
- WERL. 2018. Woods end research laboratory, Inc. Guide to Solvita Testing for Compost Maturity Index. https://woodsend.com/wp-content/uploads/2019/09/Compost-Maturity-Index_CA-Compost-Quality-Council_Buchanan_Brinton_2001.pdf
- Windhorst, H. W. 2008. A projection of the regional development of egg production until 2015. World’s Poult. Sci. J. 64(3):356–376.
- Wong, J. W. C., K. F. Mak, and N. W. Chan. 2001. Co-composting of soybean residues and leaves in Hong Kong. Bioresour. Technol. 76:99–106.
- Wong, M. 1985. Phytotoxicity of refuse compost during the process of maturation. Environ. Pollut. 37:159–74.
- Young, C., P. Rekha, and A. Arun. 2005. Compost production: A manual for asian farmers: Chapter 3: What happened during composting. Taipei: Food & Fertilizer Technology Center for the Asian and Pacific Region.
- Zheng, Z., O. Olayinka, and L. Bin. 2018. 2S-soy protein-based biopolymer as a non-covalent surfactant and its effects on electrical conduction and dielectric relaxation of polymer nanocomposites. Eng. Sci. 4:87–99.
- Zhou, Y., L. Feng, and C. Chia-Yun. 2019. Use of BN-coated copper nanowires in nanocomposites with enhanced thermal conductivity and electrical insulation. Adv. Compos. Hybrid Mater. volume 2:46–50. doi:10.1007/s42114-019-00077-9.
- Zucconi, F., A. Monaco, and M. Forte. 1985. Phytotoxins during the stabilization of organic matter. In Composting of agricultural and other wastes, ed. J. K. R. Gasser, 73–86. New York: Elsevier Applied Science Publication.
- Zucconi, F., A. Pera, M. Forte, and M. de Bertoldi. 1981b. Evaluating toxicity of immature compost. Biocycle 22 (4):54–57.
- Zucconi, F., M. Forte, A. Monaco, and M. de Bertoldi. 1981a. Biological evaluation of compost maturity. Biocycle 22 (2):27–29.