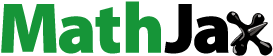
ABSTRACT
Rapid urbanization has resulted in the production of large quantities of sewage sludge over worldwide. The disposal of sewage sludge in landfills or open areas is not considered as an environmentally friendly solution. Therefore, the use of sewage sludge as ash can provide a better solution in geotechnical applications. Keeping this in view, this study investigates the possibility of using sewage sludge ash (SSA) to improve the geotechnical properties of soft clay soil. The sewage sludge ash (SSA) is attained by burning the sludge for 2 h at 900°C. The tests conducted on the clayey soil are specific gravity, standard Proctor test, sieve analysis, Atterberg limits, CBR test and unconfined compression test. Three curing times were used for the untreated soils and soils treated with sewage sludge ash (SSA) which are 3 days, 14 days and 28 days. The sewage sludge ash (SSA) was mixed with the soil at different percentages by weight of the soil at 10%, 15% and 20% of dry soil weight. The results showed that the sludge ash increases the unconfined compressive strength of the soft soil at 3, 14, 28-day curing time. The bearing capacity of the soil decreases after adding SSA at the first, but curing time led to improve the bearing capacity of clayey soil after adding SSA and the percentage of SSA of 10% gave the optimum value of CBR. The results indicated that a better performance of the unconfined shear strength was obtained at 28-day curing time. The findings of this research indicate that sewage sludge ash (SSA) can be used to improve the soft clayey soil and further reduce the environmental risks associated with this substantial.
Implications: Rapid urbanization has resulted in the production of large quantities of sewage sludge over worldwide. The disposal of sewage sludge in landfills or open areas is not considered as an environmentally friendly solution. Therefore, the use of sewage sludge as ash can provide a better solution in geotechnical applications. Keeping this in view, this study investigates the possibility of using sewage sludge ash (SSA) to improve geotechnical properties of soft clay soil. In this paper, Sewage Sludge Ash (SSA) is recycled as a new type of chemical addition to soft clay soil and used as a stabilizer to improve a clayey soil. This study proposes that a percent of the total amount of clay to be reinforced is replaced with sewage sludge ash (sewage sludge ash plus soil) in order to achieve the need of improvement in the geotechnical properties of clayey soil and make use of industrial wastes. The sewage sludge ash (SSA) is attained by burning the sludge for 2 h at 900°C. The tests conducted on the clayey soil are specific gravity, standard Proctor test, sieve analysis, Atterberg limits, CBR test, and unconfined compression test. Three curing times were used for the untreated soils and soils treated with sewage sludge ash (SSA) which are 3 days, 14 days, and 28 days. The sludge ash (SSA) was mixed with the soil at different percentages by weight of the soil at 10%, 15%, and 20% of dry soil weight. The results showed that the sludge ash increases the unconfined compression strength of the soft soil at 3, 14, 28-day curing time. The bearing capacity of the soil decreases after adding SSA at the first, but curing time led to improve the bearing capacity of clayey soil after adding SSA and the percentage of SSA of 10% gave the optimum value of CBR. The results indicated that a better performance of the unconfined shear strength was obtained at 28-day curing time. The findings of this research indicate that sewage sludge ash (SSA) can be used to improve the soft clayey soil and further reduce the environmental risks associated with this substantial.
Introduction
An increase in environmental protection and awareness is another driving force pushing researchers in both academia and industry to examine sludge treatment thoroughly because of sewage sludge ash possesses have the property of pozzolan activity. Tay and Show (Citation1992) found that the strength activity index of sludge ash varied from 58% to 67%, which is close to a class pozzolanic material. Incinerated (SSA) has the pozzolanic reaction nature and so was used in the stabilization of soft subgrade soil. The chemical formulae of the pozzolanic reaction are:
Portland cement, when mixed with water, leads to the beginning of the initial hydration reaction, which in turn leads to a rapid accumulation of calcium in the soil. Then, the clay soil is effectively stabilized as the calcium ions (Ca+2) are released from the mixture. The amount of calcium decreases after 12 h after placing the cement mixture in the soil, and this leads to a decrease in calcium and water. Eventually, Ca(OH)2 and C-S-H are produced. Therefore, “the calcium in the cement meets the initial needs of the soil” (Prisinski and Bhattacharja Citation1999).
Monzo et al. (Citation1999) further proved that sewage sludge ash exhibits pozzolanic activity. They manufactured mortar specimens with part of the cement replaced by sewage sludge ash, they found that the initial strength of the mortar was better, and the compressive and bending strengths improved as the sewage sludge ash (SSA) increased. Thus, part of the cement can be replaced by sludge ash to meet the demands of different engineering applications. Among them, geotechnical engineering applications like soft subgrade soil treatment can easily require large amounts of sludge. Conventionally, cement or fly ash is used to stabilize soft soil. Lin et al. (Citation2005) added sewage sludge ash to reinforce the soft cohesive clayey soil; they found that the untreated A-4 soil was enhanced from mid to low plastic soil, CL (clay), to ML (silt) soil according to the Unified Soil Classification System USCS. They also suggested that incinerated sewage sludge ash (SSA) reduced the swelling behavior of A-4 soil and enhanced the 95% CBR values, unconfined compressive strength, and A-4 soil triaxial shear strength.
In order to improve the properties and strength of the soil, Lin et al. (Citation2007a) recycled (SSA) and hydrated lime to improve soft cohesive clayey soil; five ratios of sludge ash-hydrated lime were mixed with cohesive soil, the results indicated that the swelling behaviors of the treated clayey soil were effectively decreased and the unconfined compressive strength of the soft clayey soil increased to “three to seven times better than that of untreated soil”. The researchers proposed that sewage sludge ash (SSA)/hydrated lime could particularly improve the geotechnical properties of soft clayey subgrade soil. In addition, Lin, Lin, and Luo (Citation2007b) compared the effects of fly ash and sewage sludge ash in terms of ability to improve soft cohesive subgrade soil. They observed that both kinds of ash are adequate for stabilizing the geotechnical properties of such soil samples, although the improvements with fly ash in terms of certain engineering properties were better than with sewage sludge ash, they suggested that (SSA) has the potential to substitute fly ash to improve weakly cohesive subgrade soil.
Chen and Lin (Citation2009) used burned SSA and cement at a 4:1 ratio to stabilize the weakly cohesive subgrade soil. Their results indicated that “the unconfined compressive strength” of treated soil was improved to approximately “three to seven times better than that of the untreated soil”. Moreover, the CBR values of the processed soil were up to “30 times” that of unprocessed soil, they concluded that sewage sludge ash/cement may be helpful for “many potential applications in the field of geotechnical engineering”. Tempest and Pando (Citation2013) presented that the usage of SSA as a soil stabilizing substantial, can increase the stiffness and bearing capacity of the soil in contrast with the untreated soil.
The effect of sewage sludge, stabilized with steelmaking slag, on soil chemical properties and fertility and on wheat (Triticum aestivum L.) growth was evaluated by Samara, Matsi, and Balidakis (Citation2017). Dewatered sewage sludge [75% (wet weight basis)] stabilized with steelmaking slag (25%) and three soils with different pH values were used in a pot experiment with winter wheat. The following treatments were applied: (i) sludge addition of 30 g kg−1, (ii) sludge addition of 10 g kg−1, (iii) addition of the common inorganic N fertilization for wheat (120 kg N ha−1) as NH4NO3, (iv) control (no fertilizer, no sludge). Sludge application at both rates to all soils resulted in a significant increase of pH, electrical conductivity of the saturation extract (ECse) and soil available NO3-N and P, in comparison to the other two treatments and this increase remained constant till the end of the pot experiment.
Norouzian, Abbasi, and AbediKoupai (Citation2018a) added both sewage sludge ash and lime to weak clayey soil in different percentages. The results showed a decrease in the maximum dry density with an increase in the optimum water content. The results also showed that the maximum compressive strength of the treated soil was improved more efficiently, the mixture of 10% (SSA), 5% hydrated lime were found to be as the most efficient mixture.
Norouzian, Abbasi, and AbediKoupai (Citation2018b) added different percentages of sewage sludge ash (0, 5, 10 and 15) % and lime at a ratio of (0, 1, 3, and 5) % to the soil samples, where they prepared 288 samples that were tested under the optimum water content and maximum dry density. The results showed a decrease in the absorption of water for the samples by increasing the curing time, and that the absorption of water increases with the increase of SSA and decreases with increasing of lime. The results also showed that the use of “sewage sludge ash” with lime leads to an increase in the coefficient of softening factor and that the curing time increases the coefficient of softening factor. They also found that the most significant effect on the softening coefficient is the result of adding (0% SSA with 5% lime) and (10% SSA with 5% lime).
Roslia et al. (Citation2020) proposed the recycling of sewage sludge (SS) and red gypsum (RG) as potential temporary landfill cover materials. Mixtures with different SS and RG compositions were prepared and tested in determining the most suitable design mix based on the resulting physical, mechanical, and geotechnical properties, namely the hydraulic conductivity, compressive strength, and plasticity. A maximum compressive strength of 524 kPa was achieved for the optimum SS:RG composition of 1:1, corresponding to Ca:Si composition of 2.5:1, which was appropriate to form the calcium silicate hydrate (CSH) gel. The SS and RG compositions did not affect the hydraulic conductivity, which was in the order 10 5 cm/s for all mixtures. The optimum mixture had compressive strength greater than the specified minimum of 345 kPa, medium hydraulic conductivity, and moderate plasticity.
Two methods related to compaction energy have been applied by Shah et al. (Citation2020) to study the relation of compaction energy with the strength of soil before and after addition of alum sludge as a soil stabilizer. An advanced Artificial Neural Networks (ANNs) technique has been applied with reference to the addition of alum sludge percentage, plasticity index, specific gravity, optimum moisture content, maximum dry density, AASHTO (Citation1986) classification, USCS classification, and group index. It was found that soil strength can be improved even at a low compaction energy level of 600 kN-m/m3 by the addition of optimum percentage of 8% alum sludge as a soil stabilizer.
An eco-friendly cementitious binder was developed by Zhou et al. (Citation2020) through adding 10, 20, and 30 weight % of lime into sludge ash to obtain different lime/sewage sludge ash ratios. The same amounts of ordinary Portland cement were also added to the equivalent batches of sewage sludge ash for comparing the two systems. The results showed that sewage sludge ash accelerated the hydration rate of cement, and the lime pastes with sewage sludge ash showed larger amounts of heat and higher reactivity than the cement pastes with sewage sludge ash. The lime with sewage sludge ash mix has potential to be used for the development of new controlled low-strength materials.
In this paper, Sewage Sludge Ash (SSA) is recycled as a new type of chemical addition to soft clay soil and used as a stabilizer to improve a clayey soil. This study proposes that a percent of the total amount of clay to be reinforced is replaced with sewage sludge ash (sewage sludge ash plus soil) in order to achieve the need of improvement in the geotechnical properties of clayey soil and make use of industrial wastes.
Materials and methods
Soil properties
To study the effect of sewage sludge on the geotechnical characteristics of the clayey soil, a brown clayey soil block sample was taken from a region South of Baghdad city in Iraq. A quantity of sewage sludge was also taken from Al-Rustamiya drainage station. The experimental work included carrying out routine laboratory tests to study the effect of different percentages of sewage sludge on the geotechnical properties of fine-grained soil. Details of the physical properties are given in . The particle size distribution of the soil used is shown in .
Table 1. The physical properties of the soil
Sewage sludge ash (SSA)
The sewage sludge used in this study was brought up from a local waste water treatment plant (Al-Rustamiya drainage station) in Baghdad city. The sewage sludge was burned in an electric furnace at 900°C and ground into fine powder particles. Test results obtained from the Energy–dispersive X-Ray Spectroscopy (EDS) are presented in and . It is evident that the most available ions in the sewage sludge material are calcium and silicon.
Table 2. Results of EDS for sewage sludge ash
Preparation and storage of soil samples and routine tests
The soil samples were divided into four portions, each portion of the soil sample was mixed thoroughly with sewage sludge at different percentages of 10%, 15%, and 20% by weight of the dry weight of the soil. Then, the samples were compacted according to “standard Proctor test (ASTM D698)”. They were compacted at optimum moisture content (OMC) and maximum dry density (MDD). Specimens were subjected to routine laboratory tests including Atterberg limits, particle size distribution, CBR, unconfined compression tests.
The unconfined compression test was conducted on treated and untreated samples according to ASTM D2166-00 (Citation2012).. Curing was made on samples for periods of 3, 14, and 28 days. During these periods, the samples were stored within nylon bags to keep the moisture content unchanged. The unconfined compression machine was appointed with a maximum load of 2 kN while the compression rate was 1 mm/min.
CBR test is used to analyze the strength of soil after compaction. The test was developed by the California Department of transportation. It is described in AASHTO T193. One of the important strength parameters is known as CBR, which shows the highest level of CBR at the addition of 8% alum sludge and after that, at 10% addition, it starts reducing.
Results and discussion
Effect of SSA on the grain size distribution of the clayey soils
“Grain size distribution” was conducted in accordance with “ASTM D421-10 and D422-10”. shows the grain size distributions before and after adding of the SSA with 10%, 15%, and 20% by weight of soil. The figure shows the change in gradation of particles with the increase in percent of SSA added. The gradation curves are close together which means that gradation of the SSA is close to that of the original soil. It can be noticed that the soil becomes slightly coarser with the increase of SSA and the sedimentation time in a hydrometer test, slightly decreases with increasing SSA contents.
Atterberg limits
Atterberg limits or consistency limits are characterized by plastic and liquid limits and plasticity index. These limits control the consistency of the soils as wetting conditions change (Fattah, Al-Saidi, and Jaber Citation2014). The variation in consistency limits with SSA content (%) is shown in . It is noticed from that both liquid limit and plastic limit (after 3 day curing time) increase slightly with increase in SSA content. This may be considered to be a result of addition of SSA, which has higher water absorption affinity. Because the plasticity index PI decreases, the soil behavior becomes more like that of silty soils than clays. Therefore, the soil can be used as fine – grained aggregates (fillers) for asphalt or concrete mixes. From , and after curing time of 28 days, it can be seen that both liquid limit and plastic limit decrease with increase of SSA content and that can be attributed to the pozzolanic activity associated with the SSA reaction during the hydration process.
Effect of the SSA on the specific gravity
shows the specific gravity values of soil with different percentage of SSA. The decrease of the specific gravity values of the soil with the increase of SSA content is a result of increasing lightweight materials of the same volume due to the lower density of SSA. It is known that the specific gravity of SSA is about 2.2.
Effect of the SSA on compaction characteristics
The variation in optimum moisture content (ωopt) and maximum dry density (ρdmax) with SSA content is shown in . The (ρdmax) decreases with increase in SSA content. However, the (ωopt) increases with increase in SSA content. A decrease in the dry density may be due to the lower specific gravity of the SSA, while an increase in the optimum moisture content may be as a result of water needed to be hydrated. SSA0, SSA10, SAS15, and SSA20 refer to samples treated with percent of SSA 0%, 10%, 15%, and 20%, respectively.
Figure 7. Relationship between moisture content (ω) and dry density (ρd) for clayey soil stabilized with different SSA contents
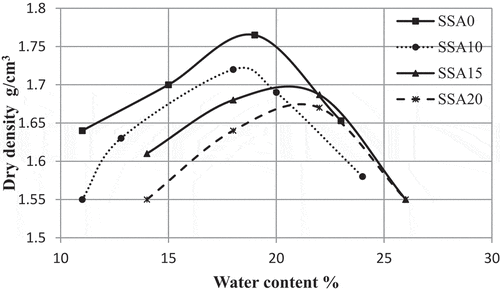
With increasing SSA, the mixture’s specific gravity reduced as the specific gravity for a clean SSA was smaller than that of soil particles. With increasing SSA content beyond the 10% ratio, the mixture became more brittle and lacking plastic behavior, as reflected by the resulting plastic limits which were nonexistent for these mixes. The mixtures with high SSA content upheld the granular formation and no clods were formed even with an increasing amount of water added. This was due to the significant amount of silica (Si) present in the mixtures, the behavior of which is known to be independent of the amount of water added as only a small amount of it would become effective in changing the plastic limit as indicated by Hunt et al. (Citation2019).
Effect of SSA on the pH values
As indicated before, four different percentages of SSA; 0%, 10%, 15%, and 20%, were added to clayey soil. In this study, the ratio of solid to liquid (30 g soil admixture: 75 ml water) was held in 1:2.5 for pH value tests. illustrates the results of pH values for samples modified with sewage sludge that were cured at 14 and 28 days, respectively. It is noted that pH increases with increasing of SSA percentage of unmodified soil with different curing ages whereas the alkaline soil and acidic sludge ash could simply affect pH values of the sludge ash/soil samples. However, the pH values of sludge ash/soil sample decreased slightly for longer curing ages. This slight decrease is caused by the alkaline products of CH and C-S-H reactions. The pH values for mixtures ranged between 8.2 and 8.7 after 14 day curing and between 8.0 and 8.3 after 28 day curing.
Eades and Grim (Citation1966) recommended that the optimum modification mixture (OMM) can be evaluated by using the pH values for soil mixed with admixture; they proposed a range of (10–20) % for the optimum modification mixture (OMM).
In this study, it can be noted that the effect of the sludge ash on the pH values is very small, that is the pH value is between 8 and 8.5 for curing age 28 days. Though, the pH values decreased after curing time of 14 days, this is due to the increasing in the amounts of reaction products as their curing ages were extended.
Effect of the SSA on shear strength
Unconfined compression test
As a common rule for improvement, the better quality of the improved materials is the higher the compressive strength. The stress–strain curves for the untreated samples and treated with SSA by 10%, 15%, and 20% are shown in with curing time 3, 14, and 28 days, respectively. The variation in the unconfined compressive strength with SSA content is shown in with curing period (3, 14, and 28) days. At first, and when the curing time is 3 days, the unconfined compressive strength increases from 113.5 kPa for the soil without SSA to 186.8 kPa for the soil with 10% SSA, which is taken as optimum. The strength increasing may be attributed to the pozzolanic reactions of SSA to form the cementious products between the Pozzolana present in SSA and Calcium hydroxide present in the soil. Also, there is possibility that the SSA containd CaO, so that Ca and Si compounds present in the SSA react with soil compounds using moisture contained in soil because the pH value of the soil was lower than those of the mixtures as shown in .
Figure 11. Relationship between strain and axial stress for the different SSA contents with curing time of 3 days
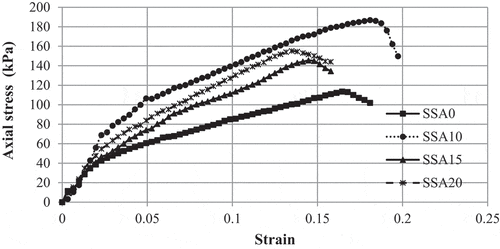
Figure 12. Relationship between strain and axial stress for the different SSA contents with curing time of 14 days
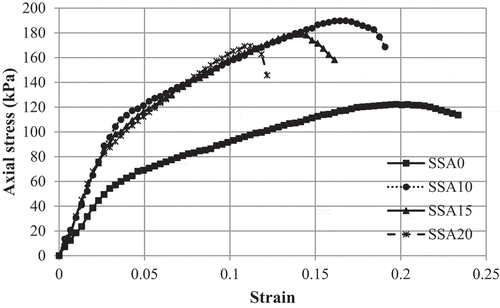
Figure 13. Relationship between strain and axial stress for the different SSA contents with curing time of 28 days
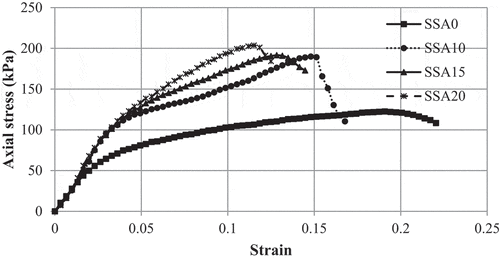
From the study area: consistency of plain clayey soil and clayey soil with 10% and 20% SSA content refers to stiff soil. The strength developed for various amounts of sludge ash added to soil and cured for 3 days, 14 days, and 28 days is shown in the figures below. The increased strength for specimens with 10% of SSA content is about 1.6 times the original strength when the curing time is 3 days. When cured for 28 days, the strength increased by 1.65 times the original strength for sludge ash/soil samples, and the strengths obtained is about 202 kPa as seen in . These strengths kept growing for curing age of 28 days especially for SSA content of 15% and 20%.
Figure 14. Relationship between SSA and unconfined compressive strength of samples at different curing ages
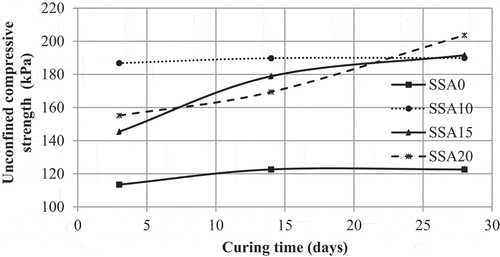
Figure 15. Relationship between the unconfined compressive strength for samples and the sewage sludge ash added to clayey soil at curing ages 3, 14 and 28 days
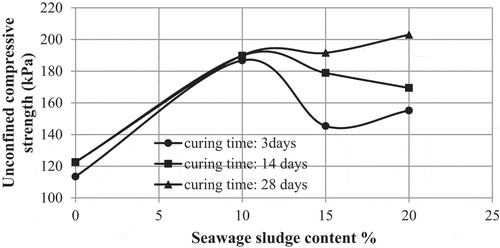
It is interesting to notice from figures that the strength of sludge ash/soil samples was much higher than the strength of untreated soil. However, increasing the amount of sludge ash limited the increase in strength for both curing times (14 and 28 days). At 20% sewage sludge ash content, the ash absorbed more water and mixed well with the soil to form clods. Therefore, a slight increase in dry density in 14 days curing time and a reduction in water content of the mixture were produced.
There is a decrease in the absorption of water for the samples by increasing the curing time, and that the absorption of water increases with the increase of SSA. This reflects the increase in the unconfined compressive strength with increasing of curing time. Although the unconfined compressive strength UCS value with SSA decreases by 15% after 3 days curing and again increases with 20% of SSA, but this does not reflect the general trend of behavior where the strength increases by increasing the curing time.
present the sample shape at the end of unconfined compression test, the samples are subjected to curing time of 14 and 28 days, respectively.
Figure 16. Clay specimens with a) 0%, (b) 10%, (c) 15%, (d) 20% of SSA and curing time 14 days after failure by unconfined compression test
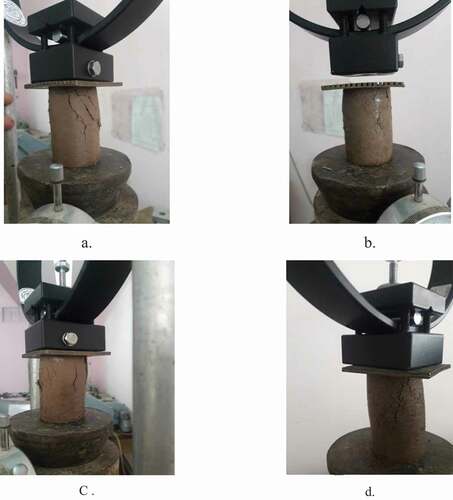
Figure 17. Clay specimens with a) 0%, (b) 10%, (c) 15%, (d) 20% of SSA and curing time 28 days after failure by unconfined compression test
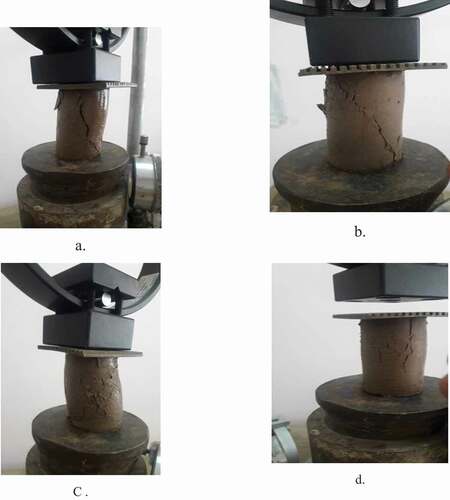
In , untreated samples failed with apparent inclined cracks that indicate the failure planes. It is evident that development of cracks in samples before failure decreases with increase of the percent of SSA and also curing time.
Effect of SSA on California bearing ratio (CBR)
To estimate the sufficiency of SSA in the improvement of weak subgrade clayey soil, sludge ash was added to the soil with (10, 15, 20) % of dry weight of the soil and tested for soil stabilization. Curing was made on samples for periods of 3, 14, and 28 days. During these periods, the samples were stored within nylon bags to keep the moisture content unchanged. The pavement design specification, AIIS-181, refers to that subgrade soil is characterized into three groups by CBR values:
CBR ≤ 3, poor subgrade soils.
3 < CBR ≤ 8, medium subgrade soils, and
CBR ≥ 8, good-to-excellent subgrade soils.
shows that CBR values for the clayey soil without any SSA content equal to 7 and the soil is classified as medium subgrade soils, when the SSA content is added to the soils, it can be seen from that CBR values decrease as the amount of SSA increased when the curing time equals 0 days, with increasing the curing time, and when the curing time is 28 days, the CBR value increased to 16% at SSA equal to 10%, 14% at SSA equal to 15% and 15% at SSA 20%. The soil is improved to be good to excellent subgrade soil. This implies that SSA replacement can effectively improve the soft subgrade untreated soil, and the CBR values are improved by 1.8 to 2 times. Two explanations exist for such good improvements in the CBR values: the soil strength was improved by the pozzolanic and hydration reaction provided by SSA, and the very low permeability of SSA/cement helped stop water from permeating the soil and softening the soil layer.
Hydration products like calcium hydroxide and C-S-H gel can increase the soil strength. However, greater amounts of SSA additive imply that more water is needed, which leads to insufficient water for the above reactions in treated soil and inefficient improvement in terms of the CBR values. As a result, the optimum amount of SSA added to treated soil is 10% in this study. The excess SSA requires more water in pores which results in a decrease in strength and CBR. Shah et al. (Citation2020) concluded that maximum dry density is directly proportional to CBR and inversely proportional to optimum moisture content showed an increased value of CBR.
The physical properties of the clayey soil after treatment with SSA are listed in . The effect of curing time on the properties of soil treated with SSA is illustrated in . The presence of SSA within the soil increased its capability to absorb water due to high specific surface of SSA so that the optimum moisture content increases.
Table 3. The physical properties of the soil treated with SSA
Table 4. The properties of the soil treated with SSA and curing time
The curing time effect can be attributed to the alkaline reaction and cementing properties of SSA. Samara, Matsi, and Balidakis (Citation2017) found that after 2 years, the stabilized sludge had a strongly alkaline reaction and high content of equivalent CaCO3, due to the alkaline nature of the added slag. Because of the high pH value, the stabilized sludge’s microbial load was not detectable. In addition, its moisture content was low, probably due to the cementing properties of the slag. The behavior of slag is close to that of SSA, so similar action is expected.
Tempest and Pando (Citation2013) also concluded that after 28 days of curing, the bearing capacity obtained from a series of plate load tests carried out at each of the test subsections showed that the relative bearing capacity improvements reached 13.9% and 31.7% when the clayey soil at this test site was treated with 5% and 10% DA (dry ash) SSA by weight, respectively.
SEM analysis
In order to understand the relationship between the micro-structure and macro behavior of specimens, scanning electron microscopy (SEM) analyses were performed on soil treated with different amounts of sewage sludge content. ) shows SEM images of clayey soils treated with SSA content of 0%, 10%, 15%, 20% respectively for curing time 14 days. As shown in , the hydration process can make the paste denser as the sewage sludge increased. This indicates that the hydration process is not fully developed after 14 days. The hydration products were loose within the structure and the paste exhibits a high porous ratio. However, when sewage sludge content equals to 20%, the hydration process is almost fully developed, and the hydration products gradually mature. These products may fill certain pores to reduce the paste porous ratio. As shown in ), the irregular laminated C-S-H gel and with laminar lumps of CH form an integrated paste structure and most pores were filled, although there remain some fine cracks or pores. This indicates that the addition of SSA to the clayey soil paste was helpful in the hydration process. This became more noticeable after curing for 28 days. Thus, the addition of SSA may improve both soil strength and CBR values. This advantage of SSA additive became more evident as the curing time increased.
Conclusion
Based on the results presented in this study from experiments conducted on soil samples treated with different percents of SSA, the following conclusions can be drawn:
The gradation of soil particles was affected by SSA, so the soil becomes coarser than the untreated soil.
The specific gravity of solid particles (Gs) decreased about 3% when the SSA is increased to 20% by weight of the dry soil sample due to light weight of SSA.
The addition of SSA to clayey soil caused a decrease in the plasticity index as SSA increased to 20% by weight of the dry soil and this result was connected with the SSA reaction in the hydration process, including the bonding and hardening processes. This produced hydration products like calcium aluminate and calcium silicate.
The optimum water content decreased significantly by about 33% with increase of SSA content to 20% in the clayey soil, while the maximum dry density is reduced slightly by about 5% with the same increase of SSA.
The unconfined compressive strength increases with addition of 10% SSA which is considered as optimum percent at the first curing time (3 days). Curing time leads to increase the unconfined compressive strength for all SSA contents. The pozzolanic reaction is a time-dependent process and with increasing curing time, the greater amounts of sewage sludge ash participate in the pozzolanic reactions.
The bearing capacity of the soil decreases after adding SSA, CBR values for soils improved with curing time and the CBR values are improved by 2 times that of untreated soil at SSA content 10% and curing time 28 days.
Test results obtained from CBR values, and unconfined compression test demonstrated that SSA was able to efficiently improve the engineering properties of soft cohesive subgrade soil. The optimum amount of sludge ash was 10% by weight for improving the basic properties and strength of soft cohesive subgrade soil in this study.
Disclosure statement
No potential conflict of interest was reported by the authors.
Additional information
Notes on contributors
Yasser M. Kadhim
Mohammed Y. Fattah is a Professor of Geotechnical Engineering. Civil Engineering Department, University of Technology – Iraq.
Rana A.J. Al-Adhamii
Yasser M. Kadhim is Ph.D. in geotechnical engineering, University of Baghdad, 2010 Lecturer – Civil Engineering Department – Dijla University – Baghdad, Iraq.
Mohammed Y. Fattah
Rana A.J. Al-Adhamii is M.Sc. in geotechnical engineering, University of Baghdad, 2003. Assistant Lecturer – Civil Engineering Department – Dijla University – Baghdad, Iraq.
References
- AASHTO. 1986. Standard specification for transportation materials and methods of sampling and testing. Part II. 14th ed. Washington, DC: AASHTO (American Association of State Highway and Transportation Officials).
- ASTM D 2166-00. 2012. Standard test method for unconfined compressive strength of cohesive soil. Philadelphia: Annual book of ASTM standards, ASTM international.
- ASTM D 2487-11. 2012. Standard practice for classification of soils for engineering purposes (unified soil classification system). Philadelphia: Annual book of ASTM standards, ASTM international.
- ASTM D 422-10. 2007. Standard test method for particle size analysis of soils. Philadelphia: Annual book of ASTM standards, ASTM international.
- ASTM D 4318-10. 2012. Standard test methods for liquid limit, plastic limit, and plasticity index of soils. Philadelphia: Annual book of ASTM standards, ASTM international.
- ASTM D 698-00. 2012. Standard test methods for laboratory compaction characteristics of soil using standard effort (12,400 ft-lbf/ft3 (600 kN-m/m3)). Philadelphia: Annual book of ASTM standards, ASTM international.
- ASTM D 854-10. 2012. Standard test method for specific gravity of soils. Philadelphia: Annual book of ASTM standards, ASTM international.
- Chen, L., and D. F. Lin. 2009. Stabilization treatment of soft subgrade soil by sewage sludge ash and cement. J. Hazard. Mater. 162 (1):321–27. doi:https://doi.org/10.1016/j.jhazmat.2008.05.060.
- Eades, J. L., and R. E. Grim. 1966. A quick test to determine lime requirement for lime stabilization. Highway Research, Record3, 61–70.
- Fattah, M. Y., A. A. Al-Saidi, and M. M. Jaber. 2014. Consolidation properties of compacted soft soil stabilized with lime-silica fume mix. Int. J. Sci. Eng. Res. 5 (7), July:1675–82.
- Hunt, S. A., M. L. Whitaker, E. Bailey, E. Mariani, C. V. Stan, and D. P. Dobson. 2019. An experimental investigation of the relative strength of the silica polymorphs quartz, coesite, and stishovite. Geochem. Geophys. Geosyst. 20 (4):1975–89. doi:https://doi.org/10.1029/2018GC007842.
- Lin, D. F., K. L. Lin, M. J. Hung, and H. L. Luo. 2007a. Sludge ash/hydrated lime on the geotechnical properties of soft soil. J. Hazard. Mater. 145 (1–2):58–64. doi:https://doi.org/10.1016/j.jhazmat.2006.10.087.
- Lin, D. F., K. L. Lin, and H. L. Luo. 2007b. A comparison between sludge ash and fly ash on the improvement in soft soil. J. Air Waste Manage. Assoc. 57 (1):59–64. doi:https://doi.org/10.1080/10473289.2007.10465294.
- Lin, D. F., H. L. Luo, D. H. Hsiao, and C. C. Yang. 2005. The effects of sludge ash on the strength of soft subgrade soil. J. Chinese Inst. Environ. Eng. 55 (1):1–10.
- Monzo, J., J. Paya, M. V. Borrachero, and E. Peris-Mora. 1999. Mechanical behavior of mortars containing sewage sludge ash (SSA) and portland cements with different tricalcium aluminate content. Cem. Concr. Res. 29 (4):87–94. doi:https://doi.org/10.1016/S0008-8846(98)00177-X.
- Norouzian, K., N. Abbasi, and J. AbediKoupai. 2018a. Use of sewage sludge ash and hydrated lime to improve the engineering properties of clayey soils. Geotech. Geol. Eng. 36:1575–86. doi:https://doi.org/10.1007/s10706-017-0411-9.
- Norouzian, K., N. Abbasi, and J. AbediKoupai. 2018b. Evaluation of softening of clayey soil stabilized with sewage sludge ash and lime. Civil Eng. J. 4 (4):743–54. doi:https://doi.org/10.28991/cej-0309129.
- Prisinski, J. R., and S. Bhattacharja. 1999. Effectiveness of portland cement and lime in stabilizing clay soils. Transp. Res. Rec. 1652:215–27. doi:https://doi.org/10.3141/1652-28.
- Roslia, N. A., H. A. Azizb, M. R. Selamatb, and L. L. P. Lima. 2020. A mixture of sewage sludge and red gypsum as an alternative material for temporary landfill cover. J. Environ. Manage. 263:110420. doi:https://doi.org/10.1016/j.jenvman.2020.110420.
- Samara, E., T. Matsi, and A. Balidakis. 2017. Soil application of sewage sludge stabilized with steelmaking slag and its effect on soil properties and wheat growth. Waste Manage. 68:378–87. doi:https://doi.org/10.1016/j.wasman.2017.06.016.
- Shah, S. A. R., Z. Mahmood, A. Nisar, M. Aamir, A. Farid, and M. Waseem. 2020. Compaction performance analysis of alum sludge waste modified soil. Constr. Build. Mater. 230:116953. doi:https://doi.org/10.1016/j.conbuildmat.2019.116953.
- Tay, J. H., and K. Y. Show. 1992. The use of lime-blended sludge for production of cementitious material Water Environ. Res. 64 (1):6–12. doi:https://doi.org/10.2175/WER.64.1.2.
- Tempest, Q. B., and M. A. Pando. 2013. Characterization and demonstration of reuse applications of sewage sludge ash. Int. J. Geomate 552–59. doi:https://doi.org/10.21660/2013.8.1620.
- Zhou, Y. F., J. S. Li, J. X. Lu, C. Cheeseman, and C. S. Poon. 2020. Recycling incinerated sewage sludge ash (ISSA) as a cementitious binder by lime activation. J. Leaner Prod. 244:118856. doi:https://doi.org/10.1016/j.jclepro.2019.118856.