ABSTRACT
This research conducted the effect of different ethanol blends in a conventional gasoline engine under various load and speed conditions for its performance and emission characteristics. The experiment was tested on the test motorcycle designed initially to run by gasoline fuel. The experimental procedure was performed in the motorcycle testbed equipped, measuring power, fuel consumption, and exhaust emissions. NOx and HC emissions of the test vehicle using ethanol blends are lower than gasoline. However, NOx emissions have an opposite trend; they increased approximately 7.4% with E5, 12.3% with E10, and 18.51% with E20 due to the temperature increase. Furthermore, the ethanol contents provide the leaning effect to enhance the air-fuel equivalence ratio to a more excellent value and result in the burning closer to stoichiometric. As a result, improved combustion and increased power output are possible.
Implications: This paper aims to find out the solution to solve the problem related to the energy crisis and polluted environment from the motorcycles in Vietnam.
Introduction
With the rapid development of the industry and society, the energy demand has been growing dramatically for the last several decades due to the industry and society; therefore, there is great anxiety about energy depletion because of limited oil reserves or political crisis. Optimal engine operating conditions and design are the right solutions to save fuel; furthermore, finding alternative energy sources to replace fossil fuel (Duc, Tien, and Duy Citation2018a; Duy et al. Citation2019; Duy and Kim Citation2020; Lee et al. Citation2015; Nguyen Duc et al. Citation2019) partially. Also, polluted environment issues have been considered worldwide, and thus it is urgent to find some clean and suitable alternative fuels to meet environmental needs. Renewable energies produced from agricultural production can solve the problems mentioned above. Based on Vietnam’s experimental conditions, we concluded that ethanol fuel solves the energy crisis and environmental pollution and supports the agricultural sector’s development.
Indeed, due to the difference in ethanol and gasoline’s physical-chemical properties, some parts of the fuel supply system need to be modified. The ethanol’s octane number is higher than gasoline; it increases the engine’s antiknock property (Danaiah, Kumar, and Kumar Citation2013). Therefore, it is the basis for raising the engine-fueled compression ratio by ethanol to increase the heat efficiency and engine power output. However, it is not easy to modify original gasoline engines to run on ethanol fuel, as mentioned in (Dinh et al. Citation2020; Duc, Tien, and Duy Citation2018b; Han et al. Citation2020; Sahoo, Sahoo, and Saha Citation2009). Wei-Dong et al. conducted a study using different ethanol-gasoline blends for an internal combustion engine (Dorney et al. Citation2001). They found that ethanol combined contributed to decreasing CO and HC emissions about 10–90% and 20–80%, respectively. They also concluded that the blended fuels’ Reid vapor pressure improves to a maximum at 10% ethanol content and then reduces with increasing the ethanol content. An improvement in the engine characteristics could be observed in the same topic research performed by Al-Hasan. The results illustrated that ethanol in gasoline fuel blends increased the power output, torque, engine efficiency, and fuel consumption while reducing fuel consumption and equivalence air-fuel ratio. Also, the test engine’s CO and HC emission was decreased; meanwhile, the CO2 concentration was slightly increased (Al-Hasan Citation2003).
Many other publications performed different ethanol/gasoline blends for the original gasoline engines. For instance, Farhad et al. evaluated the effect of ethanol content in the mixture on gasoline in a spark-ignition engine. The results showed that 10% ethanol (E10) blends lead to a 5% improvement in the output power and octane number increase. Furthermore, it reduces CO emissions by up to 30% (Salek et al. Citation2021). Bata et al. concluded that ethanol blends reduce HC and CO emissions because of the ethanol’s oxygenated characteristic and wide flammability (Rice et al. Citation1991). In another study, Altun et al. conducted the effect of 10% methanol (M10) and ethanol (E10) blending used for a spark-ignition engine (Iliev Citation2015). They showed that adding methanol and ethanol into gasoline reduces exhaust emissions. Indeed, the HC emissions were reduced respectively by 13% and 15%, and the CO emissions were decreased by 10.6% and 9.8%. However, this caused an increase in brake-specific fuel consumption (BSFC), and thus the CO2 emissions were slightly increased.
Generally, it can be observed in the previous studies that the exhaust emissions for ethanol-gasoline blends are lower than that of original gasoline fuel (Iliev Citation2015; Iodice, Langella, and Amoresano Citation2018; Kim, Ge, and Choi Citation2020; Lin, Chang, and Hsieh Citation2010; Najafi et al. Citation2009; Turner et al. Citation2018). However, a conclusion was made that the exhaust emission and engine performance of various ethanol concentration mixtures in gasoline engines have not been investigated sufficiently. Besides, almost all previous studies considered and conducted research in automobile vehicles or internal combustion engines (Li et al. Citation2019; Roso et al. Citation2019; Tian et al. Citation2020; Wu et al. Citation2020; Yontar Citation2020a, Citation2020b, Citation2018; Zhen et al. Citation2020). Therefore, this work aims to examine ethanol-gasoline fuel blends’ effects on the test motorcycle’s engine characteristics under various wheel speeds, comparing them with those of original gasoline. Ethanol fuel blend has already been used in commercial vehicles worldwide. However, in this research, we investigated Vietnam’s conditions. Currently, the Vietnam government only allows E5 fuel (5% of ethanol in fuel). The blend with an ethanol content of more than 5% has not been approved, and customers are still suspecting the ethanol blend’s effects. This research contributes to confirming the suitable ethanol blend content with the in-used motorcycle in Vietnam.
Experimental setup
The fuel and test vehicle characteristics
This research evaluates the effects of the ethanol concentration on the selected motorcycle without modifying any vehicle component. And thus, a test carburetor motorcycle named Honda Wave Repsol was chosen in this research since it is popular in Vietnam. The properties of the test vehicle are listed in .
Table 1. Specifications of test engines and motorcycles.
The fuel supply system uses the air’s dynamic pressure to deliver fuel into the intake port as a droplet stream; generally, the evaporation process may occur at the delivery point due to the heat supply of the inlet manifold wall during the passage of the charge into the cylinder. However, the heat addition is usually limited, directly affecting the fuel evaporation quality. As described in many previous studies, the low heating value of ethanol is lower than gasoline. Therefore, to run smoothly with the ethanol-gasoline blend, the test vehicle’s fuel system must flexibly adjust the amount of supplied fuel to respond to the load conditions using the electrical fuel supply system. The carburetor supply system is very complicated to adjust the fuel quantity to adapt to the demand power and fuel evaporation quality when the fuel evaporation characteristic and latent value change due to the dual-fuel mixture effect. It is recommended that the fuel characteristics should not change significantly with the original fuel supply system. Thus, this research has prepared to achieve a maximum of 20% ethanol-blending with gasoline.
This study’s test fuels are commercial gasoline Mogas 92 and 99% ethanol in the Vietnam market. The characteristics of the test fuels are described in , including E5. E10, E15, and E20 (e.g., E5 contains 5% ethanol and 95% gasoline in volume).
Table 2. Properties of test fuels.
Experimental testing equipment
This research used the testing equipment for the experiment, including a chassis dynamometer, fuel consumption measurement, and exhaust emission measurement devices. The testbed enables vehicle weight simulation from small mopeds to heavy two-wheel vehicles ranging from 60–400 kg. A variable-speed fan was set up in front of the test vehicle to generate the airflow simulating actual operating conditions. Accordingly, the cooling fan speed was controlled corresponding to the roller speed, and at roller speeds lower than 3 km/h, the air velocity was zero. Before every experimental test, the vehicle was kept at a stable temperature between 25°C and 30°C for at least 24 hours. The speed and power can be controlled and measured at the rated power of up to 10 kW, and the maximum rated value of the speed is 120 km/h. Each experimental measurement point was taken three times and averaged to reduce the uncertainty in this research.
The fuel consumption was measured using the gravimetrical method using the fuel-consumption measurement device. It has a high-precision measurement even at the low consumed rate and rapid measuring time. The precision measurement accuracy of 0.12% was verified according to ISO9001 within a few minutes on the vehicle test by the integrated calibration unit. Regulated exhaust emissions were considered in this research, including carbon monoxide (CO), nitrogen oxides (NOx), and un-hydrocarbons (HC), particulate mass PM. The exhaust emission measurement was performed in the different analyses through a usual analytical, technical method such as nondispersive infrared for CO, chemiluminescence for NOx, and flame ionization detection for HC. All of the devices have their computer interface and were controlled directly from a computer by a dedicated. shows the schematic of the experimental system of the test vehicle assembled in the test room. The Air pollutant emissions from motorcycles in the real world are strongly influenced by the performance of the emission control system, operation mode, driving pattern, and fuel compositions. However, this research compared the vehicle fueled by different ethanol blends in the same testing conditions. Accordingly, the experiment fixed the emission control system, operation mode, driving pattern, and fuel compositions.
Figure 2. Influence of ethanol-gasoline blended fuels on the vehicle performance corresponding to: (a) gear 1, (b) gear 2, (c) gear 3, (d) gear 4.
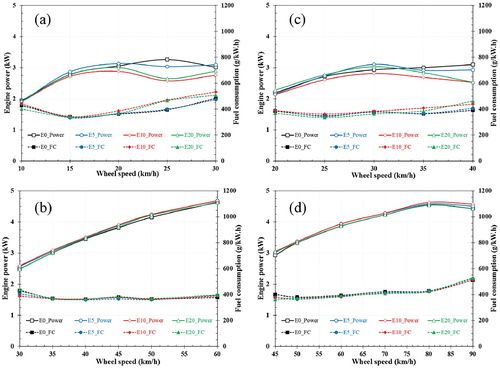
The experimental conditions were conducted according to the in-wheel vehicle speed, gear shift lever, and throttle position as showed in . Accordingly, corresponding to the shift lever 1–2 and 3–4 position, the throttle was adjusted to the 50% and full throttle position, respectively. The in-wheel speed was adjusted to vary from 10 km/h to 100 km/h by controlling the chassis dyno speed. The experimental process was repeated three times to get the average data for every test point of each fuel type of E0, E5, E10, and E20. Roller test benches were used; the motorbike was evaluated on a two-wheeler chassis dynamometer, which replicates vehicle inertia and road load resistance. The bench is configured so that it replicates road load conditions and allows for the measurement of exhaust emissions during dynamic speed cycles. It is also feasible to conduct experimental testing in constant speed, constant tractive force, and constant acceleration modes using this chassis dynamometer.
Additionally, a variable speed blower mounted in front of the car served as the road’s cooling breeze. A driver assistance system presented a speed trace of the driving cycle to be followed with a tolerance of one kilometer per hour. Each experimental test was conducted under cold-start circumstances, and the motorbike was held at a generally consistent temperature of roughly 20°C for at least 24 hours with each blended gasoline. The motorcycle’s exhaust gases were diluted with filtered ambient air during the experimental experiments using a Constant Volume Sampling with Critical Flow Venturi device. A dilution tunnel is positioned upstream of the sample for gas analysis to provide a consistent flow state. A portion of diluted exhaust was taken downstream of the dilution tunnel to continually monitor the CO and HC contents using a flame ionization detector (FID) and a non dispersive infrared (NDIR) analyzer from an exhaust gas analysis system. The signals are merely corrected for their delay with speed.
Results and discussions
Engine performance characteristic comparison
The brake power is the most crucial factor determining the engine’s performance. shows the brake power and fuel consumption variation with speed obtained at 50% and full-throttle condition for pure gasoline E0, E5, E10, E20. Generally, the ethanol content in the blended fuel affected the engine characteristics and resulted in the engine power and fuel consumption difference. The ethanol’s heat of evaporation is higher than gasoline fuel, and thus, it contributes to air-fuel charge cooling and improving the charge density. Furthermore, ethanol’s oxygen atoms join forces with the air to support ethanol burn more completely. It also helps gasoline in the blend burn better, leading to better combustion. The high-octane number of ethanol would generate greater thermal efficiency through more aggressive spark timing in thermally-critically operating conditions. However, ethanol has a lower latent heat per liter than conventional gasoline, and it can neutralize the previous positive effects causing a lower power output. The engine did not operate steadily when running at the first and second gear, especially in high-speed conditions. There was a minor difference of powers in the range from 10 km/h to 20 km/h, but it changed dramatically when the vehicle speed was over 25 km/h. Accordingly, the vehicle’s power was inversely proportional to the ethanol content in the mixture, with a maximum difference of approximately 10%.
The throttle was kept to the full position at the gear three and gear four positions. It can be observed that the engine power increased with increasing speed and reached the maximum power at the speed of 80 km/h. The test motorcycle running on E10 fuel demonstrates superior power than running with the other ethanol contents. However, the difference in power when different fuels fuel the vehicle is trivial and can only be observed at low speeds. For E20 fuel, the test power was significantly lower than other fuels at low speeds but improved as speed increased. This result can be explained by the fact that the engine temperature increases when accelerating, thereby contributing to the intake heating, reduced by ethanol evaporation. The difference in the engine power of ethanol-gasoline blends clearly showed that the greatest power loss of blend fuels compared to pure gasoline was found at 20 km/h. Still, the difference in power output of the fuels compared to gasoline was not much. E20, E10, E5 emitted 7%, 6% and 2.5% less power lower than gasoline, respectively.
Meanwhile, the engine’s fuel consumption varied significantly with speed when the throttle was held in a fixed position. Generally, it decreased with increasing speed and increased after reaching the minimum value at a certain speed of 15 km/h, 25 km/h, 50 km/h, and 60 km/h corresponding to respectively the 1st gear, 2nd gear, 3rd gear, and 4th gear. Meanwhile, the difference in fuel consumption when the vehicle was tested with different ethanol blends was slight. The most significant difference is about 3% at the low speeds of the test vehicle. At the 1st gear, the fuel consumption of the test motorcycle running with E10 and E20 was quite different from E0 and E5. This is mainly due to the vehicle running unstable at speeds higher than 20 km/h. In fact, at the 1st gear, the motorcycle typically runs at a speed of less than 15 km/h. When increasing the vehicle speed, a significant difference in the fuel consumption among fuel types can be observed, and the highest value belongs to E20 fuel because the mixture of air and fuel is rich in this stage. In addition, the oxygen content in E20 is the largest compared to the other fuels, and the calorific value of ethanol is only about 0.6 times that of gasoline. So with the same power output, E20 needs to consume more fuel than other fuels. However, oxygen atoms in the fuel support the combustion process; thus, fuel consumption is not much. Indeed, the ethanol contents provide the leaning effect to enhance the air-fuel equivalence ratio to a more excellent value and result in the burning closer to stoichiometric. As a result, improved combustion and increased power output are possible. This may be explained by the brakes’ power being proportional to speed and torque. It was evident that the addition of ethanol aids in increasing the engine’s power output.
Engine exhausts emission analysis
CO emission
In general, when the engine operates in a rich fuel mixture, the exhaust emissions include a high concentration of CO since there is insufficient oxygen to convert all of the carbon atoms to CO2. Thus, the air-fuel equivalency ratio is the most critical CO emission parameter. Even when the engine operates in stoichiometric or lean conditions, CO emission is still generated due to the lack of oxygen in some local areas or a quick-burning process. illustrates the CO emission concentration fluctuations for various fuels at various vehicle speeds. The impact of ethanol concentrations on CO emissions is expected to be reduced due to oxygen enrichment resulting from ethanol. This contribution may be seen as a pre-mixed oxygen effect enhancing the reaction’s completion. illustrates the CO emission concentration fluctuations for various fuels at various vehicle speeds. CO emissions decreased in E0, E5, E10, and E20, respectively. It can be seen the lowest CO concentration at a speed of 25 km/h reaching the lowest value of about 11,000 ppm corresponding to E20. On average, over the entire speed range, the emission reductions of E20, E10, and E5 compared to E0 were 61%, 48%, and 39%, respectively. As a result, the amount of oxygen in ethanol-gasoline blends affects CO emissions. The presence of oxygen in these fuel mixes enhances both combustion and leaning effects in rich mixtures, lowering CO exhaust emission levels. Other experiments found comparable outcomes when CO emissions were lowered by adding alcohol to gasoline. Additionally, the drop in CO emission levels may result from ethanol’s quicker flame speed, which boosts combustion efficiency.
HC emission
The leading causes of the HC formation in the exhaust are malfunctioning or inadequate combustion, flame quenching near surfaces of the combustion chamber, and the formation of deposits on internal walls of the combustion chamber that absorb fuel during the intake release fuel exhaust stroke. illustrates that HC emissions for all load/speed conditions decreased due to the ethanol content in the blend. The advantage of HC reductions with ethanol mixtures is slight at low speeds and more dramatic at high and speed conditions. As mentioned above, HC is mainly generated due to the lack of oxygen; as a result, HC emission of the vehicle when running with E0 fuel is the highest compared to other fuels, it peaked at the value of about 5600 ppm at a speed of 20 km/h and then gradually decreases. Considering the average value for the whole test speed, E20 emitted the most negligible HC emission concentration of about 2500 (ppm), corresponding to 35% lower than pure gasoline.
Figure 4. Influence of ethanol-gasoline blended fuels on the HC emissions corresponding to: (a) gear 1, (b) gear 2, (c) gear 3, (d) gear 4.
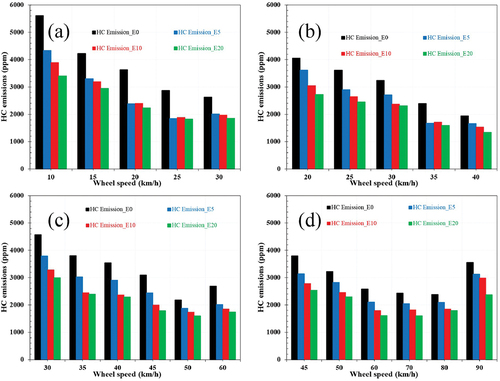
Furthermore, E10 and E5 contributed to a decrease of 29% and 27%. The HC emission reduced when engine speed increased, as shown in ). HC emission decreased as the ethanol ratio increased, owing to the high flammability limitations of ethanol and the comparatively high in-cylinder pressure and temperature produced by the high flame velocity. Furthermore, oxygen augmentation due to ethanol allows for more thorough burning, which lowers HC emissions. The decrease of HC emissions was caused by high oxygen content in alcohol and the leaning effect that improves the combustion efficiency. Additionally, since ethanol has a higher flame speed than gasoline, it may enhance the complete combustion of ethanol-gasoline blends, resulting in fewer HC exhaust emissions. Other experimental experiments have shown that adding alcohols decreases HC emissions, especially at higher engine speeds, since the air/fuel mixture becomes more homogeneous at higher engine speeds; this raises the in-cylinder temperature, increasing combustion efficiency.
NOx emission
NOx is a nitrogen oxide combination that includes a minor amount of other nitrogen oxides, nitric oxide, and nitrogen dioxide. It is affected by the air-fuel ratio, combustion chamber temperatures, and engine operating conditions. NOx is formed due to the thermal NOx, fuel NOx, and N2O intermediate processes. depicts the nitrogen oxides (NOx) emissions measured at different engine speeds. NOx emissions rose with increasing load and speed for all of the test fuels. The highest average emission of NOx corresponding to the test vehicle running on E20, E10, and E5 was respectively 67%, 47%, and 37% higher than that of E0. This trend shows that the higher the alcohol percentage in the blend, the more NOx the fuel produces. The resultant nitrogen oxides are produced in the atmosphere from nitrogen and oxygen, and the temperature is the most significant component influencing the formation. Thermal NOx generation is also a substantial process in ethanol-gasoline mixtures. The lower heating value and high ethanol heat reduce temperature, resulting in a temperature drop in the cylinder. NOx production is reduced when the adiabatic flame temperature is low. More fuel is used in this research realized at stoichiometry because of rising volumetric efficiency owing to stoichiometric rate reduction parallel with increasing ethanol rate, high vapor density, and evaporation heat.
Figure 5. Influence of ethanol-gasoline blended fuels on the NOx emissions corresponding to: (a) gear 1, (b) gear 2, (c) gear 3, (d) gear 4.
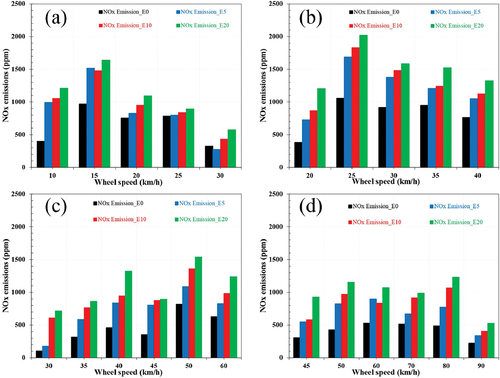
Furthermore, the thermal efficiency gained from fast flame speed raises peak pressure and temperature. Moreover, oxygen atoms have a rising impact on NOx emissions while decreasing CO and HC emissions. Since forward and reverse NOx reactions have different rate coefficients at different temperatures, NOx formations freeze early in the expansion stroke. It is estimated that combustion completed in less time due to the high flame speed allows for fewer reversible reactions, resulting in more NOx releases. As a consequence of these effects, NOx levels were inversely related to HC and CO emissions, rising with the ethanol content of the gasoline, as can be observed in .
Exhaust emissions for the test vehicle according to the Euro standard
The regulated gaseous emissions over the ECE driving cycle, an emission test cycle is a protocol contained in an emission standard were measured and evaluated in g/km. The emission rates of motorcycles for CO, NOx, HC are summarized in . NOx and HC emissions of the bike using ethanol blends are lower than gasoline. However, NOx emissions have an opposite trend; they increased approximately 7.4% with E5, 12.3% with E10, and 18.51% with E20 due to the temperature increase. The reason for reducing CO and HC emissions is the rise in the percentage of ethanol and additive concentrations due to leaner combustion due to the presence of oxygen in ethanol. Owing to the leaning, CO and HC emissions decrease tremendously. In general, CO and HC emissions are reduced when concentration increases for all concentration blends.
Conclusion
This study measured the engine performance and pollutant emissions on the ethanol–gasoline-blended fuel utilization under different loads and vehicles. The results showed that the ethanol content plays a vital role in the combustion process. Indeed, Using E10 as the fuel for the engine can improve torque output and reduce fuel consumption. With the increase of ethanol content, CO and HC emission are reduced due to oxygen enrichment coming from ethanol. Furthermore, ethanol addition increases NOx emissions; it will be helpful to consider the post-combustion emission reduction methods and performance optimization of these methods for ethanol blend operating conditions. The engine was also unstable when the ethanol concentration was higher than 20%. And thus, we selected 0%, 5%, 10%, and 20% without changing the engine’s parameters for the comparison in this research. The extension of ethanol percentage in the blend and engine modification will be conducted in future research.
Nomenclature
Disclosure statement
No potential conflict of interest was reported by the author(s).
References
- Al-Hasan, M. 2003. Effect of ethanol-unleaded gasoline blends on engine performance and exhaust emission. Energy Convers. Manag. 44 (9):1547–61. doi:https://doi.org/10.1016/S0196-8904(02)00166-8.
- Danaiah, P., P. R. Kumar, and D. V. Kumar. 2013. Effect of methanol gasoline blended fuels on the performance and emissions of SI engine. Int. J. Ambient Energy 34 (4):175–80. doi:https://doi.org/10.1080/01430750.2012.755609.
- Dinh, T., K. Nguyen, T. Pham, and V. Nguyen. 2020. Study on performance enhancement and emission reduction of used carburetor motorcycles fueled by flex-fuel gasoline-ethanol blends. J. Chinese Inst. Eng. Taylor & Francis:1–12. doi:https://doi.org/10.1080/02533839.2020.1751719.
- Dorney, D. J., H. C. Flitan, D. E. Ashpis, and W. J. Solomon. 2001. The effects of blade count on boundary layer development in a low-pressure turbine. Int. J. Turbo Jet Engines 18 (1):1–13. doi:https://doi.org/10.1515/tjj.2001.18.1.1.
- Duc, K. N., H. N. Tien, and V. N. Duy. 2018a. A study of operating characteristics of old-generation diesel engines retrofitted with turbochargers. Arab. J. Sci. Eng. 43 (9). Springer Berlin Heidelberg:4443–52. doi:https://doi.org/10.1007/s13369-017-2902-7.
- Duc, K. N., H. N. Tien, and V. N. Duy. 2018b. Performance enhancement and emission reduction of used motorcycles using flexible fuel technology. J. Energy Inst. 91 (1). Elsevier Ltd:145–52. doi:https://doi.org/10.1016/j.joei.2016.09.004.
- Duy, V. N., K. N. Duc, D. N. Cong, H. N. Xa, and T. Le Anh. 2019. Experimental study on improving performance and emission characteristics of used motorcycle fueled with ethanol by exhaust gas heating transfer system. Energy Sustain. Dev. 51. Int. Energy Initiative 56–62. doi:https://doi.org/10.1016/j.esd.2019.05.006.
- Duy, V. N., and H. M. Kim. 2020. A study of the movement, structural stability, and electrical performance for harvesting ocean kinetic energy based on IPMC material. Processes 8 (6). doi: https://doi.org/10.3390/PR8060641.
- Han, J., L. M. T. Somers, R. Cracknell, A. Joedicke, R. Wardle, and V. R. R. Mohan. 2020. Experimental investigation of ethanol/diesel dual-fuel combustion in a heavy-duty diesel engine. Fuel 275 (March). Elsevier:117867. doi:https://doi.org/10.1016/j.fuel.2020.117867.
- Iliev, S. 2015. A comparison of ethanol and methanol blending with gasoline using a 1-D engine model. Procedia Eng. 100 (January). Elsevier BV:1013–22. doi:https://doi.org/10.1016/j.proeng.2015.01.461.
- Iodice, P., G. Langella, and A. Amoresano. 2018. Ethanol in gasoline fuel blends: effect on fuel consumption and engine out emissions of SI engines in cold operating conditions. Appl. Therm. Eng. 130:1081–89. doi:https://doi.org/10.1016/j.applthermaleng.2017.11.090.
- Kim, H. Y., J. C. Ge, and N. J. Choi. 2020. Effects of ethanol-diesel on the combustion and emissions from a diesel engine at a low idle speed. Appl. Sci. 10 (12):1–15. doi:https://doi.org/10.3390/APP10124153.
- Lee, J., H.-M. Kim, T. Kim, V. N. Duy, J. Ahn, S. Park, and K. Kim. 2015. Dynamic simulations of under-rib convection-driven flow-field configurations and comparison with experiment in polymer electrolyte membrane fuel cells. J. Power Sources 293. Elsevier BV:447–57. doi:https://doi.org/10.1016/j.jpowsour.2015.05.107.
- Li, Y., X. Duan, Y. Liu, J. Liu, G. Guo, and Y. Tang. 2019. Experimental investigation the impacts of injection strategies coupled with gasoline/ethanol blend on combustion, performance and emissions characteristics of a GDI spark-ignition engine. Fuel 256 (February). Elsevier:115910. doi:https://doi.org/10.1016/j.fuel.2019.115910.
- Lin, W. Y., Y. Y. Chang, and Y. R. Hsieh. 2010. Effect of ethanol-gasoline blends on small engine generator energy efficiency and exhaust emission. J. Air Waste Manag. Assoc. 60 (2):142–48. doi:https://doi.org/10.3155/1047-3289.60.2.142.
- Najafi, G., B. Ghobadian, T. Tavakoli, D. R. Buttsworth, T. F. Yusaf, and M. Faizollahnejad. 2009. Performance and exhaust emissions of a gasoline engine with ethanol blended gasoline fuels using artificial neural network. Appl. Energy 86 (5). Elsevier Ltd:630–39. doi:https://doi.org/10.1016/j.apenergy.2008.09.017.
- Nguyen Duc, K., V. Nguyen Duy, L. Hoang-Dinh, T. Nguyen Viet, and T. Le-Anh. 2019. Performance and emission characteristics of a port fuel injected, spark ignition engine fueled by compressed natural gas. Sustain. Energy Technol. Assessments 31 (January 2018). Elsevier:383–89. doi:https://doi.org/10.1016/j.seta.2018.12.018.
- Rice, R. W., A. K. Sanyal, A. C. Elrod, and R. M. Bata. 1991. Exhaust gas emissions of butanol, ethanol, and methanol-gasoline blends. J. Eng. Gas Turbines Power 113 (3):377–81. doi:https://doi.org/10.1115/1.2906241.
- Roso, V. R., N. D. S. A. Santos, C. E. C. Alvarez, F. A. Rodrigues Filho, F. J. P. Pujatti, and R. M. Valle. 2019. Effects of mixture enleanment in combustion and emission parameters using a flex-fuel engine with ethanol and gasoline. Appl. Therm. Eng. 153 (November 2018). Elsevier:463–72. doi:https://doi.org/10.1016/j.applthermaleng.2019.03.012.
- Sahoo, B. B., N. Sahoo, and U. K. Saha. 2009. Effect of engine parameters and type of gaseous fuel on the performance of dual-fuel gas diesel engines-a critical review. Renew. Sustain. Energy Rev. 13 (6–7):1151–84. doi:https://doi.org/10.1016/j.rser.2008.08.003.
- Salek, F., M. Babaie, A. Shakeri, S. V. Hosseini, T. Bodisco, and A. Zare. 2021. Numerical study of engine performance and emissions for port injection of ammonia into a gasoline ethanol dual-fuel spark ignition engine. Appl. Sci. 11 (4):1–17. doi:https://doi.org/10.3390/app11041441.
- Tian, Z., X. Zhen, Y. Wang, D. Liu, and X. Li. 2020. Comparative study on combustion and emission characteristics of methanol, ethanol and butanol fuel in TISI engine. Fuel 259 (September 2019). Elsevier:116199. doi:https://doi.org/10.1016/j.fuel.2019.116199.
- Turner, J. W. G., A. G. J. Lewis, S. Akehurst, C. J. Brace, S. Verhelst, J. Vancoillie, L. Sileghem, F. Leach, and P. P. Edwards. 2018. Alcohol fuels for spark-ignition engines: performance, efficiency and emission effects at mid to high blend rates for binary mixtures and pure components. Proc. Inst. Mech. Eng. Part D J. Automob. Eng. 232 (1):36–56. doi:https://doi.org/10.1177/0954407017752832.
- Wu, G., D. Wu, Y. Li, L. Meng, and D. Zhou. 2020. Effect of acetone- n -butanol-ethanol (ABE) as an oxygenate on combustion, performance, and emission characteristics of a spark ignition engine. J. Chem. 2020:1–11. (x). doi:https://doi.org/10.1155/2020/7468651.
- Yontar, A. A. 2018. Numerical comparative mapping study to evaluate performance of a dual sequential spark ignition engine fuelled with ethanol and E85. Int. J. Automot. Eng. Technol. 7 (3):98–106. doi:https://doi.org/10.18245/ijaet.486405.
- Yontar, A. A. 2020a. Impact of ethanol, methyl tert-butyl ether and a gasoline–ethanol blend on the performance characteristics and hydrocarbon emissions of an opposed-piston engine. Biofuels 11 (2). Taylor & Francis:141–53. doi:https://doi.org/10.1080/17597269.2019.1661146.
- Yontar, A. A. 2020b. Effects of ethanol, methyl tert-butyl ether and gasoline-hydrogen blend on performance parameters and HC emission at Wankel engine. Biofuels 11 (3). Taylor & Francis:377–88. doi:https://doi.org/10.1080/17597269.2019.1613765.
- Zhen, X., X. Li, Y. Wang, D. Liu, and Z. Tian. 2020. Comparative study on combustion and emission characteristics of methanol/hydrogen, ethanol/hydrogen and methane/hydrogen blends in high compression ratio SI engine. Fuel 267 (December 2019). Elsevier:117193. doi:https://doi.org/10.1016/j.fuel.2020.117193.