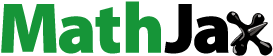
ABSTRACT
Water vapor has been one of the vital problems in purification of volatile organic compounds. In this study, the adsorption-desorption equilibrium of water vapor were conducted at 298, 308, 318, and 328 K on three adsorbents: hypercrosslinked polymeric adsorbents (HPA), activated carbon fiber (ACF) and granular activated carbon (GAC). The obtained isotherms were type V and the adsorption capacity at the same condition was: GAC>ACF>HPA. cluster formation induced micropore filling (CIMF) model was adopted to fit the adsorption isotherms and the fitting parameters showed that adsorption capacities of water vapor on micropores and functional groups had a negative logarithmic linear relationship with temperature. The existence of functional groups could weaken the negative influence of temperature on the water adsorption performance, while the influence of temperature had negligible relationship with microporous volume. The hysteresis loops at different temperatures on three adsorbents had similar shape, the size of which were also: GAC>ACF>HPA. They mainly occurred in micropore adsorption, but their size had positive relationships with both functional groups and microporous volume. The hysteresis became smaller along with the increase of temperature, closely related with the stability of water clusters. In conclusion, temperature, functional groups and porous structure played crucial roles for water vapor adsorption and the formation of hysteresis.
Implications: Water vapor is one of the vital influence for VOCs recovery, so studying the adsorption mechanism of water vapor is important to weaken its negative effect. Adsorption capacities of water vapor on both micropores and functional groups had a negative logarithmic linear relationship with temperature. The existence of functional groups could weaken the negative influence of temperature on the water adsorption performance, while the influence of temperature had negligible relationship with microporous volume. The hysteresis loops on three adsorbents mainly occurred in micropore adsorption, but their size had positive relationships with both functional groups and microporous volume.
Introduction
Adsorption is widely accepted to be an effective technique to control the emission of volatile organic compounds (VOCs) and to recover valuable materials. While water vapor is ubiquitous in the environment and may compete with VOCs during the adsorption process (Carter, Katz, and Speitel Citation2011; Lee and Reucroft Citation1999), which involves a decrease of adsorption capacity (Agueda et al. Citation2011; Marban and Fuertes Citation2004; Sager and Schmidt Citation2010), kinetics (Qi et al. Citation2006), and selectivity of given adsorbents toward a mixture of VOCs (Zhao et al. Citation2015). Incontrast, water vapor could reduce the temperature rise during adsorption (Jia, Shi, and Xie Citation2018). Hence, it has beenvital to explore the adsorption equilibrium characteristics of water vapor on different adsorbents to reduce the negative influence of water vapor (Fletcher, Uygur, and Thomas Citation2007; Fletcher, Yuzak, and Thomas Citation2006; Long, Li, and Yu Citation2012; Qian, Sunohara, and Kato Citation2008).
Generally, adsorption isotherms of water vapor are S-shaped type IV or V (Kim, Zheng, and Agnihotri Citation2008; Yao, Li, and Li Citation2015). What is extensively accepted is that at low relative pressure (less than 0.2), water vapor initially adsorbs on functional groups (Devlin, Sadlej, and Buch Citation2001; Do and Do Citation2000). Then the adsorbed water molecules, being secondary sites, could adsorb more water molecules to form clusters, which grow until a critical size is reached. With enough dispersion energy (when the relative pressure is higher than 0.8), these clusters finally fill the micropores (Horikawa, Sakao, and Do Citation2013; Lagorsse, Campo, and Magalhaes Citation2005; Nguyen, Horikawa, and Do Citation2014). In addition, it has been discussed widely that temperature, functional groups and porous structure of adsorbent could affect the water vapor adsorption (Barton, Evans, and MacDonald Citation1994; Brennan, Bandosz, and Thomson Citation2001; Hanzawa and Kaneko Citation1997; Jia, Yao, and Ma Citation2017; Jorge, Schumacher, and Seaton Citation2002; Kaneko, Hanzawa, and Iiyama Citation1999; Liu et al. Citation2021; Nishino Citation2002; Thommes, Morell, and Cychosz Citation2013; Tu, Kwag, and Barnette Citation2012; Velasco et al. Citation2017; Xiao, Liu, and Kim Citation2013). Several studies stated that water vapor can adsorb in micro- and mesopores (Kaneko, Hanzawa, and Iiyama Citation1999; Liu et al. Citation2021; Qian, Sunohara, and Kato Citation2008; Velasco et al. Citation2017), while Hanzawa and Kaneko (Citation1997) argued that mostly water vapor adsorbed on micropores even with the existence of mesopores. For the effect of functional groups, it is accepted that the carboxyl group had the greatest affinity with water molecules and was more conducive to the adsorption of water vapor (Nishino Citation2002; Thommes, Morell, and Cychosz Citation2013). However, Xiao et al. (Citation2013) stated that functional groups played different roles in different relative partial pressures, for example, –COOH and –S = O played a major role in RH<40%, but –OH affected adsorption at medium and high humidity (40%<RH<70%). Barton et al. (Citation1994) and Jorge et al. (Citation2002) found that water vapor adsorption was related to the content of functional groups. Finally, as for the temperature, Horikawa et al. (Citation2013) stated that water vapor adsorption showed was independent on temperature among 263 K to 298 K, while Wang, Tian, and Li (Citation2019) presented that the influence of temperature may change at different adsorption stages for water vapor. Therefore, the factors that have an effect on water vapor adsorption are worth further research.
Hysteresis, closely related with equilibrium adsorption mechanism of water vapor, is one of the least understood phenomena (Morishige, Kawai, and Kittaka Citation2014; Oh, Shim, and Lee Citation2003), including its formation mechanism and influencing factors. Capillary condensation is widely accepted for the existence of hysteresis in mesopores. While in water vapor adsorption-desorption systems, various explanations and theories were argued (Horikawa, Do, and Nicholson Citation2011; Horikawa, Muguruma, and Do Citation2015; Nakamura, Ohba, and Branton Citation2010; Zimny, Finqueneisel, and Cossarutto Citation2005). Hysteresis for water vapor adsorption on microporous adsorbents has been widely published (Miyawaki, Kanda, and Kaneko Citation2001; Sullivan, Stone, and Hashisho Citation2007). Horikawa et al. (Citation2015) believed that the hysteresis during water adsorption on microporous carbons did not come from capillary condensation. It is widely accepted that hysteresis is due to the manifestation of metastable states of adsorbed water (Nakamura, Ohba, and Branton Citation2010; Shi and Avramidis Citation2017). Ohba and Kaneko (Citation2011) stated that hysteresis comes from the kinetically forbidden structural transformations of adsorbed water. Other researchers argued that during adsorption, water molecules were adsorbed by forming water clusters, but desorption of water vapor was carried out by single water molecule (Dubinin, Zaverina, and Serpinsky Citation1955; Kimura, Kanoh, and Kanda Citation2004; Ohba and Kaneko Citation2007). We need to further research the hysteresis formation of water vapor.
In addition, formation hysteresis is related to the adsorption mechanism of water vapor, so the temperature, functional groups and porous structure of adsorbent might also affect the formation, size and location of hysteresis(Miyawaki, Kanda, and Kaneko Citation2001; Sullivan, Stone, and Hashisho Citation2007; Velasco, Guillet-Nicolas, and Dobos Citation2016; Wongkoblap and Do Citation2007). Miyawaki et al. (Citation2001) and Ohba and Kaneko (Citation2011) found that hysteresis loop became larger along with increasing of pore width when the average pore diameter(w) was smaller than 1.1 nm. Velasco et al. (Citation2016) observed that with pore width lower than 3 nm, hysteresis of water vapor could appear. They found that both the surface chemistry and porous structure of adsorbent could affect the shape and width of hysteresis loops. Some other studies expressed that the hysteresis loop size was closely related with the concentration of the functional group(Sullivan, Stone, and Hashisho Citation2007; Wongkoblap and Do Citation2007). Therefore, the effect of properties of adsorbent on hysteresis formation is worth further research. Regardingthe effect of temperature on hysteresis, Rudisill, Hacskaylo, and Levan (Citation1992) concluded that with increasing temperature, the hysteresis loop shrank and migrated toward high pressure. Liu, Zeng, and Tan (Citation2019) presented that with increasing temperature, the onset of filling the micropore shifts to lower relative pressures and the onset of evaporation shifts to higher relative pressures, in contrast with other studies (Centineo, Nguyen, and Espinal Citation2019; Ohba and Kaneko Citation2004). Hence, the influence of temperature on the size and location of the hysteresis loop still need to be studied thoroughly and deeply. This is another motivation for further investigation.
The adsorption equilibrium of water vapor have been studied on different materials, such as activated carbon (Qian, Sunohara, and Kato Citation2008; Yao, Li, and Li Citation2015; Zeng, Zhang, and Li Citation2015), zeolite (Dawoud Citation2013), metal organic frameworks (Küsgens et al. Citation2009), goethite (Song and Boily Citation2013). To the best of our knowledge, few investigations for adsorption hysteresis of water vapor on hypercrosslinked polymeric adsorbents have been reported, which are of critical importance in recovering VOCs. In the present paper, we chose hypercrosslinked polymeric adsorbents (HPA) as adsorbents, comparing them with granular activated carbons (GAC) and activated carbon fiber (ACF). Then we investigated the adsorption and hysteresis formation of water vapor, and the influencing factors (temperature, functional groups, and porous structure of adsorbent) systematically. Adsorption-desorption equilibrium of water vapor were conducted at four temperatures (298 K, 308 K, 318 K, and 328 K), to interpret the influence of temperature.
Materials and methods
Materials
HPA were prepared via post-crosslinking of low-crosslinked macroporouspoly (styrene-divinylbenzene) (Jia, Yao, and Ma Citation2017). GAC and ACF were supplied by MeadWestvaco Company (Richmond, USA) and Jiangsu Sutong Carbon Fiber Co. Ltd. (Jiangsu, China) separately. The porous texture of HPA, ACF and GAC were determined by N2 adsorption-desorption isotherms data at 77 K, using an adsorption analyzer ASAP 2020 (Micromeritics Instrument Co., USA). Brunauer–Emmett–Teller (BET), Dubinin–Astakhov(DA), and Barrett–Joyner–Halenda(BJH) equation were applied to calculate their specific surface area (SBET), micropore volume (Vmicro) and mesopore volume (Vmeso). The functional groups of carbon–oxygen complexes on adsorbent were measured according to Boehm titration (Boehm Citation1994). In detail, the amount of various acidic sites were calculated by assuming that sodium bicarbonate only neutralizes the carboxylic group, sodium carbonate reacts with carboxylic and lactonic groups, sodium hydroxide neutralizes carboxylic, lactonic and phenolic groups, and sodium ethoxide neutralizes carboxyl, lactone, phenolic and carbonyl groups. The physical-chemical properties of three adsorbents were listed in .
Table 1. Selected properties of HPA, ACF and GAC (Jia, Yao, and Ma Citation2017)
Adsorption methods
The detailed adsorption experimental apparatus were shown in . Briefly, the experimental setup included three functional sections: vapor generation, adsorption and data analysis (Jia, Yao, and Ma Citation2017). About 40 mg of adsorbents was placed on the sample pan in a thermostatic chamber. Prior to use, adsorbents were kept in a drying vacuum oven at 383 K for more than 2 hr to remove impurities. Water vapor at certain relative pressure (0.1–0.95), controlled by mass flow controller, was passed through the adsorbents and when the weight change was stable for 1.0 hr, the relative pressure was adjusted to a higher one. After the equilibrium adsorption of water vapor at P/P0 = 0.95, that is, the adsorption isotherm was finished, the relative pressure was adjusted from 0.95 to 0 gradually to obtain desorption isotherm. The adsorption and desorption temperature were set at 298 K, 308 K, 318 K and 328 K.
CIMF model
The cluster formation induced micropore filling (CIMF) model, shown in EquationEq. (1(1)
(1) ), modified from D.D. Do equation (Kim and Agnihotri Citation2008), but it assumed that the cluster size of water molecules depend upon the concentration of the functional groups and the pore width, not fixing to a pentamer or heptamer. CIMF model is a more suitable semi-empirical equation for interpreting the water vapor adsorption isotherms.
where Cμ(mg/g) is the total amount of adsorbed water at a specific P/P0, S0(mg/g) is the concentration of primary adsorption sites, n is the maximum number of water molecules adsorbed onto the functional groups, Kf is the equilibrium constant including water chemisorption on the functional groups and hydrogen bonding of water on the adsorbed water molecules, Cμs(mg/g) is the maximum adsorption capacity in micropore, m is the average number of water molecules forming the clusters that migrate into the pores, and Kμ is the equilibrium constant for water cluster filling into the pores.
Results and discussion
Adsorption equilibrium of water vapor
Effect of adsorbent properties
showed the adsorption-desorption equilibrium isotherms (a–c) of water vapor on all three adsorbents at four temperatures, with the zoomed-in view of isotherms at 318 and 328 K on the right parts (d–f). The adsorption isotherms were type V, in accordance with other literature (Linders, Van Den Broeke, and Kapteijn Citation2001; Lodewyckx, Rompaey, and Verhoeven Citation2001). That is, water vapor begins to adsorb at specific active functional groups at low relative humidity. Then acting as secondary sites, the adsorbed water molecules could further adsorb water molecules to form clusters, which grow and finally fill the micropores as the relative pressure increases.
Figure 2. Adsorption-desorption isotherms (a,b,c) of water vapor on HPA, ACF and GAC at four temperatures and the zoomed-in view at 318 K and 328 K(d,e,f).
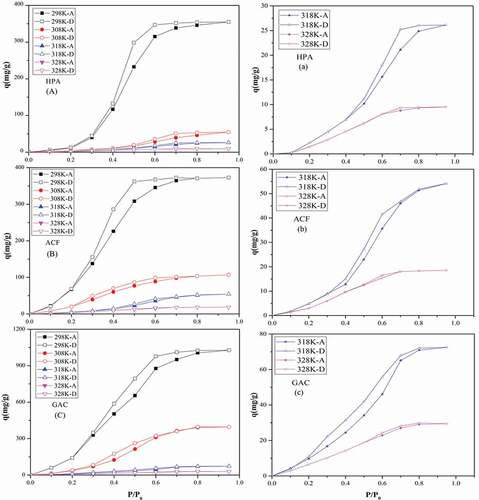
We used CIMF model to fit the adsorption and desorption isotherms of water vapor on three adsorbents at four temperatures and investigated the influence of temperature, surface chemistry and porous structure. The explanation of CIMF parameters were in supporting Information, and the fitting results were given in and Table S1, showing a good fitting result (R2 >0.92). This confirmed further that in this study, water vapor adsorbed by filling of water clusters into micropores. According to the values listed in , we could observe that both maximum adsorption capacity in micropore (Cus) and adsorption capacity on hydrophilic functional groups (S0) varied with temperatures and properties of adsorbents.
Table 2. CIMF fitting results of water vapor adsorption on HPA, ACF and GAC
It is clear in that the adsorption capacities of water vapor were: HPA<ACF<GAC, relevant to the microporous volume and concentration of functional groups on three adsorbents. At 298 K, the saturated adsorption amount of water vapor on HPA, ACF and GAC was 0.35, 0.37 and 1.03 cm3/g at P/P0 = 0.95, approximate to the microporous volume of three adsorbents, which was 0.43, 0.45 and 0.84 cm3/g, separately (Brennan, Bandosz, and Thomson Citation2001). Considering the mesoporous volume of three adsorbents, we could find that the values for HPA, ACF and GAC were 0.36, 0.03 and 0.78 cm3/g, which showed neither negative (e.g., ACF) nor positive (e.g., GAC) effect on water vapor adsorption. Hence, we support the standpoint that water vapor adsorption mainly occurred on functional groups and micropores. For the GAC, saturated adsorption volume of water vapor was slightly higher than its microporous volume. It may come from its higher concentration of functional groups on GAC, enhancing its water adsorption uptake (Thommes, Morell, and Cychosz Citation2013). And water clusters are feasible to penetrate into micropores due to the hydrogen-bond with the same physical restriction (Kim, Meyer, and Agnihotri Citation2009).
Based on , we made the relationship between fitting parameters (S0 and Cus) with functional groups and microporous volume of adsorbents. Firstly, the fitting parameter S0 was found to be related to the acidic group concentration of adsorbents, shown in . The positive correlation between S0 and total acidic group concentration in ), indicated that acidic group concentration helps to increase the adsorption capacity on functional groups. However, it is noticeable that plot between S0 value and acidic group concentration were an upward convex, which may be related to the various roles of different acidic groups. From the concentration data of surface functional groups on three adsorbents in Brennan et al. (Citation2001), we can see that –COOH and –COOR were not determined on ACF. To further explore the influence mechanism of adsorbents surface chemistries, plots of S0 vs concentration of separate –OH, –C = O, -COOH and –COOR groups were made, shown in Figure S1. The results showed that –OH, –C = O and –COOH groups could help to increase the adsorption capacity of water vapor on functional groups, while –COOR had a negative relationship with S0, without any linear relationship for S0 with concentrations of separate acidic groups. Hence, in ), we could observe good linear relationships between S0 and the summary concentration of –OH, –C = O and –COOH, excluding –COOR, demonstrating that all three groups play important roles for water adsorption. This result was similar to the studies in the literature (Thommes, Morell, and Cychosz Citation2013; Tu, Kwag, and Barnette Citation2012).
Figure 3. Relationship between the adsorption capacity of water vapor on acidic groups with (a) the concentration of total acidic groups and (b) acidic groups except for –COOR.
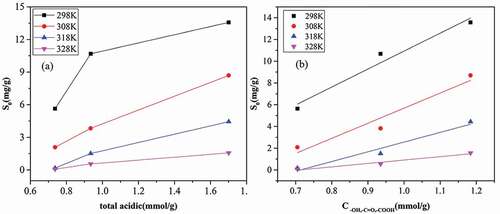
Secondly, the parameter Cµs was positive to the N2 microporous volume of adsorbent. Linear relationship between adsorption capacity in micropore (Cus) and microporous volume (Vmicro) was shown in , with rather higher coefficient (R2 >0.99), confirming the cluster induced pore-filling mechanism of water vapor again. Water vapor begins to adsorb at functional groups, and then on the adsorbed water molecules (secondary sites). Water clusters grow and finally fill the micropores as the relative pressure increases.
Effect of temperature
From , we could also find that water vapor adsorption capacity on three adsorbents decreased quickly with the increase of temperature during 298–308 K, while adsorption capacity at 308–328 K decreased gradually. This phenomenon may be due to the stability of water cluster reduced obviously with the increasing of temperature(Ohba and Kaneko Citation2004). With increasing temperature, the onset of adsorption in micropores shifts to higher relative pressures.
To better understand the effect of temperature on water vapor adsorption, we evaluated the adsorption behavior on functional groups and pores under different temperatures. Fitting parameters presented in , especially S0 and Cus, showed apparent dependenceupon temperature. It is clear that the value of S0 and Cμs decreased with the increasing temperature, which reduced adsorptive contributions on both functional groups and micropores. From the results, a map of the change in water vapor adsorption on functional groups and micropores with increasing temperatures was created, presented in , revealing a negative correlation with logarithm of temperature. This result was closely related to the adsorption mechanism of water vapor: adsorption through hydrogen-bond and water cluster formation followed by micropore filling. In addition, the lowering of S0 suggested water adsorbing on fewer functional groups. Thus, S0 does not mean the characterized concentration of functional groups but only the sites detected by water vapor. As for Cμs, because water molecules must obtain higher potential to stay in the micropore with the temperature rising and it became more difficult for water molecules to form clusters as the temperature increases (Wang, Tian, and Li Citation2019), the adsorption of water vapor in micropores decreased along with the increase of temperature.
Figure 5. Variation of S0 and Cμs with temperature for water vapor adsorption on HPA, ACF and GAC at four temperatures (298, 308, 318, and 328 K).
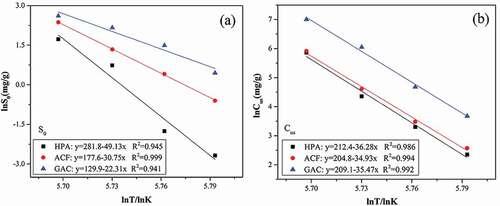
What is more, from the slopes in , we foundthat the effect of temperature on water adsorption on functional groups and micropores were different, related to the two different adsorption interactions: hydrogen bond and micropore filling. In ), the slope for HPA, ACF and GAC was −49.13, −30.75 and −22.31, respectively, that is HPA<ACF<GAC, similar with the order of functional groups concentration of three adsorbents: HPA (0.737 mmol/g) <ACF (0.935 mmol/g) <GAC (1.701 mmol/g). It is obvious that functional groups could weaken the negative influence of temperature on the adsorption of water vapor on functional groups, because the higher temperature could supply higher energy for the formation of hydrogen bond and water molecules adsorbed to functional groups could display better thermal stability (Horikawa, Tan, and Do Citation2017). While from ), the slopes on three adsorbents were approximate, indicating the effect of temperature on water vapor adsorption in micropores had negligible relationship with microporous volume. In a word, concentration of functional groups on adsorbents play a key roleduring the adsorption of water vapor at different temperatures.
Hysteresis of water vapor
Hysteresis formation of water vapor
also demonstrated that water vapor adsorption on three adsorbents showed representative hysteresis. As we addressed earlier, water vapor was adsorbed through hydrogen bond with functional groups, formation of water clusters and micropore filling. The hysteresis formation of water vapor in this study did not come from traditional capillary condensation (Kaneko, Hanzawa, and Iiyama Citation1999; Qian, Sunohara, and Kato Citation2008).
To illuminate the hysteresis formationn of water vapor, we calculated the adsorption and desorption capacities of water vapor on functional groups and micropore respectively according to fitting parameters in and Table S1, shown in and Figures S2–S4. In four figures, the left parts showed both of the hysteresis in micropore and functional groups, and the right parts showed the zoomed-in view of hysteresis on functional groups. It could be observed that for water vapor adsorption on functional groups, there existed hysteresis, but there were more obvious hysteresis loops for water adsorption in micropores. This could lead to a conclusion that the hysteresis loop for water vapor adsorption on functional groups was negligible. Hence, even though both functional groups and microporous volume affected the adsorption of water vapor, the formation of its hysteresis mainly happens in microporous adsorption, where the water clusters and their micropore filling wasprocessed. Water clusters were stable (Ohba and Kaneko Citation2004) and the kinetically forbidden structural transformations of adsorbed water could lead to a hysteresis loop (Ohba and Kaneko Citation2011).
Effect of adsorbent properties
As is widely known, during the water vapor adsorption, hydrogen-bonded water, water clusters and micropore filling are interdependent. Even though we concluded from the former sectionthat the hysteresis loop mainly occurs on water vapor adsorption in micropores, functional groups concentration could affect the water clusters formation and their micropore filling, and then affect the hysteresis formation. We calculated the hysteresis loop size (Shysteresis) of water vapor on three adsorbents with an integral method, to analyze the relationship between Shysteresis and properties of adsorbents (concentration of functional groups (Cacidic) and microporous volume (Vmicro). To eliminate the interactional influence of functional groups and porous structure, we made the relationship of Shysteresis/Vmicro with Cacidic, shown in ) and relationship between Shysteresis/Cacidic and Vmicro, shown in ). We could observe that the hysteresis loop size were proportional positively to both of the functional groups concentration and microporous volume of three adsorbents. Hence, both surface chemistry and pore structure of adsorbents played vital voles for the formation and size of hysteresis loop, even though the hysteresis mainly displayed during the water vapor adsorption in micropores. It is worth mentioning that the hysteresis loop size (GAC>ACF>HPA) had no obvious relationship with the average pore diameter, as shown in (GAC(2.73)>HPA(2.70)>ACF(1.63)).
Effect of temperature
also presented that the rising and dropping pressures where hysteresis existed were not necessarily sensitive to the temperature. But the hysteresis loops of water vapor shrank with the increasing of temperature (Ohba, Kanoh, and Kaneko Citation2005; Striolo et al. Citation2005). It could be observed clearly in that the hysteresis loop size of water vapor on three adsorbents decreased gradually and at higher temperature (318–328 K), the hysteresis loops were approximate to zero. In and Figure S2-S4, we could notice that both the hysteresis size on functional groups and micropores decreased with increasing temperature. For the hysteresis on functional groups, the higher temperature could supply energy for breaking of hydrogen bond, leading to smaller hysteresis. In the micropores, the size and stability of clusters can also be affected by temperature. At higher temperatures, hydrogen bond between water molecules became weak and water clusters changed to unstable (Ohba and Kaneko Citation2004) and might decentralize easily, resulting in easier desorption of water vapors and a smaller hysteresis loop. This further confirmed that the formation of hysteresis mainly resulted from the water clusters that filled the micropore. In conclusion, the formation of hysteresis could be affected by temperature, functional groups and microporous volume of adsorbent, but it mainly occurred during the adsorption in micropore.
Conclusion
(1) Adsorption capacity of water vapor were: HPA<ACF<GAC, basically similar to their microporous volume and functional groups concentrations. The adsorption capacity on functional groups and micropores had a logarithmic linear relationship with temperature. The existence of functional groups could weaken the negative influence of temperature on the water vapor adsorption on functional groups, while the influence of temperature on water vapor adsorption in micropores had negligible relationship with microporous volume.
(2) The hysteresis loops at different temperatures on three adsorbents had similar shape, the size of which were as follows: GAC>ACF>HPA. They mainly occurred in microporous adsorption, but their size had positive relationships with both functional groups and microporous volume. The hysteresis became smaller along with the increase of temperature, closely related to the stability of water clusters.
Supporting information
CIMF parameters were listed in Table S1. Fig. S1 displayed the relationship between the water vapor adsorption capacity on functional groups and the concentrations of different functional groups on adsorbents. Adsorption and desorption isotherms of water vapor respectively on micropore and functional groups on three adsorbents were presented in Fig. S2-S4.
Supporting_Information.docx
Download MS Word (2.1 MB)Acknowledgment
This research was financially funded by the National Natural Science of China (Grant No. 51808485), Department of Education, Shanxi Province (Grant No. 2019L0853), Department of Science and Technology, Shanxi Province (Grant No. 201901D211459) and Research Project Supported by Shanxi Scholarship Council of China (Grant No. 2021-150).
Data availability statement
The data supporting the findings of this study are available within the paper and its supporting information.
Disclosure statement
No potential conflict of interest was reported by the author(s).
Supplemental data
Supplemental data for this paper can be accessed on the publisher’s website.
Additional information
Funding
Notes on contributors
Lijuan Jia
Lijuan Jia is an associate professor in Department of Applied Chemistry, Yuncheng University, Yuncheng, China.
Ben Niu
Ben Niu is a manager and experimenter in the Department of Logistics, Yuncheng University, Yuncheng, China.
Xiaoxia Jing
Xiaoxia Jing is an associate professor in Department of Applied Chemistry, Yuncheng University, Yuncheng, China.
Yangfang Wu
Yangfang Wu is a lecturer in Department of Applied Chemistry, Yuncheng University, Yuncheng, China.
References
- Agueda, V. I., B. D. Crittenden, J. A. Delgado, and S. R. Tennison. 2011. Effect of channel geometry, degree of activation, relative humidity and temperature on the performance of binderless activated carbon monoliths in the removal of dichloromethane from air. Sep. Purif. Technol. 78 (2):154–63. doi:https://doi.org/10.1016/j.seppur.2011.01.036.
- Barton, S. S., M. J. B. Evans, and J. A. F. MacDonald. 1994. Adsorption of water vapor on nonporous carbon. Langmuir 10 (11):4250–52. doi:https://doi.org/10.1021/la00023a055.
- Boehm, H. P. 1994. Some aspects of the surface-chemistry of Carbon-Blacks and other carbons. Carbon 32 (5):759–69. doi:https://doi.org/10.1016/0008-6223(94)90031-0.
- Brennan, J. K., T. J. Bandosz, and K. T. Thomson. 2001. Water in porous carbons. Colloid Surface A. 187– 188:539–68. doi:https://doi.org/10.1016/S0927-7757(01)00644-6.
- Carter, E. M., L. E. Katz, and G. E. Speitel. 2011. Gas-phase formaldehyde adsorption isotherm studies on activated carbon: Correlations of adsorption capacity to surface functional group density. Environ. Sci. Technol. 45 (15):6498–503. doi:https://doi.org/10.1021/es104286d.
- Centineo, A., H. G. T. Nguyen, and L. Espinal. 2019. An experimental and modelling study of water vapour adsorption on SBA-15. Microporous Mesoporous Mater. 282:53–72. doi:https://doi.org/10.1016/j.micromeso.2019.03.018.
- Dawoud, B. 2013. Water vapor adsorption kinetics on small and full scale zeolite coated adsorbers; A comparison. Appl. Therm. Eng. 50 (2):1645–51. doi:https://doi.org/10.1016/j.applthermaleng.2011.07.013.
- Devlin, J. P., J. Sadlej, and V. Buch. 2001. Infrared spectra of large H2O clusters: New understanding of the elusive bending mode of ice. J. Phys. Chem. A. 105 (6):974–83. doi:https://doi.org/10.1021/jp003455j.
- Do, D. D., and H. D. Do. 2000. A model for water adsorption in activated carbon. Carbon 38 (5):767–73. doi:https://doi.org/10.1016/S0008-6223(99)00159-1.
- Dubinin, M. M., E. D. Zaverina, and V. V. Serpinsky. 1955. The sorption of water vapour by active carbon. J. Chem. Soc. 77:1760–66. doi:https://doi.org/10.1039/jr9550001760.
- Fletcher, A. J., Y. Uygur, and K. M. Thomas. 2007. Role of surface functional groups in the adsorption kinetics of water vapor on microporous activated carbons. J. Phys. Chem. C 111 (23):8349–59. doi:https://doi.org/10.1021/jp070815v.
- Fletcher, A. J., Y. Yuzak, and K. M. Thomas. 2006. Adsorption and desorption kinetics for hydrophilic and hydrophobic vapors on activated carbon. Carbon 44 (5):989–1004. doi:https://doi.org/10.1016/j.carbon.2005.10.020.
- Hanzawa, Y., and K. Kaneko. 1997. Lack of a predominant adsorption of water vapor on carbon mesopores. Langmuir 13 (22):5802–908. doi:https://doi.org/10.1021/la970498r.
- Horikawa, T., D. D. Do, and D. Nicholson. 2011. Capillary condensation of adsorbates in porous materials. Adv. Colloid Interface. 169 (1):40–58. doi:https://doi.org/10.1016/j.cis.2011.08.003.
- Horikawa, T., T. Muguruma, and D. D. Do. 2015. Scanning curves of water adsorption on graphitized thermal carbon black and ordered mesoporous carbon. Carbon 95:137–43. doi:https://doi.org/10.1016/j.carbon.2015.08.034.
- Horikawa, T., N. Sakao, and D. D. Do. 2013. Effects of temperature on water adsorption on controlled microporous and mesoporous carbonaceous solids. Carbon 56:183–92. doi:https://doi.org/10.1016/j.carbon.2013.01.003.
- Horikawa, T., S. J. Tan, and D. D. Do. 2017. Temperature dependence of water adsorption on highly graphitized carbon black and highly ordered mesoporous carbon. Carbon 124:271–80. doi:https://doi.org/10.1016/j.carbon.2017.08.067.
- Jia, L., Q. Shi, and S. Xie. 2018. Effect of pre-adsorbed water in hydrophobic polymeric resin on adsorption equilibrium and breakthrough of 1, 2-dichloroethane. Adsorption 24 (1):73–80. doi:https://doi.org/10.1007/s10450-017-9919-9.
- Jia, L., X. Yao, and J. Ma. 2017. Adsorption kinetics of water vapor on hypercrosslinked polymeric adsorbent and its comparison with carbonaceous adsorbents. Microporous Mesoporous Mater. 241:178–84. doi:https://doi.org/10.1016/j.micromeso.2016.12.028.
- Jorge, M., C. Schumacher, and N. A. Seaton. 2002. Simulation study of the effect of the chemical heterogeneity of activated carbon on water adsorption. Langmuir 18 (24):9296–306. doi:https://doi.org/10.1021/la025846q.
- Kaneko, K., Y. Hanzawa, and T. Iiyama. 1999. Cluster-mediated water adsorption on carbon nanopores. Adsorption 5 (1):7–13. doi:https://doi.org/10.1023/A:1026471819039.
- Kim, P., and S. Agnihotri. 2008. Application of water–activated carbon isotherm models to water adsorption isotherms of single-walled carbon nanotubes. J. Colloid Interface Sci. 325 (1):64–73. doi:https://doi.org/10.1016/j.jcis.2008.06.002.
- Kim, P., H. M. Meyer, and S. Agnihotri. 2009. Effect of surface oxygen and temperature on external and micropore adsorption of water in single-walled carbon nanotubes by gravimetric and spectroscopic experiments. J. Phys. Chem. C 113 (28):12109–17. doi:https://doi.org/10.1021/jp900609a.
- Kim, P., Y. Zheng, and S. Agnihotri. 2008. Adsorption equilibrium and kinetics of water vapor in carbon nanotubes and its comparison with activated carbon. Ind. Eng. Chem. Res. 47 (9):3170–78. doi:https://doi.org/10.1021/ie0713240.
- Kimura, T., H. Kanoh, and T. Kanda. 2004. Cluster-associated filling of water in hydrophobic carbon micropores. J. Phys. Chem. B. 108 (37):14043–48. doi:https://doi.org/10.1021/jp048934n.
- Küsgens, P., M. Rose, I. Senkovska, H. Fröde, A. Henschel, S. Siegle, and S. Kaskel. 2009. Characterization of metal-organic frameworks by water adsorption. Microporous Mesoporous Mater. 120 (3):325–30. doi:https://doi.org/10.1016/j.micromeso.2008.11.020.
- Lagorsse, S., M. C. Campo, and F. D. Magalhaes. 2005. Water adsorption on carbon molecular sieve membranes: Experimental data and isotherm model. Carbon 43 (13):2769–79. doi:https://doi.org/10.1016/j.carbon.2005.05.042.
- Lee, W. H., and P. J. Reucroft. 1999. Vapor adsorption on coal- and wood-based chemically activated carbons (I) Surface oxidation states and adsorption of H2O. Carbon 37 (1):7–14. doi:https://doi.org/10.1016/S0008-6223(98)00181-X.
- Linders, M. J. G., L. J. P. Van Den Broeke, and F. Kapteijn. 2001. Binary adsorption equilibrium of organics and water on activated carbon. AICHE J. 47 (8):1885–92. doi:https://doi.org/10.1002/aic.690470818.
- Liu, L., W. Zeng, S. Tan, M. Liu, and D. D. Do. 2021. On the characterization of bimodal porous carbon via water adsorption: The role of pore connectivity and temperature. Carbon 179:477–85. doi:https://doi.org/10.1016/j.carbon.2021.04.041.
- Liu, L., Y. Zeng, and S. J. Tan. 2019. On the mechanism of water adsorption in carbon micropores- A molecular simulation study. Chem. Eng. J. 357:358–66. doi:https://doi.org/10.1016/j.cej.2018.09.160.
- Lodewyckx, P., D. V. Rompaey, and L. Verhoeven. 2001. Water isotherms of activated carbons with small amounts of surface oxygen groups: Fitting the mesopore region. Carbon 39:309–10.
- Long, C., Y. Li, and W. Yu. 2012. Adsorption characteristics of water vapor on the hypercrosslinked polymeric adsorbent. Chem. Eng. J. 180:106–12. doi:https://doi.org/10.1016/j.cej.2011.11.019.
- Marban, G., and A. B. Fuertes. 2004. Co-adsorption of n-butane/water vapour mixtures on activated carbon fibre-based monoliths. Carbon 42 (1):71–81. doi:https://doi.org/10.1016/j.carbon.2003.09.018.
- Miyawaki, J., T. Kanda, and K. Kaneko. 2001. Hysteresis-associated Pressure- shift-induced water adsorption in carbon micropores. Langmuir 17 (3):664–69. doi:https://doi.org/10.1021/la000175m.
- Morishige, K., T. Kawai, and S. Kittaka. 2014. Capillary condensation of water in mesoporous carbon. J. Phys. Chem. C 118 (9):4664–69. doi:https://doi.org/10.1021/jp4103564.
- Nakamura, M., T. Ohba, and P. Branton. 2010. Equilibration-time and pore-width dependent hysteresis of water adsorption isotherm on hydrophobic microporous carbons. Carbon 48 (1):305–12. doi:https://doi.org/10.1016/j.carbon.2009.09.008.
- Nguyen, V. T., T. Horikawa, and D. D. Do. 2014. Water as a potential molecular probe for functional groups on carbon surfaces. Carbon 67:72–78. doi:https://doi.org/10.1016/j.carbon.2013.09.057.
- Nishino, J. 2002. Adsorption of water vapor and carbon dioxide at carboxylic functional groups on the surface of coal. Fuel 80 (5):757–64. doi:https://doi.org/10.1016/S0016-2361(00)00136-8.
- Oh, J. S., W. G. Shim, and J. W. Lee. 2003. Adsorption equilibrium of water vapor on mesoporous materials. J. Chem. Eng. Data. 48 (6):1458–62. doi:https://doi.org/10.1021/je0301390.
- Ohba, T., and K. Kaneko. 2004. Cluster-growth-induced water adsorption in hydrophobic carbon nanopores. J. Phys. Chem. B. 108 (39):14964–69. doi:https://doi.org/10.1021/jp048323v.
- Ohba, T., and K. Kaneko. 2007. Cluster-associated filling of water molecules in slit-shaped graphitic nanopores. Mol. Phys. 105 (2–3):139–45. doi:https://doi.org/10.1080/00268970701192081.
- Ohba, T., and K. Kaneko. 2011. Kinetically forbidden transformations of water molecular assemblies in hydrophobic micropores. Langmuir 27 (12):7609–13. doi:https://doi.org/10.1021/la201115s.
- Ohba, T., H. Kanoh, and K. Kaneko. 2005. Structures and stability of water nanoclusters in hydrophobic nanospaces. Nano Lett. 5 (2):227–30. doi:https://doi.org/10.1021/nl048327b.
- Qi, N., W. S. Appel, M. D. LeVan, and J. E. Finn. 2006. Adsorption dynamics of organic compounds and water vapor in activated carbon beds. Ind. Eng. Chem. Res. 45 (7):2303–14. doi:https://doi.org/10.1021/ie050758x.
- Qian, Q., S. Sunohara, and Y. Kato. 2008. Water vapor adsorption onto activated carbons prepared from cattle manure compost (CMC). Appl. Surf. Sci. 254 (15):4868–74. doi:https://doi.org/10.1016/j.apsusc.2008.01.111.
- Rudisill, E. N., J. J. Hacskaylo, and M. D. Levan. 1992. Coadsorption of hydrocarbons and water on BPL activated carbon. Ind. Eng. Chem. Res. 31 (4):1122–30. doi:https://doi.org/10.1021/ie00004a022.
- Sager, U., and F. Schmidt. 2010. Binary adsorption of n-butane or toluene and water vapor. Chem. Eng. Technol. 33 (7):1203–07. doi:https://doi.org/10.1002/ceat.201000086.
- Shi, J., and S. Avramidis. 2017. Water sorption hysteresis in wood: I review and experimental patterns -geometric characteristics of scanning curves. Holzforschung 71 (4):307–16. doi:https://doi.org/10.1515/hf-2016-0120.
- Song, X., and J. F. Boily. 2013. Water vapor adsorption on goethite. Environ. Sci. Technol. 47 (13):7171–77. doi:https://doi.org/10.1021/es400147a.
- Striolo, A., K. Gubbins, M. Gruszkiewicz, D. Cole, J. Simonson, and A. Chialvo. 2005. Effect of temperature on the adsorption of water in porous carbons. Langmuir 21 (21):9457–67. doi:https://doi.org/10.1021/la051120t.
- Sullivan, P. D., B. R. Stone, and Z. Hashisho. 2007. Water adsorption with hysteresis effect onto micropoorus activated carbon fabrics. Adsorption 13 (3–4):173–89. doi:https://doi.org/10.1007/s10450-007-9033-5.
- Thommes, M., J. Morell, and K. A. Cychosz. 2013. Combining nitrogen, argon, and water adsorption for advanced characterization of ordered mesoporous carbons (CMKs) and Periodic Mesoporous Organosilicas (PMOs). Langmuir 29 (48):14893–902. doi:https://doi.org/10.1021/la402832b.
- Tu, A., H. R. Kwag, and A. L. Barnette. 2012. Water adsorption isotherms on CH3-, OH-, and COOH-terminated organic surfaces at ambient conditions measured with PM-RAIRS. Langmuir 28 (43):15263–69. doi:https://doi.org/10.1021/la302848k.
- Velasco, L. F., I. Berezovska, Y. Boutillara, and P. Lodewyckx. 2017. The use of organic vapour preadsorption to understand water adsorption on activated carbons. Microporous Mesoporous Mater. 241:21–27. doi:https://doi.org/10.1016/j.micromeso.2016.12.005.
- Velasco, L. F., R. Guillet-Nicolas, and G. Dobos. 2016. Towards a better understanding of water adsorption hysteresis in activated carbons by scanning isotherms. Carbon 96:753–58. doi:https://doi.org/10.1016/j.carbon.2015.10.017.
- Wang, T., S. Tian, and G. Li. 2019. Experimental study of water vapor adsorption behaviors on shale. Fuel 248:168–77. doi:https://doi.org/10.1016/j.fuel.2019.03.029.
- Wongkoblap, A., and D. D. Do. 2007. Adsorption of water in finite length carbon slit pore: Comparison between computer simulation and experiment. J. Phys. Chem. B. 111 (50):13949–56. doi:https://doi.org/10.1021/jp0747297.
- Xiao, J., Z. Liu, and K. Kim. 2013. S/O-functionalities on modified carbon materials governing adsorption of water vapor. J. Phys. Chem. C 117 (44):23057–65. doi:https://doi.org/10.1021/jp408716e.
- Yao, X., L. Li, and H. Li. 2015. Water vapor adsorption in activated carbon modified with hydrophilic organic salts. J. Cent. South Univ. 22 (2):478–86. doi:https://doi.org/10.1007/s11771-015-2546-2.
- Zeng, Q., D. Zhang, and K. Li. 2015. Kinetics and equilibrium isotherms of water vapor adsorption/desorption in cement-based porous materials. Transp. Porous Med. 109 (2):469–93. doi:https://doi.org/10.1007/s11242-015-0531-8.
- Zhao, Z. X., S. Wang, Y. Yang, X. M. Li, J. Li, and Z. Li. 2015. Competitive adsorption and selectivity of benzene and water vapor on the microporous metal organic frameworks (HKUST-1). Chem. Eng. J. 259:79–89. doi:https://doi.org/10.1016/j.cej.2014.08.012.
- Zimny, T., G. Finqueneisel, and L. Cossarutto. 2005. Water vapor adsorption on activated carbon preadsorbed with naphtalene. J. Colloid Interface Sci. 285 (1):56–60. doi:https://doi.org/10.1016/j.jcis.2004.11.029.