ABSTRACT
The purpose of this study was to determine the most efficient airborne asbestos filter for use in an HEPA negative air machines through filter performance testing. The filter classes applied conformed with ISO and European standard (EN 1822) regarding fractional efficiency by dust loading amount for filters, fractional efficiency for negative air machines, and consumption of electrical power at filter size 0.3 μm. Class H13 had the highest fractional efficiency among the three experimental filter classes by particle size, at face velocity of (1, 2, and 3) m/s. Melt-blown (MB) filters exhibited higher fractional performance than did glass-fiber filters at all particle sizes tested (0.3, 0.5, and 1.0 µm). The power consumption of glass-fiber filters was higher (at 10 m3/min) than that of melt-blown filters. Therefore, melt blown filters would be more cost-effective than glass fiber filters for use in HEPA negative air machines, for protection against airborne asbestos.
Implications: Air cleaner and related systems were developed to control a variety of airborne pollutants in general indoor environments, but there was no certified system for focusing on asbestos fractional efficiency using filter tests. Class H13 had the highest fractional efficiency among the three experimental filter classes by particle size, at face velocity of (1, 2, and 3) m/s. Melt-blown filters exhibited higher fractional performance than did glass-fiber filters at all particle sizes tested (0.3, 0.5, and 1.0 µm). The power consumption of glass-fiber filters was higher (at 10 m3/min) than that of melt-blown filters.
Introduction
Asbestos has been used since ancient times owing to its affordability and desirable physical properties, such as thermal resistance and its ability to insulate against heat. Asbestos fiber is defined for counting purposes as a particle that is >5 μm in length and a length with width ratio of at least 3:1. For the most other crafts, average fiber concentrations were found to typically range from <0.01 to 1 fibers/cc with higher concentrations observed during the use of powered tools, the mixing or sanding of drywall cement, and the cleanup of asbestos insulation or lagging materials (Williams, Phelka, and Paustenbach Citation2007). Recently, the WHO reported that there are about 125 million people in the world exposed to asbestos at the workplace, and that at least 107,000 people die each year from asbestos-related lung cancer, mesothelioma, and asbestosis due to occupational exposure (WHO Citation2014). The International Agency for Research on Cancer concluded that all forms of asbestos, including chrysotile, are causally associated with an increased risk of cancer of the lungs, larynx, and ovary, as well as mesothelioma and asbestosis (IARC Citation2012). Thus, it is important to manage asbestos properly in working conditions in both indoor and outdoor environments.
Asbestos removal work would be very dangerous work if there was not appropriate equipment such as personal protective equipment (mask, goggle, gloves, etc.), and high efficiency particulate air filters (HEPA) for negative pressure systems and vacuum cleaning. HEPA and ultra-low penetrating particulate air (ULPA) filters are widely used, and are among the most important tools for establishing a clean space. In order to guarantee product quality and environmental control, every country makes its own strict test standards (Zhou and Shen Citation2007). Agencies of the United States apply various HEPA filter standards (MIL-STD-282) with average particle size of 0.3 μm (USDOD Citation1956). In 2005, the Institute of Environment Sciences and Technology (IEST) issued IEST-RP-CC001.4 “HEPA and ULPA Filters,” and IEST-RPCC034.2 “HEPA and ULPA Filter Leak Tests” (Zhou and Shen Citation2007). Agencies of the UK are embracing national standards that conform to the standard HEPA filter (EN 1822-1:2009) of the European Union (UKHSC Citation2006). This EU standard for the HEPA filter is a unified one, which has replaced a disorderly situation with a unified European standard. The biggest new feature is that the filter efficiency against the most penetrating particle size (MPPS) is measured considering the filter medium and type of filter (Zhou and Shen Citation2007). The Korean Industrial Standards (KS) for domestic HEPA filter are KS A4812 and KS B 6740. The main difference between these two standards is that the KS A4812 standard is efficiency evaluated using DOP (dioctyl phthalate) particles of 0.15 μm (KATS Citation2001), while the KS B6740 standard is efficiency evaluated using DOP particles of 0.3 μm (KATS Citation1995). However, there are no certified specifications or test standards for HEPA filters in South Korea. In many countries around the world, air cleaner and related systems were developed to control a variety of airborne pollutants in general indoor environments, but there was no certified system for focusing on asbestos fractional efficiency using filter tests.
Glass fibers are used in HEPA filters because they have physical properties similar to those of asbestos fibers. Fine fiber diameters provide increased surface area and produce filters with improved efficiency, resulting in an ideal filtration fiber (Rathey Citation2006). Melt-blown (MB) nonwovens are known for their high surface area per unit weight, high insulation value, and high barrier properties. These properties make them excellent candidates for use in the construction of high-quality filters (Albrecht, Fuchs, and Kittelmann Citation2003). Advancements in melt-spinning and MB technology have helped broaden the list of raw materials usable for nonwoven filters because fibers in the sub-micron and even nano-scale range can now be produced (Hutten Citation2007). This study was carried out to find the filter with the highest efficiency for use in HEPA negative air machines. This was done using filter performance tests of properties such as filtration performance, pressure by filter classes, and dust loading, using both glass-fiber and MB filters.
Therefore, the purpose of this study was to carry out a filter collection efficiency test for MB and glass-fiber filters considering such as filtration performance, pressure by filter classes, and dust loading, in relation to particle size. This comparison was extended by comparing filters of commercial glass fiber, which are currently used in negative air machines to remove airborne asbestos from closed controlled areas and melt blown filters, which have recently been applied for use in negative air machines, to find out which filter has better efficiency when protecting against airborne asbestos.
Methods
Particle filtration
To evaluate the fractional efficiency, in relation to dust loading amount for filter media by particle size, we used TOPAS, PAF 111 (Topas GmbH, Germany) in the air-filter test system. This device included a high-efficiency particulate air (HEPA) filter, aerosol generator, dust dispenser, throttle valve to control the airflow, blower, flow rate sensor, differential pressure sensor to measure the pressure (before and after the subject filter), and an optical particle counter to get particle counts before and after the test filter. Pleated module is placed inside the filter chamber and fixed. To obtain a representative sample independent of particle size, isokinetic air sampling was done with the same velocity as the main stream. Test conditions and components are as indicated in and .
Table 1. Parameters and values of automated filter tester and particle filtration
Measurement of fractional efficiency for negative air machines
To evaluate the performance tests of fractional efficiency for the negative air machines, we used an airborne particle counter, KC-01C (Rion Co., LTD, Japan), and a light scattering method to measure the particle concentration. In sample volumes of 1 L, particles were counted in particle sizes of (0.3, 0.5, and 1) µm at a flow of 0.5 L min−1. We measured particle concentrations at the inlet and outlet of the negative air machines after the airflow rate was stable. The MB and glass-fiber filters were measured 10 times to test the performance of E 11 (Efficiency Particulate Air filter), E12, and H13 under the same experimental conditions.
Filter media
Polypropylene was used for the MB non-woven fabric, and it was used for spreading the MB filter media with 2, 5-dimethyl-2, 5-di-(tertbutylperoxy)-hexane. Isotactic polypropylene with adjusted melt flow rate of 1500 g 10 min−1 was used for the H11 (CS-H11), H12 (CS-H12), and E13 (CS-E13) filter media (CNTUS-SUNGJIN Co., Ltd. Seoul, Korea). Glass fiber was applied to the testing filter media to compare with the MB filter media for H11 (model HD2063), H12 (model HC4393), and E13 (model HB5793) (Hollingsworth & Vose. Co., Ltd. Suzhou, China). MB and glass-fiber filters were used for the negative air machines. The size of the pleated filters was (277 × 361 × 25) mm (width, length, and thickness) and each consisted of 81 pleats at both filters (). Particle sizes were determined (from 0.1 to 10 μm) using TOPAS equipment with face velocity of 1, 2, and 3 m/s. The actual filtration area is 1.12 m2, and the actual filtration velocity is 0.89 m s−1∙m2.
In this experiment, a filter of glass fiber for use in the HEPA negative air machines was compared with a filter of MB for this study. These were used to sample particle size filtration effectiveness, using an airborne particle counter KC-01C.
The International Organization for Standardization (ISO) standard was developed using the EN 1822-1 standard as its basis, with revisions to accommodate the practices of other national standards of the U.S., Japan, and others (Vijayakumar Citation2015). This study followed the ISO and European standard (EN 1822-1): E11 (≥95% efficiency), E12 (≥9 99.5% efficiency), and H13 (≥9 99.95% efficiency)
Measurement of consumption of electrical power
Consumption of electrical power was measured (when the airflow rate of the negative air machines was stable) using a power meter (Wattman HPM-100A, AD Power Co., Ltd, Korea). The electrical power supply was 60 Hz with rated frequency, and the rated voltage was 220 V with single-phase alternating current. Measurement was carried out more than 10 times with one-minute intervals.
Asbestos sampling and analysis
Air sampling was performed on-site during asbestos removal work at different asbestos removing sites with MB filter media. Samples were evaluated using high-volume pump (Model 1532; SKC, USA) with an open-face attached mixed-cellulose ester filter (25 mm diameter, pore size 0.8 µm) at a flow rate of 10 L min−1 during asbestos removal within 30 cm radius of the area of removal. Phase contrast microscopy (PCM) and Transmission electron microscopy (TEM) were applied to identify asbestos using the National Institute of Occupational Safety and Health Method 7400 for PCM and 7402 for TEM (NIOSH, Citation1994a, Citation1994b).
Statistical analysis
The test results for the two types of filters (MB and glass fiber) were analyzed for fractional efficiency by particle size, using H13 class, and applying analysis of variance (ANOVA). The data for the two types of filters were analyzed by applying a t-test, in conjunction with power consumption and flow rates, for the filters at class H13. All data were analyzed using SAS software version 9.3 (SAS Institute, Cary, NC, USA). All differences were considered significant if p < .05.
Results
EPA (Efficiency Particulate Air filter) and HEPA filter fraction efficiency at three classes (E11, E12, H13), with face velocity 1 m/s, and particle size from (0.1 to 10) µm, are shown in . In all types of filters, and at all classes, 100% of the coarse particles (4–10 µm) were collected. Although class E11 (for particle seize <1 µm) exhibited the lowest efficiency, class H13 had the highest efficiency (almost 100%).
Figure 3. Fractional efficiency of melt-blown filters by particle size with 1 m/s face velocity through class E11, E12, and H13 filters.
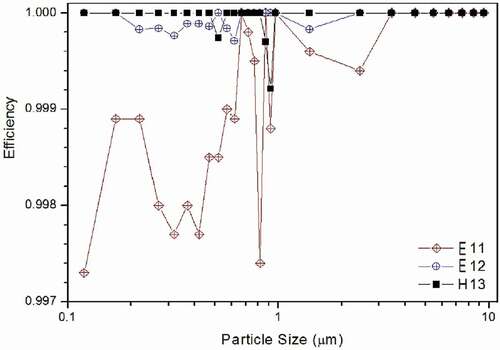
The HEPA filter efficiency of the three classes (E11, E12, H13) with face velocity 2 m/s, and particle size from (0.1 to 10) µm, is shown in . All types and classes of filters collected 10% of the coarse particles (>5 µm). Class E12 and H13 had relatively higher efficiency than E11, while all types and classes of filters showed decreased efficiency when the particle size was <1 µm.
Figure 4. Fractional efficiency of melt-blown filters by particle size with 2 m/s face velocity through class E11, E12, and H13 filter.
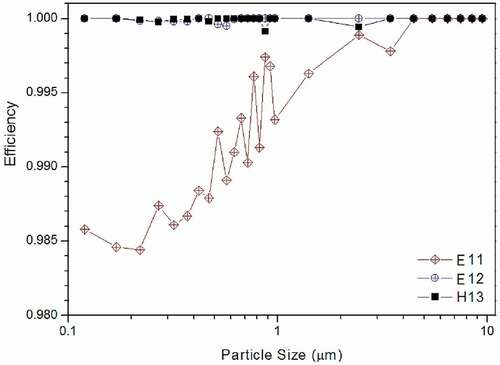
The filter fractional efficiency of all three classes (E11, E12, H13) with face velocity 3 m/s, and particle size from (0.1 to 10) µm, is shown in . All types and classes of filters collected 100% of the coarse particles (>5 µm), but showed less efficiency at face velocities of (1 and 2) m/s.
Figure 5. Fractional efficiency of melt-blown filter by particle size with 3 m/s face velocity through class E11, E12, and H13 filters.
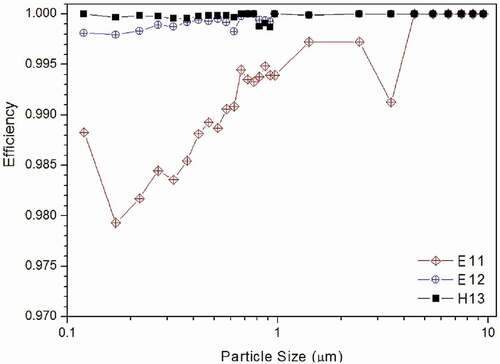
Comparison of efficiency between melt-blown and glass-fiber filters in a negative air machines, in relation to particle size (0.3, 05, and 1.0 µm), is presented in . The MB filters showed better filtration performance than did the glass-fiber filters for sizes of (0.3 and 0.5) µm, but they all showed the same efficiency when the particle size was 1 µm.
shows variation of the pressure drop in a HEPA filter device due to the dust-loading amount, at a flow of 1 m/s for the three classes (a), and for an HEPA filter with H13 by face velocity (b). The classes of HEPA devices with melt-blown filters were regularly increased by increasing the amount of particle fractional (). In case of the HEPA device considered by face velocity, the pressure drop from dust loading was lowest at class E11, and the highest pressure drop was at class H13 (). shows the morphological structure of both MB and glass-fiber filter media (H13) before and after particle collection.
Figure 6. Variation of pressure in HEPA filter by dust loading amount at 1 m/s by classes (a), and H13 class by face velocity (b).
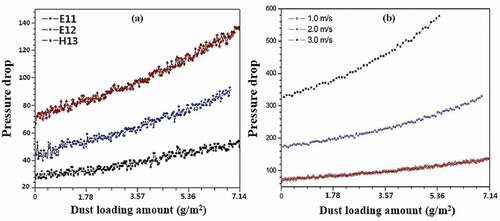
Figure 7. Morphological structures of melt-blown and glass-fiber media (H13) taken before and after dust loading using an electronic microscope.
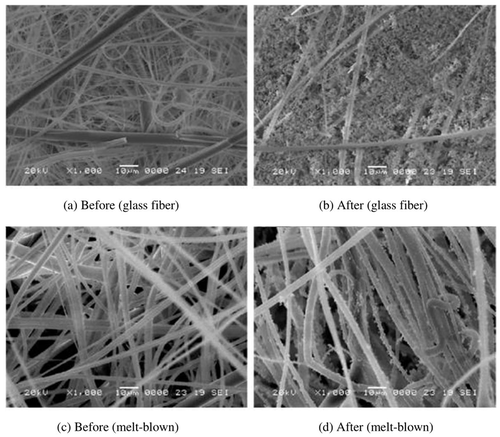
shows a comparison of power consumption between MB and glass-fiber filters, in relation to increased flow rate. For both types of filter, power consumption increased with increasing flow rates. There was no difference in power consumption between MB and glass-fiber filters up to 5 m3/min, while power consumption of the glass-fiber filter was highest for >10 m3/min.
Figure 8. Comparison of power consumption between melt-blown and glass-fiber media by increasing flow rate through class H13 filters (p < .05).
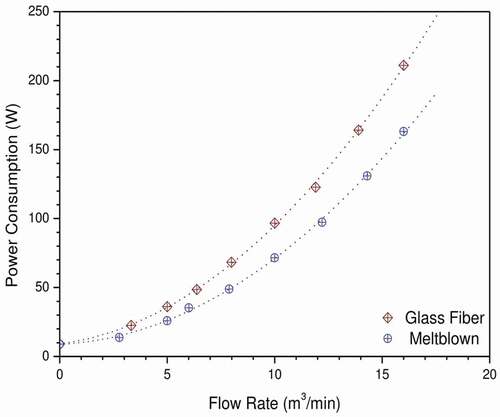
Although the airborne asbestos concentrations in a school classroom and office sites using PCM did not exceed the asbestos guideline (0.01 fiber cm−3), higher concentrations based on PCM were detected before turning on the negative air machine, with 0.004 fiber cm−3 in the classroom and 0.003 fiber cm−3 in the office compared to after asbestos removal, the classroom and office concentrations were 0.003 fiber cm−3 and 0.001 fiber cm−3, respectively.
Discussion
We confirmed that class H13 and particle size <1 µm, resulted in the highest efficiency, while class E11 exhibited the lowest efficiency among the three filter classes. This result supports the notion that class H13 is a higher performance filter than E11 and E12 because the H13 standard must collect more than 99.97% of particles of size 0.3 µm. The EPA and HEPA specifications usually used in this study were the same as the ISO and European Norm EN 1822-1:2009. This standard defines several classes of EPA and HEPA filters by their efficiency: E11 (≥95% of particle size 0.3 µm), E12 (≥99.5% of particle size 0.3 µm), and H13 (≥99.97% of particle size 0.3 µm) (UKHSC Citation2006). These results mean that the EPA and HEPA filters of these three classes provided acceptable performance at the face velocity of 1 m/s because each filter class had higher efficiency than the standard for E11 (≥95%), E12 (≥99.5%), and H13 (≥99.95%) with particle size 0.3 µm (). The trends in the performance of melt-blown filters were the same with face velocity of 2 and 3 m/s (). The fractional efficiency and pressure drop of the filters were strongly dependent on filter type, particle size, and airflow velocity (Zikova, Ondracek, and Zdimal Citation2015). In our study, we confirmed that efficiency differed by filter type. MB filters had higher efficiency, especially for particle 0.3 µm than did the glass fiber filters, which are commonly used in asbestos workplaces in South Korea as the asbestos filters in negative air machines. However, the fractional efficiency of the MB filters did not satisfy the ISO and EU standard for H13 and particle size 0.3 µm (≥99.95%) ().
Table 2. Characteristics of filter media by class
Table 3. Comparison of efficiency by particle size, between melt-blown and glass-fiber filters in a negative air machine
The pressure drop of the MB filters was high when the face velocity was steadily increased from (1 to 3) m/s, and filter classes were changed from E11 to H13 (). The reason why there was a high-pressure drop at the filter when the face velocity was increased, was because the increased airflow exceeded the amount able to pass quickly through the dense nonwoven structure of the MB filter causing increased pressure at the incoming face of the filter. This pressure drop was also found to be strongly dependent on four different pore sizes across the other filter types, and the pressure drop increased with increased flow rate for all pore sizes (Soo et al. Citation2016; Zikova, Ondracek, and Zdimal Citation2015). To be able to use negative air machines to filter large indoor areas while removing asbestos, it is necessary that the newly developed filters have a much greater volume of air passage, and consequently, a smaller pressure drop. Thus, it is essential to maintain a lower pressure drop and higher fractional efficiency than is possible with glass fiber filters. During the life cycle of a filter, the operating cost is considerably affected by the pressure drop. Lower pressure drop means less energy consumption by air filtration systems (Fisk et al. Citation2003; Montgomery, Green, and Rogak Citation2012), which in turn leads to lower operating cost and longer service life. An ideal filter should have a low pressure-drop and high capture efficiency (Bharadwaj, Patel, and Bharadwaj Citation2008). Other benefits of low pressure-drop systems are less noise, and sometimes lower total first cost (PG&E, Citation2011).
It was confirmed that the power consumption of melt-blown and glass-fiber filters, in relation to increasing flow rate was very different: about 48 W under the same conditions (). Power consumption increased with decreased area of the filter; this is because of the increase in the pressure drop. The difference in power consumption between MB and glass-fiber filters of about 48 W converts to about USD 10 in South Korea. This means that using MB filters instead of glass-fiber filters might save as much as USD 10 of the cost for electricity. If using MB filters for a long time, with gradual increase in the flow rate to more than 16 m3/min, it could save more than USD 10 for electricity. Another reason why power consumption increases with the flow rate, is that it requires substantial increase in the revolutions per minute (r min−1) of the motor and fan blades, to make a higher airflow rate. At the point of asbestos removal, it is critical to collect airborne asbestos particles with high efficiency, while the power consumption when doing so is an important factor in the saving of energy and cost. If asbestos removal occurred over a long time, the difference in power consumption between these two filter materials would be bigger, and the difference in the cost of electricity would also be large. This important condition favors using MB filters for negative air machines. When using HEPA filters of glass fiber in negative air machines, the motor speed would increase to overcome the pressure increase at the filter, which causes high power consumption, a higher noise level of motor and fans, as well shorter life spans for all the mechanical moving parts.
MB fiber is easy to fabricate and provides financial benefits, both of which are desirable for filter media production. Because modified MB lines are capable of producing submicron and nano-sized fibers, they showed great potential for filter media. The MB filter media showed notable fractional efficiency and lower operating pressure (pressure drop across the filter) (Skomra Citation2010). Glass fibers historically have also played a major role in HEPA filtration because they tend to be inexpensive and have small cross-sectional diameters. However, they are short and thus unable to be easily processed using standard nonwoven carding equipment (Dugan and Homonoff Citation2006).
Asbestos was detected at both sites after operating the negative air machines based on the PCM analysis (). This was likely due to limitations in the PCM analysis method, because any fibers that satisfied a ratio of 1:3, including asbestos, were considered as asbestos fibers (NIOSH Citation1994a). After the TEM analysis, the fibers initially determined to be asbestos in the PCM analysis were determined not to be asbestos. These results show that the application of MB filter media in these negative air machines can be effectively applied to real asbestos removal sites.).
Table 4. Application of melt-blown filter media in negative air machines to asbestos removal sites
Although there is no other data comparison of pressure drops for MB and glass-fiber filters, one previous study reported that the pressure drop across an MB filter was comparable to that of a commercial glass-fiber one (Hassen et al. Citation2013). Also, the pressure drop was similar with this study.
In the present study, highly efficient performance of MB filters was proven by their lower power consumption. This makes them a cost-effective alternative to glass-fiber filters for use in negative air machines.
Conclusion
This study was conducted to test and compare the filter efficiency of melt-blown and glass fiber in HEPA negative air machines. There are several limitations that should be considered. First, we could not evaluate the filtration velocity (flow rate divided by the filtering area) between the two types of filters. Thus, additional studies are needed to assess other factors, such as particle roughness, cohesiveness, and physical detail. Second, the absence of important features of asbestos fibers such as density, morphology, and dimensions is not making the study complete for the real field of asbestos removal, but we have confirmed that the application of MB filter media in negative air machine can be effectively applied (). Third, A2 fine aerosols were used to confirm the efficiency in both filter media because applying asbestos is banned since 2009. Finally, the measurement results in this work are valid for the conditions of the experiments, such as temperature, airflow, and dust concentration. Nevertheless, the findings that MB filters offer higher fractional efficiency and lower power consumption mean that this material is a cost-effective alternative to glass fiber for use in HEPA negative air machines during asbestos removal.
Disclosure statement
No potential conflict of interest was reported by the author(s).
Additional information
Funding
Notes on contributors
Sung Ho Hwang
Sung Ho Hwang is a Senior Scientist and Assistant Professor at National Cancer Control Institute, National Cancer Center, Goyang-si, South Korea.
Byong Hyoek Lee
Byong Hyoek Lee is a researcher at the Environmental Technology Institute, Coway Co., Ltd.
References
- Albrecht, W., H. Fuchs, and W. Kittelmann. 2003. Nonwoven fabrics: Raw materials, manufacture, applications, characteristics, testing processes. Hoboken: Wiley.
- Bharadwaj, R., A. Patel, and R. Bharadwaj. 2008. Oriented fiber filter media. JEFF Spec. Issue 2008:29–34.
- Dugan, J., and E. Homonoff. 2006. Synthetic split microfiber technology for filtration, INDA filtration, 28–30. Accessed November 20, 2006. http://www.fitfibers.com/files/Microfibers%20for%20Filtration.doc .
- Fisk, W. J., D. Faulkner, J. Palonen, and O. Seppanen. 2003. Performance and costs of particle air filtration technologies. Indoor Air 12:223–34. doi:https://doi.org/10.1034/j.1600-0668.2002.01136.x.
- Hassen, M. A., B. Y. Yeom, A. Wilkie, B. Pourdeyhimi, and S. A. Khan. 2013. Fabrication of nanofiber meltblown membranes and their filtration properties. J. Membr. Sci. 427:336–44. doi:https://doi.org/10.1016/j.memsci.2012.09.050.
- Hutten, I. M. 2007. In handbook of nonwoven filter media. Oxford: Elsevier Ltd.
- International Agency for Research on Cancer (IARC). 2012. IARC monographs volume 100C: Arsenic, metals, fibres and dusts; a review of human carcinogens [ Internet]. [Accessed June 3, 2016]. http://monographs.iarc.fr/ENG/Monographs/vol100C/mono100C.pdf .
- Korean Agency for Technology and Standards (KATS). 1995. Air filter performance test method for clean room. KS B 6740. Chungcheongbuk-do, Korea: Korean Agency for Technology and Standards (KATS).
- Korean Agency for Technology and Standards (KATS). 2001. High-performance air filter for radioactive aerosol. KS A 4812. Chungcheongbuk-do, Korea: Korean Agency for Technology and Standards (KATS).
- Montgomery, J. F., S. Green, and S. N. Rogak 2012. Predicting the energy use and operation cost of HVAC air filters under variable-air-volume operation. 11th world filtration congress, Graz, Austria, April 16–20. Paper No. G09-01-322.
- NIOSH Manual of Analytical Methods (NMAM). 1994a. Asbestos and other fibers by PCM 7400. 4th ed. Government Printing Office Washington, D.C.: National Institute for Occupational Safety & Health.
- NIOSH Manual of Analytical Methods (NMAM). 1994b. Asbestos and by TEM 7400. 4th ed. Government Printing Office Washington, D.C.: National Institute for Occupational Safety & Health.
- Pacific Gas and Electric Company (PG&E). 2011. High performance clean rooms A design guidelines sourcebook. CTM-0311-0875, January. The California, USA: The California Public Utilities Commission.
- Rathey, A. 2006. HEPA help. Am. Sch. Univ. 78 (10):47–49.
- Skomra, E. 2010. Production and characterization of novel air filtration media. All Dissertations. Paper 683.
- Soo, J. C., K. Monaghan, T. Lee, M. Kashon, and M. Harper. 2016. Air sampling filtration media: Fractional efficiency for respirable size-selective sampling. Aerosol Sci. Technol. 50 (1):76–87. doi:https://doi.org/10.1080/02786826.2015.1128525.
- UK Health and Safety Commission (UKHSC). 2006. The management of asbestos in non-domestic premises: Regulation 4 of the control of asbestos regulations 2006. Suffolk: HSE Books.
- US Department of Defense (USDOD). 1956. Filter unit, protective clothing, gas-mask components and related products: Performance test methods. MIL-STD-282, Department of Defense Test Method Standard.
- Vijayakumar, R. 2015. ISO 29463: A global standard for HEPA and ULPA filters. Int. Filtr. News Aerfil. Accessed January 13, 2017. http://www.filtnews.com/featured-articles/iso-29463-a-global-standard-for-hepa-and-ulpa-filters/ .
- Williams, P. R. D., A. D. Phelka, and D. J. Paustenbach. 2007. A review of historical exposures to asbestos among skilled craftsmen (1940–2006). J. Toxicol. Environ. Health B Crit. Rev. 10 (5):319–77. doi:https://doi.org/10.1080/10937400601034191.
- World Health Organization (WHO). 2014. Chrysotile asbestos [ Internet]. [Accessed January 11, 2017]. http://www.who.int/ipcs/assessment/public_health/chrysotile_asbestos_summary.pdf .
- Zhou, B., and J. Shen. 2007. Comparison of HEPA/ULPA filter test standards between America and Europe. Proceedings of Clima 2007, Helsinki, Finland: Well being indoors.
- Zikova, N., J. Ondracek, and V. Zdimal. 2015. Size-resolved penetration through high-efficiency filter media typically used for aerosol sampling. Aerosol Sci. Technol. 49:239–49. doi:https://doi.org/10.1080/02786826.2015.1020997.