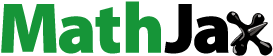
ABSTRACT
Asbestos is a known carcinogen and a banned hazardous material. However, the generation of asbestos-containing waste (ACW) is increasing because of the demolition of old constructions, buildings, and structures. Therefore, asbestos-containing wastes need to be effectively treated to render them harmless. This study aimed to stabilize asbestos wastes by using for the first time three different ammonium salts at low reaction temperatures. The treatment was performed with ammonium sulfate (AS), ammonium nitrate (AN), and ammonium chloride (AC) at concentrations of 0.1, 0.5, 1.0, and 2.0 M and reaction times of 10, 30, 60, 120, and 360 min intervals at 60 °C. Asbestos waste samples were treated in both plate and powder form during the experiment. The results demonstrated that the selected ammonium salts could extract the mineral ions from asbestos materials at a relatively low temperature. Concentrations of the minerals extracted from powdered samples were higher than those extracted from plate samples. AS treatment demonstrated better extractability compared to that of AN and AC, based on the concentrations of magnesium and silicon ions in the extract. The results implied that among the three ammonium salts, AS had better potential to stabilize the asbestos waste. This study demonstrated the potential of ammonium salts for treating and stabilizing asbestos waste at low temperatures by extracting the mineral ions from the asbestos fibers.
Implications: This study aims to establish an effective treatment to stabilize the hazardous asbestos waste to harmless forms. We have attempted treatment of asbestos with three ammonium salts (ammonium sulfate, ammonium nitrate, ammonium chloride) at relatively lower temperature. The selected ammonium salts could extract the mineral ions from asbestos materials at a relatively low temperature. These results suppose that asbestos containing materials could change the harmless state by using simple method. Among the ammonium salts, especially, AS has better potential to stabilize the asbestos waste.
Introduction
Asbestos is a widely used building material because of its advantageous physico-chemical and thermal characteristics, such as high strength, fire resistance, insulation, and durability (Gadikota et al. Citation2014; Kusiorowski et al. Citation2015; Pawelczyk et al. Citation2017). It was commonly utilized worldwide in construction and building materials in the past (Nam, Jeong, and Lim Citation2014; Pawelczyk et al. Citation2017). However, International Labor Organization (ILO) Convention classified asbestos as a harmful and toxic material in the 1960s ILO (International Labor Organization). Thereafter, most countries prohibited the use of asbestos-containing materials (ACMs), especially European countries (Kazan-Allen Citation2003). Despite this prohibition, the generation of asbestos-containing waste (ACW) materials has been increasing because of previously erected buildings constructed with ACMs getting dismantled (Candela et al. Citation2007; Kusiorowski et al. Citation2013). Asbestos consists of mineral silicate fibers with serpentine chrysotile type being one of the most commonly occurring form (Belardi and Piga Citation2013; Horikoshi et al. Citation2014). Chrysotile is a fibrous Mg phyllosilicate composed of alternating Mg octahedra and Si tetrahedral sheets, rolled together to form tubes (McCutcheon, Wilson, and Southam Citation2016; Pawelczyk et al. Citation2017). ACMs can disseminate fibrils into the atmosphere due to natural weathering or during dismantling and transport (Belardi and Piga Citation2013; Pawelczyk et al. Citation2017). Asbestos is known to cause lung cancer and/or pneumoconiosis when its scattered particles enter the human body (Candela et al. Citation2007). Therefore, several technologies have been reported for the treatment of the ACW materials (Valouma et al. Citation2017).
Chemical and/or thermal treatment and landfill are well-known methods for the treatment of asbestos waste. The chemical treatment method involves the use of strong acid or alkali solutions (Arce Ferrufino et al. Citation2018; Fusi, Monti, and Primicerio Citation2012; Maletaškić et al. Citation2017; McCutcheon, Wilson, and Southam Citation2016; McCutcheon et al. Citation2015; Naidu and Scherer Citation2012). Chemical treatment extracts the positive ions from asbestos, like magnesium, silicon, and iron. Thus, its morphological changes can be deduced from the needlelike fibers and/or an amorphous form. However, chemical treatment has its limitations since it reacts mostly with the ions in the outer layer of asbestos (David et al. Citation2021; Maletaškić et al. Citation2017). Thermo-chemical methods combining chemical and thermal treatments have been developed to address this limitation (Gandolfi et al. Citation2016). Generally, these treatments permeate the inner layers of asbestos, enhancing its reactivity to extract the chemical components from the inner layers (Belardi and Piga Citation2013; Candela et al. Citation2007; Fujishige et al. Citation2006; Kusiorowski et al. Citation2012; Lemos et al. Citation2016; Znamenáčková et al. Citation2016). However, these thermo-chemical methods are not economically viable due to their chemical and thermal requirements, since they consume a large amount of energy (>1000 °C in HCl solution) to change its morphology (Belardi and Piga Citation2013).
The second method used to render asbestos harmless is thermal treatment. Thermal treatment methods can be divided into vitrification and recrystallization (Paolini et al. Citation2018; Spasiano and Pirozzi Citation2017). Vitrification involves a high energy consumption process to change the morphology and shape. Generally, the thermal treatments use high temperatures of more than 1,600 °C as a result of which asbestos melts and gets converted into slag (Horikoshi et al. Citation2014). During this process, the asbestos is converted into a glass like substance. This implies that its elements do not change although its amorphous or other crystalline structures get altered. The thermal method is very effective as it destroys the asbestos structure completely by fusion, reduces the volume of waste, and converts asbestos into inert residues. However, it is highly energy intensive.
The third treatment method is encapsulation. It is meant to prevent asbestos from mingling with the atmosphere during dismantling and transport. This method generally uses resin to cover the ACM (Paolini et al. Citation2018). It stabilizes the asbestos fibers to coat a surface of resin. However, it cannot completely prevent ACW release into the atmosphere. Further, coated resin needs periodic maintenance because it loses its characteristics with time (Vergani et al. Citation2022).
The last treatment method for asbestos waste is landfill. This treatment is well-known and one of the most commonly used final disposal methods (Belardi and Piga Citation2013; Pawelczyk et al. Citation2017). However, landfill is a different treatment from other methods as mentioned because it is the final disposal methodology (Marian et al. Citation2021). Since, most of the ACW is generated from construction and/or building materials used in the past (Nam, Jeong, and Lim Citation2014), its generation depends upon the dismantling activity, durability of materials, life of the building, and redevelopment activities. However, even while using the landfill method, there is a potential risk of particles getting disseminated into the atmosphere during dismantling and transportation (Belardi and Piga Citation2013; Gadikota et al. Citation2014; Pawelczyk et al. Citation2017). In addition, it releases ACM into the atmosphere when the environmental conditions change. Considering these factors, burying untreated asbestos is not a permanent treatment method. Further, increasing ACW generation cannot be accommodated in this method because available landfill sites are limited.
The use of microbial treatment to convert the ACW to a harmless material has been reported recently (David et al. Citation2020a, Citation2020b; McCutcheon, Wilson, and Southam Citation2016; McCutcheon et al. Citation2017). The microbial treatment mechanism needs a relatively long treatment duration and appropriate experimental conditions such as pH, temperature, and humidity. Therefore, alternative technologies and additional studies are needed to efficiently treat the increasing quantities of ACW.
Based on previous research data, morphology control is the most suitable method to render asbestos inert. Most previous researchers have examined treatments with chemicals, high temperatures, or hybrid mechanisms to convert the asbestos morphology and shape. These have focused on the magnesium component in asbestos as it is a constituent of naturally occurring serpentine forms, namely chrysotile and lizardite (Arce Ferrufino et al. Citation2018; McCutcheon, Wilson, and Southam Citation2016; McCutcheon et al. Citation2015; Valouma et al. Citation2017; Znamenáčková et al. Citation2016). However, asbestos waste also contains calcium, silicon, iron, etc., because of its use as a building and construction material. Among these, calcium ions in the waste material can form carbonates via natural weathering with time (Kusiorowski et al. Citation2013). It implies that the weathered outer layer of asbestos waste is more amenable to treatment in comparison to the inner layer. Furthermore, there is a need to develop a methodology that permeates the asbestos waste and modifies both the structure of the inner and outer layers in a single treatment for better stabilization of asbestos.
This study aimed to modify the hazardous structural configuration of ACW into an inert state by using ammonium salts and low temperatures. The selected ammonium salts extracted positive ions from asbestos. Additionally, the study also aimed to check the possibility of reusing these extracted positive ions. Thus, metal ion extraction from ACW can change the asbestos morphology and shape by breaking its structure. As previously mentioned, other researchers have focused to use high temperatures or strong acids to extract magnesium ions from asbestos. However, ACW also contains other positive ions including calcium. Therefore, the treatment to convert the asbestos into an inert safe material must consider the reactivity of not only magnesium but also other positive ions. This study design included the removal and extraction of all the positive ions from asbestos, like magnesium, and silicon. To develop a feasible treatment method based on the above-mentioned strategy, three different ammonium salts which have not been investigated for asbestos treatment earlier, were selected.
Consequently, this research tested the possibility of treating ACW by using the selected ammonium salts at lower temperature conditions and the possibility of reuse of the stabilized material after treatment.
Materials and methods
The ammonium salts selected for the study were ammonium sulfate (AS), ammonium nitrate (AN), and ammonium chloride (AC). There are no research reports to date on the use of the selected ammonium salts for asbestos treatment applications. All experiments for asbestos treatment were performed at a 60 °C.
For this study, roofing slate waste containing asbestos (raw waste) was collected. Because the samples had been neglected in outside for a long time before collection, It meant that the amount of CaCO3 might have remained on the surface of the sample. Therefore, ammonium salt needed relatively long time to react with magnesium and silicon in the sample. After considering the effect of weathering on the slate samples, the change in the concentration of the positive ions in the raw waste material was measured at intervals of 10, 30, 60, 120, and 360 min.
The roofing slate waste was analyzed by using X-ray diffraction (XRD) and X-ray fluorescence (XRF) to determine its major components. The treatment experiment was performed by using two forms of the waste: plate (solid), and powder. Plate samples were cut into square pieces of 0.5 cm in length and breadth. The powdered sample was prepared by grinding the waste in the ball mill to a particle size of lesser than 25 μm. The powdered sample was separated by a sieve. The roofing slate waste samples were added to the three different ammonium salt solutions. The concentrations of the solutions of ammonium salts prepared in distilled water were 0.1, 0.5, 1.0, and 2.0 M. The treatment was performed in batch type reactor with a 400-mL capacity. The waste sample and the respective concentration of the ammonium salt treatment solution were added into the reactor, heated to maintain the temperature at 60 °C, and mixed by using a magnetic stirrer at 120 rpm. At defined time intervals, the mixed treatment slurry was separated by a vacuum pump to obtain the solid and liquid fractions. The liquid was filtered through a filter with a pore size of 0.7 μm. The minerals in the filtered separated solution were analyzed by an inductively coupled plasma optical emission spectrometer (ICP-OES) ().
Results and discussion
The major components of the roofing slate waste materials revealed by XRF analysis were CaO (35.1%), SiO2 (18.7%), and MgO (6.77%). Other components in the waste were Al2O3 (4.44%), Fe2O3 (3.6%), K2O (0.28%), Na2O (0.11%), and MnO (0.07%). The analysis revealed calcium as the major component because the roofing slate was constructed using cement. Since the surface of the roofing slate was exposed to nature for a long time, there is a possibility that calcium could have been converted to calcium carbonate by weathering (Kusiorowski et al. Citation2013).
shows the XRD results, which revealed calcite (calcium carbonate) as the major peak, confirming the weathering effect on the roofing slate material. Although calcite was the major component, chrysotile peaks were detected at 12, 24, and 36, 2-Theta. The selected ammonium salt solutions could react with the calcium carbonate on the surface layer first (Kusiorowski et al. Citation2013). Although the ACW is homogeneous material, it is simpler to explain the reaction mechanism when the layer by layer reaction is envisioned as a sandwich model. Through the surface reaction, a thin film of the ACW broke aiding the ammonium salt solution to permeate and react with the asbestos components in the inner layers. According to Maletaškić et al., asbestos could react via leaching and/or extraction when acid was used for treatment (Maletaškić et al. Citation2017). They also reported an increased change in asbestos morphology with increasing acid concentration and retention time (Maletaškić et al. Citation2017). Similar to the results of Maletaškić et al., asbestos removal and associated morphological changes were also observed in this study.
Characteristics of extracted metal ions by ammonium salts from the roofing slate waste plate
show positive ions extracted in each ammonium salt solution. Extracts from plate samples show that the concentration of metal ions depended on the treatment solution strength. For instance, calcium, magnesium, and silicon concentrations in 2.0 M AS were higher than those in 0.1, 0.5, and 1.0 M solutions. A similar treatment solution-dependent trend was observed for calcium and magnesium concentration in each of the ammonium salt treatments. The ammonium salt solutions react first with the surface layers of the roofing slate waste. Notably, the major components of the outer layer of roofing slate are calcium and magnesium (Kusiorowski et al. Citation2013). A significant difference in the extracted magnesium concentration was not observed with an increase in ammonium salts concentration from 0.1 to 0.5 M with higher retention time.
Table 1. Extracted calcium ion concentration from ACW sample in each solution (mg/l).
Table 2. Extracted magnesium ion concentration from ACW sample in each solution (mg/l).
Table 3. Extracted silicon ion concentration from ACW sample in each solution (mg/l).
Ammonium salts are well-known hydrophilic chemicals. Due to their high solubility, they dissociate, forming ammonium ions in the water (Publishing Citation2013). During this process, ammonium salt acts as a weak acid, and the generated weak acid extracts the positive ions from the roofing slate waste and gets neutralized in turn. Also, some of the ammonium ions could get emitted into the atmosphere with rise in temperature. Through this reaction, the acidic components could change into dissociated chemicals such as CaSO4, CaNO3 and CaCl2, representatively. As a result, the major chemical components will be OH− group. Finally, the ammonium salt solution becomes alkaline because of OH− ions are formed. EquationEquations (1)(1)
(1) –(Equation3
(3)
(3) ) show the chemical reaction of ammonium salts with water and subsequent acid generation.
Ammonium sulfate (AS)
(1)
(1)
Ammonium nitrate (AN)
(2)
(2)
Ammonium chloride (AC)
(3)
(3)
Considering EquationEquations (1)(1)
(1) –(Equation3
(3)
(3) ), the three types of ammonium salts generate the ammonium ion and acid in water. In this process, the acid generated reacted with the outer layer in roofing slate waste directly (Kusiorowski et al. Citation2013).
Interestingly, the magnesium concentration decreased in 0.1 M AS solution with retention time. According to McCutcheon et al., some of the magnesium ions could have been adsorbed on the amorphous silica layer when sulfuric acid was used by them to extract the magnesium (McCutcheon et al. Citation2015). Our observation of the decreasing magnesium concentration may also have been on account of a similar magnesium ion adsorption phenomenon. The major constituent of the surface layer in roofing slate waste is calcium, and its calcium carbonate form can easily react with acids. This implies that the initial reaction step during treatment is calcium component removal. As a result, the amorphous silicate layer is exposed, and it is assumed that ionized magnesium was adsorbed on the exposed amorphous silicate layer.
Characteristics of extracted metal ions by each ammonium salt from roofing slate waste powder
show the characteristics of positive ions extracted in each of the ammonium salt solutions from powdered roofing slate waste. The concentrations of extracted calcium, magnesium, and silicon from the powder sample are higher than those in the plate sample. Generally, the specific surface area of the powder was higher than that of the plate samples. These results demonstrated that reactivity was dependent on the specific surface area under similar experimental conditions (Kusiorowski et al. Citation2013; Maletaškić et al. Citation2017). The major content of calcium ions was observed in each ammonium salt treatment during the initial reaction time of 10 min (). Although most of the results indicated increasing calcium ion concentration in the extracts with an increase in reaction time, which was as expected, except in the case of AS treatment. In AS treatment, the extracted calcium concentration rapidly decreased with time, although maximum extraction concentration was observed with 2.0 M AS. The calcium ion may have reacted with SO4 ions in AS after extraction resulting in the conversion of the extracted calcium ion to an insoluble CaSO4 salt (McCutcheon et al. Citation2015). The decrease in calcium ions in the extracts with time may have resulted from its precipitation as CaSO4 (McCutcheon et al. Citation2015). Among the ammonium salt solutions, AN and AC extracts contained higher calcium ions than those in AS solution. The higher extraction may also be due to the increased specific surface area of the powdered form of the sample. The powdered sample reacted better than the plate sample where the initial reaction occurred only on the outer layer. Another reason for higher calcium concentration is the reactivity of AN and AC with calcium ions. NO3 and Cl2 ions react with calcium ions easily and the resultant Ca(NO3)2 and CaCl2 formed are highly hydrophilic and dissolve easily in water. Given the high solubility, most of the calcium ions can be extracted and are retained in solution in the separated liquids (Goodman, Underwood, and Grassian Citation2000). Therefore, it was assumed that higher calcium ion concentrations were due to Ca(NO3)2 and CaCl2 dissolution in the separation process. The chemical reactions are as follows:
Roofing slate waste surface and precipitation reactions with acids formed in the ammonium salts solution
Considering EquationEquations (4)(4)
(4) –(Equation9
), some magnesium ions from ACW could be extracted because the reactions occurred concurrently. Although the dominant reaction extracted calcium ions from roofing slate waste, most of the calcium ions were extracted during the initial process. Magnesium concentration was observed to increase with increasing concentration of each ammonium salt solution (). Therefore, the AC solution is better than the other ammonium salt solutions for magnesium extraction. Although extracted magnesium concentration was lower with AS and AN treatment, they displayed enough potential to extract the magnesium ions from asbestos waste. However, the trend for magnesium extraction with 0.1 M AS solutions was different from all the other treatments. In this case, the magnesium concentration in the extract remained constant with increasing retention time. As seen in the case of plate samples, this trend may be due to the adsorption of magnesium ions on the amorphous silicon layer, which was exposed by extraction reactions (McCutcheon et al. Citation2015).
In the case of silicon extraction, AS exhibited better performance than that of AN and AC solutions. Silicon concentration in the extracts was maintained constant or decreased with time (). Silicon reactivity affects magnesium extraction when silicon concentration is dominant (McCutcheon, Wilson, and Southam Citation2016). According to Pawełczyk et al., magnesium could not be extracted or removed efficiently because of silicon reactivity when H2SO4 was applied to ACMs (Pawelczyk et al. Citation2017). However, this study was performed using plate (solid) asbestos and because of this limitation, our study results cannot be explained by it. According to Nicola et al., the outer layer in ACW is generally composed of calcium, magnesium, and iron, and most of the inner layer is composed of silicon (Gandolfi et al. Citation2016). Therefore, ACM in powder form could become saturated after adsorbing the magnesium ions to a certain extent on the amorphous silicon layer. For this reason, magnesium ion concentration remained constant in the extract with time when treated with AS solution due to their simultaneous adsorption. Consequently, magnesium ions from ACW could be extracted continuously when AS solution was used. According to McCutcheon et al., sulfuric acid has better efficiency at extracting the magnesium ion compared to other acids (McCutcheon, Wilson, and Southam Citation2016). AS also has the potential to change the ACW into harmless materials.
shows the SEM results for each solution. In , it is observed that the fibers in the raw material decreased after the reaction with ammonium salts. Especially, 0.5 M ammonium solution showed better results compared to other concentrations. Thus, Ca and Mg ions in asbestos were extracted in an ammonium salt solution. These results suggested a negative effect because the thickness and aspect ratio of asbestos were decreased. Generally, small and fine particles are known to be more dangerous. Especially, the danger will increase when raw ACW is changed into small sized, fine particles. According to World Health Organization (WHO), asbestos fiber has an aspect ratio greater than 3:1 (Kusiorowski et al. Citation2015). However, our results indicated an aspect ratio over 3:1, caused by extracting the magnesium and silicon in ACW with ammonium salt solution. Considering these results, ammonium salts showed a negative effect in spite of extracting the ACW to increase its inertness. Therefore, this study shows the possibility of reducing AWC and extracting the mineral ions from it.
Figure 3. Representative SEM results of ACW in each solution and concentration (a = plate, b = powder).
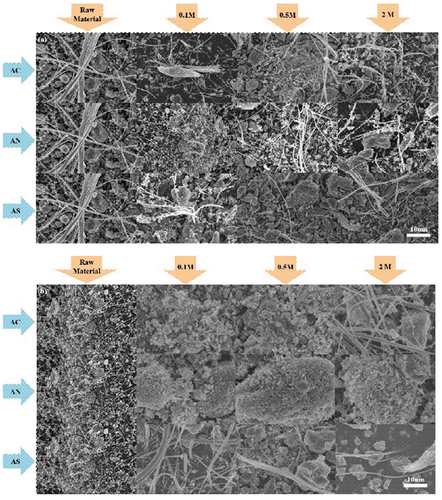
The ACW technology described in this study can be re-used in various industries because its shape and morphology were changed rendering it inert and harmless. Among these, the by-product of ACW treatment could be used for removing phosphorus and the volume of waste from building materials. According to Satoshi et al., serpentine dust could absorb phosphorus (Mymrin et al. Citation2020). In addition, Masao et al., reported that asbestos morphology and shape change, and decomposition reduced the volume of waste from building materials. Further, decomposed ACM can be utilized as an admixture with cement because it can increase the adhesive strength of cement (Fujishige et al. Citation2006).
Conclusion
This study aimed to extract the positive ions from asbestos by treatment using ammonium salt solutions for the first time. Based on the research results, it is evident that the three selected ammonium salts could change and decompose the morphology of ACW by extracting the positive ions. This method has adequate potential because of the relatively low treatment temperature (60 °C) and low concentrations of ammonium salts required for treatment. The extraction efficiency depended on the shape of the roofing slate waste, the efficiency being better in the case of powdered samples. Moreover, selected ammonium salts revealed the possibility to form other chemical compounds from the extracted ions from asbestos morphology such as CaSO4, Ca(NO3)2, and CaCl2. Among the ammonium salts, AS demonstrated the best performance for magnesium and silicon layer decomposition. Therefore, the research results demonstrate the feasibility to change the asbestos waste shape and morphology nevertheless its aspect ratio was increasing.
Disclosure statement
No potential conflict of interest was reported by the author(s).
Data availability statement
The data that support the findings of this study are available from the corresponding author, [Gwangmok Kim] and first author [Sangwon Park] upon reasonable request.
Additional information
Funding
Notes on contributors
Sangwon Park
Sangwon Park is a Ph.D in chemical engineering. He has been studying CO2 utilization and waste reuse method. His specialities include: CO2 absorption, CO2 utilization and developing its process, recovery of the valuable metals and minerals from waste, waste management etc. He is currently working for Korea institute of geoscience and mineral resources(KIGAM) as a principle researcher.
Yeon-Sik Bong
Yeon-Sik Bong is a Ph.D scientist who has worked in the Korea Basic Scientist Institute(KBSI) for more than 20 years. Her specialties include isotope geochemistry, and traces country of geographical origin using stable isotope ratio mass spectrometer.
Gwangmok Kim
Gwangmok Kim is a Ph.D in civil engineering. His research interests are multi- and cross-disciplinary, and are primarily concerned with micromechanics of advanced engineering materials, and development of sustainable and functional construction materials with an emphasis on the integration with nano-technology. He is currently working for Korea institute of geoscience and mineral resources as a senior researcher.
References
- Arce Ferrufino, G. L. A., S. Okamoto, J. C. Dos Santos, J. A. de Carvalho, I. Avila, C. M. Romero Luna, and T. Gomes Soares Neto. 2018. CO2 sequestration by pH-swing mineral carbonation based on HCl/NH4OH system using iron-rich lizardite 1T. J. CO2 Util. 24:164–73.
- Belardi, G., and L. Piga. 2013. Influence of calcium carbonate on the decomposition of asbestos contained in end-of-life products. Thermochim. Acta 573:220–28. doi:10.1016/j.tca.2013.08.019.
- Candela, P. A., C. D. Crummett, D. J. Earnest, M. R. Frank, and A. G. Wylie. 2007. Low-pressure decomposition of chrysotile as a function of time and temperature. Am. Mineral. 92 (10):1704–13. doi:10.2138/am.2007.2559.
- David, S. R., D. Ihiawakrim, R. Regis, and V. A. Geoffroy. 2020a. Efficiency of pyoverdines in iron removal from flocking asbestos waste: An innovative bacterial bioremediation strategy. J. Hazard. Mater. 394:122532.
- David, S. R., D. Ihiawakrim, R. Regis, and V. A. Geoffroy. 2020b. Iron removal from raw asbestos by siderophores-producing Pseudomonas. J. Hazard. Mater. 385:121563.
- David, S. R., A. Jaouen, D. Ihiawakrim, and V. A. Geoffroy. 2021. Biodeterioration of asbestos cement by siderophore-producing Pseudomonas. J. Hazard. Mater. 403:123699. doi:10.1016/j.jhazmat.2020.123699.
- Fujishige, M., R. Sato, A. Kuribara, I. Karasawa, and A. Kojima. 2006. Low-temperature decomposition of sprayed-on asbestos. J. Ceram. Soc. Japan 114 (12):1133–37.
- Fusi, L., A. Monti, and M. Primicerio. 2012. Determining calcium carbonate neutralization kinetics from experimental laboratory data. J. Math. Chem. 50 (9):2492–511. doi:10.1007/s10910-012-0045-3.
- Gadikota, G., C. Natali, C. Boschi, and A. H. Park. 2014. Morphological changes during enhanced carbonation of asbestos containing material and its comparison to magnesium silicate minerals. J. Hazard. Mater. 264:42–52. doi:10.1016/j.jhazmat.2013.09.068.
- Gandolfi, N. B., A. F. Gualtieri, S. Pollastri, E. Tibaldi, and F. Belpoggi. 2016. Assessment of asbestos body formation by high resolution FEG-SEM after exposure of Sprague-Dawley rats to chrysotile, crocidolite, or erionite. J. Hazard. Mater. 306:95–104. doi:10.1016/j.jhazmat.2015.11.050.
- Goodman, A., G. Underwood, and V. Grassian. 2000. A laboratory study of the heterogeneous reaction of nitric acid on calcium carbonate particles. J. Geophys. Res. 105 (D23):29053–64.
- Horikoshi, S., T. Sumi, S. Ito, R. Dillert, K. Kashimura, N. Yoshikawa, M. Sato, and N. Shinohara. 2014. Microwave-driven asbestos treatment and its scale-up for use after natural disasters. Environ. Sci. Technol. 48 (12):6882–90.
- ILO (International Labor Organization) C162 - Asbestos Convention. 1986.
- Kazan-Allen, L. 2003. The asbestos war. Int. J. Occup. Environ. Health. 9 (3):173–93. doi:10.1179/oeh.2003.9.3.173.
- Kusiorowski, R., T. Zaremba, A. Gerle, J. Piotrowski, W. Simka, and J. Adamek. 2015. Study on the thermal decomposition of crocidolite asbestos. J Therm Anal Calorim 120 (3):1585–95.
- Kusiorowski, R., T. Zaremba, J. Piotrowski, and J. Adamek. 2012. Thermal decomposition of different types of asbestos. J. Therm. Anal. Calorim. 109 (2):693–704.
- Kusiorowski, R., T. Zaremba, J. Piotrowski, and A. Gerle. 2013. Thermal decomposition of asbestos-containing materials. J. Therm. Anal. Calorim. 113 (1):179–88.
- Kusiorowski, R., T. Zaremba, J. Piotrowski, and J. Podwórny. 2015. Utilisation of cement-asbestos wastes by thermal treatment and the potential possibility use of obtained product for the clinker bricks manufacture. J. Mater. Sci. 50 (20):6757–67.
- Lemos, B. R. S., E. A. R. Soares, A. P. C. Teixeira, J. D. Ardisson, L. E. Fernandez-Outon, C. C. Amorim, R. M. Lago, and F. C. C. Moura. 2016. Growth of carbon structures on chrysotile surface for organic contaminants removal from wastewater. Chemosphere 159:602–09.
- Maletaškić, J., N. Stanković, N. Daneu, B. Babić, M. Stoiljković, K. Yoshida, and B. Matović. 2017. Acid leaching of natural chrysotile asbestos to mesoporous silica fibers. Phys. Chem. Miner. 45:343–351.
- Marian, N. M., G. Giorgetti, C. Magrini, G. C. Capitani, L. Galimberti, A. Cavallo, R. Salvini, C. Vanneschi, and C. Viti. 2021. From hazardous asbestos containing wastes (ACW) to new secondary raw material through a new sustainable inertization process: A multimethodological mineralogical study. J. Hazard. Mater. 413:125419. doi:10.1016/j.jhazmat.2021.125419.
- McCutcheon, J., G. M. Dipple, S. A. Wilson, and G. Southam. 2015. Production of magnesium-rich solutions by acid leaching of chrysotile: A precursor to field-scale deployment of microbially enabled carbonate mineral precipitation. Chem. Geol. 413:119–31.
- McCutcheon, J., C. Turvey, S. Wilson, J. Hamilton, and G. Southam. 2017. Experimental deployment of microbial mineral carbonation at an asbestos mine: Potential applications to carbon storage and tailings stabilization. Minerals 7 (10):191.
- McCutcheon, J., S. A. Wilson, and G. Southam. 2016. Microbially accelerated carbonate mineral precipitation as a strategy for in situ carbon sequestration and rehabilitation of asbestos mine sites. Environ. Sci. Technol. 50 (3):1419–27. doi:10.1021/acs.est.5b04293.
- Mymrin, V., P. Presotto, K. Alekseev, M. A. Avanci, P. H. B. Rolim, V. Petukhov, A. Taskin, E. Gidarakos, A. Valouma, and G. Yu. 2020. Application of hazardous serpentine rocks’ extraction wastes in composites with glass waste and clay-sand mix to produce environmentally clean construction materials. Constr. Build. Mater. 234:117319.
- Naidu, S.; G. W. Scherer. 2012. Development of hydroxyapatite films to reduce the dissolution rate of marble. Proceedings of 12th International Congress on Deterioration and Conservation of Stone, 1–9, New York, NY.
- Nam, S. N., S. Jeong, and H. Lim. 2014. Thermochemical destruction of asbestos-containing roofing slate and the feasibility of using recycled waste sulfuric acid. J. Hazard. Mater. 265:151–57. doi:10.1016/j.jhazmat.2013.11.004.
- Paolini, V., L. Tomassetti, M. Segreto, D. Borin, F. Liotta, M. Torre, and F. Petracchini. 2018. Asbestos treatment technologies. J. Mater. Cycles Waste Manag. 21 (2):205–26. doi:10.1007/s10163-018-0793-7.
- Pawelczyk, A., F. Bozek, K. Grabas, and J. Checmanowski. 2017. Chemical elimination of the harmful properties of asbestos from military facilities. Waste Manag. 61:377–85.
- Publishing, R. 2013. Merck index. 15th ed. Whitehouse Station, N.J.: Royal Society of Chemistry.
- Spasiano, D., and F. Pirozzi. 2017. Treatments of asbestos containing wastes. J. Environ. Manage. 204 (Pt 1):82–91.
- Valouma, A., A. Verganelaki, I. Tetoros, P. Maravelaki-Kalaitzaki, and E. Gidarakos. 2017. Magnesium oxide production from chrysotile asbestos detoxification with oxalic acid treatment. J. Hazard. Mater. 336:93–100. doi:10.1016/j.jhazmat.2017.04.019.
- Vergani, F., L. Galimberti, N. Marian, G. Giorgetti, C. Viti, and G. Capitani. 2022. Thermal decomposition of cement–asbestos at 1100 °C: How much “safe” is “safe”? J. Mater. Cycles Waste Manag. 24 (1):297–310.
- Znamenáčková, I., S. Dolinská, M. Lovás, S. Hredzák, M. Matik, J. Tomčová, and V. Čablík. 2016. Application of microwave energy at treatment of asbestos cement (eternit). IOP Conf. Ser. 44:052023.