ABSTRACT
Boilers are combustion devices that provide process heat and are integral to many industrial facilities. Historically, outside of the pulp and paper industry, most boilers burned fossil fuels, although interest in decarbonization has been leading to an increased use of renewable fuels in boilers. These boilers, including those in the biorefineries, are often large sources of air pollutant emissions, and the characterization of these emissions is critical to obtaining air permits and ensuring protection of the surrounding air quality. Several industrial boilers and new biorefineries allow utilization of biomass-derived fuels (e.g. wastewater sludge, lignin, etc.) produced during their operation as a fuel for the boiler to meet process energy needs. However, there is limited empirical data on emission factors for the burning of unconventional fuels, such as solid-gas mixtures containing biomass residues. To fill this gap, we carry out a comprehensive data survey, collecting information on emission factors for boilers burning either a single or a mixture of solid and gaseous biomass-derived fuels. We review multiple hard-to-obtain and unconventional data sources, such as air permit applications, stack test data, and industry-sponsored data collection efforts, to compile emission factors for biomass-derived fuels. We then compare this data with wood residue emission factors from the U.S. Environmental Protection Agency’s AP-42 emission factor database. Our results indicate that the emission factors for boilers burning unconventional fuels vary widely depending on the fuel composition, boiler type, and fuel characteristics. Overall, we find that median emission factors of selected biomass-derived fuels are typically lower than those of wood residue boilers in AP-42. The information collected herein could be useful to permitting agencies and industries utilizing boilers who may want to reduce the carbon impact of their facilities by combusting biomass-derived wastes for process energy needs, for more accurate emission estimation for permitting.
Implications: Emission factors are often used for air permitting, specifically for emission estimation purposes. This study carries out a comprehensive data survey of emission factors burning unconventional biomass-derived fuels from underutilized sources such as air permits, stack test data, and industry-led efforts, and compare the results to EPA’s wood residue emission factor database. The results from this study can be used can be used by multiple stakeholders such as air permitting agencies, industries burning biomass-derived fuels, and biorefineries, that utilize more advanced boiler technologies. The findings can help mitigate risks to industry owners and operators and helps to avoid delays in obtaining the required air permits that arise due to inappropriate emission estimates in permit applications.
Introduction
The industrial sector uses energy in a number of ways, including (1) raising the temperature of materials in the manufacturing process, i.e., process heating (43% share of total energy use), and (2) feeding a boiler that generates steam or hot water (15% share of total energy use) (Office of Energy Efficiency and Renewable Energy Citation2018). Boilers are typically an essential operation, converting water into steam at desired pressure and temperature conditions (Reynolds Citation1961). This steam is utilized to fulfill the heat demands of the industrial plant, as a fuel to power turbines for electricity generation, or to power the plant’s heating and cooling units to maintain proper operating temperatures (Allen’s Tristate Mechanical Citation2017). Boilers are used in power plants and industrial facilities, including chemical industries, petroleum refineries, wastewater treatment plants, and pulp and paper industry (Nielsen et al. Citation2000; Singh et al. Citation2018). The common types of fuels utilized in industrial boilers include coal, oil, and natural gas. Although the use of fossil-derived fuels in industrial applications is common practice, they contribute to criteria and hazardous air pollutants that impact air quality and human health (Carreras-Sospedra, Williams, and Dabdub Citation2016; Meyer and Leung Citation2018; Tucki et al. Citation2020).
Although most industrial boilers utilize fossil-based fuels, some industries, like pulp and paper, utilize alternative biomass-based fuels such as black liquor (a waste product from the chemical pulping process) along with bark, wood chips, and production wastes (Energy and Environmental Analysis, Inc. Citation2005). Biorefineries, which produce biofuels from biomass, also have opportunities to utilize their renewable waste streams as boiler fuels to meet their heat and power needs in an energy self-sufficient manner. For example, biorefineries using biochemical conversion processes yield several biomass-derived waste streams, including lignin, biogas, sludge, and off-gases, which can be used as fuels in boilers. Research has shown that biomass fuels from wood and plant residues are important sources of primary energy, accounting for about 13% of global fuel consumption (Slade, Bauen, and Gross Citation2014).
The most common type of boiler commercially utilized for biomass combustion is a fluidized bed boiler (Anthony Citation1995; Singh et al. Citation2018), with stoker-fired boilers, originally utilized for coal and wood combustion, also being used for biomass combustion as well. Through thermodegradation and oxidation reactions, burning biomass results in emissions of several criteria and toxic air pollutants (Ushakova et al. Citation2017). These pollutants include carbon monoxide (CO), nitrogen oxides (NOx), sulfur oxides (SOx), hydrocarbons/volatile organic compounds (VOCs), and particulate matter (PM; PM includes filterable PM [PMf] that can be captured on a filter, and condensable PM [PMc], which is in the gas phase when exiting the stack, but rapidly condenses to form submicron particles once exposed to atmospheric conditions). CO emissions are mainly from incomplete combustion of biomass fuels. NOx emissions are predominantly from nitrogen in the fuel (Mitchell et al. Citation2016), referred to as fuel-bound NOx, but a smaller portion comes from thermal NOx, which produces NOx from nitrogen in the atmosphere reacting within the flame. SO2 emissions from biomass combustion result from sulfur in the fuel, whereas PM is a result of incomplete combustion, the presence of inert material in the fuel, and an improper air/fuel ratio (Jenkins et al. Citation1998).
Because boilers can be the largest emission sources for certain pollutants within biorefineries (e.g., boilers contribute the largest fraction of some gaseous pollutants, and PM emissions have been attributed to boilers in some studies), it is important to characterize their emissions and develop control strategies to protect air quality when building a new biorefinery with an on-site boiler. To maintain compliance with the National Ambient Air Quality Standards (NAAQS) (EPA Citation1970), the U.S. Environmental Protection Agency (EPA) sets emission standards for new and modified stationary pollution sources and/or unit operations, such as boilers, under the New Source Performance Standards (NSPS) and National Emission Standards for Hazardous Air Pollutants (NESHAP) in the form of emission limits (Federal Register Citation2017; Federal Register, NSPS Citation2023). The emission limits in the federal standards control the emissions of pollutants, generally defined as a parameter that relates the quantity of a pollutant with the activity associated with release of that pollutant, also known as the emission factor (EPA Citation2022). In the case of boilers, burning fuel is considered an activity whose emission factor is expressed as the weight of the pollutant (pounds, kilograms, etc.) divided by the heat input capacity of the boiler (British thermal units or standard cubic feet).
Although emission factors have been used in preparing the national and regional emissions inventory and designing emission control strategies, they are often also used for other purposes, such as air permitting. The current data and guidelines published in EPA’s emission factor database (AP-42) address emission factors for boilers burning coal, natural gas, fuel oil, wood, and wood residues (EPA Citation2009). These emission factors typically represent average emissions based on emission testing and monitoring of data collected during normal operating conditions. Many of the data sources used to calculate the emission factors reported in the AP-42 database were collected 20+ years ago and may not represent the more advanced boiler technologies developed for newly emerging, unconventional fuels (EPA Citation2009, Citation2015). Additionally, in some cases (e.g., for boilers using wood residue), the emission factors have low reliability ratings. For example, biorefineries can now utilize biomass waste produced on-site as boiler fuel(s); the existing AP-42 emission factors for wood and wood residues are likely inaccurate for these boilers.
Factors affecting the types and magnitudes of emissions from the boiler include fuel type (e.g., gas, biomass, coal, or a mixture), type of boiler (fluidized bed, stoker, etc.), fuel properties (such as sulfur, nitrogen, ash, and moisture content), and firing practices employed by the facility. For example, a biorefinery that utilizes biomass as a feedstock may pretreat the feedstock using sulfuric acid and ammonia to break down the cellulose for further processing (Davis et al. Citation2022), resulting in much higher levels of nitrogen and sulfuric acid in the boiler fuel downstream than the typical woody biomass. The emission factors of combusting biomass could be considerably different than those of combusting solid fossil fuels such as coal. This is due to biomass’ higher heterogeneous particle size, higher volatility, higher moisture content and oxygen level, and lower sulfur content and energy density. Moreover, the transport-limited devolatilization characteristics of biomass particles could affect carbon conversion, heat release, pollutant generation, ash formation (from silicon, potassium, etc.), and deposition, among others (Panagiotis Citation2010).
Previous research has investigated emission factors for boilers that combust a mixture of coal and biofuels (Cao et al. Citation2008; Cheng et al. Citation2018; Jetter and Kariher Citation2009; Jetter et al. Citation2012), but there is a lack of empirical data on emission factors for boilers that burn unconventional biomass-derived fuels. Such an information gap could pose risks to industry owners (specifically biorefineries) and operators, as they may encounter delays in obtaining the required permits prior to construction and operation due to inappropriate emission estimates in their permit applications.
The goal of this study is to carry out a comprehensive survey to identify available information on empirical emission factors for boilers that burn one or a mixture of biomass-derived fuels. We use several existing sources, including little-known/underutilized sources such as permits/permit applications, stack testing, and industry-sponsored collection efforts. The information collected and analyzed can be useful to a broad audience, including federal and state air quality planners, permit writers, industrial facilities utilizing biomass-derived waste fuels, and biofuel industries and investors, who may have a keen interest in utilizing biomass-derived fuels as a source of process energy. Our research aims to leverage previous research that is less known to stakeholders, who could benefit from accessing such information. Although our review does not focus on each individual factor mentioned, the reviewed data implicitly incorporates the variability introduced by these factors.
Methodology
Approaches for emission estimation
Emissions inventories are important for air quality management and are utilized by local, state, and federal agencies as well as industries and consultants for projecting air quality impacts. Developing an emissions inventory is essential for supporting the development of air permit applications, determining the permitting requirements for a new source or modification of an existing source, projecting future air quality impacts, characterizing pollution transport, and developing emission control strategies (Bhatt et al. Citation2022; Komariah et al. Citation2013). Emissions (typically expressed in tons per year) can be calculated or estimated by one or a combination of the several different approaches (no particular order of importance), including:
Emission measurement based on continuous emission monitoring systems
Emission data from performance tests (e.g., by boiler manufacturers, chemical kinetic data from combustion labs, industries using boilers)
Material balance analysis (e.g., amount of sulfur or nitrogen in the feed and product streams)
Emission factors (e.g., EPA’s AP-42 compilation of emission factors (EPA Citation1995).
In the absence of emission measurements and data from performance tests, emissions from boilers are often estimated based on emission factors (approach d). An emission factor for a boiler is typically expressed as pounds (lbs) of pollutant per million British thermal units (MMBtu) of the boiler heat input capacity (Eberle et al. Citation2017; Komariah et al. Citation2013). EPA has compiled emission factors for approximately 200 emission source categories, which are available in the Compilation of Air Pollutant Emissions Factors (AP-42) database. AP-42 emission factors are developed and compiled using data from multiple source tests, material balance studies, and engineering estimates. The most relevant emission factors for burning biomass fuels in a boiler are those in AP-42 Chapter 1.6: “Wood Residue Combustion in Boilers.” The database provides data quality ratings ranging from A to E (with A being the best) for emission factors to indicate the quality and robustness of the data. A rating of D is assigned when the emission factors are developed based on a small number of facilities, and thus are not necessarily representative of the industry, or when there is evidence of variability within the source population. In the case of wood residue combustion, the emission factors were last updated in 2003, and the ratings for the pollutants range from A to D, depending on the type of feedstocks in the industry population. Ratings for several pollutants are poor (C or D), suggesting relatively high uncertainties in the estimates.
Over the last two decades, continued efficiency improvements in industrial boilers have likely led to decreased emission factors (Bowman et al. Citation2009). The efficiency improvement could be due to several efforts, including boiler design that takes fuel parameters (moisture, density, size) into consideration to maximize combustion efficiency as well as boiler optimization and tuning (through monitoring of temperature, automatic adjustment of air-to-fuel ratios, and fuel feed rate adjustment) (ACHR News Citation2016; Bases Citation2011; Industrial Controls Citation2021).
Emission factor data sources
We focus on emission factors for six criteria air pollutants (NOx, SO2, CO, VOC, Pb, and PM2.5/PM10) as well as selected hazardous air pollutants (HAPs; including acetaldehyde, formaldehyde, and two acid gases – hydrochloric acid [HCl] and hydrogen fluoride [HF]), which are expected to be the major contributors to total HAP emissions. Because the emission factors from Chapter 1.6 of the AP-42 database are mainly representative of various wood residues burned in the form of hogged wood, bark, sawdust, shavings, chips, mill rejects, sanderdust, or wood trim, they may not accurately represent emissions from boilers burning unconventional biomass fuels. So, our review starts by analyzing unconventional literature sources, such as technical reports, industry-sponsored surveys, permits and permit applications, and stack testing, that do not appear in typical online search engines and bibliographic databases. Where appropriate, we use a material balance approach to estimate emission factors for certain pollutants. We then compare the emission factors collected from these data sources with those for wood residue combustion in boilers in AP-42.
We made the following efforts to identify emission data from boilers that burn unconventional fuels.
We identified specific operational boilers that burn unconventional biomass-derived fuel(s) such as sludge, lignin, biogas, off-gases from the plant, etc. and contacted the facilities and the respective state regulatory agencies for air permits and permit applications. Air permits contain detailed information on the emission factors, boiler fuel, and boiler characteristics, which makes them a preferred source of data when information is not available publicly.
We also contacted regulatory agencies for stack test data tied to boilers that burn unconventional biomass fuels. Stack test data is one of the most preferred data sources for estimating emission factors; however, detailed information on control efficiencies, stream characteristics, and operating capacity of the boiler at the time of testing is required for estimating the uncontrolled emission factors, which is not always available in the stack testing reports.
We reviewed publications on emissions from wood combusting boilers, including data collected by the National Council for Air and Stream Improvement (NCASI), an independent research organization established by a consortium of pulp and paper industry members in North America. The data on emission factors from NCASI includes detailed information on boiler feed and composition along with boiler type, which makes it a useful source for characterizing data.
We analyzed the Compliance and Emissions Data Reporting Interface (CEDRI), a U.S. EPA program designed to manage and collect environmental data submitted to the agency to meet various reporting requirements, for relevant emission factors.
We connected with Consortium for Research on Renewable Industry Materials (CORRIM), a research organization that focuses on developing life cycle assessment-related data specific to the wood industry. CORRIM conducted a survey and collected data from 16 different wood mills located in the northwest and southeast regions of the United States, of which data for 12 mills contained emissions of aerosol and gaseous pollutants we report in this paper.
Results and discussion
We analyzed the emission data from each source obtained, which includes boilers burning either a single biomass fuel or a mixture of solid and gaseous biomass fuels. The statistics show a varying range of values depending on the fuel mixture, process technology utilized, variance in time when the data was reported, and other process parameters. summarizes the data obtained from our analysis as compared to EPA’s AP-42 emission factor data. A total of five data sources for emission factors are reported. We also include compliance or permit limits specified in permit applications or stack test data when available.
Table 1. Summary of data sources obtained in this research for biomass boiler combustion as compared to AP-42 for wood residue combustion. Timeframe refers to the time when the data were collected, not the years in which the boiler was set up.
To ensure consistency, we report uncontrolled emission factors for all data sources. In cases where the original data sources do not specify the emission reduction efficiencies, thereby preventing us from back calculating the uncontrolled emission factors, we use the average emission reduction efficiencies for the specific control technologies reported in EPA’s factsheet to estimate the uncontrolled emission factors. Also, we only report certain air toxics (HAPs) because the emission factors for other hazardous pollutants were several orders of magnitude lower and thus, through engineering judgment, considered to be insignificant. We exclude emission data that (1) utilizes fuels containing a large portion of conventional fuels, such as coal or natural gas; and/or (2) does not provide sufficient information on emission controls or boiler heat input capacity to allow us to estimate emission factors. Details on other data sources and air toxics not included in the main paper are in the Supplementary Information (SI). The following subsections describe the detailed results for all the sources considered.
Stack test data
We obtained stack test data on several air pollutants from boilers burning a mixture of solid biomass and gaseous fuel at two facilities: (a) POET Biorefining (Dutch State Mines [DSM] Advanced Biofuels Project Liberty) in Emmetsburg, Iowa, and (b) Wisconsin Electric (We) Energies Biomass Cogeneration facility in Madison, Wisconsin. Biomass Cogeneration facilities produce heat and electricity using biomass wastes. We use the emissions (pounds per hour [lb/hr]) reported in the stack test data and heat input capacities, when available, to estimate the emission factors in lb/MMBtu.
POET Biorefining (DSM Advanced Biofuels Project Liberty)
The POET facility utilizes a solid fuel boiler rated at 290 MMBtu/hr (operated at 267 MMBtu/hr) that burns a mixture of biomass, biogas, digester sludge, filter cake, syrup, biomass fines, and net wrap. The emissions of PM, NOx, and SO2 from the boiler are controlled using baghouse, selective noncatalytic reduction (SNCR) and flue gas desulfurization (FGD), respectively. The stack test data covers the years 2015, 2016, 2017, and 2019, with information on fixed boiler nameplate capacity but different operating boiler heat input capacities (ARI Environmental, Inc. Citation2015, Citation2016, Citation2017; Montrose Air Quality Services, LLC. Citation2019).
We Energies Biomass Cogeneration Facility
We Energies is a 50-MW biomass-fueled cogeneration facility located next to a paper mill facility in Milwaukee, Wisconsin. The boiler is a circulating fluidized bed boiler rated to operate at 800 MMBtu/hr that burns a mixture of logging residue, wood, bark, wood waste, forest residues, and wastewater sludge. Natural gas is only used for startup. The emissions of PM and NOx from the boiler are controlled using baghouse and SNCR, respectively. We obtained the data on the recent stack testing performed for the facility in 2019 (Wisconsin Department of Natural Resources Citation2019a).
Summary of emission factors from stack test data
summarizes the operating information on type of feed, operating capacity, control technologies, and year of air permit (refer to SI for detailed information). shows the emission factors reported from stack test data sources described above.
Figure 1. Summary of emission factors for aerosol, gaseous, and hazardous air pollutants from stack testing data.

Table 2. Summary of operating information from stack test data sources.
As shown in , the stack-test-derived emission factors for the POET Biorefining pollutants are higher than those for We Energies, except for NOx and CO in 2017 and 2019, where POET has lower emission factors. POET does not report any emissions data for PM10, PM2.5, and HF. The emission factors for PMf, PM10, and PM2.5 are estimated to be similar for We Energies and closely match the data reported in AP-42 (0.5 lb/MMBtu and 0.43 lb/MMBtu for PM10 and PM2.5, respectively). Because POET Biorefining used a technology in which biomass goes through a series of pretreatment steps (utilizing several chemicals to break cell walls so that basic sugar components can be extracted for the downstream fermentation process), it is likely that many unwanted components (e.g., chemicals, enzyme residues) were added to the fuel streams fed to the boiler, increasing emissions. For POET, the emission factors reported for PM, NOx, and CO vary year to year demonstrating wide ranges (note that emission factors for some years are not available from the data source), whereas emission factors for SO2 and VOC are more consistent, ranging from 0.48–0.6 lb/MMBtu and 0.013–0.019 lb/MMBtu, respectively. One possibility is that the variation in fuel compositions could lead to changes in combustion performance and conditions across different years.
Air permits
We obtained air permit applications and construction permits for two facilities burning biomass fuels in their boilers: Abengoa Bioenergy Biomass of Kansas (ABBK) and We Energies Biomass Cogeneration Facility. Given that the information on emission controls, boiler feed, and boiler heat input capacity is described in detail in the air permits and application, we use the uncontrolled emission factors directly when explicitly reported.
Abengoa Bioenergy Biomass of Kansas (ABBK)
ABBK (not operational as of 2020) was intended to convert biomass to ethanol with a 22-MW cogeneration process that included a biomass boiler rated at 500 MMBtu/hr burning a mixture of lignin, hydrolysis residual stillage syrup, biomass, biogas, and sludge. The application for an air permit to construct was submitted in 2011 (WLA Consulting, Inc. Citation2011) and was amended in 2014 for the construction of four emergency engines as a prevention of significant deterioration (PSD)Footnote1 permit (Burns & McDonnell Citation2014). The air permit application includes two sets of emission factors for the biomass-fired stoker boiler, one for a nominal typical scenario and another for a maximum worst-case scenario, based on varying blended fuel composition depending on the plant’s steam demand. A nominal typical fuel blend consists of 41.6% lignin-rich stillage, 27.2% ethanol thin stillage syrup, 24.0% corn stover, 6.8% biogas, and 0.40% wastewater sludge, based on dry ton per day feed rates. The emissions of PM and NOx from the boiler were reduced by using baghouse and SNCR, respectively. A scrubber was used to reduce emissions of SO2, VOC, and sulfuric acid. ABBK does not have any stack test data reported in the public domain.
We Energies Biomass Cogeneration Facility
As stated in “Stack test data” Section, the We Energies Biomass Cogeneration Facility operates an 800-MMBtu/hr circulating fluidized bed boiler. The permit application (Wisconsin Department of Natural Resources Citation2019b) from 2019 includes controlled emission factors, which we use, along with average destruction efficiencies for emission controls from EPA’s factsheets, to calculate the uncontrolled emission factors (EPA Citation2016).
Summary of emission factors from air permits
summarizes the operating information on type of feed, operating capacity, control technologies, and year of air permit (refer to SI for detailed information). shows the emission factors reported in ABBK’s and We Energies’ air permit applications.
Figure 2. Emission factors used for air permits and air permit applications for selected pollutants.
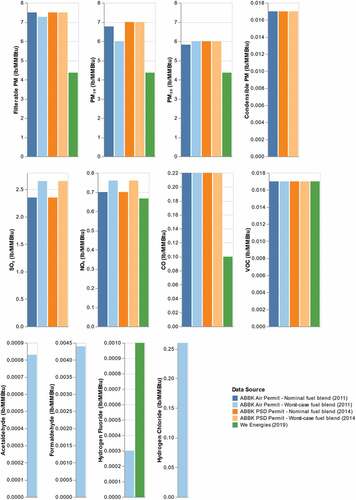
Table 3. Summary of operating information from air permit data sources.
As shown in , the emission factors estimated for ABBK are higher than those for We Energies Cogeneration facility for all the pollutants except HF. The pretreatment chemicals used in ABBK that break down cellulose and hemicellulose in the biomass add nitrogen and sulfur downstream of the process (e.g., in the boiler feed), likely increasing the emission rate of NOx and SO2. In ABBK’s permit applications, the emission factors for the maximum worst-case fuel blend include a 20% safety buffer (on a mass basis) applied to the nominal residual components (ethanol lignin-rich stillage and ethanol thin stillage syrup) used as the boiler fuel. This increase in residual components changes the blended biomass fuel composition, leading to a decrease in the overall ash content in the blended biomass and thus lower PM emission factors than the worst-case scenario. The HAP emission factors for ABBK are taken from EPA’s Chapter 1.6: “Wood Residue Combustion in Boilers,” whereas We Energies’ permit only lists an HF emission factor, which is higher than that reported by ABBK.
NCASI technical reports
NCASI is an organization that supports environmental science and data to promote the sustainable use of resources and has technical expertise in developing emission factors for the forest product industry, including wood products manufacturing and forest management (NCASI Citation2023). The technical reports and white papers published by NCASI provide a wealth of information on emission factors related to wood and forest residue combustion. NCASI reports the average emission factors based on statistical analysis NCASI performed using data from several boiler emission sources including EPA’s emission limits as well as stack tests conducted by boiler facilities. These emission factors reported by NCASI depend on the sources they considered for their analysis, as reported in the next subsections. Because EPA is a member of NCASI, their reports have been made available to the public in EPA libraries. After screening the full catalog of NCASI reports available in EPA libraries, we identified and reviewed three NCASI technical reports relevant to biomass combustion and analyzed the data on emission factors for boilers that burn unconventional biomass fuels. Below subsections provide an overview of each NCASI technical bulletin, followed by a summary in Section 4.3.4.
Comprehensive compilation and review of wood-fired boiler emissions (Technical bulletin no. 1013)
NCASI technical bulletin no. 1013 summarizes emission factors developed from statistical analysis of stack test data and associated laboratory reports (NCASI Citation2013). The majority of data was drawn from (1) EPA-mandated major source boiler maximum available control technology (MACT) and commercial and institutional solid waste incineration units (CISWI) testing conducted in 2009 and 2010, (2) testing conducted by individual facilities from 2009 to 2012, and (3) some pre-2009 emission factor data not previously available in the technical bulletins. The emission factors are believed to best characterize pollutant emissions from industrial wood-fired boilers operating in the 2009–2012 timeframe and can be considered to be replacements of the existing emission factors in EPA’s AP-42 Chapter 1.6. The data utilized to develop the emission factors include boilers burning biomass fuels or combinations of biomass and fossil fuels. The biomass fuels include bark, hog fuel, wood chips, sawdust, sanderdust, and planer shavings, which are similar to the biomass fuels represented in EPA’s AP-42 Chapter 1.6: “Wood Residue Combustion in Boilers.”
Comprehensive compilation and review of combination wood-fired boiler emissions (Technical bulletin no. 1026)
NCASI technical bulletin no. 1026 summarizes emission factors for boilers that burn wood in conjunction with at least one other nontraditional fuel, such as tire-derived fuel, petroleum coke, dewatered pulp mill sludge, or old corrugated container recycling rejects (NCASI Citation2015). The bulletin contains information on emission factors from individual facilities’ stack testing data. From this bulletin, we chose to analyze the relevant emission factors for five facilities that either burn 100% nontraditional fuel (e.g., sludge) or burn wood in conjunction with other fuels in a boiler. These boilers include:
A fluidized bed boiler (78.7 MMBtu/hr) burning 100% wastepaper sludge, equipped with baghouse and SNCR controls.
A stoker boiler (160.4 MMBtu/hr) burning 39%–59% bark (mass basis), with the remaining fuel being deinking sludge and mechanical pulping sludge, equipped with a wet scrubber control.
A stoker boiler (103.5 MMBtu/hr) burning 80% wood bark and 20% deinking sludge (mass basis), equipped with an electrostatic precipitator control.
A stoker boiler (349.2 MMBtu/hr) burning 71% bark and 29% wastewater residuals (mass basis), equipped with an electrostatic precipitator (ESP) control.
A fluidized bed boiler (210 MMBtu/hr) burning 76% bark, 21% sludge (municipal solid waste), and 3% gas (mass basis), equipped with a baghouse control.
Compilation of “air toxic” and total hydrocarbon emissions data for pulp and paper mill sources: a second update (Technical bulletin no. 973)
The data reported in technical bulletin no. 973 is based on 64 wood-fired boilers, all of which burn inland wood residues (NCASI Citation2010). In their analysis, NCASI applied advanced statistical techniques to detect outliers, which were then excluded from the reported data. The bulletin does not provide raw data, and only a summary was available. The bulletin contains data on emissions of more than 75 different air toxics. Among these emitted air toxics, the predominant pollutant species are acetaldehyde, formaldehyde, and benzene. The emissions from this source are shown as “NCASI Pulp and Paper” in .
Summary of emission factors from NCASI technical bulletins
shows the emission factors for air pollutants from statistical analysis of several sources along with specific emission factors associated with boilers that burn a combination of unconventional fuels.
As shown in , the emission factors reported by NCASI across several technical bulletins vary considerably for all pollutants. One explanation for the large variations could be that NCASI reports average numbers based on detailed statistical procedure applied to the data from boilers of several facilities, whose fuel composition information is not always available. Depending on the blend of the fuel (amount of sulfur, nitrogen, ash, etc.) and the boiler type, the emissions of pollutants vary significantly. When fuel composition data for individual facilities are available, it helps us identify factors that may have caused the variations in the emission factors. For example, the highest emission factor for PM is reported for facilities that burn the maximum proportion of wood in the fuel mixture (i.e., 100% bark and wet wood or 71% wood and 29% wastewater residuals), mainly due to the high ash content. For NOx and CO, the emission factors reported in MACT/CISWI testing were the highest, followed by boilers burning 49% wood and 51% sludge. The reported emission factor for SO2 is highest for boilers burning 49% wood and 51% sludge, which could be because of the high sulfur content in the sludge. The reported VOC emission factors are much lower compared to other pollutants. The emission factors reported by NCASI technical bulletins are based on data obtained from several biomass combustion sources across years, shown as a mean value. Moreover, the feed composition varies from other data sources, resulting in discrepancies among air pollutant emission factors when compared with each other. This explains why the emission factors reported by NCASI may be several orders of magnitude higher or lower than either stack test or air permit data, depending on the type of feed, composition and moisture content, and type of boiler.
Survey by Consortium for Research on Renewable Industrial Materials (CORRIM)
CORRIM did a survey to collect data on emission factors from 12 wood production facilities in the Pacific Northwest and Southeast United States, with an equal number of facilities surveyed in each region (Puettmann and Milota Citation2017). The data was collected for the boilers operating in the 2012 calendar year, with a mass weighted average fuel composition of 65.9% hog fuel, 13.5% sawdust, 10.7% shavings, and 9.4% wood and bark. The boiler ages were between 4 and 32 years, with most boilers at the lower end of the age limit and thus likely representing recent technology. The data were reported in pollutant mass emitted per unit fuel consumed (kilogrampollutant/kilogramwood), which we converted to lb/MMBtu using the high heating value of wood. shows the average emission factors for criteria and select toxic air pollutants. Information on emission control equipment for each mill was not available; thus, all the reported emission factors may not be considered uncontrolled. The very wide range of emission factors is due to the fact that the emission factors reported by CORRIM have a different fuel composition compared to stack test and air permit data source.
Figure 4. Emission factors for PM10, PM2.5, selected HAPs, and gaseous pollutants that are calculated from data reported by CORRIM. Y-axis units are lb/MMBtu. The data sources refer to 12 individual wood mills (wood production facilities) surveyed by CORRIM in the Pacific Northwest and Southeast United States. (Note that details about individual mills are not available since these were considered business confidential information.)
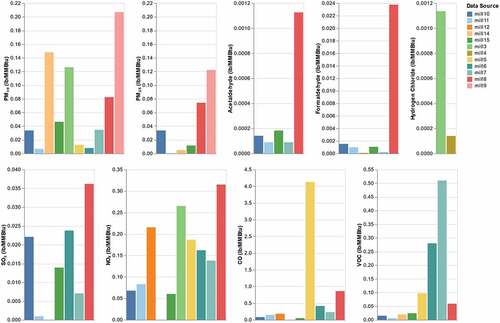
EPA’s CEDRI database
EPA developed the Compliance and Emissions Data Reporting Interface (CEDRI) and Web Factor Information Retrieval System (WebFIRE) because federal regulations require facilities to electronically submit emission test data in the form of performance test reports to EPA. WebFIRE is EPA’s online emissions data repository, retrieval, and development tool (https://www.epa.gov/electronic-reporting-air-emissions/webfire). The WebFIRE database does not provide the necessary information to estimate emission factors and is limited in the pollutants measured. Refer to the SI for details on emission factors reported from the database.
Summary of emission factors
Box plots in show the emission factors from various data sources, including air permits, stack test data, and NCASI technical bulletins, compiled and analyzed in this research. For the aerosol species, the distribution of uncontrolled emission factors is skewed to the right, and the mean emission factor is an order of magnitude greater than the median value. Also shown in are the emission factors currently included in EPA’s AP-42 Chapter 1.6 emission factors (marked as red dots). Interestingly, the emission factors from EPA’s AP-42 are slightly less than the median for filterable PM, but almost same as the median calculated from the data collected for this work for both PM10 and PM2.5. Because condensable PM is smaller than 2.5 microns in diameter, the emissions of the condensable component of PM are very small compared to PM10 and PM2.5.
Figure 5. Distribution of biomass-fueled boiler emission factors from collected data sources for each pollutant and inclusive of all fuels. Whiskers correspond to 5th and 95th percentiles, with means shown as black squares, medians as horizontal lines, and emission factors from EPA’s AP-42 as red dots. The number of data points used for each pollutant are also shown (n). These box plots report uncontrolled emission factors and thus do not include the CORRIM data.
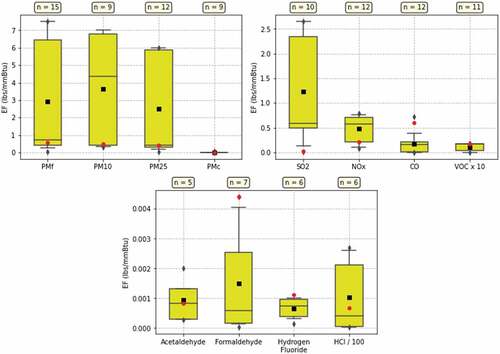
For the gaseous pollutants, the emission factor for NOx from EPA’s AP-42 is 12% lower than the calculated median. However, the emission factor for CO in AP-42 is higher by a factor of four compared to the calculated median emission factor. The emission factor for SO2 in AP-42 is smaller by a factor 20 compared to the calculated median emission factor, which is due to the high sulfur content in biomass fuels as compared to wood residues. Among the HAPs, the median emission factor for acetaldehyde aligns well with EPA’s AP-42 database, but the calculated median emission factor for formaldehyde is about a tenth of the emission factor used in EPA’s AP-42 database. The median emission factors for two acid gases, HCl and HF, are only about 59% and 67% of those in AP-42, respectively. There is a large range in the emission factors for HCl, and that is likely due to the type of particulate control technology. Wet scrubbers are highly efficient at capturing HCl compared to dry particulate control technologies. compares the emission factors for several pollutants from AP-42 to the range of emission factors and median numbers from the new data sources, disaggregated by type of fuels. Note that the plots in are based on uncontrolled emission factors and thus do not include CORRIM data (control technology information was not available from CORRIM to allow us to calculate uncontrolled emission factors).
Table 4. Range and median emission factors (lb/MMBtu) from data sources collected herein including air permits, stack test data, and industry research reports as compared to EPA’s AP-42 values, disaggregated by fuel type.
The results show that a boiler burning wood and wood residues with high moisture content likely emits more PM than a boiler burning dry fuel, whereas a boiler burning 100% sludge has lower PM emissions than a boiler burning wood residues. For combustion of biomass-derived waste fuels, we find PM emission factors to be higher than wood residues and sludge. Our results show that the emission factors for PM from wood residues are similar to AP-42, whereas burning sludge produces 54% less emissions as compared to AP-42. When combusting biomass waste, the emission factors are at least 23% higher than the values listed in AP-42. Overall, the emission factors for PM fractions (filterable PM10 and PM2.5) range from 0.2–7.5 lb/MMBtu, as compared to 0.5 and 0.43 lb/MMBtu for PM10 and PM2.5 from AP-42, respectively, whereas the condensable fraction of PM is expected to be relatively small, ranging from 0.005–0.06 lb/MMBtu as compared to 0.017 lb/MMBtu from AP-42. The large difference in variability of emission factors for PM may be attributed to composition of biomass fuel which is not always available in the literature. For example, if the moisture content in the fuel is high, the emission factors for reported PM are likely high as well. Filterable PM10 and PM2.5 have a large coefficient of variation (~180% for both) compared to condensable PM, which has a coefficient of variation of 80%. This is consistent with EPA’s finding that emissions of the condensable fraction of PM were independent of fuel type (EPA Citation2009).
Although the emission factors for SO2 are closely correlated with the amount of sulfur in the fuel mixture, the reported SO2 emissions vary considerably, ranging from 0.13 lb/MMBtu for wood residues, 0.02–0.51 lb/MMBtu for a mixture of wood residues and sludge, and 0.49–2.65 lb/MMBtu for biomass waste. The variation in emission factors for SO2 from different sources is mainly due to the amount of sulfur present in the fuel. The value reported in AP-42 for SO2 is at the low end of the range at 0.025 lb/MMBtu. Most sulfur in the fuel is expected to be emitted as SO2, though a small fraction could end up in ash. Thus, we recommend utilizing a mass balance approach to estimate the emission factor of SO2, as it is closely tied to the amount of sulfur in the fuel.
The reported AP-42 emission factor for NOx is 0.22 lb/MMBtu, which falls in the range from the surveyed data (0.02–0.79 lb/MMBtu). The emission factor of NOx is 3.6 times higher than in AP-42 for wood residues, whereas it could be lower by 45% or higher by 300% as compared to AP-42 when burning a mixture of wood residues and sludge. Similarly, for biomass waste, the emission factor of NOx can be up to 3.5 times higher compared to AP-42. The variability in emission factors for NOx is mainly a factor of combustion characteristics (complete vs incomplete combustion), boiler type, and composition of fuel. Fuel-bound nitrogen plays an important role in the amount of NOx to be formed and emitted. However, boiler manufacturers’ experience appears to indicate that a well-designed and properly tuned boiler should be able to reduce NOx emissions to 0.18–0.28 lb/MMBtu for stoker boilers and 0.15–0.24 lb/MMBtu for bubbling fluidized bed boilers (Bowman et al. Citation2009).
CO, which is the result of incomplete combustion, and which is inversely proportional to NOx formation, is estimated to range from 0.002–0.72 lb/MMBtu based on our surveyed data. Burning wood residues results in the highest CO emission factor, 0.72 lb/MMBtu, which is 20% higher than the value listed in AP-42. Burning a mixture of wood residues and sludge could result in a reduction of CO emissions by a factor of 1.5 as compared to AP-42, whereas burning biomass waste can further reduce CO emissions by a factor of 2.7 compared to AP-42. This is because increasing the air flow for oxidation of CO to CO2 at high temperature is expected to result in high thermal NOx formation.
Our analysis suggests that emission factors of organic compounds align reasonably well with the AP-42 emission factors, with a range of 0.003–0.019 lb/MMBtu. Although the data sources reported higher emission factors for some HAPs, most of them are within the values specified in AP-42, providing a good agreement with the published literature. However, the median emission factor for HCl is much lower than the AP-42 reported data. It should be noted that the secondary data we use has a large variability, with a coefficient of variation of 245%, which is likely due to the use of different particulate control technologies.
Conclusion
This analysis aims to compile and analyze emission factors from uncommon data sources for boilers that burn a single unconventional biomass-derived fuel or a combination of such fuels for which published data is scarce. The only emission factors that are currently available in the public domain and utilized by regulatory agencies are from EPA’s AP-42 emission factors for wood residue combustion, which do not necessarily resemble the biomass-based fuels being utilized in industrial boilers. Therefore, using the AP-42 emission factors, could result in inaccurate estimates of potential emissions from industries that utilize boilers that burn unconventional biomass fuels. Given that a chemical facility that includes a biomass fuel boiler will need air permits with detailed emission calculations prior to its construction and operation, this study surveyed a broad knowledge base to identify data that could provide a better understanding of how the emissions in permits/permit documentation, stack testing, and relevant technical reports compare to those from AP-42.
Upon a thorough review of emission factors from several data sources, which provide insights into emission factors for biomass boilers, we analyzed the data across the most important pollutants likely to be emitted from the boiler. The results suggest that the emission factors are highly variable for several air pollutants, depending on the type of fuel being combusted, the chemical composition of the fuel, and the fuel properties in the boiler. In general, the distribution of the emission factors from the studies reviewed in this work vary based on the type of fuel being combusted, with a right skewed distribution for filterable PM, PM10, PM2.5, SO2, and HCl. The median emission factors for NOx and the three PM components accounting for most of the emitted aerosol mass were about the same as the AP-42 emission factors. For SO2, the median emission factor is 20 times higher than the AP-42 emission factor. For CO, the AP-42 emission factor is four times higher than median emission factor and is far outside the 95th percentile data obtained from the new sources. Our analysis also indicates that major toxic air pollutants (HAPs) have a higher AP-42-based emission factor compared to the median emission factor reported in this work. Use of these new emission factors based on fuel composition is likely to provide a better estimate from the complex feedstock combustion in the boilers when emission testing is not feasible.
Although we provide a range of emission factors for a boiler that burns a mixture of biomass fuels, other process parameters should also be accounted for when selecting the best emission factors for each pollutant. These include the type of boiler utilized for combustion, flame temperature, and combustion practices employed at the facility. For example, fuel is combusted in stoker boilers by sitting on fixed grates, whereas fluidized bed boilers utilize inert particles for air to blow through them so that the bed behaves as a fluid. Stoker boilers are typically utilized for large wood-fired units with high steam generation, whereas fluidized bed boilers can handle fuels with more than 70% moisture content because of the larger share of thermal mass represented by hot inert bed particles. Similarly, the flame temperature and good combustion practices have significant effects on the products of combustion. This includes a proper air/fuel ratio, thorough mixing of air and fuel, and initial and sustained ignition of the mixture. Because air has a higher nitrogen content than oxygen, the required volume of air is much larger than the required volume of oxygen for a complete combustion. This affects the emissions of thermal NOx along with CO and CO2. Therefore, additional process parameters should be considered along with feed composition and fuel properties (such as moisture content) when selecting the most appropriate emission factors for the boiler to be evaluated.
The range of emission factors reported in this paper will be useful to permitting agencies, industrial facilities utilizing biomass-derived waste fuels, and biofuel developers and can facilitate estimation of potential to emit, which is required for air permitting purposes. Providing public access to such data would help save time and mitigate risks associated with permit applications being delayed due to inaccurate emission calculations. Although specifics about the boiler configuration, exact fuel properties, and other process parameters are important when selecting emission factors, this paper can be utilized as a starting point for emission estimation. Moreover, as the emission factors from published data sources are a useful starting point, performing detailed kinetic modeling of emissions from combustion of biomass-derived fuels could be a potential option for future research. In addition, obtaining stack test data for new facilities that burn a combination of unconventional biomass-derived fuels can help improve the estimates.
Boiler_emission_factor_data_Supplementary_Information.docx
Download MS Word (58.6 KB)Acknowledgment
This work was authored by Alliance for Sustainable Energy, LLC, the manager and operator of the National Renewable Energy Laboratory for the U.S. Department of Energy (DOE) under Contract No. DE-AC36-08GO28308. Support for the work was provided by ExxonMobil Research and Engineering Company (EMRE) under Agreement CRD-18-00765. We acknowledge funding from ExxonMobil. The views represented are those of the authors and do not necessarily reflect those of ExxonMobil. We thank Cynthia Randles and her team at EMRE for their valuable comments and inputs to the paper. The views expressed in the article do not necessarily represent the views of the DOE or the U.S. Government. The U.S. Government retains and the publisher, by accepting the article for publication, acknowledges that the U.S. Government retains a nonexclusive, paid-up, irrevocable, worldwide license to publish or reproduce the published form of this work, or allow others to do so, for U.S. Government purposes. We appreciate all the editing help from Susannah Shoemaker. We would like to thank Maureen Puettmann of CORRIM for sharing data on emissions from different (anonymous) boilers that CORRIM collected through a survey.
Disclosure statement
No potential conflict of interest was reported by the authors.
Data availability statement
The authors confirm that the data supporting the findings of this study are available within the article and its supplementary materials.
Supplementary data
Supplemental data for this article can be accessed online at https://doi.org/10.1080/10962247.2023.2166158
Additional information
Funding
Notes on contributors
Arpit Bhatt
Arpit Bhatt, is a Bioenergy Process Analysis Engineer at the National Renewable Energy Laboratory (NREL), USA, and focuses his research on techno-economic analysis, sustainability analysis, and air pollutant emissions modeling of renewable energy technologies.
Vikram Ravi
Vikram Ravi, Ph.D., is a research scientist at the National Renewable Energy Laboratory (NREL), USA. His research activities include using atmospheric modeling and machine learning techniques to assess and quantify air quality and public health co-benefits from biofuel generation and use, vehicle electrification, and decarbonization in the power sector.
Yimin Zhang
Yimin Zhang, is a senior researcher at the National Renewable Energy Laboratory (NREL), USA, and focuses her research on the development and application of life-cycle analysis models, sustainability analysis, and economic impact analysis of low- and zero-carbon technologies and strategies.
Garvin Heath
Garvin Heath, Ph.D., is an inaugural Distinguished Member of the Research Staff at the National Renewable Energy Laboratory (NREL), USA, specializing in the analysis of environmental impacts of energy systems–renewable and conventional, electricity and fuels.
Ryan Davis
Ryan Davis is a group manager and senior research engineer in the Economic, Sustainability, and Market Analysis group of the National Renewable Energy Laboratory (NREL), focusing on process modeling and techno-economic analysis for bioenergy technologies.
Eric C.D. Tan
Eric C. D. Tan, Ph.D., is a Senior Research Engineer in the Catalytic Carbon Transformation and Scale-up Center at the National Renewable Energy Laboratory (NREL), USA. His research activities include performing conceptual process design, techno-economic analysis, and various sustainability assessments.
Notes
1 Prevention of significant deterioration (PSD) applies to new major sources or major modifications at existing sources of pollutants where the area the source is located is in attainment or unclassifiable with the National Ambient Air Quality Standards (NAAQS). See https://www.epa.gov/nsr/prevention-significant-deterioration-basic-information for details.
References
- ACHR News. 2016. Advances in boiler construction and design [Internet]. https://www.achrnews.com/articles/131381-advances-in-boiler-construction-and-design.
- Allen’s Tristate Mechanical. 2017. How industrial boilers keep plants running efficiently [Internet]. https://www.allenstristate.com/how-industrial-boilers-keep-plants-running-efficiently/.
- Anthony, E. J. 1995. Fluidized bed combustion of alternative solid fuels; status, successes and problems of the technology. Prog. Energy Combust. Sci. 21 (3):239–68, January 1. doi:10.1016/0360-1285(95)00005-3.
- ARI Environmental, Inc. 2015. Relative accuracy test audit: Poet biorefining - project liberty solid fuel boiler exhaust.
- ARI Environmental, Inc. 2016. Relative accuracy test audit: Poet biorefining - project liberty solid fuel boiler exhaust.
- ARI Environmental, Inc. 2017. Relative accuracy test audit: Poet biorefining - project liberty solid fuel boiler exhaust.
- Bases, G. 2011. The history of the steam-generating boiler and industry [Internet]. Insulation Outlook. https://insulation.org/io/articles/the-history-of-the-steam-generating-boiler-and-industry/.
- Bhatt, A. H., Y. Zhang, R. Davis, G. Heath, and V. Ravi. 2022. Biorefinery upgrading of herbaceous biomass to renewable hydrocarbon fuels, Part 2: Air pollutant emissions and permitting implications. J. Clean. Prod. 362:132409, August 15. doi:10.1016/j.jclepro.2022.132409.
- Bowman, J., M. Davidson, J. Penterson, K. Toupin. 2009. Biomass combustion technologies: A comparison of a biomass 50MW modern stoker fired system and a bubbling fluidized bed system [Internet]. Riley Power Inc. http://www.babcockpower.com/pdf/RPI-TP-0218.pdf.
- Burns & McDonnell. 2014. Prevention of significant deterioration - air quality construction permit application: Source ID No. 1890231.
- Cao, G., X. Zhang, S. Gong, and F. Zheng. 2008. Investigation on emission factors of particulate matter and gaseous pollutants from crop residue burning. J. Environ. Sci. 20 (1):50–55, January 1. doi:10.1016/S1001-0742(08)60007-8.
- Carreras-Sospedra, M., R. Williams, and D. Dabdub. 2016. Assessment of the emissions and air quality impacts of biomass and biogas use in California. J. Air Waste Manag. Assoc. 66 (2):134–50, February 1. doi:10.1080/10962247.2015.1087892.
- Cheng, J., F. Zhou, T. Si, J. Zhou, and K. Cen. 2018. Mechanical strength and combustion properties of biomass pellets prepared with coal tar residue as a binder. Fuel Process. Technol. 179:229–37, October 1. doi:10.1016/j.fuproc.2018.07.011.
- Davis, R., A. H. Bhatt, Y. Zhang, E. C. D. Tan, V. Ravi, and G. Heath. 2022. Biorefinery upgrading of herbaceous biomass to renewable hydrocarbon fuels, Part 1: Process modeling and mass balance analysis. J. Clean. Prod. 362:132439, August 15. doi:10.1016/j.jclepro.2022.132439.
- Eberle, A., A. Bhatt, Y. Zhang, and G. Heath. 2017. Potential air pollutant emissions and permitting classifications for two biorefinery process designs in the United States. Environ. Sci. Technol. 51 (11):5879–88, June 6. doi:10.1021/acs.est.7b00229.
- Energy and Environmental Analysis, Inc. 2005. Characterization of the U.S.Industrial/Commercial boiler population [Internet]. Arlington, Virginia. https://www.energy.gov/sites/prod/files/2013/11/f4/characterization_industrial_commerical_boiler_population.pdf.
- EPA. 1970. National Ambient Air Quality Standards (NAAQS) | Technology Transfer Network | US EPA [Internet]. https://www.epa.gov/naaqs.
- EPA. 1995. Compilation of air pollutant emission factors. Volume 1: Stationary Point and Area Sources [Internet]. vol. 1, 5th ed. Office of Air Quality Planning and Standards U.S. Environmental Protection Agency. http://www3.epa.gov/ttn/chief/ap42/index.html#toc.
- EPA. 2009. AP-42: Compilation of air emissions factors [Internet]. https://www.epa.gov/air-emissions-factors-and-quantification/ap-42-compilation-air-emissions-factors.
- EPA. 2015. Air emissions inventories [Internet]. https://www.epa.gov/air-emissions-inventories.
- EPA. 2016. Clean Air Technology Center (CATC) technical resources - EPA’s air pollution technology fact sheets [Internet]. https://www3.epa.gov/ttncatc1/cica/atech_e.html#111.
- EPA. 2022. Basic information of air emissions factors and quantification [Internet]. https://www.epa.gov/air-emissions-factors-and-quantification/basic-information-air-emissions-factors-and-quantification.
- Federal Register. 2017. Environmental protection agency, 40 Code of Federal Regulations (CFR) Part 60 - Standards of performance for new stationary sources [Internet]. U.S. Government Publishing Office. https://www.ecfr.gov/on/2017-01-03/title-40/part-60.
- Federal Register, NSPS. 2023. Environmental protection agency, 40 Code of Federal Regulations (CFR) Part 63 - National emission standards for hazardous air pollutants for source categories [Internet]. U.S. Government Publishing Office. https://www.ecfr.gov/current/title-40/chapter-I/subchapter-C/part-63?toc=1.
- Industrial Controls. 2021. The evolution of boiler design & maintenance [Internet]. https://www.industrialcontrolsonline.com/training/online/evolution-boiler-design-maintenance.
- Jenkins, B. M., L. L. Baxter, T. R. Miles, and T. R. Miles. 1998. Combustion properties of biomass. Fuel Process. Technol. 54 (1):17–46, March 1. doi:10.1016/S0378-3820(97)00059-3.
- Jetter, J. J., and P. Kariher. 2009. Solid-fuel household cook stoves: Characterization of performance and emissions. Biomass Bioenergy 33 (2):294–305, February 1. doi:10.1016/j.biombioe.2008.05.014.
- Jetter, J., Y. Zhao, K. R. Smith, B. Khan, T. Yelverton, P. DeCarlo, and M. D. Hays. 2012. Pollutant emissions and energy efficiency under controlled conditions for household biomass cookstoves and implications for metrics useful in setting international test standards. Environ. Sci. Technol. 46 (19):10827–34, October 2. doi:10.1021/es301693f.
- Komariah, L. N., S. Arita, Novia, S. S. Wirawan, and M. Yazid. 2013. Emission factors of biodiesel combustion in industrial boiler: A comparison to fossil fuel. J. Renewable Sustainable Energy 5 (5):052005, September 1. doi:10.1063/1.4822036.
- Meyer, N., J. Leung. 2018. Sustainable options for reducing emissions from thermal energy: Showcasing successful outcomes from six case studies [Internet]. Center for Climate and Energy Solutions, October. https://www.c2es.org/document/case-studies-of-renewable-thermal-energy/.
- Mitchell, E. J. S., A. R. Lea-Langton, J. M. Jones, A. Williams, P. Layden, and R. Johnson. 2016. The impact of fuel properties on the emissions from the combustion of biomass and other solid fuels in a fixed bed domestic stove. Fuel Process. Technol. 142:115–23, February 1. doi:10.1016/j.fuproc.2015.09.031.
- Montrose Air Quality Services, LLC. 2019. Relative accuracy test audit: Poet biorefining - project liberty solid fuel boiler exhaust.
- NCASI. 2010. Compilation of air toxics and total hydrocarbon emissions data for the pulp and paper mill sources - a second update. Report No.: Technical Bulletin No. 973, National Council for Air and Stream Improvement, Inc, Research Triangle Park, NC
- NCASI. 2013. A comprehensive compilation and review of wood-fired boiler emissions. Report No.: Technical Bulletin No. 1013, National Council for Air and Stream Improvement, Inc, Research Triangle Park, NC.
- NCASI. 2015. A comprehensive compilation and review of combination wood-fired boiler emissions. Report No.: Technical Bulletin No. 1026, National Council for Air and Stream Improvement, Inc., Research Triangle Park, NC.
- NCASI. 2023. National Council for Air and Stream Improvement, Inc. [Internet]. https://www.ncasi.org/.
- Nielsen, H. P., F. J. Frandsen, K. Dam-Johansen, and L. L. Baxter. 2000. The implications of chlorine-associated corrosion on the operation of biomass-fired boilers. Prog. Energy Combust. Sci. 26 (3):283–98, June 1. doi:10.1016/S0360-1285(00)00003-4.
- Office of Energy Efficiency and Renewable Energy. 2018. Manufacturing energy and carbon footprints (2018 MECS) [Internet]. Office of EERE. https://www.energy.gov/eere/amo/manufacturing-energy-and-carbon-footprints-2018-mecs.
- Panagiotis, G. 2010. Solid biofuels for energy: A lower greenhouse gas alternative. vol. 28. London: Springer. doi:10.1007/978-1-84996-393-0.
- Puettmann, M. E., and M. Milota. 2017. Life-cycle assessment for wood-fired boilers used in the wood products industry*. For. Prod. J. 67 (5–6):381–89, September 1. doi:10.13073/FPJ-D-16-00064.
- Reynolds, O. 1961. On the extent and action of the heating surface of steam boilers. Int. J. Heat Mass Transf. 3 (2):163–66, September 1. doi:10.1016/0017-9310(61)90087-4.
- Singh, A., V. Sharma, S. Mittal, G. Pandey, D. Mudgal, and P. Gupta. 2018. An overview of problems and solutions for components subjected to fireside of boilers. Int. J. Ind. Chem. 9 (1):1–15, March 1. doi:10.1007/s40090-017-0133-0.
- Slade, R., A. Bauen, and R. Gross. 2014. Global bioenergy resources. Nat. Clim. Chang. 4 (2):99–105, Feb 1. doi:10.1038/nclimate2097.
- Tucki, K., O. Orynycz, A. Wasiak, A. Świć, L. Mieszkalski, and J. Wichłacz. 2020. Low emissions resulting from combustion of forest biomass in a small scale heating device. Energies 13 (20):5495. doi:10.3390/en13205495.
- Ushakova, A., V. Zatsepin, M. Varfolomeev, and D. Emelyanov. 2017. Study of the radical chain mechanism of hydrocarbon oxidation for in situ combustion process. J. Combust. 2017:2526596, March 6. Phuoc TX, editor. doi:10.1155/2017/2526596.
- Wisconsin Department of Natural Resources. 2019a. 2019 air emissions inventory summary report: Wisconsin electric power company - Rothschild biomass.
- Wisconsin Department of Natural Resources. 2019b. We energies Rothschild biomass cogeneration facility air pollution control construction permit application.
- WLA Consulting, Inc. 2011. 2011 updated facility design prevention of significant deterioration air quality construction permit application: Source ID No. 1890231.