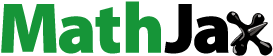
ABSTRACT
Total emissions from all sources need to be accurately quantified in an emission inventory in order for a basin to develop their air pollution management plan. The best approach is to measure both the real-world emissions and activity for a source; however, often only emissions are measured and activity is estimated from historical factors. This report focuses on harbor craft and presents data showing that using measured emissions and historical factors for activity will lead to inaccurate emission contributions. In this research, real-world activity data were collected from the engine control module (ECM) and from a surrogate method that relied on exhaust temperature. Measured real-world activity values from 10 harbor craft were compared with historical values found in the certification cycle. The vessels included four tugboats, three pilot boats, two police boats, and one supply vessel. The results showed the activity values used in the certification cycle did not reflect the real-world activity of the 10 harbor craft vessels. On average, real-world NOx emissions were only 46% of the NOx emissions estimated by ISO 8178-E3 weighting factor. In contrast, inclusion of significantly lower load factors leads to 31% higher in-use NOx emission factors on average. CARB/EPA load factor ranges from 0.45 to 0.51 while in-use load factor ranged from 0.14 to 0.44. From this finding, researchers are cautioned about accepting the activity values in a certification test cycle, like ISO 8178 E3, and instead should measure real world activity data. This change would improve the accuracy of the emission contribution from harbor craft to the local inventory.
Implications: Real-world measurements of activity and emissions are the best way to get an accurate emission contribution to emission inventory. This paper reports on the differences between the use of the traditional certification cycle and real-world activity of harbor craft. Engine control module (ECM) and exhaust temperature data from 10 harbor crafts with different types of operation were used to compare real-world activity data to certification cycle.
Introduction
Emissions from land and marine sources contribute to local air pollution (Vutukuru and Dabdub Citation2008; Wang, Corbett, and Firestone Citation2007; Yau et al. Citation2013) and are known to cause adverse health effects (Cohen et al. Citation2005; Tang et al. Citation2020). Exposure to particulate matter (PM2.5) emissions is linked to respiratory related diseases (Pope 3rd Citation2000; Pope III and Dockery Citation2006) and marine shipping PM emissions are estimated to contribute to approximately 60,000 cases of cardiopulmonary and lung cancer deaths annually (Corbett et al. Citation2007). In the past decades, emissions from on-road sources were the major source and through regulation have been greatly reduced from the previous 1990 level (Rao, Tooly, and Drukenbrod Citation2013; Wu et al. Citation2011). While the on-road sources are still significant, the off-road sources are becoming a larger percentage of the total emission inventory. For example, off-road nitrogen oxide (NOx) emissions make up 14.5% of total mobile NOx emissions in California (CARB Citation2010) and so attention is switching to off-road emissions sources. Browning and Bailey (Citation2006) report that off-road data is less available as these sources are more difficult to test. While harbor craft emissions are less than those of ocean-going vessels (OVGs), they still have significant impact on the port communities (Aldrete et al. Citation2007). In 2019, 755 tons or 12% of port-related NOx emissions, 26 tons or 23% of port-related diesel particulate matter (DPM) emissions came from harbor craft for the port of Los Angeles, POLA (Starcrest Citation2020b). For the same period 637 tons or 10% of port-related NOx emissions, 22 tons or 19% of port-related diesel particulate matter (DPM) emissions came from harbor crafts for the port of Long Beach, POLB (Starcrest Citation2020a). Rosenbaum, Hartley, and Holder (Citation2011) examined exposure to diesel particulate matter (DPM) across 43 selected major U.S. harbors and estimated the size and demographic of the affected populations. They found that more than four million people have a risk level of greater than 100 per million and 41 million people are at a risk level greater than 10 per million of carcinogenic health risk. Their model used PM2.5 values to represent DPM. Across all port communities, the most exposed are people of color who live in disadvantaged and low-income communities.
When developing an air quality management plan for an area, it is of paramount importance that the mass emissions from all sources are accurately reported. Emission inventory methods for calculating mass emissions of criteria and toxic compounds use Equationeq 1(1)
(1) to estimate emissions as documented in EPA and CARB guidance (CARB Citation2012; U.S. EPA, Citation2020).
Where EF is the emission factor in (g/kW-hr), P is the maximum rated power in kilowatts, LF is the load factor as a fraction of maximum rated power, Activity is expressed in hours per day the vessel is at a given load factor, and the Control variable represents the reduction of emission from control technology (Gysel et al. Citation2016). The above expression shows that an accurate estimate for mass emissions depends equally on the accuracy both of the measured emissions and the activity.
Commercial harbor craft, the focus of this research, include fishing vessels, ferries, excursion vessels, tugboats, tow boats, crew and supply boats, barges, dredges, and other vessel types. This broad range of vessels operate in all areas of a port and their individual contribution to the emission inventory are highly dependent on the diesel engine model, load and the engine activity. However, there is a lack of studies on measuring the activity of the harbor craft and often the activity at each load is based on the weighting factors in the ISO certification method. For example, for the ISO 8178-E3 or propeller cycle, one would say the vessel spends 50% of its time operating at 75% power but that is unlikely when operating in a harbor that enforces a no-wake zone and maximum of six knots/hr. In fact, many harbor craft spend a significant amount of time within these zones operating at well under 75% power. Recording and reporting the engine activity of these vessels is more important than assuming that the activity will correspond to the weighting factors used in the engine certification cycle.
Harbor craft are primarily powered by marine compression ignition engines which the U.S. Environmental Protection Agency (U.S. EPA) regulates. Most propeller driven harbor craft have engines that were tested on the E3 duty cycle in accordance of ISO 8178 (ISO Citation1996). This certification cycle, shown in , specifies the modal load test points and the weighting factors at those modes when calculating the overall emission factor. These weighting factors represent all marine vessels tested according to the E3 cycle; however, these weighting factors are primarily based on ocean-going vessels and unlikely to accurately characterize harbor craft operations. In the end, the certification cycle with its weighting factors is useful for showing that an engine meets emission standards, but is unlikely to reflect operation in a harbor where the engine may operate below the 25% power point used to certify the engine. Over and under reporting emissions will result in faulty emission inventories. For example, Corbett and Robinson (Citation2001) measured NOx emissions from a tow boat under real-world operation over inland rivers and reported estimation of NOx emissions by ISO E3 cycle overestimates NOx emissions by 14% compared to the actual emissions measured. Activity of harbor craft is diverse. However, there is no study published on the activity of harbor craft.
This paper aims to report activity of various harbor craft to contribute to better estimation of emission factors for the emission inventory. The activity will be directly compared to the ISO certification cycle. Seven of the 10 vessels were equipped with an ECM (electronic computer module) that relays important information such as engine speed, load, and torque. The other vessels were equipped with data loggers which recorded engine exhaust temperature to be used as a proxy for engine load.
Experimental
Measurement method
Engines on modern harbor craft are equipped with an engine control module which records and outputs activity data. While such output data is easy to record for on-road engines thanks to the presence of OBD-II regulation (U.S. EPA, Citation2009), which required manufacturers to monitor emission control components with standardized data link connectors and communication protocols, off-road engines including those in harbor craft do not require a common output format, protocol or recording device in the absence of any regulatory requirement. As such, manufacturer specific proprietary measurement equipment and software, which are not easy to access and often costly to purchase or license, were used to record and download activity data from harbor craft.
Engines on older harbor craft are not equipped with electronic engine control module and it is more difficult to measure activity from such harbor craft. The exhaust temperature was measured as a surrogate for the engine load under normal operating condition to infer activity. Two vessels that were equipped with ECM loggers were also equipped with exhaust temperature loggers. These two vessels were used to relate the exhaust temperature to engine load activity.
Test units
Data sets for 10 harbor craft consisting of ECM data and exhaust temperature data were collected over the course of a year. These data sets were selected from four separate harbor craft types from separate harbors across California to provide a broader range of activity. Regardless that the activity measurements were made years ago, the operations within a no-wake zone have not changed and are useful, even if the vessel has a new engine. Further, the majority of harbor craft we tested in 2006 are still in operation as of 2020. provides specification of each vessel and Table S1 provides vessels which have had their name changed after the testing. Half of the tugboats were equipped with an ECM logger and the other half were equipped with an exhaust temperature logger. Two pilot vessels were equipped with both an ECM logger and an exhaust temperature logger. The Campbell Foss and Morgan Foss were both tugboats and shared similar engine activity. Tugboats help maneuver larger ships through crowded harbors or canals and assist unmotorized barges. The Campbell Foss and Morgan Foss were the same size, 24 m in length and 10.3 m in width, and were each equipped with Caterpillar engines. These vessels operated a majority of the time in a harbor where there were speed restrictions. The California operated as a pilot boat, whose job was to deliver a maritime pilot to large inbound and outbound ocean-going vessels. The pilot would assume control of the large seafaring vessel and safely maneuver it into or out of the port. At 20,576 hours when the data was collected, the California had the most hours recorded among the vessels. When in the harbor the vessels must keep their speed low in the no-wake zones. Once out of the no-wake zone the pilot boat can increase to full speed to intercept an incoming vessel. The California was larger than other pilot boats at 32 m long by 9 m wide. The Phineas Banning and Stephen M. White (SMW) also operated as pilot boats but display a significantly different engine activity than the California. These two boats were the same size at 13 m long and 4 m wide, less than half the size of the California. The Strategic Horizon operated as a supply/work vessel which means that it was used for a variety of work that included being used as offshore supply vessel, offshore tugboat, or a pilot boat. This vessel mainly operated as an offshore supply vessel and would assist offshore projects with construction, maintenance, and transferring personnel to and from the site. Police Boat 17 and vessel 266–21 operated as law enforcement in and around the harbor. The activity of these vessels varied from day to day with no set schedule. The vessels would patrol different sections of the harbor until they were needed to respond to a situation.
Table 1. Overview of vessels
Results and discussions
ECM load activity
This section describes the results of the engine activity of the vessels gathered by an ECM logger. Through the ECM logger, reports of the engine use could be downloaded and analyzed. Example of the ECM downloaded data is shown in Table S2 for Strategic Horizon. shows that a large amount of time was spent in low-load conditions for each vessel. ) reveals that the Strategic Horizon, the supply vessel, had some variability between its four engines but each engine spent at least 50% or more of its time below 10% engine load. Conversely, each engine spent about 20% of time at high load ranging from 70 to 90%. Interestingly the engines spent less than 3% of time at the full load (90–100%). It is speculated that either the operators avoided running the boat at full load for longevity of the engines or there was some limiting mechanism such as fuel cutoff installed in the engine. The tugboats, the Campbell Foss and Morgan Foss, present a similar and yet a little different engine activity in ). The tugboats spent 50% or more of their time below 19% load. These tugboats showed the higher the load, less time spent. This is very much unlike the Phineas Banning and the Stephen M. White (SMW), both pilot boats, which spent a large portion of time under high load conditions. ) demonstrate that these vessels have a more even distribution of engine activity than the other vessels while they still spent 20% or more of the operating time below 10% load. Their activity displayed trimodal distributions. The pilot boats spent most of their time at 0–9% load, second-most time at 70–79% load and third-most time at 20–29% load. However, another pilot vessel, the California pilot showed quite different activity distribution in ). The California pilot spent more than 75% of time at low load of 10–19%. We do not understand zero percent of time spent at 0–9%. This could potentially be an error in ECM data logging for this vessel. We suspect the percentage of time spent at 0–9% is included at 10–19% load bin somehow. ) shows positively skewed unimodal activity distribution of the police boat, which was not observed in other boats reported in this study. The examples of the boat activity reported in this section suggests boat activities are different depending on their jobs. Even the same vocation boat could have different activity distribution.
Figure 2. ECM engine load activity of the harbor craft (a) Strategic Horizon’s port inboard (PIB) engine, port outboard (POB) engine, starboard inboard engine (SIB), and the starboard outboard engine (SOB).(b) the Campbell Foss tugboat, (c) the Morgan Foss tugboat, (d) the Phineas Banning pilot vessel, (e) the port and starboard engines of the Stephen M. White (SMW) pilot vessel, (f) the port and starboard engines of the California pilot vessel, and (g) the Police Boat 266–21.
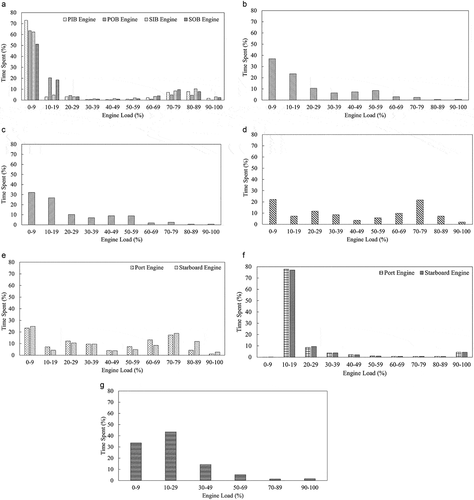
Exhaust temperature as a proxy to ECM Load/RPM activity
The vessels without an ECM logger make assessing engine activity quite difficult. Exhaust temperature can be measured by adding thermocouples to the exhaust stacks. The exhaust temperature could serve as a proxy measurement to engine load if relationships among the temperature, RPM, and engine load could be established. Two of the vessels, the SMW and the Phineas Banning, were fitted with both ECM and temperature loggers. The onboard ECM loggers on these vessels also logged the percentage of time spent per RPM. ) displays similar frequency distribution between RPM frequency and exhaust temperature frequency distribution. This suggests there may be a relationship between the exhaust temperature and the RPM for the Phineas Banning. The relationship between temperature and load was not as prevalent, as shown in ). The SMW did not show as strong a relationship between the exhaust temperature and the engine RPM as shown in ). The engine load also did not match the exhaust temperature curve as shown in ). The exhaust temperature of the SMW did not take on the characteristic U-shape of activity that was demonstrated by many of the other vessels’ activity plots. From ), we could conclude that the relationship between exhaust temperature and engine load did not show a clear correlation. The lack of success of establishing this relationship may be in part due to quality of ECM and temperature data. The ECM provided a summary of cumulative engine run time since the engine was installed, while the exhaust temperature data only captured a few short test periods. The temperature data downloaded during the test period may not have been consistent with the vessel’s normal activity. Further investigation is needed with concurrent measurements of both parameters for the same test period.
Figure 3. (a) Phineas Banning exhaust temperature and RPM per percent of total trip time. (b) Phineas Banning exhaust temperature and engine load per percent of total trip time. (c) SMW exhaust temperature and RPM per percent of total trip time. (d) Stephen M. White (SMW) exhaust temperature and engine load per total trip percent, and (e) comparison of exhaust temperature distribution for vessels that were equipped with thermocouples. Note RPM bin sizes were preselected by ECM.
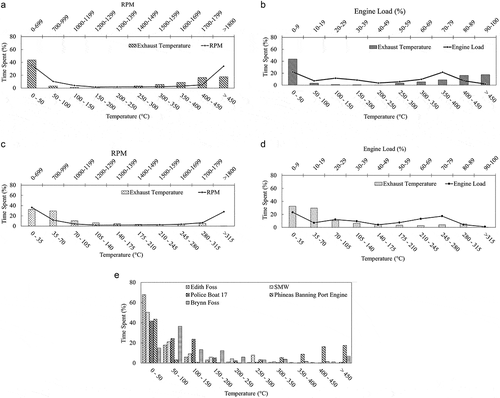
Exhaust temperature data
The exhaust temperature data can still be useful in interpreting the activity of the vessels at low load even though we were not able to create a strong relationship between them for the engine loads at medium and high load. ) displays the temperature distribution of five vessels that were equipped with temperature sensors. All five of these vessels demonstrated that most of the time was spent below 100°C. This would indicate that the vessels spent a great amount of time idling or in low engine load conditions. This conclusion would be consistent with what was observed in of the ECM engine load data.
Comparison of NOx emissions
In this analysis, the NOx emissions for four vessels (Strategic Horizon, Campbell Foss, California, and Phineas Banning) were calculated using the E3 certification weighting factors for engine activity and the real-world weighting factors that were derived from the percentage of engine use from their respective ECM. None of the harbor craft tested were equipped with emission control devices. To estimate total NOx emission using E3 weighting factors, product of time spent fraction (E3 weighting factor) and emissions at the mid-point of each E3 load bin was summed up. Real-world emissions had narrower load bins in general compared to that of E3. Product of time spent fraction from activity data and emissions at the mid-point of each load bin was summed up to determine real-world emissions. Real-world load factors were determined by summing the product of mid-point of actual load bin in fraction and time spent fraction.
As emissions were not determined at different engine loads for the four harbor craft, emission data for the main engine (CAT3512C) determined by Jayaram et al. (Citation2010) was used for all four harbor craft. Jayaram et al.'s (Citation2010) engine data showed typical engine load vs emission trend and linear fit of Jayaram et al.'s (Citation2010) emission data against engine load gave a correlation coefficient value of 0.98.
shows in-use emissions determined by real-world harbor craft activity are much lower compared to the emissions determined by E3 weighting factors. On average the real-world emissions were only 46% of the emissions estimated by E3 weighting factor. also shows CARB and EPA recommended load factor for each type of vessel and real-world load factor from real-world activity data. The inclusion of significantly lower load factors leads to 31% higher in-use emission factors on average. CARB/EPA load factor ranges from 0.45 to 0.51 while in-use load factor ranged from 0.14 to 0.44. The Port of Los Angeles inventory revised downward the average load factor for assist tugs compared with ARB or EPA’s estimate agreeing with the finding of this study (Starcrest Citation2020b). Corbett and Robinson observed a similar result with less discrepancy and smaller variations in the difference of emissions in their study of a river operated tow boat. This study reports there was a significant discrepancy between emissions estimated based on E3 cycle and actual emissions. Harbor craft have quite different engine activity pattern compared to ocean-going vessels which the E3 cycle was based upon. As such for emission inventory, the weighting factors in the certification cycle should be scrutinized before used for harbor craft, instead activity data or real-world emission testing is highly encouraged.
Table 2. Real-world NOx emissions and emission factors compared to E3 estimated NOx emissions and emission factors
Conclusion
The goal of this paper was to report on the equal importance of activity measurements as well as emission measurement. Furthermore, the report shows that accepting the weighting factors from the certification cycle will lead to errors in the calculation of the inventory contribution. Of the 10 harbor craft vessels, seven were outfitted with ECM loggers that provided an in-depth look at the vessels’ engine activity of their lifetime. This data displayed the actual real-world operation of the vessels and showed that the engine activity was weighted more heavily on the low-load activity than the certification cycle would suggest. The remaining three vessels were only outfitted with exhaust temperature sensors and two of the vessels with ECM loggers were also equipped with exhaust temperature sensors. The temperature data gathered by these vessels was meant to bolster the ECM data in showing real-world activity of the engines. The insight gained by this showed that these vessels spent most of the time below 100°C, which could indicate low load conditions. Using two vessels with both types of loggers, a connection between the engine load and exhaust temperature was attempted. However, the result of this did not materialize into a meaningful correlation that could be interpreted. The final analysis combined the results of the real-world activity and emission data provided from a similar vessel to determine a comparison of the estimated emissions between real-world activity data and the certification cycle presumed activity. A significant overestimation of the emissions was observed from the certification cycle. The E3 test cycle for these vessels did not represent real-world activity patterns of these tested vessels and showed that emission calculations may be higher than in actuality. This may mean that this cycle should not be used to estimate emissions for emission inventory. For further study of the limitations of the E3 certification cycle, it is recommended to gather more ECM data samples of various harbor craft and to couple it with real-world emission testing for more direct emission factor comparisons.
Supplemental Material
Download MS Word (608.8 KB)Disclosure statement
No potential conflict of interest was reported by the authors.
Supplementary material
Supplemental data for this paper can be accessed on the publisher’s website
Additional information
Notes on contributors
Chas Frederickson
Chas Frederickson is a graduate at the University of California Riverside.
J. Wayne Miller
J. Wayne Miller is an adjunct professor at the Department of Chemical and Environmental Engineering, and associate director at CE-CERT at the University of California Riverside.
Heejung Jung
Heejung Jung is a professor at the University of California Riverside.
References
- Aldrete, G., B. Anderson, J. Ray, M. Carlock, and S. Wells. 2007. Port of Long Beach air emissions inventory–2005. Poulsbo, WA: Starcrest Consulting Group, LLC.
- Browning, L., and K. Bailey. 2006. Current methodologies and best practices for preparing port emission inventories. ICF Consulting report to Environmental Protection Agency.
- CARB. 2010. Staff report: Initial statement of reasons for proposed rulemaking: Proposed amendments to the regulation for in-use off-road diesel-fueled fleets and the off-road large spark-ignition fleet requirements, Branch, M. S. C. D. E. R. I., ed.
- CARB. 2012. Emissions estimation methodology for commercial harbor craft operating in California. https://ww3.arb.ca.gov/msei/chc-appendix-b-emission-estimates-ver02-27-2012.pdf
- Cohen, A. J., H. Ross Anderson, B. Ostro, K. D. Pandey, M. Krzyzanowski, N. Künzli, K. Gutschmidt, A. Pope, I. Romieu, and J. M. Samet. 2005. The global burden of disease due to outdoor air pollution. J. Toxicol. Environ. Health Part A 68 (13–14):1301–07. doi:https://doi.org/10.1080/15287390590936166.
- Corbett, J. J., and A. L. Robinson. 2001. Measurements of NOx emissions and in-service duty cycle from a Towboat operating on the Inland River System. Environ. Sci. Technol. 35 (7):1343–49. doi:https://doi.org/10.1021/es0016102.
- Corbett, J. J., J. J. Winebrake, E. H. Green, P. Kasibhatla, V. Eyring, and A. Lauer. 2007. Mortality from ship emissions: A global assessment. Environ. Sci. Technol. 41 (24):8512–18. doi:https://doi.org/10.1021/es071686z.
- Gysel, N. R., R. L. Russell, W. A. Welch, and D. R. Cocker III. 2016. Impact of aftertreatment technologies on the in-use gaseous and particulate matter emissions from a tugboat. Energy Fuels 30 (1):684–89. doi:https://doi.org/10.1021/acs.energyfuels.5b01987.
- ISO. 1996. 8178-2. Reciprocating internal combustion engines: Exhaust emission measurement. Part-2: Measurement of gaseous and particulate exhaust emissions at site. International Organization of Standardization. https://www.iso.org/standard/15269.html
- Jayaram, V., M. M. Y. Khan, J. W. Miller, M. W. A. Welch, K. Johnson, and D. R. Cocker. 2010. Evaluating emission benefits of a hybrid tug boat, in Final Report to California Air Resources Board, 46–47.
- Pope, C. 3rd 2000. Epidemiology of fine particulate air pollution and human health: Biologic mechanisms and who’s at risk? Environ. Health Perspect. 108 (suppl 4):713–23. doi:https://doi.org/10.1289/ehp.108-1637679.
- Pope III, C. A., and D. W. Dockery. 2006. Health effects of fine particulate air pollution: Lines that connect. J. Air Waste Manage. Assoc. 56 (6):709–42. doi:https://doi.org/10.1080/10473289.2006.10464485.
- Rao, V., L. Tooly, and J. Drukenbrod. 2013. 2008 national emissions inventory: Review, analysis and highlights. https://www.epa.gov/sites/production/files/2018-06/documents/2008report.pdf
- Rosenbaum, A., S. Hartley, and C. Holder. 2011. Analysis of diesel particulate matter health risk disparities in selected us harbor areas. Am. J. Public Health 101 (S1):S217–S223. doi:https://doi.org/10.2105/AJPH.2011.300190.
- Starcrest. 2020a. Port of Long Beach 2019 air emissions inventory. https://polb.com/download/14/emissions-inventory/10596/2019-air-emissions-inventory.pdf
- Starcrest. 2020b. Port of Los Angeles inventory of air emissions. https://kentico.portoflosangeles.org/getmedia/4696ff1a-a441-4ee8-95ad-abe1d4cddf5e/2019_Air_Emissions_Inventory
- Tang, L., M. O. P. Ramacher, J. Moldanová, V. Matthias, M. Karl, L. Johansson, J.-P. Jalkanen, K. Yaramenka, A. Aulinger, and M. Gustafsson. 2020. The impact of ship emissions on air quality and human health in the Gothenburg area – Part 1: 2012 emissions. Atmos. Chem. Phys. 20 (12):7509–30. doi:https://doi.org/10.5194/acp-20-7509-2020.
- U.S. EPA. 2009. Control of air pollution from new motor vehicles and new motor vehicle engines, 8309-8428. https://www.federalregister.gov/documents/2009/02/24/E9-2405/control-of-air-pollution-from-new-motor-vehicles-and-new-motor-vehicle-engines-regulations-requiring
- U.S. EPA. 2020. Port emissions inventory guidance: Methodologies for estimating port-related and goods movement mobile source emissions. Office of Transportation and Air Quality, U.S. Environmental Protection Agency.
- Vutukuru, S., and D. Dabdub. 2008. Modeling the effects of ship emissions on coastal air quality: A case study of southern California. Atmos. Environ. 42 (16):3751–64. doi:https://doi.org/10.1016/j.atmosenv.2007.12.073.
- Wang, C., J. J. Corbett, and J. Firestone. 2007. Modeling energy use and emissions from North American shipping: Application of the ship traffic, energy, and environment model. Environ. Sci. Technol. 41 (9):3226–32. doi:https://doi.org/10.1021/es060752e.
- Wu, Y., R. Wang, Y. Zhou, B. Lin, L. Fu, K. He, and J. Hao. 2011. On-road vehicle emission control in beijing: Past, present, and future. Environ. Sci. Technol. 45 (1):147–153.
- Yau, P., S. Lee, Y. Cheng, Y. Huang, S. Lai, and X. Xu. 2013. Contribution of ship emissions to the fine particulate in the community near an international port in Hong Kong. Atmos. Res. 124:61–72. doi:https://doi.org/10.1016/j.atmosres.2012.12.009.