ABSTRACT
The application of dust suppressants is an effective technique to reduce fugitive emissions, but commercially available products are costly and may harm the environment. By contrast, wastes and by-products from food production and processing can be sustainable alternatives, as they are biodegradable, considered cost-effective and have adhesive properties. The study aimed to investigate the application potential of biogenic wastes and by-products from the food industry to control dust emissions from mine soils. Unconfined compressive strength tests (UCS) were conducted on medium- to coarse-grained sand treated with sixteen biomaterials at two different additive concentrations (2 wt%, 4 wt%). UCS tests showed that rinsing water from jam production (1,366 kPa), corn steep liquor (1,502 kPa), chicory vinasses (1,723 kPa), decantation syrup (2,026 kPa) and palatinose molasses (7,535 kPa) significantly enhanced the mechanical strength of the substrate (11 kPa), indicating a strong potential of these biomaterials as dust suppressants. Such biomaterials that contained biopolymers and not only mono- and disaccharides achieved on average higher UCS values, possibly due to the formation of 3D-network structures. Moreover, the data indicated a low potential for substances with high glucose and fructose content, as they had minor or no impact on soil strength.
Implications: The UCS test results indicate that food processing wastes and by-products can be sustainable alternatives to existing dust suppressants. Hence, the present study supports an environmentally friendly and cost-effective dust control of exposed surfaces at mine and mineral processing sites and provides new markets for the food industry’s wastes and by-products. Moreover, this research extends our understanding of dust suppressant treatment of soils and contributes to evaluating biogenic food processing wastes and by-products as environmentally benign dust suppressants.
Introduction
At mine and mineral processing sites, fugitive dust emissions from open pit faces, ore stockpiles, waste rock dumps, crushers, conveyor belts, concentrate sheds, tailings surfaces and unpaved roads may contribute significantly to the natural particulate loading in the local atmosphere. Such additional particle emissions can make mining and mineral processing operations major sources of local air pollution. Significant dust emission may (a) become a nuisance; (b) reduce visibility and cause a hazard to mine personnel and especially equipment operators and drivers; (c) increase the wear and tear on moving parts of mining and processing equipment; (d) pollute nearby surface waters and topsoils; (e) lead to stunted vegetation growth by shading and clogging the pores of plants; and (f) impact on human health (Rai Citation2016; Thompson and Visser Citation2006, Citation2007; Toufigh and Ghassemi Citation2020). In particular, exposure of humans to particulate matter (PM) less than 10 and 2.5 µm (PM10 and PM2.5, respectively) can lead to respiratory and cardiovascular ailments, whereas coarse particles (>PM10) may reduce visibility on haul roads (Khan and Strand Citation2018; Rushing and Tingle Citation2007). The amount of dust emitted from rock and soil surfaces correlates with the erodibility of the exposed material (i.e. the resistance of rocks and soils to erosion) and the erosive forces to which the substrate is subjected (Foley, Cropley, and Giummarra Citation1996). A reduction in the erodibility of a substrate can be achieved by applying dust suppressants that establish linkages between single substrate particles and thus increase the resistance of the substrate to wind and traffic erosion (Thompson and Visser Citation2007). Therefore, dust suppression at mine sites commonly involves the use of water trucks to distribute water, or a mixture of water and additives to hold moisture and particles in dust emitting materials and to decrease dust emissions (Thompson and Visser Citation2002; Piechota et al., Citation2004). A diversity of dust suppressants are commercially available, yet the applied chemicals are costly and in some cases, these additives are not necessarily readily available, easily applied, efficient and effective. Furthermore, the application of some of these chemicals may harm the environment, whereby water runoff from treated surface areas may impact on soils as well as surface and groundwater quality and cause toxicity to flora and fauna (Piechota et al., Citation2004). Hence, there is a clear need to identify readily available, nontoxic chemicals that are easily applied and help to suppress dust emissions efficiently and effectively at mine and mineral processing sites.
In this context, wastes and by-products from food production and processing may be sustainable alternatives to existing dust suppression chemicals, as some of them are known to agglomerate dust particles (Jones Citation2017; Jones and Surdahl Citation2014; Omane, Liu, and Pourrahimian Citation2017). Moreover, many of these materials are biodegradable and readily available and consist of low-molecular carbohydrates, polysaccharides and proteins that have adhesive characteristics (Anal Citation2017). The dust control effectiveness of polysaccharides and proteins has already been investigated by various studies, which concluded that these materials have a distinct potential to be applied in the mining sector (Chen, Lee, and Zhang Citation2015; Ding et al. Citation2018; Gillies et al. Citation1999; Reddy et al. Citation2020). Both, polysaccharides and proteins, are biopolymers that can create three-dimensional networks structures by covalent or non-covalent bonds and have film-forming properties (Azeredo and Waldron Citation2016). Stickiness in food is often attributed to the presence of mono- and disaccharides (Adhikari et al. Citation2001). In fact, Omane, Liu, and Pourrahimian (Citation2017) were able to demonstrate a dust control performance of >99% for beet molasses, which is a by-product from the refining of sugar and primarily consists of sucrose. Therefore, it is possible that biopolymer- and sugar-rich wastes and by-products from food production and processing have the potential to bind single soil particles and reduce dust emissions.
The purpose of this study was to explore whether the use of biogenic wastes and by-products from the food production and processing influences the release of dust particles from mine soils. Material characterization and unconfined compressive strength tests (UCS) were performed on sandy soils treated with aqueous solutions of different additive concentrations. The UCS test is a suitable screening method because the UCS assessment is time- and cost-effective and correlates with wind tunnel tests (Xu et al. Citation2018). A higher UCS value indicates an enhanced wind erosion resistance, and thus an improved dust control performance (Ding et al. Citation2018). Prior to treatment, standard Proctor compaction tests were carried out to investigate the optimum water content of each soil-additive matrix to achieve maximum compaction of samples. Thus, UCS and Proctor tests were completed to evaluate: (a) the erodibility behavior of treated and untreated soils during laboratory trials; and (b) the relative effectiveness of selected biomaterials in lowering dust emissions from soils. Hence, this research adds to our understanding of dust suppressant treatment of soils and contributes to evaluating biogenic food wastes and by-products as environmentally friendly dust suppressants.
Materials and methods
Soil
The soil used in this study represents a 2,500 kg bulk sample acquired from a sand and gravel pit in North-Rhine Westphalia. This material was selected for laboratory experimentation, because: (a) mine soils and surface mine exposures of industrial mineral and opencast lignite mines in North-Rhine Westphalia (Germany) have similar grain size distributions, and (b) the sample material is readily available, enabling further studies to be carried out.
The investigated soil is medium-grained (medium-coarse sand; acc. to DIN EN ISO 14688–1:2018-05) (German Institute for Standardization Citation2018a)), and has a mean grain size (D50) of 0.65 mm with a specific gravity of 2.56 g/cm3. The particle size distribution of the soil is illustrated in . The sand has an optimum moisture content (OMC) of 9.7% and a maximum dry density (MDD) of 1.741 g/cm3. provides an overview of the physical and chemical properties of the soil sample, which were determined according to DIN, EN and ISO standards at RWTH Aachen University. Preparation of the sample for laboratory experimentation was conducted according to DIN 19747:2009–07 (German Institute for Standardization Citation2009). Soil sample homogenization and subsampling were carried out using a cement mixer and the coning and quartering technique. Representative subsamples were ground to powders using a vibratory disc mill, and an aliquot sample was subsequently analyzed by SGS Serbia using inductively coupled plasma mass spectrometry (ICP-MS) to determine the elementary composition of the soil. The combination of HCl (hydrochloric acid), HNO3 (nitric acid), HF (hydrofluoric acid) and HClO4 (perchloric acid) was applied for multi-acid (4-acid) digestion. The SiO2 content was calculated by subtracting the sum of the contents of oxides and minor and trace elements from the total content. Geochemical analysis revealed major amounts of SiO2 and minor concentrations of Al2O3, K2O, Fe2O3, Na2O, CaO, MgO, TiO2, P2O5 and MnO (). The mineralogy of the soil was identified using X-ray diffraction (XRD) at the Clausthal University of Technology (Germany). The soil is dominated by quartz, with minor amounts of orthoclase, microcline and clinochlore.
Figure 1. Particle size distribution of the soil sample (acc. to DIN EN ISO 17892–4:2017-04 (German Institute for Standardization Citation2017)).
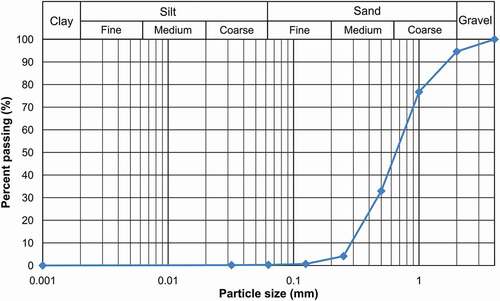
Table 1. Physical and chemical properties of the soil sample used for laboratory experimentation.
Table 2. Geochemical composition of soil sample. The soil sample was analyzed using ICP-MS techniques and multi-acid (4-acid) digestion. Note, the SiO2 content was calculated by subtracting the sum of the contents of oxides and minor and trace elements from the total content.
Food processing wastes and by-products
In the European Union (EU), approximately 16.9 Mt of food processing wastes and by-products are generated annually, with the German food industry contributing approximately 1.9 Mt (Stenmarck et al., Citation2016). In general, food production and processing generate a great diversity of wastes and by-products. Mahro et al. (Citation2015) state that most of the wastes and by-products from German food production are used as animal feedstock. In this context, the Catalog of Feed Material from the European Union (Citation2017) provides a comprehensive overview of the material flows within the food industry. This document lists feed materials that are or may be intended for feeding (European Union Citation2017). In addition, a literature survey was conducted to identify suitable food processing residuals, and companies from the food industry were contacted directly regarding their wastes and by-products and relevant properties.
Subsequently, this study completed a selection process: (a) to identify food processing wastes and by-products suitable for subsequent laboratory experimentation; and (b) to exclude substances that are not suitable for dust suppression at mine sites. Such a selection procedure was based on a number of KO-criteria that, if not fulfilled, led to the inclusion, respectively exclusion of the substances from laboratory experimentation. documents the approach and criteria to select wastes and by-products from the food processing industry for laboratory experimentation. The fundamental criterion for the use of a waste and by-product as dust suppressant is its ability to agglomerate dust particles (CRITERION 1). Such a material property is indicated by its stickiness and film-forming properties, respectively, the presence of proteins and mono-, di- and polysaccharides. Identified materials were then classified by this study into product, by-product or waste according to key provisions of Directive 2008/98/EC on waste from the European Union (Citation2012) (CRITERION 2). Following this, only wastes and by-products were selected, which are generated by German food producers, or by countries bordering the German state North-Rhine Westphalia (i.e. Belgium and Netherlands) (CRITERION 3). In addition, materials for further investigation had to have no adverse impact on the environment and be solely biodegradable and nontoxic (CRITERION 4). Also, the substance must be easy to apply at mine sites, and this criterion is fulfilled if the waste or the by-product is soluble or dispersible in water (CRITERION 5). The data required for criterion 4 and criterion 5 were provided by the identified suppliers. This study did not conduct any tests regarding the biodegradability, toxicity or water solubility/dispersibility of the substances. By contrast, economic aspects (e.g. production volume, price) were not considered in the selection process because: (a) these factors depend on local conditions (e.g. production costs, transport distance, availability); and (b) there is insufficient economic data available. As a result, sixteen substances were identified that have potential to act as dust suppressants (). provide an overview of the characteristics of the food processing wastes and by-products investigated by this study. It should be noted that food processing wastes and by-products vary in their composition depending on origin, processing conditions, processing parameters, etcetera. According to Weiss and St-Pierre (Citation2009), by-products can be classified as moderately variable, while waste materials are expected to have high variability, even if they originate from the same source. However, such variability of each material tested is not reflected within the study, possibly affecting the interpretability and generalizability of the results.
Figure 2. Flowchart showing the approach to pre-select wastes and by-products from the food processing industry for laboratory experimentation.
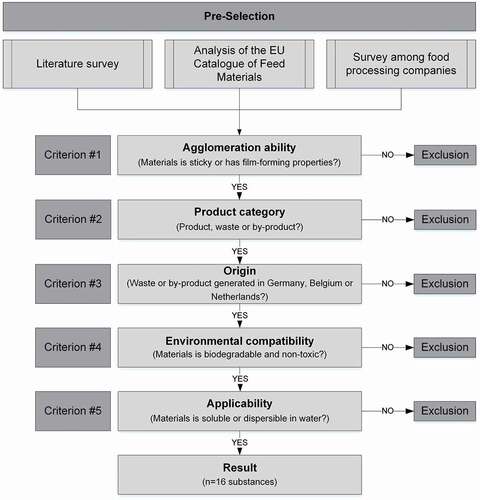
Table 3. Overview of dry matter and moisture content of the investigated food processing wastes and by-products.
Table 4. Characteristics of the studied wastes and by-products from the food processing industry (data according to supplier).
Laboratory experiments
Standard proctor compaction test
Several studies have shown that additives influence the OMC and related MDD of soils (Ding et al. Citation2018; Singh and Das Citation2020; Tingle and Santoni Citation2003). Therefore, in this study each soil-additive sample was compacted to achieve the OMC and related MDD of the tested soil-additive mixture. Proctor compaction tests were conducted for each concentration and corresponding additive according to DIN 18127:2012–09 (German Institute for Standardization Citation2012b). Such a procedure ensured that UCS tests were completed on comparable materials.
Specimen preparation for UCS testing
Each specimen was prepared following the four steps: (1) additive preparation, (2) soil-additive mixing, (3) molding and compaction, and (4) curing. For this study, two additive concentrations (2 wt% and 4 wt% by dry soil weight) were tested with three replicates for each concentration. The additive concentrations were selected because they have already been used in previous studies for similar substances and soils. Uniform concentrations were chosen due to time constraints. Water-treated soil was set up as a control group. To achieve the MDD of the soil, each specimen was compacted with the OMC.
Additive preparation
In general, by-products and waste materials of the food industry exist in both liquid and solid phases. In order to guarantee the comparability of the results, the additive concentration refers to the dry mass of the substance.
Soil-additive mixing
After calculating the required quantities of water and additive, the biomaterial was dissolved in the solvent using a magnetic stirrer. The prepared solution was then mixed into the soil in increments until a homogenous mixture was achieved.
Molding and compaction
The material was compacted in a cylindrical 10-cm-diameter x 12-cm-high standard mold (acc. to DIN 18127:2012–09 (German Institute for Standardization Citation2012b)) by a load of 2.5 kg falling 25 times. Three-layered compaction was used to achieve uniform material properties, which is critical for producing comparable results. shows the cylindrical mold and the prepared specimen.
Curing
The compacted specimen was placed in a drying oven at a temperature of 40°C for 7 days to harden the soil-additive matrix.
UCS test
A Wykeham Farrance standard triaxial system was used with a constant loading rate of 0.25 mm/min. All specimens were tested to failure, or until the maximum strain of 15% to 20% was reached (acc. to DIN EN ISO 17892–7:2018-05 (German Institute for Standardization Citation2018b)). The UCS is defined as the maximum axial compressive stress (σv) that a cylindrical sample of soil can bear under unconfined conditions DIN EN ISO 17892–7:2018-05 (German Institute for Standardization Citation2018b). For each configuration, the mean and the standard deviation were calculated.
Results
Compaction characteristics of treated soil
OMC and MDD data of each Proctor compaction test are given in . Compared to control samples, the addition of biogenic wastes and by-products reduced the OMC and increased the MDD of the soil. Only concentrated distillers solubles did not follow this trend, which increased the OMC from 9.7% to 9.8%, while the MDD increased from 1.741 g/cm3 to 1.798 g/cm3. Among the tested substances, whey permeate powder and milk permeate powder had the lowest OMC with 5.7% and 5.0%, respectively. Significant changes of the MDD were achieved by whey permeate powder and beet vinasses with a MDD of 1.845 g/cm3 and 1.847 g/cm3.
Table 5. Results of the standard proctor compaction test showing the OMC and the MDD of soils treated with additives at 2 wt% and 4 wt% concentrations (soil dry weight).
Changing additive concentrations resulted in changes of OMC and MDD values in the treated soils. With the increase of chicory molasses concentration, the OMC decreased from 7.7% to 6.3% and the MDD slightly increased from 1.744 g/cm3 to 1.754 g/cm3. By contrast, the OMC and MDD of beet molasses increased from 6.9% to 8.1%, respectively, from 1.778 g/cm3 to 1.800 g/cm3. Filter concentrate again performed differently, with an increase of the OMC from 5.9% to 6.8% and a reduction of the MDD from 1.785 g/cm3 to 1.773 g/cm3.
Effect of additive type on soil strength
presents the results of the UCS test of soils treated with 2 wt% and 4 wt% additive solutions. The studied biogenic wastes and by-products had different effects on the mechanical strength of the treated substrate. While some of the substances significantly improved the UCS of the soil, others had little or no impact on its geotechnical behavior. The control group, soil treated with pure water, showed an UCS of 11 kPa (SD = 1). Negligible improvement in the mechanical strength was achieved by glucose syrup retentate that increased the UCS to 15 kPa (SD = 5). Beet molasses, dextrose greens, beet vinasses and chicory molasses were slightly more effective, which improved the UCS to 106 kPa (SD = 49), 161 kPa (SD = 17), 225 kPa (SD = 50) and 267 kPa (SD = 66), respectively. By adding milk permeate powder, the UCS almost doubles from 327 kPa (SD = 109) to 609 kPa (SD = 102) in comparison to whey permeate powder. Apple molasses und concentrated distillers solubles showed an improved UCS of 385 kPa (SD = 80) and 457 kPa (SD = 38). Deproteinized whey concentrate and filter concentrate demonstrated a similar effect on the mechanical strength of the soil, which achieved a UCS of 1,201 kPa (SD = 1403), respectively, 1,213 kPa (SD = 274). Rinsing water from jam production, corn steep liquor, chicory vinasses and decantation syrup significantly improved the mechanical behavior of the soil and increased the UCS to 1,366 kPa (SD = 268), 1,502 kPa (SD = 109), 1,723 kPa (SD = 294) and 2,026 kPa (SD = 83), respectively. Palatinose molasses was by far the most effective in strength improvement of the soil among the tested substances with a UCS of 7,535 kPa (SD = 436).
Effect of additive concentration on soil strength
Modifying the concentration of the studied biomaterials had different effects on the mechanical strength of the soil (). An increase of the additive quantities from 2 wt% to 4 wt% resulted in both an improvement and a deterioration of the UCS. For decantation syrup, increasing its amount decreased the UCS from 2,026 kPa (SD = 83) to 1,699 kPa (SD = 221). Chicory vinasses at 4 wt% was less effective than chicory vinasses at 2 wt% that reduced the soil strength from 1,723 kPa (SD = 294) to 1,038 kPa (SD = 131). With an increase in the concentration of apple molasses, a significant reduction of the UCS from 385 kPa (SD = 80) to 47 kPa (SD = 10) was observed. Soil treated with beet vinasses and dextrose greens showed a similar behavior, with the UCS deteriorating from 225 kPa (SD = 50) to 12 kPa (SD = 1), respectively, from 161 kPa (SD = 17) to 15 kPa (SD = 2). By contrast, 4 wt% beet molasses increased the UCS from 46 kPa (SD = 32) to 106 kPa (SD = 49) compared to 2 wt% beet molasses. Milk permeate powder and whey permeate powder also showed that an increase in concentration improved the mechanical strength of the soil. Their UCS increased from 141 kPa (SD = 34) to 609 kPa (SD = 102), respectively, from 121 kPa (SD = 22) to 327 kPa (SD = 109). Soil treated with 4% filter concentrate was more effective than 2 wt% filter concentrate that improved the soil strength from 465 kPa (SD = 21) to 1,213 kPa (SD = 274). Palatinose molasses significantly improved the UCS from 1,918 kPa (SD = 308) to 7,535 kPa (SD = 436) by increasing the concentration. Increasing the amount of deproteinized whey concentrate and chicory molasses showed negligible effect on mechanical soil strength, with UCS increasing from 1,037 kPa (SD = 316) to 1,201 kPa (SD = 1403) and with UCS decreasing from 267 kPa (SD = 66) to 253 kPa (SD = 32), respectively.
Failure characteristics of treated soil
presents typical visual failure characteristics of treated specimens upon UCS testing. Soil treated with chicory molasses, apple molasses, beet molasses, beet vinasses, dextrose greens and glucose syrup retentate showed a ductile fracture behavior (). The specimens bulged in the lower and the upper third. Specimens with 4 wt% chicory vinasses and 4 wt% decantation syrup demonstrated a similar effect on the fracture behavior of the treated soil. Specimens with 2 wt% chicory vinasses, 2 wt% decantation syrup, palatinose molasses, rinsing water from jam production, corn steep liquor and concentrated distillers solubles failed under axial stress by developing slight vertical cracks mainly in the upper third of the sample body (). Despite crack formation, the specimens demonstrated high stability and were able to retain their shape while being removed from the UCS testing machine. An addition of 4 wt% whey permeate, filter concentrate and deproteinized whey concentrate led to increasing brittleness (). The specimens showed strong vertical cracks with larger fragments breaking out of the soil-additive matrix, and some samples indicated total failure. The specimens of milk permeate powder and 2 wt% whey permeate showed strong spalling in the upper third of the body, and formed either vertical cracks or failed in total (). The specimens were generally brittle and fragile.
Discussion
Compaction characteristics of treated soil
Results of the standard Proctor compaction test have shown that biogenic wastes and by-products from the food processing industry have different effects on the OMC and MDD of the investigated soil (). Effects vary with the type of additive and their applied concentration. Thus, this needs to be considered when preparing specimens for UCS test to obtain comparable results. Such findings agree with the results of Tingle and Santoni (Citation2003), who tested seven non-traditional soil stabilizers at varying concentrations and observed both an increase and a decrease of the OMC and MDD.
Moreover, the data demonstrate that the chosen additives reduced the OMC and increased the MDD of the soil. According to Proctor’s theory (Citation1933), the increase in MDD could be due to the added solution acting as a lubricant and reducing the frictional and capillary forces between soil particles. The enhanced lubrication enables the rearrangement of soil grains into a denser structure and thus increases the dry density.
Effect of additive type on soil strength
Results of this study also demonstrate that the chosen wastes and by-products increase the UCS of coarse sandy soil and improve its geotechnical behavior. The observed increase in mechanical strength is possibly a result of the added low-molecular carbohydrates, polysaccharides and proteins contained in the tested substances, which establish and enhance bonds between single soil particles through adhesive and cohesion forces. Adhesion is directly linked to molecular interactions at the interface of two phases, and thus between the soil particle and the additive. By contrast, cohesion refers to the internal strength of a material. By curing the specimen, the additive sets and achieves its cohesive strength that hardens the soil-additive matrix.
Palatinose molasses and decantation syrup achieved the highest strength improvement (). These results are unexpected, as previous studies only investigated polysaccharides and proteins as biodegradable soil stabilizers and not low-molecular carbohydrates (Ayeldeen et al. Citation2017; Blanck, Cuisinier, and Masrouri Citation2014; Chang et al. Citation2018; Soldo, Miletić, and Auad Citation2020). Palatinose molasses includes predominantly isomaltulose (α-1,6 linked) and trehalulose (α-1,1 linked) with minor constituents of sucrose (α-1,2 linked), isomaltose (α-1,6 linked), fructose and glucose (). By contrast, decantation syrup is mainly composed of sucrose, but also contains small proportions of glucose, fructose and oligosaccharides with a degree of polymerization of three ().
Glucose syrup retentate, beet molasses, dextrose greens and chicory molasses, whose dominant components (glucose, fructose and sucrose) are comparable to decantation syrup, had only a little or no impact on the geotechnical behavior of the soil (). The ductile fracture behavior of the specimens () might suggest that the mono- and disaccharides transferred predominantly into an amorphous state rather than those contained in the decantation syrup. Amorphous carbohydrates are highly hygroscopic, with the effect that they absorb moisture from the environment (Wrolstad Citation2012). Hence, it is assumed that the absorbed water decreases the cohesive strength of the additive, reducing the inter-particle bonding and increasing the plasticity of the soil. However, it is unclear why more crystalline than amorphous lattice structures were formed in the specimens treated with decantation syrup under identical conditions. Contrary to this, milk permeate powder (MPP) and whey permeate powder (WPP), rich in lactose (β-1,4 linked), produced a higher UCS but with the formation of a brittle fracture. The brittleness appears to indicate that lactose has changed into a crystalline state and not into an amorphous state. Thus, the mechanical strength of the specimen increases. However, the differences in UCS between MPP and WPP are unclear, although both have almost the same material composition (i.e. MPP: 85% lactose, 2.5% protein and max. 1% fat in DM; WPP: 84% lactose, 2.7% protein and max. 1% fat in DM ()).
As expected, protein- and polysaccharide-containing wastes and by-products significantly increased the UCS of the soil. This finding is consistent with the results of Chang et al. (Citation2015a), Ayeldeen et al. (Citation2017), and Chang et al. (Citation2018), who demonstrated that biopolymers are capable of enhancing the mechanical strength of soils. Biopolymers have the ability to form three-dimensional networks by linking polymer chains through covalent or non-covalent bonds (Azeredo and Waldron Citation2016). These network structures create bonds between the single soil particles and improve the geotechnical behavior of soils (Chen et al. Citation2016). However, the UCS test indicated a reduced UCS for those specimens treated with beet vinasses, concentrated distillers solubles and chicory molasses despite their high biopolymer content (). A possible explanation of these results could be that the contained biopolymers feature a low degree of crystallinity and cross-linking. Khatami and O’Kelly (Citation2013) state that a high degree of crystallinity and/or cross-linking contributes to high soil strength. It is assumed that the contained glucose, fructose and sucrose also effect the mechanical performance of the soil, which weaken the inter-particle bonding due to their tendency to hygroscopy (Wrolstad Citation2012). Hence, the UCS of the specimens decreases.
Effect of additive concentration on soil strength
The UCS test showed that an increase in additive concentration enhanced and reduced mechanical soil strength. Such results indicate that a particular dosage of the tested reagents leads to optimal soil strength (as established by the UCS test) and that this concentration depends on the type of additive. These findings concur with those of Blanck, Cuisinier, and Masrouri (Citation2014), who demonstrate that soil treated with 2 wt% lignosulfonate achieves a higher UCS compared to a concentration of 0.5 wt% or 5 wt%. Soldo, Miletić, and Auad (Citation2020) concluded that each biopolymer-reinforced soil has an optimum biopolymer concentration, which differs depending on the biopolymer type. Hence, this needs to be considered when using UCS values as an indicator for evaluating reagents regarding their dust control potential.
Results of this study show either reduced or enhanced UCS values with increasing additive concentration (). Such completely different responses to additive concentrations may be explained by the composition of the tested biomaterials. The chosen additives comprise mixtures of different substances with different physical and chemical properties. Thus, observed decreases in UCS values with increasing concentration might be due to changes in viscosity upon soil treatment. Also, Nair and Kannan (Citation2020) state that the UCS of biopolymer-treated soil decreases beyond the optimum concentration due to the increased viscosity, which contributes to a reduced bonding between soil particles and the stabilizer. For apple molasses, beet vinasses, chicory molasses and dextrose greens, rich in glucose, fructose and sucrose, there might be another explanation. As amorphous carbohydrates are highly hygroscopic (Wrolstad Citation2012), an increase in concentration enhances the absorption of moisture from the environment, thus weakening the bonding between the particles and decreasing the mechanical strength of the soil. However, beet molasses did not follow that trend (), whereby the UCS value increased with increasing concentration, although its primary components are hygroscopic low-molecular carbohydrates.
Comparison of food processing wastes and by-products to other biopolymers
In previous studies, various biopolymers have been investigated to enhance the mechanical strength of sandy soils, with polysaccharides being the primary focus (). By comparison, laboratory experiments of this study have revealed similar or even higher UCS values for soils treated with food processing wastes and by-products (). At a concentration of 4 wt%, palatinose molasses achieved a UCS of 7,535 kPa. Only 4 wt% xanthan gum obtained a comparable UCS of 7,087 kPa (Soldo, Miletić, and Auad Citation2020). The UCS values of rinsing water from jam production (σv: 1,366 kPa), corn steep liquor (σv: 1,502 kPa) and chicory vinasses (σv: 1,723 kPa) are in a similar range as casein and sodium caseinate with an UCS of approx. 1,550 kPa, respectively, of approximately 1,700 kPa (Fatehi et al. Citation2018). By comparison, filter concentrate, which also consists of proteins from the dairy industry, achieved a slightly reduced UCS of 1,213 kPa. Soil treated with 2 wt% decantation syrup showed a comparable mechanical strength as alginate and agar gum with an UCS of 2,044 kPa, respectively, of approximately 2,300 kPa (Chang et al. Citation2015b; Soldo, Miletić, and Auad Citation2020). However, a direct comparison of this study’s UCS test data with those of other works testing biodegradable reagents is difficult to achieve because there are considerable differences in the experimental designs. Also, Huang et al. (Citation2008) and Elkady (Citation2015) indicated that stabilizers enhance the UCS of soils by a longer curing time and an increase in curing temperature. Furthermore, selected concentration and tested soil type influence the strength improvement induced by the stabilizer, thus hindering the comparability of UCS results (Latifi et al. Citation2016; Tingle and Santoni Citation2003).
Table 6. Published UCS test results for soils treated with biopolymers.
Application potential of food processing wastes and by-products as dust suppressants
UCS test data is a widely applied evaluation indicator for dust suppressants intended for applications on exposed mine soils (Xu et al. Citation2018). A higher UCS value reflects reinforced bonding between soil particles, implying increased resistance against aerodynamic drag and lift forces. Ding et al. (Citation2018) quote that an increased UCS value indicates an enhanced dust suppression effectiveness. Consequently, palatinose molasses, decantation syrup, chicory vinasses, corn steep liquor and rinsing water from jam production have the highest application potential as dust suppressants among the tested biogenic wastes and by-products, with palatinose molasses being the most promising. By contrast, the potential of filter concentrate and deproteinized whey concentrate is considered to be lower, although both reagents achieved similar high UCS values (). The brittle failure characteristics appear to suggest that filter concentrate and deproteinized whey concentrate form inflexible and brittle crusts upon surface treatment. Such crusts are more prone to erosive forces and indicate a reduced potential for long-term dust control (Gillies et al. Citation1999). Also, a lower application potential exists for milk permeate powder and whey permeate powder, as the specimens showed brittle fracture behavior and achieved a reduced UCS value (). By comparison, the potential of apple molasses and concentrated distillers solubles might be higher, although they had a similar UCS value. The reason for this is that both reagents failed more ductile under axial stress. Hence, it can be concluded that they are more likely to form a malleable crust, which may not be as susceptible to external factors as brittle crusts.
The UCS test results indicate that glucose syrup retentate, beet molasses, dextrose greens, beet vinasses and chicory molasses have the lowest application potential, as they only slightly increased the mechanical strength of the soil. These findings partially contradict scientific publications and best practice guides that state beet molasses as a widely applied dust suppressant. (Bolander and Yamada Citation1999; Thompson and Visser Citation2007; Omane, Liu, and Pourrahimian Citation2017; Piechota et al., Citation2004). A possible explanation for this non-conformity might be the selected additive concentration, which deviates from the optimal concentration, thus falsifying the result. Moreover, food processing wastes and by-products generally show variations in their composition, possibly affecting the interpretability of the UCS test results (Weiss and St-Pierre Citation2009). Hence, future research is required to explore such variability and reevaluate reagents assessed with low application potential.
Possible applications of food processing wastes and by-products as dust suppressants at mine and mineral processing sites
This study demonstrates that food processing wastes and by-products have the potential to abate fugitive dust emissions at mine and mineral processing sites. Here, there are several application sites where the studied biomaterials can be used as dust suppressants. Possible applications include benches, bare grounds, dry tailings basins, waste rock heaps, ore stockpiles or overburden dumps. Moreover, the biomaterials could potentially be used for reducing dust emissions from unpaved roads and agricultural lands (e.g. abandoned fields).
There are generally two approaches how dust suppressants can be applied to mine soils. The additives are dissolved in water and then either applied topically, respectively, sprayed on the substrate surface or mixed into the first layer of the substrate (Edvardsson Citation2009). For barren surfaces that are primarily affected by wind erosion, a topical treatment is usually sufficient. By contrast, for accessible areas, which must withstand traffic erosion, it is recommended to incorporate the biomaterial into the aggregate (Piechota et al., Citation2004). Although the so-called mixed-in-place method entails a greater expense, it is generally more economical than topical treatments due to the increased longevity and the associated reduced re-application intervals (Jones Citation2017; Rushing and Tingle Citation2007; Thompson and Visser Citation2007). This means, however, that this method should be used primarily for areas with a long lifetime to exploit the cost advantage fully (Piechota et al., Citation2004).
Wastes and by-products from food production and processing are biodegradable and considered cost-effective. Consequently, their application as dust suppressants would reduce dust control costs and minimize the use of commercially available products that may harm the environment. Moreover, their contained carbohydrates and proteins may activate the bacterial life in soils and stimulate root activity and plant growth (Colla et al. Citation2014; Kume, Nagasawa, and Yoshii Citation2002; Wilson, Amirkhani, and Taylor Citation2018). Several studies already demonstrated that biopolymers foster seed germination and the growth of vegetation in soils (Chang et al. Citation2020, Citation2015c; Pylak, Oszust, and Frąc Citation2019). This might be of particular interest for revegetation activities of abandoned waste heaps and dry tailings basins from former mining operations. Here, inherent physical and chemical properties of the stored waste materials and processing residues may limit plant growth due to nutrient and organic matter deficiencies, low water retention, lack of texture and structure and high alkalinity (Smith and Underwood, Citation2000; Jones, Haynes, and Phillips Citation2010). Hence, the applied biomaterials would act as fertilizer and stabilize substrates (i.e. soil and seed) against erosive forces until a plant cover has been established. However, it should be noted that the application of food processing wastes and by-products as dust suppressants may also have potential adverse effects. For example, Bolander and Yamada (Citation1999) reported that sugar-based treatments could attract animals and insects at the application sites. Moreover, heavy rainfall events may leach the water-soluble biomaterials through soils into freshwater bodies. Organic matter in aquatic systems increases bacteria and other microorganisms’ growth, which reduces the availability of dissolved oxygen, thus impairing aquatic habitats and biodiversity (Ferreira et al. Citation2017). Potential hazards may also arise from the anaerobic degradation of the tested biomaterials, releasing gases such as carbon dioxide, methane or sulfur compounds. However, there is no evidence in the literature that the application of biodegradable dust suppressants (e.g. biopolymers, lignosulfonates) leads to problems with gaseous pollutants at typical application rates. Dust suppressant solutions (0.05 to 0.12 kg/L) are typically applied at application rates of 1.4 to 4.5 L/m2 (Piechota et al., Citation2004).
Limitations of the study
The study results indicate a potential of wastes and by-products from the food industry as dust suppressants intended for applications on exposed mine soils. However, these results must be interpreted with caution, and a number of limitations should be borne in mind. (1) The UCS test is an evaluation method that allows only indirect conclusions regarding the dust control performance of a reagent, and its significance depends on the type of additive to be tested (Xu et al. Citation2018). Consequently, laboratory-based wind tunnel tests need to be conducted to evaluate the actual dust control effectiveness of the tested reagents. In addition, field trials are required to establish their effectiveness under actual field conditions, indicating potential commercial-scale application. (2) Caution should be exercised when transferring the results to unpaved roads because the focus of the study was to assess the biomaterials’ potential in suppressing dust emissions from exposed mine soils, where mainly wind erosion rather than the abrasive forces of vehicles contribute to dust generation. (3) The biomaterials were tested with two uniform additive concentrations due to time constraints. However, probably each biomaterial has its optimal concentration, which may distort the results. (4) The specimens were cured in a drying oven at a temperature of 40°C for 7 days. Such curing settings do not correspond to any field applications. Environmental conditions at mine and mineral processing sites are likely to impair the effect of the tested biomaterials on soil strength. (5) The transferability of the results to other mine soils with different grain size distributions is limited because the soil type can influence the strength improvement induced by the additive (Latifi et al. Citation2016; Tingle and Santoni Citation2003).
Conclusion
This study investigated the potential of sixteen biodegradable wastes and by-products from the food processing industry to control dust emissions from sandy soils. The following conclusions can be drawn from the experimental results:
UCS tests showed that rinsing water from jam production (σv: 1,366 kPa), corn steep liquor (σv: 1,502 kPa), chicory vinasses (σv: 1,723 kPa), decantation syrup (σv: 2,026 kPa) and palatinose molasses (σv: 7,535 kPa) significantly improved the mechanical strength of the substrate (σv: 11 kPa), thus indicating a strong potential of these biomaterials as dust suppressants. Palatinose molasses and decantation syrup achieved the highest UCS values.
Protein- and polysaccharide-containing substances obtained on average higher UCS values than low-molecular carbohydrates due to the formation of three-dimensional network structures.
The data indicate a low potential for substances with high glucose and fructose content, as they had minor or no impact on soil strength.
This study demonstrates that carbohydrate- and protein-rich wastes and by-products from the food industry have the potential to be applied as dust suppressants at mine sites. Such approaches would foster environmentally benign dust control measures of large exposed areas at mine sites and provide new markets for the food processing industry for its wastes and by-products.
Acknowledgment
The authors thank Devrim Gürsel and Dr Martin Knippertz for assistance in characterizing the soil sample, and Johannes L. Sieger for commenting on the manuscript. Lastly, the authors would like to express their deep gratitude to two anonymous reviewers who helped to improve the manuscript.
Disclosure statement
No potential conflict of interest was reported by the author(s).
Data availability statement
The data that support the findings of this study are available from the corresponding author, upon reasonable request.
Additional information
Notes on contributors
Justus Freer
Justus Freer is a research associate and Ph.D. candidate at the Institute of Mineral Resources Engineering, RWTH Aachen University, Germany.
Peter G. Bucher
Peter G. Bucher is an MSc graduate from the Institute of Mineral Resources Engineering, RWTH Aachen University, Germany.
Marius Braun
Marius Braun is a senior engineer and Ph.D. candidate at the Institute of Mineral Resources Engineering, RWTH Aachen University, Germany.
Bernd G. Lottermoser
Bernd G. Lottermoser is a professor in sustainable resource extraction and director of the Institute of Mineral Resources Engineering at RWTH Aachen University, Germany.
References
- Adhikari, B., T. Howes, B. R. Bhandari, and V. Truong. 2001. Stickiness in foods: A review of mechanisms and test methods. Int. J. Food. Prop. 4 (1):1–33. doi:10.1081/JFP-100002186.
- Anal, A. K. 2017. Food processing by-products and their utilization. 1st ed. Chichester, UK: Wiley.
- Ayeldeen, M., A. Negm, M. El Sawwaf, and T. Gädda. 2017. Laboratory study of using biopolymer to reduce wind erosion. Int. J. Geotech. Eng. 12 (3):228–40. doi:10.1080/19386362.2016.1264692.
- Azeredo, H. M. C., and K. W. Waldron. 2016. Crosslinking in polysaccharide and protein films and coatings for food contact: A review. Trends Food Sci. Tech. 52:109–22. doi:10.1016/j.tifs.2016.04.008.
- Blanck, G., O. Cuisinier, and F. Masrouri. 2014. Soil treatment with organic non-traditional additives for the improvement of earthworks. Acta. Geotech. 9 (6):1111–22. doi:10.1007/s11440-013-0251-6.
- Bolander, P., and A. Yamada. 1999. Dust palliative selection and application guide. San Dimas, CA: San Dimas Technology and Development Center. 23.
- Chang, I., J. Im, A. K. Prasidhi, and G.-C. Cho. 2015a. Effects of Xanthan gum biopolymer on soil strengthening. Constr. Build. Mater. 74:65–72. doi:10.1016/j.conbuildmat.2014.10.026.
- Chang, I., A. K. Prasidhi, J. Im, and G.-C. Cho. 2015b. Soil strengthening using thermo-gelation biopolymers. Constr. Build. Mater. 77:430–38. doi:10.1016/j.conbuildmat.2014.12.116.
- Chang, I., A. K. Prasidhi, J. Im, H.-D. Shin, and G.-C. Cho. 2015c. Soil treatment using microbial biopolymers for anti-desertification purposes. Geoderma 253-254:39–47. doi:10.1016/j.geoderma.2015.04.006.
- Chang, I., J. Im, and G.-C. Cho. 2016. Geotechnical engineering behaviours of gellan gum biopolymer treated sand. Can. Geotech. J. 53 (10):1658–70. doi:10.1139/cgj-2015-0475.
- Chang, I., J. Im, M.-K. Chung, and G.-C. Cho. 2018. Bovine casein as a new soil strengthening binder from diary wastes. Constr. Build. Mater. 160:1–9. doi:10.1016/j.conbuildmat.2017.11.009.
- Chang, I., M. Lee, A. T. P. Tran, S. Lee, Y.-M. Kwon, J. Im, and G.-C. Cho. 2020. Review on biopolymer-based soil treatment (BPST) technology in geotechnical engineering practices. Transp. Geotech. 24:100385. doi:10.1016/j.trgeo.2020.100385.
- Chen, R., I. Lee, and L. Zhang. 2015. Biopolymer stabilization of mine tailings for dust control. J. Geotech. Geoenviron. Eng. 141 (2):4014100. doi:10.1061/(ASCE)GT.1943-5606.0001240.
- Chen, R., D. Ramey, E. Weiland, I. Lee, and L. Zhang. 2016. Experimental investigation on biopolymer strengthening of mine tailings. J. Geotech. Geoenviron. Eng. 142 (12):6016017. doi:10.1061/(ASCE)GT.1943-5606.0001568.
- Colla, G., Y. Rouphael, R. Canaguier, E. Svecova, and M. Cardarelli. 2014. Biostimulant action of a plant-derived protein hydrolysate produced through enzymatic hydrolysis. Front. Plant Sci. 5:448. doi:10.3389/fpls.2014.00448.
- Ding, X., G. Xu, M. Kizil, W. Zhou, and X. Guo. 2018. Lignosulfonate treating bauxite residue dust pollution: Enhancement of mechanical properties and wind erosion behaviour. Water Air Soil Pollut. 229 (7):1084. doi:10.1007/s11270-018-3876-0.
- Edvardsson, K. 2009. Gravel roads and dust suppression. Road Mater Pavement Des. 10 (3):439–69. doi:10.3166/rmpd.10.439-469.
- Elkady, T. Y. 2015. The effect of curing conditions on the unconfined compression strength of lime-treated expansive soils. Road Mater Pavement Des. 17 (1):52–69. doi:10.1080/14680629.2015.1062409.
- European Union. 2012. Guidance on the interpretation of key provisions of Directive 2008/98/EC on waste. Luxembourg, Luxemburg: Publications Office of the European Union.
- European Union. 2017. Commission Regulation (EU) 2017/1017 of 15 June 2017 amending Regulation (EU) No 68/2013 on the Catalogue of feed materials: C/2017/3980. Luxembourg, Luxemburg: Publications Office of the European Union.
- Fatehi, H., S. M. Abtahi, H. Hashemolhosseini, and S. M. Hejazi. 2018. A novel study on using protein based biopolymers in soil strengthening. Constr. Build. Mater 167:813–21. doi:10.1016/j.conbuildmat.2018.02.028.
- Ferreira, A. R. L., L. F. Sanches Fernandes, R. M. V. Cortes, and F. A. L. Pacheco. 2017. Assessing anthropogenic impacts on riverine ecosystems using nested partial least squares regression. Sci. Total Environ. 583:466–77. doi:10.1016/j.scitotenv.2017.01.106.
- Foley, G., S. Cropley, and G. Giummarra. 1996. Road dust control techniques: Evaluation of chemical suppressants’ performance: ARRB Transport Research Ltd, Vermont South, Victoria. 143.
- German Institute for Standardization. 2009. DIN 19747:2009-07. Investigation of solids - pre-treatment, preparation and processing of samples for chemical, biological and physical investigations. Berlin: Beuth.
- German Institute for Standardization. 2012a. DIN EN 15933:2012-11. Sludge, treated biowaste and soil - determination of pH. Berlin: Beuth.
- German Institute for Standardization. 2012b. DIN 18127:2012-09. Soil, investigation and testing - Proctor-test. Berlin: Beuth.
- German Institute for Standardization. 2017. DIN EN ISO 17892-4:2017-04. Geotechnical investigation and testing - laboratory testing of soil - part 4: Determination of particle size distribution. Berlin: Beuth.
- German Institute for Standardization. 2018a. DIN EN ISO 14688-1:2018-05. Geotechnical investigation and testing - identification and classification of soil - part 1: Identification and description. Berlin: Beuth.
- German Institute for Standardization. 2018b. DIN EN ISO 17892-7:2018-05. Geotechnical investigation and testing - laboratory testing of soil - part 7: Unconfined compression test. Berlin: Beuth.
- German Institute for Standardization. 2018c. DIN EN ISO 11508:2018-04. Soil quality - determination of particle density. Berlin: Beuth.
- Gillies, J. A., J. G. Watson, C. F. Rogers, D. DuBois, J. C. Chow, R. Langston, and J. Sweet. 1999. Long-term efficiencies of dust suppressants to reduce PM10 emissions from unpaved roads. J. Air Waste Manag. 49 (1):3–16. doi:10.1080/10473289.1999.10463779.
- Huang, H., B. Shi, J. Liu, and H.-T. Jiang. 2008. Experimental study on application of STW ecotypic soil stabilizer in windbreak and sand fixation. Chin. J. Geotech. Eng. 30 (12):1900–04.
- Jones, B. E. H., R. J. Haynes, and I. R. Phillips. 2010. Effect of amendment of bauxite processing sand with organic materials on its chemical, physical and microbial properties. J. Environ. Manage. 91 (11):2281–88. doi:10.1016/j.jenvman.2010.06.013.
- Jones, D., and R. Surdahl. 2014. New procedure for selecting chemical treatments for unpaved roads. Transp. Res. Rec. 2433 (1):87–99. doi:10.3141/2433-10.
- Jones, D. 2017. Guidelines for the selection, specification and application of chemical dust control and stabilization treatments on unpaved roads, 156. University of California Pavement Research Center. Davis, CA.
- Khan, R. K., and M. A. Strand. 2018. Road dust and its effect on human health: A literature review. Epidemiol Health 40:e2018013. doi:10.4178/epih.e2018013.
- Khatami, H. R., and B. C. O’Kelly. 2013. Improving mechanical properties of sand using biopolymers. J. Geotech. Geoenviron. Eng. 139 (8):87–99. doi:10.1061/(ASCE)GT.1943-5606.0000861.
- Kume, T., N. Nagasawa, and F. Yoshii. 2002. Utilization of carbohydrates by radiation processing. Radiat. Phys. Chem. 63 (3–6):625–27. doi:10.1016/S0969-806X(01)00558-8.
- Latifi, N., S. Horpibulsuk, C. L. Meehan, M. Z. A. Majid, and A. S. A. Rashid. 2016. Xanthan gum biopolymer: An eco-friendly additive for stabilization of tropical organic peat. Environ. Earth. Sci. 75 (9):2453. doi:10.1007/s12665-016-5643-0.
- Laurenti, R., Å. Moberg, and Å. Stenmarck. 2017. Calculating the pre-consumer waste footprint: A screening study of 10 selected products. Waste Manag. Res. 35 (1):65–78. Stockholm, 1 online resource. doi:10.1177/0734242X16675686.
- Mahro, B., B. Gaida, I. Schüttmann, and H. Zorn. 2015. Survey of the amount and use of biogenic residues of the German food and biotech industry. Chem. Ing. Tech. 87 (5):537–42. doi:10.1002/cite.201400023.
- Nair, L. P., and K. Kannan. 2020. Effect of biopolymers on soil strengthening. In Advances in Computer Methods and Geomechanics. IACMAG Symposium 2019, ed. A. Prashant, A. Sachan, and C. S. Desai, vol. 2. 1st ed., 2020 65–71. Singapore: Springer Singapore.
- Omane, D., W. V. Liu, and Y. Pourrahimian. 2017. Comparison of chemical suppressants under different atmospheric temperatures for the control of fugitive dust emission on mine hauls roads. Atmos. Pollut. Res. 9 (3):537–42. doi:10.1016/j.apr.2017.12.005.
- Piechota, T., J. Van Ea, J. Batista, K. Stave, and D. James. 2004. United States Environmental Protection Agency. EPA 600/ R–04/031. Potential environmental impacts of dust suppressants: Avoiding another timesbeach. Las Vegas, NV: An Expert Panel Summary.
- Proctor, R. R. 1933. Fundamental principles of soil compaction. Eng. News-Record 111 (13):245–48.
- Pylak, M., K. Oszust, and M. Frąc. 2019. Review report on the role of bioproducts, biopreparations, biostimulants and microbial inoculants in organic production of fruit. Rev. Environ. Sci. Biotechnol. 18 (3):597–616. doi:10.1007/s11157-019-09500-5.
- Rai, P. K. 2016. Impacts of particulate matter pollution on plants: Implications for environmental biomonitoring. Ecotoxicol. Environ. Saf. 129:120–36. doi:10.1016/j.ecoenv.2016.03.012.
- Reddy, N. G., R. S. Nongmaithem, D. Basu, and B. H. Rao. 2020. Application of biopolymers for improving the strength characteristics of red mud waste. Environ. Geotech. 1–20. doi:10.1680/jenge.19.00018.
- Rushing, J. F., and J. S. Tingle. 2007. Evaluation of products and application procedures for mitigating dust in temperate climates. Transp. Res. Rec. 1989-1 (1):305–11. doi:10.3141/1989-36.
- Singh, S. P., and R. Das. 2020. Geo-engineering properties of expansive soil treated with xanthan gum biopolymer. Geomech. Geoengin. 15 (2):107–22. doi:10.1080/17486025.2019.1632495.
- Smith, F. W., and B. Underwood. 2000. Mine closure: The environmental challenge. Min. Technol. 109 (3):202–09. doi:10.1179/mnt.2000.109.3.202.
- Soldo, A., M. Miletić, and M. L. Auad. 2020. Biopolymers as a sustainable solution for the enhancement of soil mechanical properties. Sci. Rep. 10 (1):195. doi:10.1038/s41598-019-57135-x.
- Stenmarck, Å., C. Jensen, T. Quested, and G. Moates. 2016. Estimates of European food waste levels: IVL Swedish Environmental Research Institute. Stockholm.
- Thompson, R. J., and A. T. Visser. 2002. Benchmarking and management of fugitive dust emissions from surface-mine haul roads. Min. Technol. 111 (1):28–34. doi:10.1179/mnt.2002.111.1.28.
- Thompson, R. J., and A. T. Visser. 2006. Selection and maintenance of mine haul road wearing course materials. Min. Technol. 115 (4):140–53. doi:10.1179/174328606X155138.
- Thompson, R. J., and A. T. Visser. 2007. Selection, performance and economic evaluation of dust palliatives on surface mine haul roads. J. S. Afr. I. Min. Metall. 107:435–50.
- Tingle, J. S., and R. L. Santoni. 2003. Stabilization of clay soils with nontraditional additives. Transp. Res. Rec. 1819 (1):72–84. doi:10.3141/1819b-10.
- Toufigh, V., and P. Ghassemi. 2020. Control and stabilization of fugitive dust: Using eco-friendly and sustainable materials. Int. J. Geomech. 20 (9):4020140. doi:10.1061/(asce)gm.1943-5622.0001762.
- Weiss, W. P., and N. R. St-Pierre. 2009. Impact and management of variability in feed and diet composition. In Tri-State Dairy Nutrition Conference Proceedings, Ed. 83–96. Michigan State University. Michigan State University.
- Wilson, H. T., M. Amirkhani, and A. G. Taylor. 2018. Evaluation of gelatin as a biostimulant seed treatment to improve plant performance. Front. Plant Sci. 9:1006. doi:10.3389/fpls.2018.01006.
- Wrolstad, R. E. 2012. Food carbohydrate chemistry. UK: Wiley. West Sussex.
- Xu, G., X. Ding, M. Kuruppu, W. Zhou, and W. Biswas. 2018. Research and application of non-traditional chemical stabilizers on bauxite residue (red sand) dust control: A review. Sci. Total Environ. 616-617:1552–65. doi:10.1016/j.scitotenv.2017.10.158.
- Yang, Q., C. Zheng, and J. Huang, 2018. Curing of sand stabilized with alkali lignin. In Proceedings of GeoShanghai 2018 International Conference. Ground Improvement and Geosynthetics, Ed. L. Li, B. Cetin, and X. Yang, 157–68. Singapore:Springer Singapore.