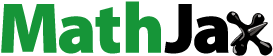
ABSTRACT
Along with the increasingly stringent dust emission concentration standards for the thermal power plants and the challenge of market competition, wet electrostatic precipitators (ESPs) have been widely used as terminal equipment for the flue gas treatment. The wet ESPs pilot test platform using the modified carbon steel collectors with hydrophilic properties was established in this paper and the experimental research on PM2.5 removal characteristics were carried out. The mechanism of the particles removal efficiency enhancement by water film on the surface of modified collectors was studied. The effect of gas temperature, residence time, working voltage, inlet concentration, and flushing water flowrate on the particles removal efficiency was investigated. The results indicated that the fibrous layer over the surface of the modified collectors could reduce the effect of the airflow recoil and the electromigration resistance of particles. The surface of the modified collectors could also maintain a uniform and stable water film with a lower amount of water consumption. The water film could restrain the back corona and the re-entrainment of dust. And the evaporation of water film increased the humidity of the flue gas, meanwhile, the particles charge capacity and electromigration velocity increased. Those all could improve the particles removal efficiency. The particles removal efficiency increased with the increase of the flue gas residence time and the applied voltage, but the particles removal efficiency improved less with the increase of the particles inlet concentration and flushing water flow rate after maintaining uniform and stable water film. The modified rigid collector provided high removal efficiency for particles with diameters of 0.04~0.48 μm under lower energy and water consumption.
Implications: The wet electrostatic precipitators (ESPs) have been widely used as terminal equipment of the flue gas treatment to purify the flue gas deeply. The surface of the modified carbon steel collectors with hydrophilic properties could maintain a uniform and stable water film with a lower amount of water consumption. The evaporation of water film improved the particle charge capacity and electromigration velocity. The wet ESPs using the modified rigid collector exhibited significantly higher removal efficiency of particles than using the conventional rigid collector especially to the particles with diameter of 0.04~0.48 μm.
Introduction
The fine particles formed by the thermal power plants (TPPs) are one of the main emission sources of the atmospheric fine particles (Cui et al. Citation2018a; He et al. Citation2015; Li et al. Citation2018). Large boilers of steelworks and industrial stoves are also the main emission sources (Zhao et al. Citation2014). Those are closely related to the large-scale and long-term severe haze weather in China. The fine particles have the characteristics of irregular shape, large specific surface area, and light weight. Because of this, the fine particles could suspend in the air for a long time and are easier to carry heavy metal and toxic organic substances, bacteria, and viruses, etc. (Cox et al. Citation2017; Rashdi et al. Citation2015; Sun et al. Citation2015; Zhao et al. Citation2013; Gu Citation2019). As a result, the fine particles have many hazardous effects on the public health and atmospheric environment. The fine particles could easily invade into human respiratory system then cause respiratory diseases and even lung cancer (Cox et al. Citation2017; Tang et al. Citation2016, Citation2014; Xie et al. Citation2019; Miller et al. Citation2016). The fine particles also have a very important influence on the atmospheric visibility, urban heat island, regional environment, and climate (Chen et al. Citation2020; Tang et al. Citation2017; Tian et al. Citation2015), thus become an environmental pollution problem attracting extensive attention from the whole society in China (Gao et al. Citation2015; Li et al. Citation2016; Yang et al. Citation2016).
At present, there are 90%~95% TPPs in China adopting dry ESPs for dust removal (Pan, Cui, and Ma Citation2016), and the removal efficiency of the coarse particles with diameter over 10 μm using the dry ESPs can be as high as 99.9% (Yang et al. Citation2018). However, the removal efficiency of fine particles (diameter less than 10 μm) is unsatisfactory due to their poor charge capacity and low electrical mobility, especially the particles diameter in the range of 0.1 ~ 1.0 μm are easy to escape from the dry ESPs (Xiong et al. Citation2015; Zhou et al. Citation2016). To decrease the discharge amount of the fine particles formed by the TPPs, the dust emission concentration standards for the TPPs became increasingly stringent, from 30 mg/m3 in 2011 to 10 mg/m3 in 2014, in China (MEP Citation2011; NDRC Citation2014). And ultra-low emissions (ULE) has been put forward to further reduce the PM emission to 5 mg/m3 especially in some provinces (DEESP Citation2019; PGZP Citation2018; Yu Citation2017; Yuan et al. Citation2017; Zhao et al. Citation2017). Such stringent pollutant emission standards pose great challenges for flue gas treatment systems in TPPs (Cui et al. Citation2018b). Using the dry ESPs as the only fine particles removal technology is difficult to satisfy the current exhaust laws. In this situation, several efficient dust removal technologies have been promoted and applied in the TPPs such as wet ESPs (Zheng et al. Citation2018), low–low temperature ESPs (Shou et al. Citation2016; Li et al. Citation2017; Hu et al. Citation2017), hybrid ESP/BAG devices (Shi et al. Citation2016), agglomeration technology (Zhang and Liao Citation2015; Xu et al. Citation2009) and so on. And among the above advanced treatment technologies to remove the fine particles deeply, the wet ESPs are used most widely (Yin Citation2019).
The wet ESPs technology adopts the water film to clean the collected dust and that could avoid the re-entrainment of dust effectively (Sui et al. Citation2016). The wet ESPs technology also have high corona current and obvious effect of particle agglomeration. Besides, there is no limit to the specific resistance of the dust to be treated. As a results, the wet ESPs technology exhibits obvious technical advantages in the emission controlling and reduction of the fine particles. The wet ESPs are normally installed behind the wet flue gas desulfurization serving as terminal control equipment to purify the flue gas deeply (Xu et al. Citation2016). In general, the flue gas temperature in wet ESPs is cooled down to 40 ~ 70°C (Anderlohr et al. Citation2015; Córdoba et al. Citation2013; Jiang et al. Citation2014; Mertens et al. Citation2014). Currently, the materials of the collectors used basically includes: 316 L stainless steel, dual-phase steel, conductive FRP, carbon or silicon fiber, organic fiber, and so on (Bayless et al. Citation2005; Chang et al. Citation2011; Jiang et al. Citation2014). In general, honeycomb or plate-type structure is used to make the collectors surfaces act as the carrier of water film (Ding et al. Citation2015).
At present, the rigid collectors still have some problems to improve, such as consuming massive washing water, non-uniform distribution of washing water and shutdown washing operation and so on. The poor tensioning and fixation of the fiber collectors could cause themselves deformation and vibration. And then the space between the electrodes could change. That could cause the instability of the electric field. Therefore, the research on modification methods for conventional rigid collectors has become a hot topic. The reasonable modification could bring well wetting properties with ultra-low water consumption to ensure the stable operation of the wet ESPs. That is supposed to solve the traditional problems.
The dust removal efficiency of ESPs can be calculated by the Deutsche formula which is the theoretical basis of classical dust removal (Deutsch Citation1922). According to the formula, the main parameters that affect the dust removal efficiency are specific dust collecting area, electrostatic field strength of dust collecting area, gas temperature (which affects the gas viscosity), etc. The water film over the surface of the collecting electrode in the wet ESPs also has an important effect on the dust removal (Wang Citation2018, Citation2013) which is related to the flushing water flowrate. In this study, the wet ESPs pilot test platform using the modified carbon steel collectors with hydrophilic properties was established in this paper and the experimental research on PM2.5 removal characteristics were carried out. The mechanism of the particles removal efficiency enhancement by water film on the surface of modified collectors was studied. The effect of gas temperature, residence time, applied voltage, inlet concentration, and flushing water flowrate on the particles removal efficiency was investigated and discussed.
Experimental
Experimental system
A schematic diagram of the experimental system is shown in . The system mainly consisted of a laboratory scale ESPs model, a water film distribution system, a simulated flue gas system, and an induced draught system.
Figure 1. Schematic diagram of the experimental apparatus: 1, wet ESPs shell; 2, insulation; 3, DC power; 4, rotameter; 5, water distribution pipe; 6, collecting electrodes; 7, discharge electrodes; 8, water tank; 9, circulating water; 10, circulating pump; 11, aerosol generator; 12, particles feed port; 13, heater; 14, induced draft; 15, regulating valve; 16, export sampling port; 17, inlet sampling port
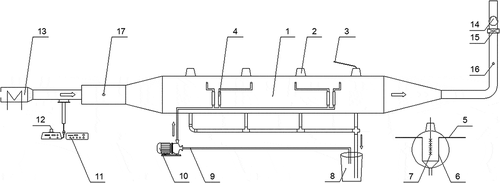
The ESPs model adopted the horizontal wire-plate structure. The distance between the discharge and the collecting electrodes was 200 mm. As shown in the ), the discharge electrodes were made of eight parallel nichrome barbed wire with each length of 500 mm. A high-voltage power supply was connected with the discharge electrodes to establish the electrostatic field and generate ions. The power supply could generate an adjustable DC negative voltage from 0 kV to 70 kV and a maximum current of 10 mA. The total discharge current and applied voltage could be acquired directly from the display panel of the high-voltage supply. As shown in the ), the modified collecting electrodes (MCE) were prepared referred to GFCIII in the reference (Xu et al. Citation2015), each collecting electrode was 500 × 500 mm2, and the total useful length was 4 m. The MCE were connected to the nylon fabric with insulation sheet and bolts; the water pipe was wrapped in the nylon fabric (See Patent No.: CN201320818783.5 for installation details). The nylon fabric acted as an auxiliary means of water film distribution. The conventional rigid collectors (CRC) adopted the frequently-used type of Z480, each collector was 500 mm in height and 385 mm in width, and the total useful length was 4 m.
Figure 2. The structure and appearance of electrodes: (a) the discharge electrodes; (b) the collecting electrodes
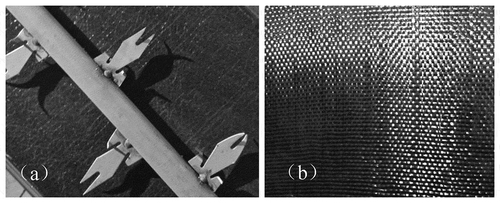
The water film distribution system supplied continuous water film on the surface of the modified collecting electrodes. The charged fine particles transferred to the collecting electrodes and were arrested by the water film under the effect of the electric field. Then, the water containing the collected particles entered the water tank to deposit. After that the circulating pump pumped the supernatant water to the water distribution pipe. The rotameter regulated the test flow rate range of 0 ~ 160 L/hr.
Compressed air was introduced to the simulated flue gas system, which composed of an aerosol generator (Topas SAG-410, Germany) and an aerosol electrostatic neutralizer (Topas EAN-581, Germany). The aerosol generator could uniformly import the screened fine particles into the aerosol electrostatic neutralizer through the belt conveyor, the concentration of particles could be controlled by adjusting the belt speed. The aerosol electrostatic neutralizer could eliminate the effect of electrostatic agglomeration on the particles diameter caused by friction between the fine particles, so that the fine particles could be distributed evenly and stably into the flue gas.
The main flue gas was generated by the induced draught system which composed of electrical heaters and an induced draft. The flue gas temperature could be raised from room temperature to 100°C by the electric heaters and controlled by changing the electric heating power. To reduce undesired heat loss, all pipes were covered with thermal insulation material. During the experiments, the compressed air carrying the suitable amount of particles mixed with the heated air generated by the electrical heaters. Then, the flue gas was introduced into the test section by the induced draft. The residence time of the fine particles in the test section was controlled by the regulating valve adjusting the flue gas velocity. The commonly used specific collecting electrode areas (SCA) in the engineering applications were 10 ~ 20 m2/(m3/sec) (Zhang et al. Citation2015). Here the residence time was adjusted from 1.6 to 4.0 seconds and the SCA was about 8~20 m2/(m3/sec). Sampling ports were set in the inlet section and the outlet section, respectively.
Experimental particles
The test particles were obtained from the final ESPs stage ash hopper of a pulverized coal boiler through the screening by a supersonic wave oscillating screen (625 mesh). The number concentration of the fine particles ()) was tested with an electrical low-pressure impactor (ELPIVI 4.00, Dekati Ltd., Finland). The number concentration of the fly ash was kept at (4.0 ± 0.2)×105 1/cm3 during the experiments. The particles’ morphology ()) was measured by the thermal field emission scanning electron microscopy (SEM, SUPRA™ 55, Germany). The component of the fine particles tested with the X-ray fluorescence (XRF, Thermo Scientific PW4400, USA) was also shown in ).
Experimental methods
The temperature of the flue gas was measured by the type K thermocouples with the test range of – 40 ~ 99°C with the measurement error of ±0.1°C.
The gas velocity was measured by hot-bulb anemometer (QDF-3, Beijing testing instrument co., Ltd., China) with the test range of 0.05 ~ 30 m/sec with the measurement error of ±4% U.
The mass concentration of particles entering and leaving the wet ESPs was measured by gravimetric method through constant velocity sampling (GB16157-Citation1996 Citation1996; HJ836-Citation2017 2018) using a dust meter (3012 H, Laoshan Applied Technology Research Institute, China).
The total dust removal efficiency (η) can be derived as:
where, Cin is the mass concentration of particles entering the wet ESPs (mg/m3) and Cout is the mass concentration leaving the wet ESPs (mg/m3).
The number concentration of the fine particles was tested by the electrical low-pressure impactor in real time at the export sampling port. The electrostatic low-pressure impactor mainly consisted of sampling probe, cyclone cutter, corona charger, impactor, electrometers, and vacuum pump. The vacuum pump was used to maintain a constant airflow rate of 10 L/min. Under the suction action of the vacuum pump, the particles successively passed through sampling probe, the cyclone cutter, the corona charge, and then enter the impactor to realize the classification of fine particles. The coarse particles larger than 10 μm were removed from the gas passing through the cyclone cutter. Then, the particles were charged to a known charge level in the corona charger. After charging, the charged particles were classified into 12 stages and separately collected in the impactor according to their aerodynamic diameter. The charged particles could generate induced current, which was detected by sensitive electrometer and the signal was inverted to calculate particles number concentrations.
The classification removal efficiency of the particles with diameter of di (ηdi) can be derived as:
where, Nin(di) is the number concentration of particles entering the wet ESPs (1/cm3) and Nout(di) is the number concentration leaving the wet ESPs (1/cm3). di is the aerodynamic diameter of the test particles.
Results and discussion
Performance of the MCE
) shows the Voltage-current (V–I) characteristics of the ESPs with the wet MCE and dry CRC. The particles inlet mass concentration (Cin) was 70 mg/m3, the residence time (t) of the particles stayed in the wet ESPs shell was 4 seconds and the temperature (T) of the flue gas was 20°C. The surface flushing water flowrate (F) over the MCE was about 20 L/h and the surfaces could be covered with water film. The applied voltage (V) for the wet MCE increased from zero to 48 kV while that for the dry CRC increased from zero to 60 kV.
Figure 4. Performance comparison between the MCE and the CRC. (a) V–I characteristics, (b) The total dust removal efficiency. (Cin: 70 mg/m3; t: 4 s; T: 20 °C; F:20 L/h)
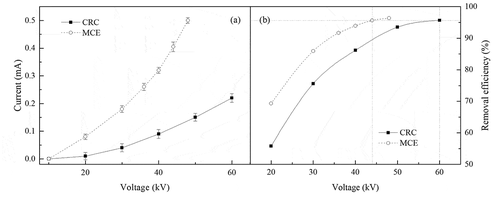
As shown in the figure, there is a nonlinear relationship between the current and the voltage. With the increase of the applied voltage, the slope of the V–I curve using the CRC changed gently with the applied voltage increasing. While the slope of the V–I curve using the MCE gradually increased which meant that the higher the voltage increased, the faster the current raised. When the applied voltage was small, the number of free electrons and ions generated by the gas ionization was also small, and the gas impedance was large. With the increase of the applied voltage, more free electrons and ions through the electron avalanche under the bombardment of more high-energy electrons were generated which rapidly enhanced the conductivity of the gas. As a result, the corona current rose with the increase of the applied voltage (Musumeci and Pollack Citation2012; Yan et al. Citation2007). This indicates that the MCE do not change the basic law of the V–I characteristics compared with the CRC.
But the corona current using the MCE was about 3 ~ 5 times higher than the CRC at the same applied voltage. The existence of water film on the surfaces of collecting electrodes using the MCE increased the corona current significantly. There were two mechanisms enhanced the corona discharge capability of wet electrostatic field. On one hand, driven by partial pressure of water vapor and ion wind, water molecules diffused into the discharge space and the collision ionization in the ionization zone produced more positive ions and electrons at the same applied voltage (Wang et al. Citation2009). On the other hand, the accumulation of negative water ions in the discharge space enhanced the electrostatic field intensity near the wet collecting electrodes (Mazen Citation1985; Wang Citation2013).
) shows the influence of the applied voltage on the total dust removal efficiency using the wet MCE and dry CRC. It could be seen from the figure that the total dust removal efficiency with both two collectors increased with the increase of applied voltage. Because the average electric field intensity and the space charge density in the electrostatic precipitator increased with the increase of the applied voltage, so that the particles removal efficiency was significantly improved (Wang Citation2013). And at the same applied voltage, the dust removal efficiency using the MCE was higher than the CRC, and the dust removal efficiency using the MCE at the applied voltage of 44 kV could catch up that with the CRC at the applied voltage of 60 kV. The wet ESPs had higher corona power than the dry ESPs, which strengthened the charge migration process of particles in the electrostatic field (Jędrusik and Swierczok Citation2013). And the particles was easier to agglomerate into large particles which were easy to be removed due to the presence of water molecules in the space. At the same time, the charge capacity of the particles became strengthened because of the collision between particles and water molecules (Wan Citation2014). Those were all advantageous to the particles removal. Besides, after reaching the collecting electrodes, the fine particles were captured and washed away by the water film which could eliminate the secondary remixing completely (Sui et al. Citation2016).
Effects of the water film on the removal efficiency
shows the influence of water film on the classification removal efficiency of the particles. The particles inlet mass concentration was 70 mg/m3, the residence time of the particles stayed in the wet ESPs shell was 4 seconds and the temperature of the flue gas was 20°C. The applied voltage was 40 kV. The surface flushing water flowrate over the MCE was about 20 L/h and the surface could be covered with water film.
Figure 5. Influence of the water film on the classification removal efficiency of particles. (a) Particles distribution using the MCE with dry and wet surfaces, (b) The classification dust removal efficiency comparison of particles using the MCE and CRC. (Cin: 70 mg/m3; t: 4 s; T: 20 °C; V: 40kV; F:20 L/h)
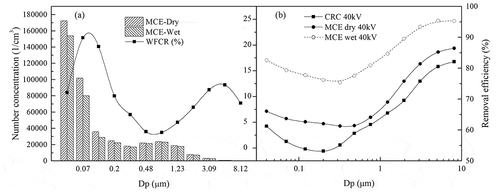
) shows that the particles number concentration using the MCE with dry and wet surfaces and the variation ratio of the particles number concentration. Here we define the variation ratio for the water film capture ratio (WFCR).
where, Cdry is the number concentration of particles using the dry MCE (mg/m3) and Cwet is the number concentration of particles using the wet MCE (mg/m3).
As shown, the fine particles were captured by the water film when they collide with the water film which could reduce the secondary remixing. And the particles with diameters smaller than 0.48 μm decreased significantly than others with water film surface. While the WFCR presented a bimodal trend. The particles with diameters between 0.32 μm and 1.96 μm located in the lower ratio. This indicated that the lower removal efficiency of particles with these diameters without external electrostatic field force was related to the properties of the particles themselves.
) shows the classification removal efficiency of particles using the CRC with dry surfaces and using the MCE with dry and wet surfaces coupling voltage. As shown, the MCE with dry surfaces presented higher removal efficiency than the CRC at same applied voltage especially to the particles with diameter of 0.04 ~ 0.48 μm. In the dry condition, the particles reached the CRC surfaces could break away to form re-entrainment under the effect of the turbulent airflow and corona wind. While the fibrous layer over the MCE surfaces could decrease the recoil airflow and the electromigration resistance of particles.
After adding the external electrostatic field force, the particles removal efficiency with wet surfaces increased much more than that with the dry surfaces using the MCE. When the MCE surfaces were uniformly covered with water film, the particles removal efficiency increased a lots more than the CRC with dry surfaces. On the one hand, there was no “dry spots” over the MCE surfaces with uniform water film, the particles once reached the water film and then were washed away without the back corona and re-entrainment. On the other hand, the water film evaporation improved the humidity of the flue gas resulting in lower onset corona voltage and stronger discharge capacity (Xiong et al. Citation2015). The water molecule and the oxygen molecule serving as carrier could form a large number of negative ions at the same voltage, and the space charge density increased significantly (Jin Citation2013), and particles charge capacity and electromigration velocity increased significantly compared with that in dry condition.
Effects of the gas temperature on the removal efficiency
The gas temperature had important influence on the particles removal efficiency to the wet ESPs. It could affect the discharge characteristics of the wet ESPs, as well as the specific resistance of particles. Through the heat transfer during the water film evaporation, it could also affect the humidity of the gas. The shows the effect of the flus temperature on the classification removal efficiency of particles. The particles inlet mass concentration was 65 mg/m3, the residence time of the particles stayed in the wet ESPs shell was 4 seconds and the temperature of the flue gas was 20 and 60℃. The applied voltage was 40 kV. The surface flushing water flowrate over the MCE was about 20 L/h.
Figure 6. Effects of flue temperature on the dust removal efficiency. (Cin: 65 mg/m3; t: 4 s; T: 20, 60°C; V: 40kV; F:20 L/h)
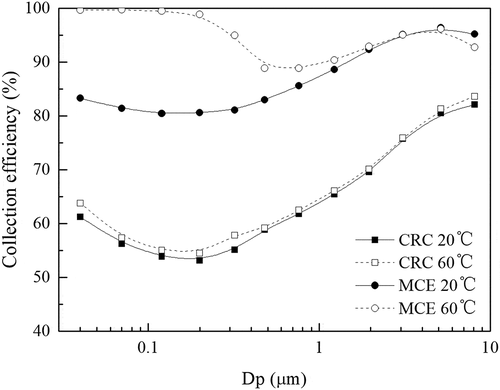
As shown in the figure, when the flus temperature increased from 20℃ to 60℃, the particles removal efficiency for large particles over 1 μm using the MCE varied slightly, while the removal efficiency of small particles less than 1 μm increased much. Noll (Noll Citation2002) found in the experiment that when the gas temperature rose, the corona current gradually increased, which was conducive to the improvement of particles removal efficiency. At the same time, with the increase of gas temperature, the thermophoretic force gradually increased, which was beneficial to improve the dust removal efficiency, and the thermophoretic force had a more significant effect on the removal of the small particles (Liu et al. Citation2010; Wang et al. Citation2011). Besides, water molecules in the water film on the surface of the collecting electrodes were continuously evaporating into the electrostatic field, and the collision probability between particles and water molecules increased. The small particles could agglomerate under the action of liquid bridge force (Zhao and Zhou Citation2016). However, with the increase of gas temperature, the gas viscosity increased, which had an inhibitory effect on the removal of particles. As a result, the removal efficiency of large particles over 1 μm varied slightly even decreased to the particles larger than 8.12 μm.
The particles removal efficiency using the CRC improved slightly. On the one hand, the corona current did not increase as much as it did in the wet state with the increase of flue gas temperature. On the other hand, with the increase of gas temperature, the real flue gas velocity in the test section increased and the particles residence time in the test section decreased. In addition, the particle re-entrainment would be more serious due to the increased gas velocity (You et al. Citation2010). Those all decrease the removal efficiency of the particles. The effect of temperature on the particles removal efficiency was complicated because of the interaction of these two opposite trends caused by the flus gas temperature increase.
And the removal efficiency of particles with diameter of 0.1 ~ 1 μm was lower compared to other diameters. The particles charging theory indicated that field charging was the dominant mechanism for particles larger than 1.0 μm and diffusion charging was the dominant mechanism for particles less than 0.1 μm, between these diameters, both mechanisms operated (White Citation1963). For the particles less than 1 μm, the thermophoresis effect was an important factor improving the removal efficiency. But, the electrostatic field force is the biggest factor affecting the removal efficiency of the particles (Pan Citation2016). As a result, removal efficiency using the CRC didn’t increase much. Therefore, the following experiments were carried out at room temperature in order to reduce operating variables and ensure the accuracy of ELPI measurement.
Effects of the residence time on the removal efficiency
shows the effects of gas residence time stayed in the wet ESPs shell on the classification removal efficiency of the particles. The particles inlet mass concentration was 70 mg/m3, the residence time of the particles stayed in the wet ESPs shell was 2 and 4 seconds and the temperature of the flue gas was 20℃. The applied voltage was 40 kV. The surface flushing water flowrate over the MCE was about 20 L/h. The figure shows that when the residence time increased from 2 s to 4 s, the removal efficiency of particles with diameter less than 1 μm using the MCE increased by 27 ~ 40%, and that with diameter larger than 1 μm increases by 7 ~ 20%.
Figure 7. Effects of gas residence time on the classification removal efficiency. (Cin: 70 mg/m3, t: 2,4s; T: 20°C; V: 40 kV; F: 20 L/h)
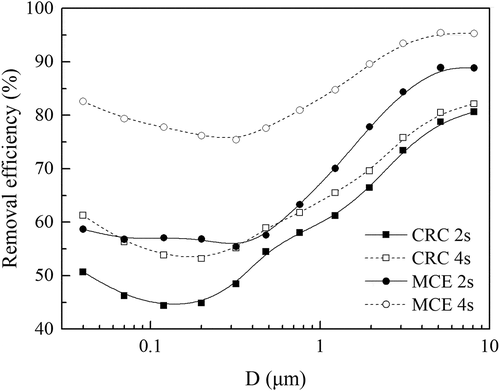
When the residence time was 4 s, the removal efficiency of particles using the MCE was significantly higher than that using the CRC. The removal efficiency of particles with diameter less than 1 μm increased by 30 ~ 40%, and particles with diameter larger than 1 μm increased by 16 ~ 30%. In addition, the particles removal efficiency using the MCE with residence time of 2 s was even higher than that using the CRC with a residence time of 4 s. This indicated that the wet ESPs using MCE were more able to deal with high-speed flue gas compared with the ESPs using CRC at the same collecting electrode areas.
In the experiment, the flus residence time was adjusted by regulating the air volume. The decrease of air volume led to the increase of residence time and the specific collecting electrode areas. That improved the removal of particles. The particle charging mechanism in the ESPs could be divided into two different subcategories, field, and diffusion charging. Under ideal conditions, the charged particles due to the field charging could be considered as saturated charging after entering the electrostatic field. When the residence time of particles in the charging area increased, the particles diffusion charging would increase (Xiong et al. Citation2014), and the particles had enough time to migrate to the surface of the collecting electrodes (Wan Citation2014). So that the removal efficiency of particles increased. At same time, it could prove that the diffusion charging was more obvious to small particles.
Effects of the applied voltage on the removal efficiency
The applied voltage was one of the important parameters in the wet ESPs, which affected the migration characteristics and removal efficiency of particles through directly affecting the distribution of electrostatic field and charge density inside the ESPs.
shows the effects of applied voltage on the classification removal efficiency of particles. The particles inlet mass concentration was 70 mg/m3, the residence time of the particles stayed in the wet ESPs shell was 4 s and the temperature of the flue gas was 20°C. The applied voltage was 30, 40, 44, 60 kV. The surface flushing water flowrate over the MCE was about 20 L/h.
Figure 8. Effects of applied voltage on the classification removal efficiency of particles. (Cin: 70 mg/m3; t: 4 s; T: 20°C; V: 30,40,44,60 kV; F:20 L/h)
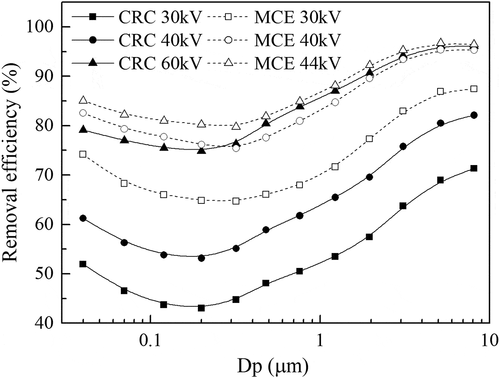
As shown in the figure, the removal efficiency of particles with diameter of 0.1 ~ 1 μm was lower compared to other diameters and the removal efficiency all presented V-shaped distribution. That was consistent with the particles charging theory (Wan Citation2014). When the applied voltage increased from 30kV to 60kV, the removal efficiency of particles using the CRC increased distinctly. The applied voltage increased, the space charge density increased, the charge capacity of particles increased, and the particles migration velocity increased (Zhang et al. Citation2015). The particles were more likely to reach the surfaces of the collecting electrodes and then be captured under the action of electrostatic field.
To compare the removal efficiency between the MCE and the CRC, here we define a parameter that is the relative removal efficiency. The relative removal efficiency is the specific value of the particles removal efficiency using wet MCE to that of using dry CRC at the same applied voltage and other operating conditions. As shown in the ), the dust removal efficiency using MCE at the applied voltage of 44 kV could catch-up with that using the CRC at the applied voltage of 60 kV. In view of this situation, the relative removal efficiency was compared between using the MCE with the applied voltage of 44kV and using the dry CRC with the applied voltage of 60kV.
As shown in the , the relative removal efficiency at the applied voltage of 30kV present double peak phenomenon with the increase of particles diameter. The relative removal efficiency of particles with diameter of 0.1 and 2 μm appeared local maximum values. The relative removal efficiency at the applied voltage of 40kV first increased and then decreased. The relative removal efficiency at the applied voltage of 44kv first decreased, then increased and then decreased with the increase of particle diameter. Those were different to the wet penetrating polypropylene collectors (Wang et al. Citation2016). The relative removal efficiency decreased when the applied voltage increased from 30kV to 40kV except for the particles with diameter between 0.2 and 0.76 μm. This meant that the improvement effect of the water film on the particles removal efficiency was more obvious at lower applied voltage. And the coupling action of the water film and electrostatic field effectively improved the removal efficiency of particles with diameters between 0.2 and 0.76 μm. When the applied voltage continued to increase from 40kV, the relative removal efficiency decreased much. The results showed that the improvement effect of wet ESPs on particles removal efficiency was more obvious when the voltage was low. And the effect of raising the applied voltage on the particles removal efficiency was limited when the applied voltage raised to a certain degree.
Effects of inlet mass concentration of particles on the removal efficiency
shows the effects of particles inlet mass concentration on the removal efficiency of particles. The particle inlet mass concentration was 37, 94, 167 mg/m3 at the applied voltage of 30, 40, 44 kV using the MCE. The particle inlet mass concentration was 40, 90, 160 mg/m3 at the applied voltage of 30, 40, 60 kV using the CRC. The residence time of the particles stayed in the ESPs shell was 4 s and the temperature of the flue gas was 20℃. The surface flushing water flowrate using the MCE was about 20 L/h. As shown in the figure, the particles removal efficiency improved less with the particles inlet concentration increase. At the same time, the improvement in particles removal efficiency began to slow down with the increase of the voltage.
Figure 10. Effects of particle inlet mass concentration on the removal efficiency. (Cin:37 ~ 167 mg/m3; t: 4 s; T: 20°C; V: 30 ~ 44, 60 kV; F: 20 L/h)
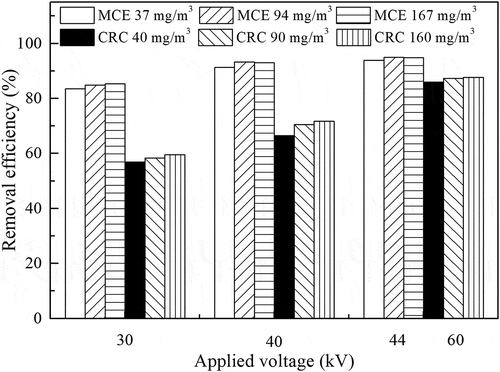
When the applied voltage was constant, with the increase of particles concentration in the flus gas, the collision distance between particles decreased and the collision probability increased. So that small particles had more probability to agglomerate into large particles. Besides, the number of charged particles increased, the distribution of electric field forces on particles would change. The particles in the near-electrodes region were affected by stronger electric field force, and their migration speed increased. As a result, the particles removal efficiency increased (Wan Citation2014). At the same time, the particles concentration in the flue gas increased, the number of charged particles increased. As a result, the charged particles in the space increased which would inhibit the generation of corona current. So that the particles couldn’t obtain sufficient charge. That could decrease the particles removal efficiency (Hu, Zhao, and Liu Citation2006). Under the simultaneous action of these two opposite trends, the increase of removal efficiency with the particle inlet concentration was not obvious.
Effects of flushing water flowrate on the removal efficiency
shows the effects of surface flushing water flowrate on the removal efficiency of particles. The particle inlet mass concentration was 70 mg/m3, the residence time of the particles stayed in the wet ESPs shell was 4 s and the temperature of the flue gas was 20°C. The applied voltage was 40 kV. The surface flushing water flowrate over the MCE was about 20 ~ 60 L/h.
Figure 11. Effects of flushing water flowrate on the removal efficiency. (Cin: 70 mg/m3; t: 4 s; T: 20°C; V: 40 kV; F: 20 ~ 60 L/h)
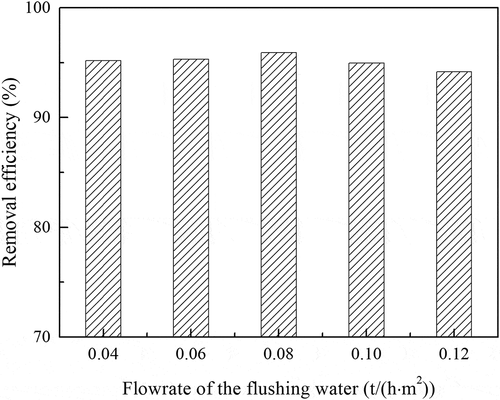
As shown in the figure, the increase of surface-flushing water flowrate had little influence on the removal efficiency of particles. A stable water film had formed over the surfaces of the MCE with the flushing water flowrate of 20 L/h (that was, the surface water supply was 0.04 t/(h·m2)). The uniform stability of water film has an important effect on the reliable and secure operation of wet ESPs. Usually, the water film on the surfaces of metal-plate collecting electrodes is impossible to achieve uniform distribution due to the liquid surface tension and the surface machining flatness deviation, which could result in water channels, dry spots, localized corrosion, and induced spark discharge during flushing process. So that the particles removal efficiency of wet ESPs would increase with the increase of flushing water flowrate when the water film was not uniform . After the collecting electrodes maintaining stable water film, the increase of water flowrate had no significant influence on the particle removal efficiency of wet ESPs in the experiment (Wan Citation2014; Wang Citation2013). When the flushing water flow rate increased to 60 L/h, the water would flow out of the holes of the pipe at a higher speed, which would lead to spatter. That would go against to the stable operation of the wet ESPs. Therefore, in the practical operation of wet ESPs, a small flushing water flowrate would be selected under the premise of ensuring the stable operation of the system. The reference (Wang Citation2013) concluded that the minimum water consumption using the stainless-steel plate was 0.3 t/(h·m2). In this experiment, the minimum water consumption forming stable and uniform water film was 0.04 t/(h·m2), which was far lower than that using the stainless-steel plate. This indicated that the MCE had great advantage in water conservation in the operation of wet ESPs.
Conclusion
The particles removal performance using the MCE with hydrophilic properties in wet ESPs was studied. The surface of the MCE could maintain a uniform and stable water film with a lower amount of water consumption. The water film could restrain the back corona and the re-entrainment of particles during the operation. And the evaporation of water film increased the humidity of the flue gas, meanwhile, the particles charge capacity and electromigration velocity increased. Besides, the fibrous layer over the MCE surfaces could decrease the recoil airflow and the electromigration resistance of particles. Those all could improve the particles removal efficiency using the MCE especially to the particles with diameter of 0.04 ~ 0.48 μm. The particles removal efficiency increased with the increase of the flue gas residence time and the applied voltage, but the particles removal efficiency improved with the increase of the particles inlet concentration and flushing water flow rate after maintaining uniform and stable water film. The increase of flue gas temperature could increase the removal efficiency of small particles less than 1 μm because of the thermophoretic force.
Disclosure statement
The authors declare no conflict of interest.
Additional information
Funding
Notes on contributors
Chunyan Xu
Chunyan Xu is a postdoctoral researcher.
Pengfei Chen
Pengfei Chen is a Master candidate.
Jingcai Chang
Jingcai Chang is a professor at the School of Environmental Science and Engineering, Shandong University, China.
Zhenlei Deng
Zhenlei Deng is an engineer at SanRong Environmental Protection Company, China.
Shan Qing
Shan Qing is a professor at the Faculty of Metallurgical and Energy Engineering, Kunming University of Science and Technology, China.
References
- Anderlohr, C., L. Brachert, J. Mertens, and K. Schaber. 2015. Collection and generation of sulfuric acid aerosols in a wet electrostatic precipitator. Aerosol. Sci. Technol. 49 (3):144–51. doi:https://doi.org/10.1080/02786826.2015.1008624.
- Bayless, D. J., L. M. Shi, G. Kremer, B. J. Stuart, J. Reynolds, and J. Caine. 2005. Membrane-based wet electrostatic precipitation. Air Repair 55 (6):784–91.
- Chang, J. C., Y. Dong, Z. Q. Wang, P. Wang, P. Chen, and C. Y. Ma. 2011. Removal of sulfuric acid aerosol in a wet electrostatic precipitator with single terylene or polypropylene collection electrodes. J. Aerosol. Sci. 42 (8):544–54. doi:https://doi.org/10.1016/j.jaerosci.2011.05.006.
- Chen, C., L. D. AMu, C. L. Li, J. X. Sun, and H. Li. 2020. Impacts of atmospheric fine particulate matter (PM2.5) on urban heat island with multi-source data: A case study of Beijing. J. Earth Environ. 11 (2):143–50.
- Córdoba, P., C. Ayora, N. Moreno, O. Font, M. Izquierdo, and X. Querol. 2013. Influence of an aluminium additive in aqueous and solid speciation of elements in flue gas desulphurisation (FGD) system. Energy 50:438–44. doi:https://doi.org/10.1016/j.energy.2012.11.020.
- Cox, L. A., Jr., X. B. Liu, L. H. Shi, K. Zu, and J. Goodman. 2017. Applying nonparametric methods to analyses of short-term fine particulate matter exposure and hospital admissions for cardiovascular diseases among older adults. Int. J. Environ. Res. Public Health 14 (9):1051. doi:https://doi.org/10.3390/ijerph14091051.
- Cui, L., X. D. Song, Y. Z. Li, Y. Wang, Y. P. Feng, L. F. Yan, and Y. Dong. 2018b. Synergistic capture of fine particles in wet flue gas through cooling and condensation. Appl. Energy 225 (SEP.1):656–67. doi:https://doi.org/10.1016/j.apenergy.2018.04.084.
- Cui, L., Y. Li, Y. Z. Tang, Y. F. Shi, Q. S. Wang, X. L. Yuan, and J. Kellett. 2018a. Integrated assessment of the environmental and economic effects of an ultra-clean flue gas treatment process in coal-fired power plant. J. Clean. Prod. 199:359–68. doi:https://doi.org/10.1016/j.jclepro.2018.07.174.
- DEESP. 2019. Regional and integrated emission standard of air pollutants. Jinan: The Department of Ecology and Environment of Shandong Province.
- Deutsch, W. 1922. Bewegung und ladung der elektrizitastragerim zylinderkondensator. Annal Phys. 373 (12):335–44. doi:https://doi.org/10.1002/andp.19223731203.
- Ding, Z. J., Z. Y. Yang, L. C. Xiao, Q. B. Zhan, and M. M. Zheng. 2015. Experimental research on performance of corona discharge and dust remove of wire-plate wet electrostatic precipitator. J. Yanshan Univ. 39 (2):177–81.
- Gao, J. J., H. Z. Tian, K. Cheng, L. Lu, M. Zheng, S. X. Wang, J. M. Hao, K. Wang, S. B. Hua, and C. Y. Zhu. 2015. The variation of chemical characteristics of PM2.5 and PM10 and formation causes during two haze pollution events in urban Beijing, China. Atmos. Environ. 107 (apr.):1–8. doi:https://doi.org/10.1016/j.atmosenv.2015.02.022.
- GB16157-1996. 1996. The determination of particulates and sampling methods of gaseous pollutants emitted from exhaust gas of stationary source. Beijing: Issued by Chinese State Environmental Protection Administration.
- Gu, J. W. 2019. A review on heavy metals in atmospheric suspended particles of China cities and its implication for future references. Earth Environ. 47 (3):385–96.
- He, J. Y., L. Yan, Y. Lei, and X. Y. Wang. 2015. Emission characteristics of particulate matter from coal-fired power plants in china. Res. Environ. Sci. 6 (28):862–68.
- HJ836-2017. 2018. Stationary source emission-determination of mass concentration of particulate matter at low concentration-manual gravimetric method. Beijing: Ministry of Ecology and Environment of the People’s Republic of China.
- Hu, B., L. Zhang, Y. Yi, F. Luo, and L. J. Yang. 2017. PM2.5 and SO3 collaborative removal in electrostatic precipitator. Powder Technology 318:484–490.
- Hu, M. Y., Y. Zhao, and Z. Liu. 2006. Dust technology. Beijing: Chemical Industry Press.
- jędrusik, M., and A. Swierczok. 2013. The correlation between corona current distribution and collection of fine particles in a laboratory-scale electrostatic precipitator. J. Electrost. 71 (3):199–203. doi:https://doi.org/10.1016/j.elstat.2013.01.002.
- Jiang, H. T., S. G. Tian, Y. L. Fu, M. H. Jia, and Y. B. Zhang. 2014. Application of wet electrostatic precipitator in coal-fired power plants. Power Equip. 28:61–64.
- Jin, X. 2013. Research on the capture technology of fine particles in electrostatic precipitator. Beijing: Tsinghua University.
- Li, J. G., Y. Z. He, H. X. Liu, and H. B. Zhao. 2017. Characteristics of Low-Low Temperature ESP Technology and Its Application in Ultra-Low Emissions for High-Ash Coal-Fueled Power Plant. Electric Power 50 (3):28–33.
- Li, L. J., Z. S. Wang, D. W. Zhang, T. Chen, and Y. T. Li. 2016. Analysis of heavy air pollution episodes in Beijing during 2013~2014. China Environ. Sci. 36 (1):27–35.
- Li, X. L., D. B. Zhou, J. X. Duan, W. J. Zhang, J. Z. Li, and J. B. Yan. 2018. Characteristics of particle emission from coal-fired power plants under the ultra low emission standard. Environ. Monitor. China 34 (3):45–50.
- Liu, R. L., C. F. You, R. C. Yang, and J. C. Wang. 2010. Direct numerical simulation of kinematics and thermophoretic deposition of inhalable particles in turbulent duct flows. Aerosol. Sci. Technol. 44 (12):1146–56. doi:https://doi.org/10.1080/02786826.2010.515270.
- Mazen, A. S. 1985. Positive wire-to-plane coronas as influenced by atmospheric humidity. IEEE Trans. Ind. Appl. IA-21 (1):35–40. doi:https://doi.org/10.1109/TIA.1985.349640.
- MEP. 2011. Emission standards for air pollutants in thermal power plants. https://wenku.baidu.com/view/451b248c680203d8cf2f2404.html.
- Mertens, J. C., A. P. Rogiers, L. Brachert, P. Khakharia, E. Goetheer, and K. Schaber. 2014. A wet electrostatic precipitator (WESP) as countermeasure to mist formation in amine based carbon capture. Int. J. Greenhouse Gas Control 31:175–81. doi:https://doi.org/10.1016/j.ijggc.2014.10.012.
- Miller, A. B., D. L. Crouse, R. V. Martin, C. Wall, A. Tomczak, S. A. Weichenthal, T. To, A. Van Donkelaar, and P. J. Villeneuve. 2016. Long-term exposure to fine particulate matter air pollution and the risk of lung cancer among participants of the Canadian National Breast Screening Study. Int. J. Cancer 139 (9):1958–66. doi:https://doi.org/10.1002/ijc.30255.
- Musumeci, F., and G. H. Pollack. 2012. Influence of water on the work function of certain metals. Chem. Phys. Lett. 536 (none):65–67. doi:https://doi.org/10.1016/j.cplett.2012.03.094.
- NDRC. 2014. Action plan on upgrading and upgrading of coal power energy conservation and emission reduction (2014-2020).
- Noll, C. G. 2002. Temperature dependence of dc corona and charge-carrier entrainment in a gas flow channel - ScienceDirect. J. Electrost. 54 (3):245–70. doi:https://doi.org/10.1016/S0304-3886(01)00169-3.
- Pan, X. H. 2016. Study on multi field synergy effect of fine particles in electrostatic precipitator. Jinan: Shandong University.
- Pan, X. H., L. Cui, and C. Y. Ma. 2016. Influence of water film on the distribution and motion characteristics of fine particles in electrostatic precipitator. Proc. Csee 36 (16):4333–41.
- PGZP. 2018. Emission standard of air pollutants for coal-fired power plants. Hangzhou: The People’s Government of Zhejiang Province.
- Rashdi, S. A., A. A. Arabi, F. M. Howari, and A. Siada. 2015. Distribution of heavy metals in the coastal area of Abu Dhabi in the United Arab Emirates. Mar. Pollut. Bull. 97 (1/2):494–98. doi:https://doi.org/10.1016/j.marpolbul.2015.05.052.
- Shi, W. Z., M. M. Yang, X. H. Zhang, S. Q. Li, and Q. Yao. 2016. Ultra-low emission technical route of coal-fired power plants and the cooperative removal. Proceedings of the Csee 36 (16):4308–4318.
- Shou, C. H., Z. F. Qi, W. Y. Xie, Z. W. Zou, C. H. Liu, M. Li, J. W. Li, X. D. Li, and W. H. Li. 2016. Experimental Study on Engineering Application of Particulate Matter Removal Characteristics of Low-low Temperature Electrostatic Precipitator. Proceedings of the CSEE 36 (16):4326–4332.
- Sui, Z. F., Y. S. Zhang, Y. Peng, P. Norris, Y. Cao, and W. P. Pan. 2016. Fine particulate matter emission and size distribution characteristics in an ultra-low emission power plant. Fuel 185 (dec.1):863–71. doi:https://doi.org/10.1016/j.fuel.2016.08.051.
- Sun, L. M., Z. Q. Lin, K. Liao, Z. G. Xi, and D. Y. Wang. 2015. Adverse effects of coal combustion related fine particulate matter (PM2.5) on nematode Caenorhabditis elegans. Sci. Total Environ. 512-513:251. doi:https://doi.org/10.1016/j.scitotenv.2015.01.058.
- Tang, G. Q., J. Q. Zhang, X. W. Zhu, T. Song, C. Münkel, B. Hu, K. Schäfer, Z. R. Liu, J. K. Zhang, L. L. Wang, et al. 2016. Mixing layer height and its implications for air pollution over Beijing, China. Atmos. Chem. Phys. 16 (4):2459–75. doi:https://doi.org/10.5194/acp-16-2459-2016.
- Tang, G. Q., P. S. Zhao, Y. H. Wang, W. K. Gao, M. T. Cheng, J. Y. Xin, X. Li, and Y. S. Wang. 2017. Mortality and air pollution in Beijing: The long-term relationship. Atmos. Environ. 150 (FEB.):238–43. doi:https://doi.org/10.1016/j.atmosenv.2016.11.045.
- Tao, Y., Y. M. Liu, S. Q. Mi, and Y. T. Guo. 2014. Atmospheric pollution characteristics of fine particles and their effects on human health. Acta Scientiae Circumstan. 34 (3):592–97.
- Tian, P., G. F. Wang, R. J. Zhang, Y. F. Wu, and Y. Peng. 2015. Impacts of aerosol chemical compositions on optical properties in urban Beijing, China. Particuology 18 (1):155–64. doi:https://doi.org/10.1016/j.partic.2014.03.014.
- Wan, Y. 2014. Uniformity of water film and enhancement of fine particles removal in wet electrostatic precipitator. Hangzhou: Zhejiang University.
- Wang, Q. L., L. Zhang, Z. Li, J. B. Fan, Y. Yin, C. Gu, and Q. G. Zhang. 2009. Influence of humidity on conductor corona onset voltage. Electric Power Constr. 30 (8):38–41.
- Wang, X. 2018. Study on mechanism of heat and moisture transfer on electrical field and fine particles. Jinan: Shandong University.
- Wang, X., J. C. Chang, C. Y. Xu, J. Zhang, P. Wang, and C. Y. Ma. 2016. Collection and charging characteristics of particles in an electrostatic precipitator with a wet membrane collecting electrode. J. Electrost. 83:28–34. doi:https://doi.org/10.1016/j.elstat.2016.07.007.
- Wang, X. H. 2013. Enhancement mechanism and process of water on particle removal in electrostatic filed. Beijing: Tsinghua University.
- Wang, X. H., C. F. You, R. L. Liu, and R. C. Yang. 2011. Particle deposition on the wall driven by turbulence, thermophoresis and particle agglomeration in channel flow. Proc. Combus. Inst. 33 (2):2821–28. doi:https://doi.org/10.1016/j.proci.2010.08.009.
- White, H. J. 1963. Industrial electrostatic precipitation. Boston: Addison-Wesley Pub. Co.
- Xie, Z., Y. Qin, Z. Zheng, and L. I. Yang. 2019. Assessing the death effect of PM_(2.5) pollution in cities of atmospheric pollution transmission channel in the Beijing-Tianjin-Hebei district. Acta Scientiae Circumstantiae 39 (3):843–52.
- Xiong, G. L., S. Q. Li, S. Chen, X. H. Zhang, and Q. Yao. 2015. Development of advanced electrostatic precipitation technologies for reducing PM2.5 emissions from coal-fired power plants. Proc. CSEE 35 (9):2217–23.
- Xiong, G. L., S. Q. Li, X. H. Zhang, S. Chen, M. M. Yang, and Q. Yao. 2014. Removal research of PM2.5 from coal-fired power plant with wet electrostatic precipitators. Xian: CSETCS.
- Xu, C. Y., J. C. Chang, Z. Meng, X. Wang, L. Cui, and C. Y. Ma. 2015. Wetting characteristics experiment of modified carbon steel surface with single strand flow. CIESC J. 66 (2):669–77.
- Xu, F., Z. Y. Luo, B. Wei, L. N. Wang, X. Gao, M. X. Fang, and K. F. Cen. 2009. Electrostatic Capture of PM2.5 Emitted from Coal-fired Power Plant by Pulsed Corona Discharge Combined with DC Agglomeration. 11th international conference on electrostatic precipitation, Hangzhou.
- Xu, Y. S., X. W. Liu, J. Cui, D. Chen, M. H. Xu, S. W. Pan, K. Zhang, and X. P. Gao. 2016. Field measurements on the emission and removal of PM2.5 from coal-fired power stations: 4. PM removal performance of wet electrostatic precipitators. Energy Fuel 30 (9):7465–73. doi:https://doi.org/10.1021/acs.energyfuels.6b00426.
- Yan, H., J. B. Zhu, X. Y. Li, and K. P. Yan. 2007. Experimental study on I-V characteristics of ESP. Proceedings of 12th Conference of ESP, Shijiazhuang, 10.19.
- Yang, X. W., Y. Zhou, S. Y. Cheng, G. Wang, and X. Q. Wang. 2016. Characteristics and formation mechanism of a heavy winter air pollution event in Beijing. China Environ. Sci. 36 (3):679–86.
- Yang, Z. D., C. H. Zheng, S. J. Liu, Y. S. Guo, C. S. Liang, W. Yi, D. Q. Hu, and G. Xiang. 2018. A combined wet electrostatic precipitator for efficiently eliminating fine particle penetration. Fuel Proc. Technol. 180:122–29. doi:https://doi.org/10.1016/j.fuproc.2018.08.013.
- Yin, Z. C. 2019. Experimental research on migration and transformation of mercury in wet electrostatic precipitator system. Wuhan: Huazhong University of Science & Technology.
- You, C. F., X. H. Wang, R. L. Liu, and R. C. Yang. 2010. Simultaneous effects of electrostatic field and thermophoresis on inhalable particulate matter removal. Powder Technol. 202 (1–3):95–100. doi:https://doi.org/10.1016/j.powtec.2010.04.022.
- Yu, W. J. 2017. Research of application and development for dust ultra-low emission technologies in coal-fired plants. Chem. Ind. Eng. Prog. 36 (b11):428–35.
- Yuan, X. L., M. F. Zhang, Q. S. Wang, Y. T. Wang, and J. Zuo. 2017. Evolution analysis of environmental standards: Effectiveness on air pollutant emissions reduction. J. Clean. Prod. 15 (149):511–20. doi:https://doi.org/10.1016/j.jclepro.2017.02.127.
- Zhang, D. Y., C. Wang, X. H. Zhu, and B. X. Qi. 2015. Precipitator manual. 2nd ed. Beijing: Chemical Industry Press.
- Zhang, W. F., and C. L. Liao. 2015. Research Review on Agglomeration Technologies of Submicron Particles PM2.5 in China. Journal of east china jiaotong university 32 (004):124–130.
- Zhao, H. J., H. Z. Che, X. Y. Zhang, Y. J. Ma, Y. F. Wang, H. Wang, and Y. Q. Wang. 2013. Characteristics of visibility and particulate matter (PM) in an urban area of Northeast China. Atmos. Pollut. Res. 4 (4):427–34. doi:https://doi.org/10.5094/APR.2013.049.
- Zhao, L., and H. G. Zhou. 2016. Particle removal efficiency analysis of WESP in an ultra low emission coal-fired power plant. Proc. CSEE 36 (2):468–73.
- Zhao, S., Y. Duan, T. Yao, M. Liu, J. Lu, H. Tan, X. Wang, and L. Wu. 2017. Study on the mercury emission and transformation in an ultra-low emission coal-fired power plant. Fuel 199 (JUL.1):653–61. doi:https://doi.org/10.1016/j.fuel.2017.03.038.
- Zhao, S. Z., H. H. Yi, X. L. Tang, F. Y. Gao, and Y. R. Zuo. 2014. Source and harm of air fine particulate matter pollution and control strategies. Sci. Technol. Rev. 32 (33):61–66.
- Zheng, C. H., Y. F. Wang, X. F. Zhang, Z. D. Yang, and X. Gao. 2018. Current density distribution and optimization of the collection electrodes of a honeycomb wet electrostatic precipitator. RSC Advances 8 (54):30701–30711.
- Zhou, D. L., S. Q. Li, X. Jin, G. L. Xiong, and W. Huang. 2016. Experiments and numerical simulations of the removal of fine particles in the coupling field of electrostatic precipitators. Proc. CSEE 36 (2):453–58.