ABSTRACT
The Liikavaara Östra Cu-(W-Au) deposit is situated close to the operating Aitik Cu-Au mine in northern Sweden. It is scheduled for production in 2023. Modern geological descriptions of the deposit are lacking though knowledge of geological and mineralogical details prior to operation is beneficial to avoid surprises. In this study, petrological, mineralogical and geochemical investigations of the wall rocks, host rock and mineralisation, and zircon U-Pb analysis of a footwall granodioritic intrusion were carried out. The mineralisation is hosted by quartz±tourmaline-calcite veins, calcite veins and aplite dykes that cross-cut biotite-amphibole schists and gneisses. The wall rocks to the ore are metavolcaniclastic rocks of basaltic to andesitic composition. A granodiorite intrusion occurs in the footwall. The mineralisation is mainly chalcopyrite, pyrrhotite and pyrite with some sphalerite, galena, scheelite, molybdenite and magnetite. It shows slight enrichments in Au, Ag and Bi. Gold and Ag occur as electrum and Ag also in hessite and an Ag-sulphide. The Bi mineralogy includes native Bi, Bi-tellurides and Bi-sulphides. These minerals are found as inclusions, along the borders of and in cracks in chalcopyrite, pyrite, pyrrhotite, sphalerite, molybdenite and quartz. The footwall granodiorite intrusion was dated at 1.87 Ga. It is suggested here to be the source for ore genesis based on its spatial relation to the mineralisation, as well as on high-salinity fluids and metal composition of the ore. The aplite dykes may have acted as pathways for the magmatic hydrothermal fluids that carried the metals from the intrusion to the host rock.
Introduction
The Liikavaara Östra Cu-(W-Au) deposit is located in the ore district of northern Norrbotten in Sweden (). It is situated about 3.5 km east of the renowned Aitik Cu-Au deposit (Wanhainen et al. Citation2012), one of the largest Cu mines in Europe. Both deposits were discovered in the 1930s by Boliden AB. Findings of glacial boulders with disseminated chalcopyrite lead to geological investigations and follow-up electromagnetic surveying that indicated two zones of interest – Aitik and Liikavaara (Zweifel Citation1976). Aitik went into production in 1968 while Liikavaara was considered sub-economic. Technical advances in production and processing of the ore have allowed Boliden AB to extend the lifetime of Aitik several times and to increase production considerably. Likewise, these advances lead Boliden AB to resume exploration activities on the Liikavaara Östra Cu-(W-Au) deposit and to re-evaluate it as economic. Boliden AB is now preparing for production of the deposit in 2023. A resource estimate is shown in .
Table 1. Official resource estimates (complying with the PERC Code) for the Liikavaara Östra Cu-(W-Au) deposit (date: 2018.12.31). Tungsten and Mo are currently not considered for production but Mo might be produced in the future.
Figure 1. Location of the Liikavaara Östra Cu-(W-Au) deposit and geology of the Gällivare area (modified from Martinsson & Wanhainen Citation2004). The SWEREF99 TM coordinate system is used. Note, all rocks are metamorphosed, the prefix “meta-” is left out to keep rock names short.
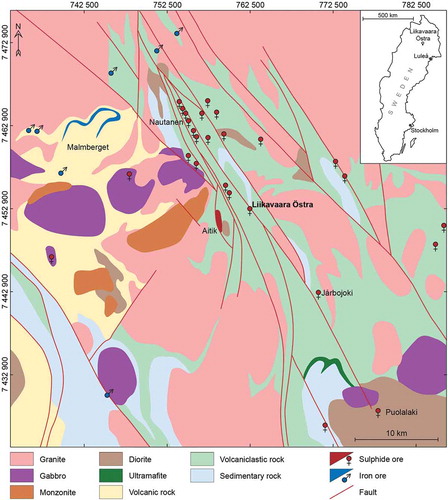
Due to the focus on the Aitik deposit, geological studies on the Liikavaara Östra Cu-(W-Au) deposit are limited. To date, the most comprehensive study is the PhD thesis by Zweifel (Citation1976), which covered geological investigations on both Aitik and Liikavaara deposits. Other publications include Malmqvist & Parasnis (Citation1972), Monro (Citation1988), Martinsson & Wanhainen (Citation2004), Sammelin et al. (Citation2011) and Estholm (Citation2014). Beside the bachelor thesis of Estholm (Citation2014), none of the above mentioned studies have Liikavaara as a focus but is included to provide a regional context for their data sets. Additionally, all studies refer to Zweifel’s (Citation1976) work when discussing geological characteristics of the deposit. Consequently, more investigations are needed to better constrain the genesis, mineralisation and character of the Liikavaara Östra Cu-(W-Au) deposit.
Chemical analyses by Boliden AB of drill cores have indicated enrichments in Cu, W, Au, Ag and Bi. Among these elements, W and Bi are included in the list of ‘Critical Raw Materials’ (CRMs) first published by the European Commission in 2011 and updated in 2014 and 2017, respectively, (European Commission Citation2017). The European Commission defines CRMs to be raw materials of high economic importance to the EU but with a high risk associated with their supply (European Commission Citation2017). Currently, Boliden AB plans to feed the Liikavaara ore to the Aitik plant and thus Au and Ag will be produced as by-products next to Cu, the main commodity. However, in spite of their criticality, W and Bi are deemed economically non-feasible to produce and will consequently be treated as waste. Nevertheless, characterisation of W and Bi is important to predict their behaviour during processing (e.g., Bi can lower the quality of Cu-metal) and to investigate possibilities for future extraction. Likewise, variability of Cu, Au and Ag mineralisation between Liikavaara and Aitik could affect recovery efficiency and thus detailed characterisation is crucial.
The goal of this study is a refined geological and mineralogical description of the Liikavaara Östra Cu-(W-Au) deposit to provide a base for subsequent studies focused on ore characterisation of trace minerals using advanced micro-analytical techniques. Interpretations are based on drill core logging, chemical assays, petrography, and dating of a key rock unit. The results of this study should also support processing of the ore and exploration for similar deposits in the area.
Regional geology
The Liikavaara Östra Cu-(W-Au) deposit is situated in the Gällivare area in the ore district of northern Norrbotten in Sweden (). The district is famous for its world-class Kiirunavaara and Malmberget apatite-iron deposits and the Aitik and Nautanen Cu-Au deposits. The bedrock in northern Norrbotten is part of the Fennoscandian Shield which spans across parts of Norway, Sweden, Finland and Russia. It is constituted of mostly Archean and Palaeoproterozoic rocks and is the product of a complex series of geological events including plume activity, rifting, subduction, accretion and collision (Nironen Citation1997; Lahtinen et al. Citation2005; Weihed et al. Citation2005; Lahtinen et al. Citation2009; Melezhik & Hanski Citation2012; Stephens & Bergman Weihed Citation2020).
Deformed 2.8–2.6 Ga metagranitoids and gneiss form the Archean basement in northern Norrbotten (Bergman et al. Citation2001). The basement is only exposed in the northern parts of the region. Elsewhere it is unconformably overlain by supracrustal successions of Palaeoproterozoic age (Bergman et al. Citation2001). Stratigraphically lowest of these supracrustals are rift-related Karelian rocks (2.4–1.96 Ga), which in the Kiruna area are represented by the Kovo Group (2.8–2.3 Ga) and the overlying Kiruna Greenstone Group (2.3–2.0 Ga; Martinsson Citation1997; Bergman et al. Citation2001). Terrestrial to shallow water-deposited Svecofennian successions of metasedimentary and metavolcanic rocks are conformably to disconformably overlying the Karelian units. They comprise the Porphyrite Group, the Kurravaara Conglomerate, the Kiirunavaara Group and the Hauki Quartzite in the Kiruna area (Martinsson Citation2004; Bergman Citation2018). Metasedimentary sequences have limited extents (Martinsson et al. Citation2016). In the Gällivare area, the Muorjevaara Group, a lower Svecofennian unit (1.89–1.88 Ga), comprises both calc-alkaline andesitic metavolcanic and clastic metasedimentary rocks (). Different proportions between these rocks and variations in grain sizes and compositions of the sedimentary rocks record rapid facies changes (Martinsson & Wanhainen Citation2004). The volcanic rocks were likely formed by an early Svecofennian continental arc magmatism during north-east directed subduction at the margin of the Archean craton (Martinsson & Perdahl Citation1995; Martinsson Citation2004).
The Palaeoproterozoic metasupracrustal rocks were intruded by several plutonic suites. The 1.89–1.87 Ga Haparanda and Perthite Monzonite Suites are calc-alkaline to alkali-calcic in character and considered co-magmatic with the Svecofennian volcanic rocks (Witschard Citation1984; Martinsson Citation2004). Minimum melt granites and pegmatites are characteristic of the 1.81–1.78 Ga Lina Suite (Bergman Citation2018) and they are temporally related to TIB 1 (Transscandinavian Igneous Belt) monzonitic to granitic intrusions in the Kiruna-Narvik area (Romer & Wright Citation1992; Romer et al. Citation1994; Martinsson & Wanhainen Citation2004). Transscandinavian Igneous Belt 2 granitoids near the Swedish-Norwegian border are the youngest plutonic rocks at ca. 1.71 Ga (Romer & Wright Citation1992; Martinsson & Wanhainen Citation2004). At least two events of orogenic deformation and metamorphism occurred during the Palaeoproterozoic (Bergman et al. Citation2001). The first affected the Haparanda Suite but seemingly pre-dates the Perthite Monzonite Suite. The second event, at 1.8 Ga, was at least locally developed and took place contemporaneously with the emplacement of the Lina Suite rocks.
In the Gällivare area, rocks in the eastern part are dominated by volcaniclastic rocks belonging to the Muorjevaara Group while those in the western part are intermediate to felsic volcanic rocks of the Kiirunavaara Group (Martinsson & Wanhainen Citation2004; ). The supracrustal rocks were intruded by plutonic rocks of ultramafic to felsic compositions. Diorites and granodiorites representing the Haparanda Suite are common as minor intrusions in the Muorjevaara Group while monzonites of the Perthite Monzonite Suite are restricted to the west. Several 1.88 Ga and 1.80 Ga gabbroic to troctolitic intrusions, such as the Dundret intrusion, occur throughout the bedrock of the Gällivare area (Sarlus et al. Citation2018). Lina Suite granites cover large areas and related pegmatites are mainly found within the Muorjevaara Group (Martinsson & Wanhainen Citation2004; Wanhainen et al. Citation2005; Sarlus et al. Citation2018; ). The supracrustal rocks and older intrusions were metamorphosed under amphibolite facies conditions (Zweifel Citation1976; Wanhainen et al. Citation2006; Tollefsen Citation2014). A major shear zone, the Nautanen Deformation Zone, runs north-northwest through the area and many of the Cu-Au occurrences in the area are spatially related to it (Bergman et al. Citation2001; Martinsson & Wanhainen Citation2004; Sammelin et al. Citation2011; Wanhainen et al. Citation2012).
Deposit geology
The Liikavaara Östra Cu-(W-Au) deposit is mainly enriched in Cu and somewhat in Au, Ag and W (; ). It also shows some enrichments in Zn, Mo, Sn, Sb, Pb and Bi, but their production is currently economically unfeasible. The mineralised zone extends for about 1 km along a N15°W strike, is 100 m wide and dips 80°W to a depth of roughly 500 m (indicated by drilling; Zweifel Citation1976; ). The deposit has been interpreted to be hosted by the eastern limb of a south-southeast dipping syncline (Zweifel Citation1976; Lynch et al. Citation2015).
Figure 2. Geological map and profile A-B of the Liikavaara Östra Cu-(W-Au) deposit. The SWEREF99 TM coordinate system is used. A. Plan view of the Liikavaara Östra Cu-(W-Au) deposit at 100 m below surface. Numbered drill holes marked in white were studied and sampled during this study. B. Cross section from A to B through the deposit. The deepest drill hole reaches a depth of approximately 500 m below surface.
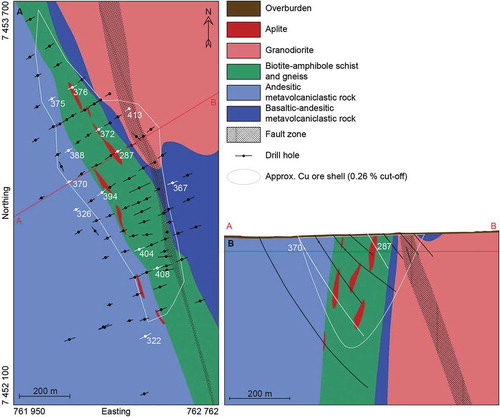
According to Zweifel (Citation1976), the wall rocks can be divided into a lower and upper formation of meta-arenites. The footwall includes feldspar quartzites, phyllitic feldspar quartzites and conglomerates. The hanging wall consists of meta-arenites intercalated with amphibolitic rocks. Zweifel (Citation1976) discusses a volcanic origin of these rocks based on the geochemical signatures but chooses an epiclastic classification based on observations of cross- and graded bedding and of conglomerates. In contrast, Estholm (Citation2014) classifies the rocks as meta-andesites and meta-andesitic tuffites based on petrological and geochemical studies ().
The rocks hosting the ore are described by Zweifel (Citation1976) as biotite schist which grades to biotite gneiss and biotite quartzite, and by Monro (Citation1988) as quartz-biotite gneiss ().
Malmqvist & Parasnis (Citation1972) describe mineralisation at Liikavaara to be dominantly of a vein type. They characterise the mineralisation as being associated with an irregular pattern of small fissures and veins, mostly 1–20 cm in width. The main gangue minerals are quartz and calcite; other minerals include tourmaline and fluorite (Malmqvist & Parasnis Citation1972; Zweifel Citation1976).
Zweifel (Citation1976) identifies chalcopyrite as the only mineral of economic importance, but also notes the occurrence of pyrrhotite, pyrite, galena, sphalerite, scheelite, magnetite and molybdenite. Zweifel (Citation1976) mentions scheelite as dominantly associated with quartz veinlets and locally calcite veinlets. He also noted scheelite connected with quartz veinlets containing chalcopyrite in a granite 1 km north of Liikavaara. Sammelin et al. (Citation2011) describe the occurrence of gold in quartz veins alongside chalcopyrite, pyrrhotite, tourmaline and calcite.
The Liikavaara Östra deposit is cut by several partly mineralised aplite dykes (). A granite intrusion in the footwall 100 to 200 m northeast of the Liikavaara mineralisation has been described by Zweifel (Citation1976) as of Lina Suite type ().
Methods
This study includes three parts: (1) drill core logging and geochemical analysis of the wall and host rocks, (2) mineralogical studies of the wall and host rocks and mineralisation, (3) and U-Pb isotopic analysis of zircons from the footwall intrusion.
Drill core logging, sampling and geochemical analysis
Three drill cores (370, 372 and 322; ) were fully logged at metre scale for a total of 890 m for sampling of the various rock types, and nine cores were partially logged at centimetre scale for ~60 m for targeted sampling of the mineralisation. Cores 370 and 372 were chosen based on their location centrally in the ore zone. Core 370 cuts through the hanging wall into the ore zone while core 372 begins in the ore zone and ends in the footwall. The drill core of hole 322, located outside the ore zone, was logged for comparison.
Based on mineralogical and textural variations in the rocks, 22 samples were collected for whole rock analysis (). Sixteen samples were taken from drill core 370 and four samples from core 372. Two additional samples were taken from the drill core of hole 413 that intersects the footwall intrusion.
Figure 3. Drill cores sampled for whole rock analysis and mineralogical studies. Depth of each sample is shown. Whole rock samples are to the left of each drill core, mineralogical samples to the right. Samples in italic letters were collected to support the whole rock data with petrographic observations. Samples in bold were targeted at mineralogy of specific metals (metals of interest are listed above each drill core). The remaining samples were collected to study vein and mineralisation mineralogy.
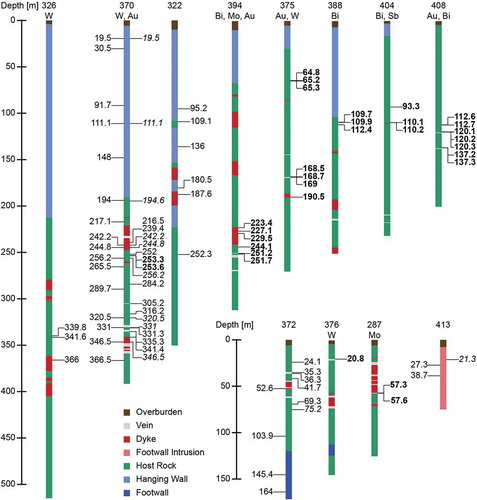
Whole rock analysis was performed by ALS Scandinavia AB. Method of sample preparation and analysis for each element are listed in the . Several replicates, lab standards and blanks were analysed for quality control. The data were plotted in several volcanic and plutonic rock classification diagrams. Data of one sample from core 370 (331 m) had to be omitted due to intersection of a quartz vein.
For comparison, whole rock data from the bachelor thesis of Estholm (Citation2014) was included in the plots. This data set comprises 17 analyses from the hanging wall, three analyses from the mineralisation zone of core 370, and three analyses from the footwall of core 367. Additionally, data from Boliden AB of 63 environmental samples (whole-rock analyses for mine environment monitoring) from 20 drill cores were used as well. The samples from Estholm (Citation2014) were analysed by AcmeLabs while the environmental samples were also analysed by ALS Scandinavia AB. Methods of sample preparation and analysis are listed in the .
Grades of S, Cu, Zn, Mo, Ag, Sn, W, Au, Pb, Bi, Sb, Th and U, routinely checked for in drill cores by Boliden AB, were used to assess metal distribution downhole and in the deposit.
Mineralogical studies
Twenty samples were selected from drill cores 370, 372 and 322 for micro-analytical analysis of the various vein- and mineralisation styles observed during logging (). Another 34 samples were collected from drill cores known to be enriched in various metals (based on the chemical assays by Boliden AB) to ensure sufficient amounts of metals in the samples for future analysis with micro-analytical techniques. To constrain potential mineralogical variation across the deposit a total of nine drill cores were sampled (). Additionally, for petrographic characterisation of the various rock types at Liikavaara and for comparison with the whole rock data another eleven samples were collected (). The locations of all sampled drill cores are marked in .
Polished thin sections were prepared from samples and analysed with a petrographic microscope (Nikon ECLIPSE E600 POL) in transmitted and reflected light and with scanning electron microscope (Zeiss Merlin FEG-SEM). Mineralogy, texture and structure of each thin section were studied with emphasis on the occurrence and association of ore minerals. For SEM analysis, the thin sections were coated with carbon. The SEM was operated at an acceleration voltage of 20 kV and a probe current of 1 nA. For SEM-EDS analysis the Oxford AZtec software and the included element standards were used. Prior to every session the beam energy was calibrated against Cu-tape. Mineral identification was performed by spot analysis at a total count rate of 250 000 counts per spot.
U-Pb isotopic analysis
Several kilograms of sample were collected from the intrusion in the footwall northeast of the mineralisation. From this sample zircons were extracted for age dating. The extraction procedure included grain size reduction, density and magnetic separation and hand-picking.
The rocks were crushed with a jaw mill and further reduced in size with a chromium swing mill. The crushed rocks were sieved for a size fraction of 40–500 µm. Density separation with a Wilfley table was followed by magnetic separation using a hand magnet and a Frantz Isodynamic magnetic separator before a second density separation with Methylene Iodide (CH2I2 3.2 g cm−3). Lastly, zircons were hand-picked using an optical microscope/stereoscope.
The zircons were mounted and polished by staff at the Vegacenter micro-analytical facility at the Swedish Museum of Natural History in Stockholm, Sweden. Here, U–Pb isotopic analysis was carried out with Laser Ablation Multi Collector Inductively Coupled Plasma Mass Spectrometry (LA-MC-ICP-MS). The instrument settings are summarised in . Prior to and post analysis the internal structure of the crystals was examined with backscattered electron (BSE) imaging in a scanning electron microscope (Zeiss Merlin FEG-SEM).
Table 2. The instrument settings of the LA-MC-ICP-MS used for U/Pb-analysis on zircons at the Vegacenter micro-analytical facility at the Swedish Museum of Natural History in Stockholm, Sweden. The zircons were sampled from an intrusion in the footwall of the Liikavaara Östra Cu-(W-Au) deposit suggested to be associated with its formation.
Isotope ratios were normalised to the 91 500 Zircon bracketing standard (drift and accuracy correction) with age 1065 Ma (Wiedenbeck et al. Citation1995). GJI, Plesovice and Temora were used as secondary standards. Conventional common Pb correction was applied for samples with 206Pb/204Pb <7000 according to Petrus & Kamber (Citation2012).
Inverse concordia (Tera–Wasserburg) diagrams were constructed and a 207Pb–206Pb weighted average age was calculated using the Isoplot plugin (ver. 4.15) of Ludwig (Citation2003) in Excel.
Results
Logging and petrography of wall and host rocks
The rocks forming the hanging wall are (green)-(brown)-greyish in colour and porphyritic with a very fine-grained (<0.1 mm) matrix of mainly feldspar and quartz with lesser biotite, sericite, epidote, calcite, chlorite and disseminated hematite (, B). Biotite laths define a weak to strong lepidoblastic texture. Phenocrysts of plagioclase and K-feldspar vary in abundance from 1 to 5 % and range in shape from an- to euhedral and in size from 0.2–2.5 mm (). Many phenocrysts are affected by sericitisation and locally saussuritisation.
Figure 4. Drill core images of the different rock types at Liikavaara (A–G) and vein-, alteration- and mineralisation-styles (H–M). A. Hanging wall (metavolcaniclastic rock). B. Hanging wall (clast-rich metavolcaniclastic rock). C. Host rock (biotite schist). D. Aplite dyke. E. Host rock (amphibole gneiss). F. Footwall (metavolcaniclastic rock). G. Footwall intrusion (granodiorite). H. Porphyritic aplite dyke. I. Foliated aplite dyke with thin quartz-tourmaline-sulphide veins parallel to foliation. J. Quartz-calcite veins in biotite schist, parallel to foliation. Some sulphides occur in the veins, especially molybdenite at the borders. A dark selvage of biotite, tourmaline and epidote is developed at the contact of the largest quartz vein within the biotite schist (see black rectangle). K. Cross-cutting quartz-calcite vein in biotite schist. The biotite schist transitions to amphibole gneiss towards the bottom. L. Deformed quartz-calcite-sulphide vein in biotite-amphibole schist. M. Quartz-tourmaline-calcite-sulphide vein. Chalcopyrite fills interstices between tourmaline grains and more massive chalcopyrite and pyrrhotite extend into quartz. Several scheelite grains occur in the quartz-rich part of the vein (within the black rectangle).
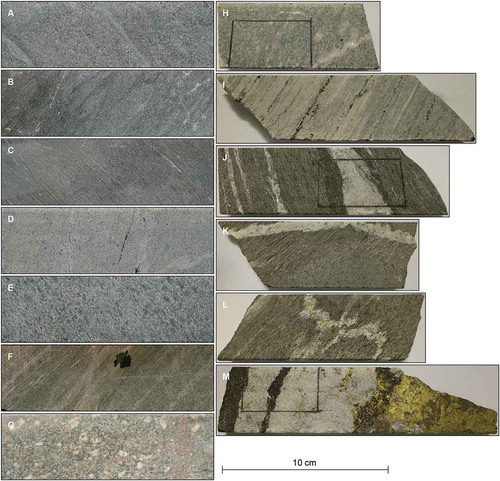
Figure 5. Transmitted light photomicrographs of the various rocks in the Liikavaara Östra Cu-(W-Au) deposit. A. Euhedral plagioclase phenocrysts in the matrix of a metavolcaniclastic rock of the hanging wall. B. Altered feldspar phenocrysts in the matrix of a metavolcaniclastic rock of the footwall. C. Amphibole gneiss. D. Biotite-amphibole gneiss. E. Biotite schist. F. Granodiorite. G. Aplite. H. Biotite-altered aplite. I. Quartz-dominated aplite. J. Muscovite-altered quartz-dominated aplite. Note: 5 C is taken with plane polarised light, the rest with crossed polarised light. Abbreviations: Amph – amphibole, Bt – biotite, Cal – calcite, Ep – epidote, Feld – feldspar, Mc – microcline, Ms – muscovite, Pl – plagioclase, Qz – quartz, Seri – sericite.
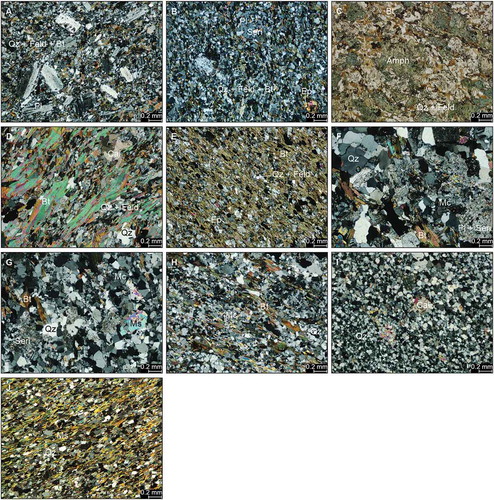
Up to several centimetres large, rounded and elongated clasts of light to dark grey and green colour occur in the hanging wall in some places (). They are elongated parallel to the main biotite-defined foliation. Most clasts resemble the surrounding rock in texture and mineralogy except for a lack of distinct biotite alignment. Colour differences are caused by varying amounts of biotite and epidote. Highly flattened dark clasts of biotite-rich rock are locally observed.
Based on the above observations the hanging wall rocks are interpreted as metavolcaniclastic
Mineralisation at Liikavaara is hosted by biotite-amphibole schists and gneisses (Zweifel Citation1976; , E and 5C–E). The degree of biotitisation is considerably variable within the rock with strong effects on composition and texture. Three common textures are observed: (1) the least altered areas comprise a matrix of partly euhedral plagioclase laths (0.2–0.5 mm) and fine-grained (<0.2 mm) K-feldspar, quartz and biotite with minor calcite, sericite, epidote and chlorite and some disseminated magnetite and sulphides. Green, subhedral amphibole phenocrysts (mostly ~0.5 mm) are homogeneously distributed and make up 20–30 % of the rock ( and ). Biotite laths are poorly aligned and partly replace amphibole. (2) With increasing biotitisation the amphiboles are almost entirely replaced and biotite is organised in aligned bands (). (3) Eventually, the biotite bands get more numerous and interconnected turning the rock into a biotite schist ( and ). Here, the biotite is finer-grained than in texture (2) (, E). At this stage no more amphiboles are observed.
The footwall rock is green-greyish in colour and porphyritic (). The matrix is formed by fine-grained (<0.2 mm) feldspar, quartz, biotite, amphibole and epidote with minor disseminated magnetite, hematite, pyrite and chalcopyrite. Biotite laths and amphibole form a lepidoblastic texture and are in parts altered to chlorite. Phenocrysts (0.2–1.5 mm) include altered plagioclase, K-feldspar and occasionally amphibole (). Some relict phenocrysts are recrystallized to smaller grains of feldspar. Clasts, several centimetres in size, rounded and elongated, occur throughout the footwall unit. They are elongated parallel to the main foliation. Their mineralogy is similar to that of the surrounding rock, but is more fine-grained (<0.1 mm), has less biotite and granular texture. Also, phenocrysts are more abundant and more euhedral. Calcite can be found disseminated in the clasts and along their borders.
Like the hanging wall rocks, the footwall rocks are interpreted as metavolcaniclastic
The porphyritic granitoid in the footwall to the mineralisation ( and ) has a matrix comprised of fine- to medium-grained (0.5–1.2 mm) quartz, microcline, plagioclase, biotite and chlorite (). Pyrite, chalcopyrite, sphalerite, and magnetite are disseminated. Phenocrysts of subhedral plagioclase make up ~5 vol. % of the intrusion. They range in size from 1 to 8 mm and are affected by sericitisation. The rock is massive in character with no clear oriented fabric.
Light grey aplite dykes, ranging from a few centimetres up to 30 m in width, are frequent in the ore zone (, H, I and 5G–J). They appear to be subparallel to the mineralised zone and display partly distinct and partly diffuse contacts to the surrounding rocks. Variations in texture and mineralogy exist within and between dykes but two common types can be observed.
The first type is porphyritic. Its matrix is fine-grained (<0.2 mm), comprised of quartz and microcline and slightly overgrown by biotite, muscovite, calcite and tourmaline (). Sericite alteration of feldspar is partly developed. Pyrite, chalcopyrite, pyrrhotite, sphalerite and galena are locally disseminated. Phenocrysts include 0.5–7 mm K-feldspars, often altered to sericite or muscovite, and 1–2 mm sized quartz phenocrysts. Matrix and phenocrysts are weakly oriented following foliation.
The second type is more fine-grained (<0.1 mm) and dominated by granoblastic quartz grains with varying amounts of microcline (). Phenocrysts are less abundant.
Both types are partly affected by biotitisation and muscovitisation (, J). The micas form a lepidoblastic texture.
Lithogeochemistry
For chemical classification of the different rock types identified by logging and petrographic analysis, whole-rock chemical data were plotted in various volcanic and plutonic classification diagrams ().
Figure 6. Classification of rock types in Liikavaara based on three sets of whole rock chemical data. One set was analysed in this study, one set is from Estholm (Citation2014) and one set is from Boliden AB (environmental samples). A. Volcanic TAS diagram by Le Maitre et al. (Citation1989). Data are shifted towards more trachytic composition compared to C and D. B. Igneous Spectrum plot by Hughes (Citation1973). Data of the wall rocks and footwall intrusion plot mainly within the igneous spectrum, only a few hanging wall samples show Na-metasomatic alteration. In contrast, most data of the host rock and dykes show K-metasomatic alteration. C. Modified immobile element ratio plot by Pearce (Citation1996). Composition is variable between basaltic (host rock) and andesitic (hanging wall) with the footwall in between. Dykes and the intrusion are more felsic. D. Immobile element classification diagram for deeply weathered rocks by Hallberg (Citation1984). Although not deeply weathered, strong hydrothermal alteration of the host and wall rocks make this diagram interesting. The results are consistent with the classification after Pearce (Citation1996). E. AFM diagram after Kuno (Citation1968) and Irvine & Baragar (Citation1971). Most data lie in the calc-alkaline field. F. R1-R2 plutonic chemical variation diagram by De La Roche et al. (Citation1980). Only data of intrusive rocks (dykes and footwall intrusion) are plotted excluding the strongly altered host rock. Most data plots in the granite field. G. IUGS QAP plutonic rock classification diagram. Normative calculation was performed on data used in F. Dyke data lie in the monzogranite field, footwall intrusion data on the alkali side of the granodiorite field.
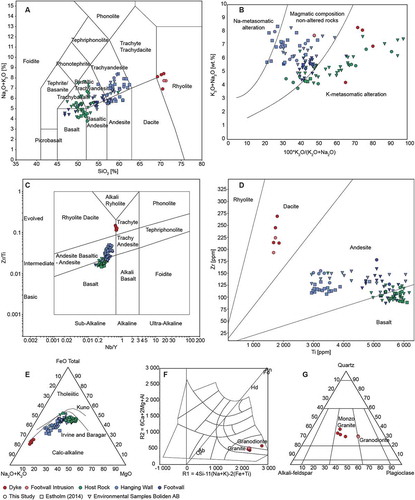
The TAS diagram by Le Maitre et al. (Citation1989) shows a systematic shift of a large fraction of the data, compared to immobile element classification diagrams, towards trachytic composition (). This may suggest sodium and/or potassium alteration of the rocks. However, the wall rocks and the footwall intrusion lie within the igneous spectrum defined by Hughes (Citation1973; ), only a few hanging wall samples show slight Na-metasomatic alteration. In contrast, dykes and most host rock data are affected by K-metasomatic alteration (). K-enrichment is most prominent in host rock samples of type (3).
Consequently, the immobile element ratio plot by Pearce (Citation1996; modified after Winchester & Floyd (Citation1977)) and the immobile element classification diagram for deeply weathered rocks by Hallberg (Citation1984) provide more reliable results (, D). Most data from the hanging wall show an andesitic composition while data from the host rock are basaltic (, D). The footwall data lie chemically in between. Data from aplite dykes and the footwall intrusion are chemically similar and show a felsic composition (, C, D). In the AFM diagram after Kuno (Citation1968) and Irvine & Baragar (Citation1971) the data of all rocks follow a calc-alkaline trend ().
Using the limited amount of chemical data from this study, the variation diagram R1-R2 by De La Roche et al. (Citation1980) show that the footwall intrusion and aplite dykes are mostly granitic (). In contrast, the QAP plutonic rock classification diagram separates the footwall intrusion (granodiorite) from the aplite dykes (monzogranite) (). Normative mineral calculation for the QAP diagram was achieved via the CIPW Norm calculation program by Hollocher (Citation2004). Data of the host rock of the mineralised zone, although potentially intrusive, was not plotted in the plutonic rock diagrams due to its strongly altered character.
Overall, the chemical classifications are in support of the petrographic observations. There are no notable variations between data of different drill cores and only minor discrepancies between different data-sets.
Mineralisation
Metal distribution
Chemical assays of drill cores intersecting the Liikavaara Östra Cu-(W-Au) deposit () show that the mineralised zone is mostly confined to the host rock and that metal enrichment decreases towards the wall rocks. The assays cover a limited suite of elements routinely analysed for by Boliden AB. However, fewer elements were analysed in older drill cores and thus interpretation of potential variations in distribution for some metals (e.g. Zn, Pb, Bi, Sb, U) is limited. Nevertheless, lateral element distributions appear largely to be similar, although S enrichment, mainly in the form of pyrite and pyrrhotite mineralisation, extends further south than Cu enrichment.
Figure 7. A. Relative concentration of various metals over 124 drill cores intersecting the Liikavaara Östra Cu-(W-Au) deposit. Each dot represents a value measured for a section in a drill core. Note that sections vary in length and values are not normalised, consequently concentrations are not actual metal grades. Analysis of older drill cores covered a smaller suit of elements which is why some elemental maps (e.g. Bi, Sb etc.) are represented by fewer dots than others. The approximate 0.26 % Cu ore shell is shown as a black line in each map as visual reference. The SWEREF99 TM coordinate system is used. B. Downhole grades of metals in drill core 394 in the Liikavaara Östra Cu-(W-Au) deposit. Metals are enriched in the host rock and especially within and in proximity to aplite dykes and veins. Copper, W, Au, Ag and Bi show a similar distribution pattern. Molybdenum also shares their major peak. Zink and Pb are similar to each other but show a different distribution than the other metals. S shows, as expected, a mixture of the two groups.
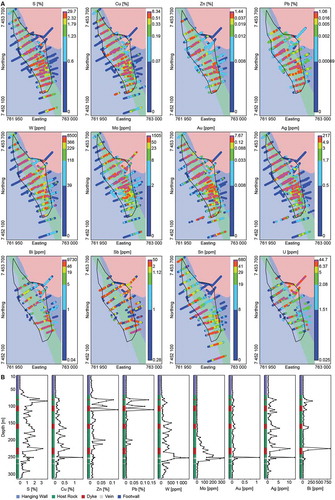
The spatial distribution of mineralisation in the Liikavaara Östra Cu-(W-Au) deposit is largely controlled by the aplite dykes, i.e. most mineralisation occurs within or in the proximity of these dykes (). The mineralisation is hosted by veins although some dissemination of aligned pyrite, pyrrhotite, chalcopyrite and magnetite is present in the host rocks as well (). In the veins, the ore minerals form aggregates and disseminations and are commonly complexly intergrown.
Veins
Veins are found in host and wall rocks and aplite dykes but are more voluminous in the mineralised zone.
Quartz-dominated veins are the most abundant. They are variable in sizes from a few microns to several metres in width but millimetre- to centimetre-wide veins dominate (–M). Likewise, vein density is variable and is highest in the mineralised zone with a vein every few centimetres. The veins are mostly parallel to the foliation in the host rocks (), but in places cross-cut it, while others show ductile deformation or emplacement style (, L). Both sharp and diffuse contacts to the surrounding rocks are observed. Especially wider (>1 cm) veins are enriched in black tourmaline, calcite and sulphides ( and ) and metre-wide quartz-tourmaline-(calcite) veins are the main carrier of the ore. Tourmaline commonly forms accumulations of euhedral grains of blue-green pleochroic colour and may in some veins be more abundant than quartz. However, it is rarely observed in veins outside the mineralised zone. Calcite occurs as aggregates and thin bands (). Sulphide mineralisation is mainly associated with tourmaline and calcite in the quartz veins (, M and 8A, D). Scheelite is locally observed associated with quartz ( and ). Minor gangue minerals include microcline and plagioclase, and some veins are overprinted by biotite, chlorite, epidote and sericite. Average grain size of the vein minerals ranges between 0.1 and 1 mm and varies between veins. Generally, quartz, scheelite and the major sulphides (chalcopyrite, pyrite and pyrrhotite) are coarsest, followed by tourmaline and calcite with the remaining minerals at the lower end of the size range.
Figure 8. Transmitted light microphotograph images of veins in the Liikavaara Östra Cu-(W-Au) deposit. A. Quartz-tourmaline-calcite vein with mineralisation of scheelite and sulphides; scheelite occurs with quartz, sulphides with tourmaline. B. Calcite-sulphide vein crosscutting a quartz vein in biotite schist. C. Biotite selvage around a quartz vein. D. Quartz-calcite-sulphide vein in biotite schist. Note: 9a taken with parallel polarisers, rest crossed polarisers. Abbreviations: Bt – biotite, Cal – calcite, Qz – quartz, Sch – scheelite, Sulp – sulphide, Tour – tourmaline.
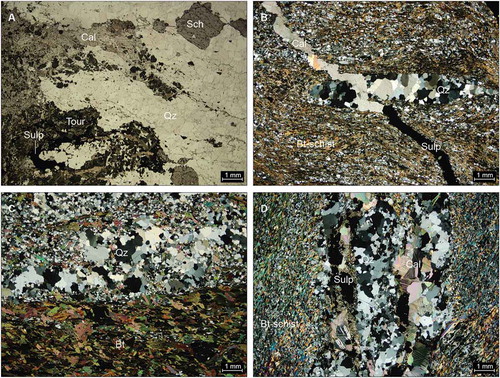
Veinlets of calcite, ranging in width and grain size from a few microns to millimetres, locally occur in the deposit (). They are equigranular and largely mono-mineralic with the occasional occurrence of chalcopyrite, sphalerite and galena. The veinlets are planar, mostly sharp and cross-cut host and wall rocks, aplite dykes, quartz veins and mineralisation ().
Alteration
The rocks of the Liikavaara Östra Cu-(W-Au) deposit are altered and metamorphosed. Most abundant is pervasive biotitisation where biotite replaces amphibole in the host and wall rocks (–F and 8 C, D). Vein-related biotitisation, in places accompanied by tourmalinisation and epidotisation, form selvages in the host rock at the contacts to veins and fissures (, K and 8 C). Biotitisation is also observed in the aplite dykes together with muscovitisation replacing feldspar (, J). The micas mainly form a lepidoblastic texture. Secondary biotite is partly replaced by chlorite which also forms thin veinlets that cut the foliation. Calcite and epidote are frequent but less abundant alteration minerals dispersed in the rocks and similar in grain size to the matrix minerals. Epidote forms both anhedral and euhedral grains and commonly replaces feldspar phenocrysts. Sericite also replaces plagioclase phenocrysts and lepidoblastic secondary biotite but is more pervasive than epidote (, F). Especially in the hanging wall and footwall, sericitisation can affect areas up to tens of centimetres (). Red K-feldspars locally stain the rocks.
Ore minerals at Liikavaara Östra
Chalcopyrite is the dominant Cu-rich mineral and most abundant sulphide in the ore zone. It shows anhedral growth and ranges in grain size from a few microns to several centimetres. Chalcopyrite is often intergrown with pyrrhotite (, D, F). Association with and replacement of pyrite is also common (, E). Sphalerite and galena often rim chalcopyrite and pyrrhotite grains while the latter also form inclusions in sphalerite (chalcopyrite/pyrrhotite disease). Other associations of chalcopyrite in veins include scheelite, molybdenite and marcasite as well as the gangue phases quartz, calcite and tourmaline (, C, E, F). Chalcopyrite often fills interstices in tourmaline aggregates in veins (). Remobilised or a second generation of chalcopyrite is also found in late stage calcite veinlets ().
Figure 9. Reflected light (G–H) and backscattered electron (I, J) images of the mineralisation at the Liikavaara Östra Cu-(W-Au) deposit. A. Chalcopyrite and pyrrhotite surrounded by calcite in a quartz vein. B. Pyrite and pyrrhotite with quartz in aplite. C. Skeletal pyrite and marcasite replacing chalcopyrite associated with pyrrhotite and scheelite in a quartz vein. D. Assemblage of chalcopyrite, pyrrhotite, sphalerite and galena in a quartz-tourmaline-calcite vein. E. Molybdenite associated with chalcopyrite, pyrite and pyrrhotite in a quartz vein. F. Scheelite grains partly associated with chalcopyrite and pyrrhotite in a quartz vein within aplite. G. Disseminated chalcopyrite, pyrite, pyrrhotite and magnetite in biotite schist. H. Intergrowth of a Bi-telluride and a Bi-Te-sulphide next to sphalerite in a quartz vein. I. Cassiterite inclusions at the edge of pyrite next to biotite. J. Euhedral uraninite with calcite and galena in a chalcopyrite-filled crack within scheelite. Abbreviations: Cal – calcite, Ccp – chalcopyrite, Cst – cassiterite, Gn – galena, Mcs – marcasite, Mgt – magnetite, Mol – molybdenite, Po – pyrrhotite, Py – pyrite, Qz – quartz, Sch – scheelite, Sp – sphalerite, Urn – uraninite.
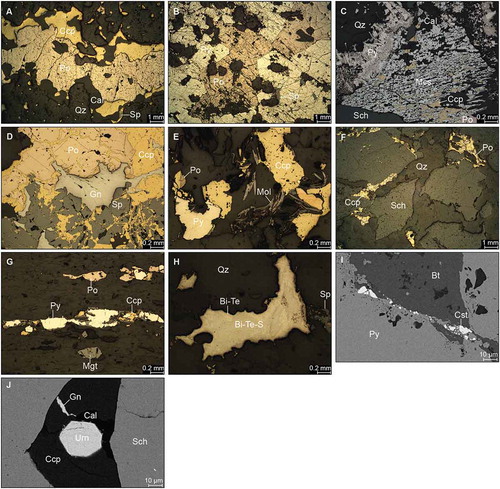
Pyrite is another major sulphide within the ore zone. It is commonly developed in the quartz-dominated parts of quartz-(±tourmaline-calcite) veins. Similarly to chalcopyrite, pyrite crystals range from micron to centimetre size (<50 µm–15 mm). Pyrite grains form at least two generations. Older and larger an- to subhedral grains often show partial replacement by chalcopyrite and pyrrhotite (, E). Some are thinly rimmed by chlorite. Younger and smaller sub- to euhedral grains occur isolated, as inclusions in pyrrhotite, or intergrown with marcasite, chalcopyrite and pyrrhotite. Skeletal growth texture is common ().
Pyrrhotite is the third major sulphide. It is similar in form, size and distribution to chalcopyrite. However, it does not occur in late stage calcite veinlets. Pyrrhotite is generally associated with most ore minerals although its occurrence together with chalcopyrite and pyrite, as described above, is most common (, B, D, F).
Sphalerite and even more so galena are less abundant sulphide phases. Both are mainly anhedral although euhedral grains are locally observed. Their grain size is smaller than that of the major sulphides and do usually not exceed half a millimetre. Sphalerite and galena predominantly occur together and often rim chalcopyrite-pyrrhotite grains (, B, D). Sphalerite is often associated with scheelite as rims, crack infillings and in small veinlets together with chalcopyrite. It also fills interstices in tourmaline aggregates together with chalcopyrite and pyrrhotite. Galena shows replacement of other sulphide phases such as marcasite. Sphalerite and galena are found in the late-stage calcite veinlets along with chalcopyrite.
Marcasite is only locally observed. It commonly replaces and fills cracks in pyrrhotite and is intergrown with chalcopyrite and pyrite. It is partly replaced by galena. Marcasite is developed in a characteristic ‘zebra-like’ texture ().
Scheelite is the only W-mineral found and a minor ore mineral in the deposit. It has not been observed disseminated in the major host rocks but seems to be confined to the quartz-(±tourmaline-calcite) veins, commonly in the quartz-dominated part of veins both within the host rock and intrusive dykes (). Scheelite mainly forms large (several millimetres in width) an- to subhedral single grains (), aggregates of small (tenths of micrometres) rounded grains and locally disseminated fine (<0.2 mm) anhedral grains. Undulose extinction is common. It is associated with quartz, calcite and sulphides (especially chalcopyrite, sphalerite and pyrrhotite; ). These minerals rim the scheelite grains and fill fractures and holes. Cross-cutting veinlets of calcite, chalcopyrite and sphalerite as well as chlorite are locally observed.
Molybdenite is the only Mo-phase and rather rare. It forms characteristic bended laths, and grains are commonly ~0.2 mm and smaller (). Intergrowths with other sulphides are not so common, although some association with pyrrhotite, chalcopyrite and scheelite is observed (). Few grains and small aggregates are disseminated in quartz-(±tourmaline-calcite) veins or developed along their rims, generally in the quartz-dominated parts ().
Magnetite is only locally observed. It occurs predominantly disseminated in the host rocks as subhedral, partly fractured grains, 0.2–0.4 mm in size (). In places, it is enriched as a second generation in quartz veins together with chalcopyrite and pyrite. Grains are generally isolated or associated with pyrite and chalcopyrite, commonly being replaced by these ().
Anhedral and irregular-shaped grains of ilmenite are scarcely observed in the quartz veins. They are elongated, oriented and up to a few millimetres in length. Titanite replaces ilmenite forming rims.
Cassiterite is the only Sn-bearing mineral observed. It occurs in groups of very fine (<10 µm), rounded anhedral grains at grain boundaries between pyrite and biotite and within chalcopyrite ().
One sample of a quartz vein in biotite schist (, drill core 370, 253.3 m) contains traces of uraninite. Uraninite forms sub- to euhedral grains, 10–30 µm in size and occurs associated with chalcopyrite, scheelite, and quartz ().
Minerals of Au, Ag, Bi and Te at Liikavaara Östra
The following description of the mineralogy of Au, Ag, Bi and Te (~10 ppm and less on average) is based on SEM-EDS analysis (see the method section). Since no proper quantitative analysis and complimentary crystallographic analysis were done, exact identification was partly not possible.
Based on geochemical data of drill cores from Boliden AB, Bi is a common trace metal in the deposit. Its mineralogy is diverse and includes native Bi, Bi-tellurides and Bi-sulphides (, B, C). Their compositions are variable and partly include other elements such as Ag, Pb and Se (, D). The Bi-minerals are found in quartz-(±tourmaline-calcite) veins and aplite dykes.
Figure 10. Backscattered electron images of various Bi, Ag and Au minerals in the Liikavaara Östra Cu-(W-Au) deposit. A. Intergrowth of a Bi-telluride and hessite at the edge of chalcopyrite and associated with calcite. B. Intergrowth of hessite, a Bi-telluride and a Bi-Ag-telluride in chalcopyrite next to native Bi. C. Inclusion of native Bi and a Bi-sulphide associated with chalcopyrite in pyrite. D. Disseminated native Bi and Bi-Te-Se associated with chalcopyrite and magnetite. E. Droplet-shaped grains of native Bi with some gold in quartz. F. Hessite at the edges of arsenopyrite intergrown with sphalerite. G. An Ag-sulphide at the border of chalcopyrite and associated with calcite and chlorite. H. Electrum in a crack in pyrite and inclusion of native Bi with chalcopyrite. Abbreviations: Apy – arsenopyrite, Cal – calcite, Ccp – chalcopyrite, Chl – chlorite, Ele – electrum, Hes – hessite, Mgt – magnetite, Po – pyrrhotite, Py – pyrite, Qz – quartz, Sp – sphalerite, Tour – tourmaline.
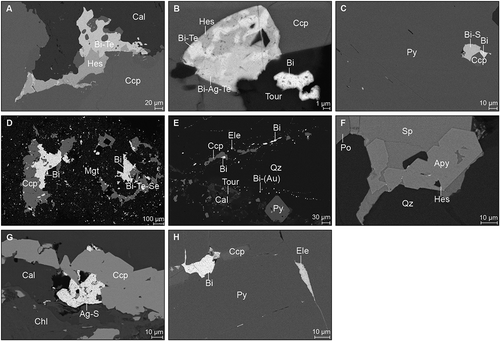
Bi-tellurides are commonly intergrown with hessite (Ag2Te) and in places with an additional Bi-Ag-telluride (), and occur as an- to subhedral inclusions, within cracks and along the border of pyrrhotite, pyrite, chalcopyrite, sphalerite and molybdenite (, B). At grain boundaries they are associated with calcite, tourmaline, sphalerite, and magnetite. Grains are generally <50 µm and most even <20 µm.
In a section of quartz veins in aplite, where Bi is high according to analyses, 0.2–0.5 mm grains of a Bi-Te-sulphide rimmed by a Bi-telluride are found (). The Bi-Te-sulphide can also be found alongside Bi-tellurides in molybdenite and with hessite in sphalerite.
Native Bi and Bi-sulphides are often intergrown with each other but also occur individually (). Grains are interspersed in chalcopyrite, pyrite, pyrrhotite, biotite and quartz and associated with chlorite, feldspar, sphalerite and magnetite. Native Bi is also observed together with hessite, electrum and Bi-tellurides (). Grains of native Bi and Bi-sulphides are anhedral and mainly <10 µm. One sample contains <5 µm droplet-shaped grains of native Bi with Au in quartz ().
Hessite is the most commonly observed Ag-mineral. It is mostly intergrown with Bi-(Ag)-tellurides but also occurs with native Bi and singularly (, B). Hessite is also found associated with scarce arsenopyrite (). Hessite grains are mostly <20 µm. Ag-sulphide occurs as <30 µm grains at the border of chalcopyrite ()
Silver is also found in electrum, the main Au-mineral observed. Electrum occurs as very fine (<10 µm) and often elongated grains in quartz veins and aplite dykes. The grains are commonly found as inclusions in quartz and in cracks or holes in pyrite, pyrrhotite, molybdenite and chalcopyrite, often with native Bi (, H). Electrum is also observed associated with chlorite, tourmaline and sphalerite next to Bi-tellurides.
Geochronology
The granodiorite intrusion in the footwall northeast of the Liikavaara Östra Cu-(W-Au) deposit was dated as it is suggested in this study to be linked to the genesis of the deposit. The mineralogy of the intrusion is described in the logging and petrography section and chemical data is presented in . The zircon grains extracted from the intrusion are 50–200 µm in size, stubby to elongated in shape and partly rounded and broken (). Fractures, holes and oscillatory zoning are common features and many fractured grains show a partially dark core (in BSE images) occasionally with inclusions of nanometre-sized Pb-phases.
5.6. A. Backscattered electron images of zircons. Ages marked with “**” are corrected for common Pb. The crystals are sub- to euhedral, stubby to elongated in shape and roughly 50–200 µm in size. Crystals 1–5 and 7 show oscillatory zoning. In crystals 2, 3 and 5 spots intersect several zones close to the rim of the crystals and give mixed discordant 207Pb–206Pb ages younger than the crystallisation age of the unit. In crystals 1, 2, 4, and 5 spots in the bright part of the core provide concordant 207Pb–206Pb ages close to the crystallisation age of the unit. For Crystals 4–8 the BSE images show a dark area in the central part of the core with cracks originating from it. Spots in these areas give discordant 207Pb–206Pb ages younger than the crystallisation age of the unit and often younger than the zoned rims. Crystal 8 has a spot in the bright part of the core of discordant age. This is due to overlap with a fracture. The same crystal shows inclusion of white Pb-phases in the dark part of the core. These Pb-phases when intersected by an analysis cause erroneous data due to IC tripping. B. Reverse concordia (Tera-Wasserburg) diagram with intercept ages for the discordia covering 66 analyses from various areas within 58 zircons. C. Concordia age calculation based on 20 concordant analyses from spots in the bright area in the cores of the zircons. D. Calculated 207Pb–206Pb weighted average age of the same 20 analyses as in C.
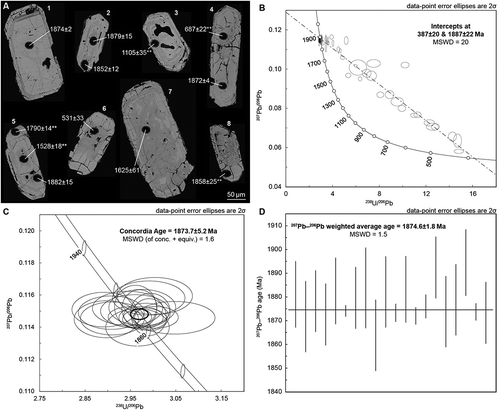
In total, analysis covered 79 spots in 68 zircons. This comprises 56 crystals analysed with single spot analyses, 10 crystals with two-spot analyses, and one crystal with three-spot analysis to study the core-rim relationship in zoned crystals. Thirteen measurements in cores with Pb-phase inclusions caused IC tripping and consequently erroneous data that were discarded. Common Pb correction was necessary for 27 spots. Plotting of the data (66 non-erroneous analyses) in an inverse concordia (Tera–Wasserburg) diagram outlines a discordia with an upper intercept age of 1887 ± 22 Ma and a lower intercept age of 387 ± 20 Ma with a MSWD of 20 ().
Of the 66 non-erroneous analyses only 20 are concordant. These give 207Pb–206Pb ages between 1895–1864 Ma and are measured in bright cores (in BSE images) or the bright part of partially dark cores (). Spots intersecting fractures exhibit near-concordant to discordant ages both younger and older than the concordant data. Stronger discordancy is displayed by spots intersecting oscillatory zoning and analyses of dark cores, both commonly younger than the concordant ages (). For the latter, discordant 207Pb–206Pb ages range between 424–1729 Ma.
A crystallisation age was calculated from the 20 concordant analyses from the bright cores. A concordia age yields 1873.7 ± 5.2 Ma with a MSWD (of concordance and equivalence) of 1.6 (). A 207Pb–206Pb weighted average age of the same analyses yields 1874.6 ± 1.8 Ma with a MSWD of 1.5 ().
Discussion
Host and wall rock origins
The character and origin of the wall rocks at the Liikavaara Östra Cu-(W-Au) deposit have previously been discussed. Zweifel (Citation1976) suggested a volcanic origin and classified the rocks as epiclastic based on the observations of sedimentary structures like cross- and graded bedding and conglomerates. Estholm (Citation2014) agreed on a volcanic origin but suggested the wall rocks to instead be volcaniclastic. She based this on consistent chemical classification into andesite and basalt, on remnants of euhedral plagioclase phenocrysts, and on the occurrence of epidote, plagioclase and amphibole as common minerals in metabasalts.
Findings of this study support an igneous origin of the wall rocks suggested by both Zweifel (Citation1976) and Estholm (Citation2014). Geochemical data clearly show an andesitic-basaltic composition of the footwall rocks and an andesitic composition of the hanging wall rocks (, C, D). A volcaniclastic character of the wall rocks is favoured based on the dominant porphyritic texture with partly euhedral phenocrysts and varying abundance of several centimetre large clasts with mineralogy similar to that of the surrounding rock. Apparent sedimentary features like graded bedding could be caused by a variation in the intensity of eruption and pyroclastic flow but may also be the result of sub-aqueous re-working.
The host rock to the mineralisation is geochemically identified to be of basaltic composition (, C, D). Its original texture is obscured by hydrothermal alteration and metamorphism which has turned it into biotite-amphibole schists and gneisses. However, more pristine areas show fine-grained (<1 mm) euhedral plagioclase laths and amphibole phenocrysts. Due to its thickness in drill cores (~200 m), we hypothesize that the protolith was a dolerite sill, though an argument for an extrusive volcanic product could also be made. Zweifel (Citation1976) interpreted the amphibolitic rocks as metamorphosed dolomitic sediments. This is not supported by the chemistry in this study. Additionally, if it was an alteration product of a dolomite it should have turned into skarn, but no calc-silicate minerals have been recorded.
Granodiorite intrusion in footwall and aplite dykes
The footwall intrusion has previously been described as granite (Zweifel Citation1976). Petrographic observations from this study favour a classification as granodiorite based on the normative plagioclase to K-feldspar ratio. However, the geochemical and the normative data are not unequivocal and range between a classification as granite and granodiorite (, G). The dykes are termed aplite due to their granitic composition in both chemistry and mineralogy but much finer grain size. The stratigraphically higher emplacement of the dykes and their chemical, mineralogical and textural similarity to the footwall intrusion, albeit being slightly more felsic and fine-grained, permit that they are related to the footwall intrusion. In this scenario, slightly more fractionated magma intruded from the footwall intrusion into the overlying rocks and crystallised as dykes. Later, they were deformed which is displayed by varying intensity of biotite/muscovite foliation.
Hydrothermal alteration
The wall and host rock in Liikavaara are strongly altered by biotitisation, sericitisation, and chloritisation. This can partly be explained by mineral alteration of e.g. primary pyroxene, amphibole, feldspar, and biotite. However, chemical data suggests an additional influx of potassium to the host rock and dykes (). Also, the occurrences of veins rich in quartz, calcite, tourmaline and sulphides need an external source to explain the high contents of Si, Ca, B and metals. Sammelin et al. (Citation2011) interpreted the Cu-mineralisation to be related to saline and CO2-bearing fluids. This and a weak dissemination of sulphides in the aplite dykes and the footwall intrusion possibly suggest magmatic-hydrothermal fluids (Roedder Citation1971; Nash Citation1976; Richards Citation2011) derived from the degassing granodiorite intrusion and aplite dykes. The high density of mineralised veins, especially in proximity to the dykes, supports an idea of the fracture system related to the dyke emplacement to have acted also as major pathways for these magmatic-hydrothermal fluids to ascend and penetrate the surrounding rocks.
Ore mineral paragenesis
A paragenetic sequence for ore mineralisation based on our petrographic studies is presented below and summarised in . Magnetite and hematite appear to be the oldest ore minerals due to their predominantly disseminated character in the wall and host rocks rather than occurring in veins. Magnetite also shows replacement by sulphides. However, some occurrences of sub- to euhedral magnetite in the quartz veins suggest a second generation too.
Figure 12. Paragenetic sequence of mineralisation at the Liikavaara Östra Cu-(W-Au) deposit. Approximate timing of quartz-tourmaline-calcite veins and calcite veins is indicated. Mineralisation earlier than veins is found disseminated in the host rock.
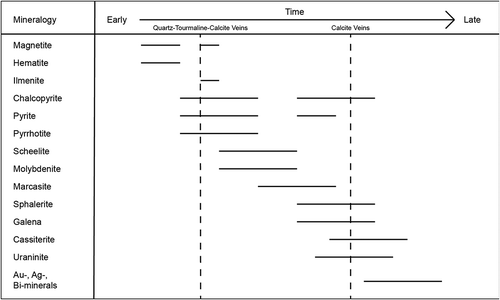
Ilmenite is scarce in the deposit; it is largely replaced by titanite and only shows some association with scheelite. Since there are no signs of it being younger than other ore minerals, it is grouped with the secondary vein magnetite.
The major sulphides (chalcopyrite, pyrrhotite and pyrite) are tightly intergrown and likely of similar age, although pyrite and chalcopyrite appear to have formed in two generations. The first generation of pyrite crystallised together with chalcopyrite and pyrrhotite, the second came somewhat later forming skeletal, often sub- to euhedral grains also in quartz-(±tourmaline-calcite) veins. Chalcopyrite occurs in the host rock, in quartz-tourmaline veins but also in crosscutting calcite veinlets and thus appears in most phases of ore genesis.
Scheelite grains are large and partly subhedral. They only occur in quartz-(±tourmaline-calcite) veins and are often rimmed by other sulphides and cut by calcite, chalcopyrite, sphalerite, and chlorite. Scheelites crystallisation probably started later than that of the major sulphides but ended before the introduction of sphalerite, the second generation of chalcopyrite and calcite veins.
The mineralisation of molybdenite is similar to that of scheelite. It is not as abundant but intergrowths with other sulphides exist. There are no particular signs of either a relatively early or late crystallisation.
Marcasite likely formed from pyrrhotite and was observed intergrown with skeletal pyrite
Sphalerite and galena often occur together. They rim and replace other ore minerals and fill cross-cutting calcite veins. Consequently, it appears that sphalerite and galena formed late in the paragenetic sequence. This is also true for other trace metals.
Uraninite forms euhedral grains and occurs as inclusions in chalcopyrite and in cracks of scheelite. Cassiterite and the Bi-, Au- and Ag-bearing minerals occur dominantly as inclusions and crack fillings in the major and minor sulphides.
Overall, the paragenetic sequence at Liikavaara is consistent with the sequence proposed for the nearby Aitik deposit (Wanhainen et al. Citation2005) – early magnetite followed by the major sulphides and later the minor phases.
Regarding the mineralogy of the trace metals, the occurrence and association of Au-Ag-Bi-tellurides/selenides is not uncommon in ore deposits and has been reported for numerous deposits of various types including epithermal Au-Te systems, deposits of the porphyry-epithermal continuum, intrusion-related Au deposits, gold skarns, and Archaean orogenic gold deposits (Cook & Ciobanu Citation2005; Cook et al. Citation2009). Observations from ore deposits (Cook et al. Citation2009 and references therein), experimental work (Douglas et al. Citation2000; Tooth et al. Citation2011) and thermodynamic modelling (Wagner Citation2007; Tooth et al. Citation2008) have shown Bi-melt to be a powerful scavenger for Au in ore-forming systems and its capability to form economic Au deposits from Au-undersaturated aqueous fluids. An overlap of the formation conditions of particularly skarn, intrusion-related and orogenic Au deposits with the temperature range (234–475 °C) of the 10 eutectics in the Au-Bi-Te system was discussed by Ciobanu et al. (Citation2005), and Ciobanu et al. (Citation2006) observed that generation of Bi-melt is initiated at conditions as low as upper greenschist facies. The occurrence of Au together with Bi-tellurides, in many cases as droplets or droplet-derived patches hosted within common ore minerals, was described by Ciobanu & Cook (Citation2002) for various telluride-enriched Au deposits.
The Liikavaara Östra Cu-(W-Au) deposit is suggested to be genetically related to its footwall intrusion and affected by the 1.8 Ga metamorphism. Hence, conditions at Liikavaara may have been suitable for Bi-melt generation. Gold and Bi-minerals in Liikavaara are not always directly associated, but the two metals and Ag show similar enrichment patterns and are found proximal to each other. Furthermore, droplet-shaped grains of native Bi with partition of Au were observed (see ). It is therefore possible that a Bi-melt played some role in Au scavenging and distribution in the Liikavaara Östra deposit.
A tectonic foliation is prevalent in wall and host rocks at Liikavaara but not in the footwall intrusion. Although clasts in the wall rocks are aligned with the foliation their inner texture seems unaffected by deformation. Foliation is especially pronounced by biotite schistosity in the host rock ( and ). To some extent it also affects the aplite dykes. Several aplite dykes contain lepidoblastic muscovite oriented parallel to the metamorphic foliation in the wall and host rocks (). Veins are parallel to foliation in the biotite schist or have a ductile character and some veins have selvages of biotite (–M). Cross-cutting veins of especially calcite occur too, which indicates different generations of veins (). Disseminated sulphides and magnetite in the host rock are aligned in direction of foliation (). In contrast, ore minerals in veins are partly elongated along the vein but no strict orientation is observed. Regionally, a tectonic foliation is observed in other deposits, like Aitik and Nautanen (Martinsson & Wanhainen Citation2004), within the Nautanen Deformation zone, and is probably related to the Svecofennian orogeny. At least two compressional events at 1.88 Ga and 1.80 Ga have been recorded (Bergman et al. Citation2001; Wanhainen et al. Citation2012; Martinsson et al. Citation2016; Lynch et al. Citation2018).
The granodiorite intrusion at Liikavaara has a U-Pb zircon age of c. 1.88–1.87 Ga and thus does not support Zweifel’s (Citation1976) interpretation as of Lina type. Instead, its age overlaps with those of the Haparanda and the Perthite Monzonite suites of intrusions (c. 1.89–1.86 Ga; Bergman Citation2018). This means that ore formation at Liikavaara Östra occurred after the 1.88 Ga compressional event. It also demonstrates that intrusion, alteration, veining and mineralisation are pretectonic to the 1.80 Ga compressional event which subsequently caused reactivation and redistribution of veins and mineralisations.
Timing of mineralisation with respect to regional tectonothermal events
The 1.87 Ga granodiorite is slightly younger than the 1.89 Ga quartz monzodiorite responsible for formation of the Aitik Cu-Au deposit (Wanhainen et al. Citation2006). Compared to Aitik, the mineralised zone in Liikavaara is enriched in Zn, Pb, Bi, Mo, Sn and most notably W (Zweifel Citation1976; this study). Additionally, the abundance of calcite and different mineralisation style distinguish it from Aitik. Metals such as W, Mo and Sn are usually derived from crustal rocks (Candela & Piccoli Citation2005; Seedorf et al. Citation2005) and porphyry deposits containing these metals are commonly associated with high-silica (72–77 wt.% SiO2), and strongly differentiated granitic plutons (Mutschler et al. Citation1981; White et al. Citation1981; Kooiman et al. Citation1986; Xunfan et al. Citation1988). The more evolved composition of the granodiorite intrusion at Liikavaara compared to the quartz monzodiorite at Aitik may therefore be an explanation to this difference in metal association of the mineralisation.
Conclusions
The Liikavaara Östra Cu-(W-Au) deposit is suggested to be an intrusion-related vein-style deposit. At this stage of research we hypothesize that the formation of the deposit can be connected to the emplacement of the 1.87 Ga granodiorite intrusion occurring in the footwall of the deposit. However, this model should be better constrained by further age and other geochemical data (e.g., stable and radiogenic isotopes) for the hydrothermal mineralisation. Metre-wide aplite dykes, potentially related to the footwall intrusion, cross-cut biotite-amphibole schists and gneisses. The schists and gneisses occur between metavolcaniclastic rocks of andesitic (hanging wall) to basaltic (footwall) composition. The rocks are altered by biotite, muscovite, calcite, epidote, tourmaline, sericite and K-feldspar. The mineralisation is hosted by quartz-tourmaline-calcite veins and younger calcite veins that cross-cut the aplite dykes and the host and wall rocks. Minor dissemination of some minerals (chalcopyrite, pyrite, pyrrhotite and magnetite) is also observed in the host rock and the aplite dykes.
Spatial distribution of the mineralisation is controlled by the aplite dykes and the veins. Chalcopyrite, pyrite and pyrrhotite are the main ore minerals followed by sphalerite, galena, magnetite, marcasite, scheelite and molybdenite. Ilmenite is locally observed. A number of trace metals exist including Bi, Ag, Au and Sn. They are most commonly bound in Bi-phases, hessite, electrum and cassiterite, respectively. Intergrowths, inclusions and replacements between ore minerals are common, especially for the major and minor sulphides. Minerals of Au, Ag and Bi are mainly <20 µm and occur as inclusions, along the borders, in cracks and in holes within the major sulphides, sphalerite, molybdenite and quartz.
Vegacenter_Data.xlsx
Download MS Excel (752.9 KB)Acknowledgments
This work was carried out as part of a doctoral project financed by CAMM2 (Centre for Advanced Mining & Metallurgy, Luleå University of Technology) and Boliden AB. The authors acknowledge no financial interest or benefit arising from direct application of their research. We thank Dr. Ellen Kooijman and Melanie Schmitt at the Vegacenter micro-analytical facility at the Swedish Museum of Natural History in Stockholm, Sweden for their co-operation and aid with U-Pb dating and Dr. Kjell Billström of the Swedish Museum of Natural History in Stockholm for his input in radiometric data interpretation. This is Vegacenter publication #026. Thanks to Sofia Höglund at the Exploration Department, Boliden AB, for her help with drill core logging and logistics during the first sampling session. Further thanks to Nils-Johan Bolin at Boliden AB for fruitful discussions. We wish to thank our LTU colleague Zmar Sarlus for instruction on zircon separation and Glenn Bark for training on the SEM. We appreciate the in-depth discussions with Dr. Thomas Riegler on earlier versions of the manuscript, and Drs. Edward Lynch and Ferenc Molnar are thanked for reviewing and improving the manuscript.
Disclosure statement
No potential conflict of interest was reported by the authors.
Data availability statement
The chemical data from Boliden AB that support the findings of this study are available from Boliden AB. Restrictions apply to the availability of these data, which were used under licence for this study. Other chemical data that support the findings of this study are available from the corresponding author, M.W., upon reasonable request. The authors confirm that the geochronological data supporting the findings of this study are available within the article or its supplementary materials.
Supplemental data
Supplemental data for this article can be accessed here.
References
- Bergman, S., 2018: Regional geology of northern Norrbotten County. In S. Bergman (ed.): geology of the Northern Norrbotten ore province, northern Sweden. Sveriges geologiska undersökning. Rapporter och meddelanden 141, 9–17.
- Bergman, S., Kübler, L. & Martinsson, O., 2001: Description of regional geological and geophysical maps of northern Norrbotten County (east of the Caledonian orogen). Sveriges Geologiska Undersökning Ba, 56, 110.
- Candela, P.A. & Piccoli, P.M., 2005: Magmatic processes in the development of porphyry-type ore systems. InJ.W. Hedenquist, J.F.H. Thompson, R.J. Goldfarb, & J.R. Richards (eds.): Economic geology 100th anniversary volume (pp 25–37). Littleton: Society of Economic Geologists.
- Ciobanu, C.L. & Cook, N.J., 2002: Tellurides, selenides (and bi-sulphosalts) in gold deposits. In L.J. Robb & R. Montjoie (eds.): Abstract Volume of the 11th quadrennial IAGOD symposium and Geocongress, Windhoek, Namibia, 22–26 July 2002.
- Ciobanu, C.L., Cook, N.J., Damian, F. & Damian, G., 2006: Gold scavenged by bismuth melts: an example from Alpine shear-remobilizates in the Highiş Massif, Romania. Mineralogy and Petrology, 87(3–4), 351–384. doi:10.1007/s00710-006-0125-9.
- Ciobanu, C.L., Cook, N.J. & Pring, A., 2005: Bismuth tellurides as gold scavengers. In J. Mao & F.P. Bierlein (eds.): Mineral deposit research: meeting the global challenge (pp. 1383–1386). Berlin, Heidelberg: Springer. doi:10.1007/3-540-27946-6_352.
- Cook, N.J. & Ciobanu, C.L., 2005: Tellurides in Au deposits: implications for modelling. InJ. Mao & F.P. Bierlein (eds.): Mineral deposit research: meeting the global challenge (pp. 1387–1390). Berlin, Heidelberg: Springer. doi:10.1007/3-540-27946-6_353.
- Cook, N.J., Ciobanu, C.L., Spry, P.G. & Voudouris, P., 2009: Understanding gold-(silver)-telluride-(selenide) mineral deposits. Episodes, 32(4), 249–263. doi:10.18814/epiiugs/2009/v32i4/002.
- De La Roche, H., Leterrier, J.T., Grandclaude, P. & Marchal, M., 1980: A classification of volcanic and plutonic rocks using R1R2-diagram and major-element analyses — its relationships with current nomenclature. Journal of Chemical Geology, 29(1–4), 183–210. doi:10.1016/0009-2541(80)90020-0.
- Douglas, N., Mavrogenes, J., Hack, A. & England, R., 2000: The liquid bismuth collector model: an alternative gold deposition mechanism. In C.G. Skillbeck & T.C.T. Hubble (eds.): Searching for a sustainable future: 15th Australian Geological Convention, Sydney, 3–7 July 2000, 135 pp.
- Estholm, M., 2014: Identification of the host rocks and the environment of formation of the Liikavaara Cu-Au deposit, Norrbotten, Sweden. Bachelor thesis, Stockholm University, Stockholm, Sweden. 32 pp.
- European Commission. 2017: Study on the review of the list of critical raw materials. EU Publications, Final report, 93 pp.
- Hallberg, J.A., 1984: A geochemical aid to igneous rock type identification in deeply weathered terrain. Journal of Geochemical Exploration, 20(1), 1–8. doi:10.1016/0375-6742(84)90085-2.
- Hollocher, K., 2004: CIPW norm calculation program. New York: Geology Department, Union College.
- Hughes, C.J., 1973: Spilites, keratophyres, and the igneous spectrum. GeologicalMagazine, 109(6), 513–527. doi:10.1017/S0016756800042795.
- Irvine, T.N.J. & Baragar, W.R.A.F., 1971: A guide to the chemical classification of the common volcanic rocks. Canadian Journal of Earth Sciences, 8(5), 523–548. doi:10.1139/e71-055.
- Kooiman, G.J.A., McLeod, M.J., & Sinclair, W.D., 1986: Porphyry tungsten-molybdenum orebodies, polymetallic veins and replacement bodies, and tin-bearing greisen zones in the Fire Tower Zone, Mount Pleasant, New Brunswick. Economic Geology, 81(6), 1356–1373. doi:10.2113/gsecongeo.81.6.1356.
- Kuno, H., 1968: Differentiation of basaltic magmas. In H.H. Hess & A. Poldervaart (eds.): Basalts; the poldervaart treatise on rocks of basaltic composition (pp. 623–688). New York: Interscience.
- Lahtinen, R., Korja, A. & Nironen, M., 2005: Paleoproterozoic tectonic evolution. In M. Lehtinen, P.A. Nurmi, & O.T. Rämö (eds.): Precambrian geology of finland – key to the evolution of the Fennoscandian shield (pp. 481–532). Amsterdam: Elsevier B.V.. doi:10.1016/S0166-2635(05)80012-X.
- Lahtinen, R., Korja, A., Nironen, M. & Heikkinen, P., 2009: Palaeoproterozoic accretionary processes in Fennoscandia. Geological Society, London, Special Publications, 318(1), 237–256. doi:10.1144/SP318.8.
- Le Maitre, R.W., Bateman, P., Dudek, A., Keller, J., Le Bas, L., Sabine, M.J., Schmid, P.A., Sorensen, R., Streckeisen, H., Wooley, A. & Zanettin, B., 1989: A Classification of Igneous Rocks and Glossary of Terms (pp. 193). Oxford: Blackwell.
- Ludwig, K.R., 2003: Isoplot 3.00: A Geochronological Toolkit for Microsoft Excel. (Vol. 4, pp. 70). Berkeley: Berkeley Geochronology Center Special Publication.
- Lynch, E.P., Bauer, T.E., Jönberger, J., Sarlus, Z., Morris, G.A. & Persson, P.-O., 2018: Petrological and structural character of c. 1.88 Ga meta-volcanosedimentary rocks hosting iron oxide-copper-gold and related mineralisation in the Nautanen–Gällivare area, northern Sweden. In S. Bergman (ed.): geology of the Northern Norrbotten ore province, northern Sweden. Sveriges geologiska undersökning. Rapporter Och Meddelanden, 141, 107–149.
- Lynch, E.P., Jönberger, J., Bauer, T.E., Sarlus, Z. & Martinsson, O., 2015: Meta-volcanosedimentary rocks in the Nautanen area, Norrbotten: preliminary lithological and deformation characteristics, Barents project 2014. Sveriges Geologiska Undersökning, Rapport, 30, 51.
- Malmqvist, D. & Parasnis, D.S., 1972: Aitik: geophysical documentation of a third-generation copper deposit in north Sweden. Geoexploration, 10(3), 149–200. doi:10.1016/0016-7142(72)90030-0.
- Martinsson, O., 1997: Paleoproterozoic greenstones at Kiruna in northern Sweden: a product of continental rifting and associated mafic-ultramafic volcanism. In O. Martinsson (ed.): Tectonic setting and metallogeny of the Kiruna Greenstones. Ph. D. thesis, Luleå University of Technology, Luleå, Sweden. 49 pp.
- Martinsson, O., 2004: Geology and metallogeny of the Northern Norrbotten Fe-Cu-Au Province. Society of Economic Geology, 33, 131–148.
- Martinsson, O., Billström, K., Broman, C., Weihed, P. & Wanhainen, C., 2016: Metallogeny of the northern Norrbotten ore province, northern Fennoscandian Shield with emphasis on IOCG and apatite-iron ore deposits. Ore Geology Reviews, 78, 447–492. doi:10.1016/j.oregeorev.2016.02.011.
- Martinsson, O. & Perdahl, J.A., 1995: Paleoproterozoic extensional and compressional magmatism in northern Sweden. In J.A. Perdahl (ed.): Svecofennian volcanism in northern Sweden. Ph. D. thesis, Luleå University of Technology, Luleå, Sweden. 169 pp.
- Martinsson, O. & Wanhainen, C., 2004: Character of Cu-Au mineralizations and related hydrothermal alterations along the Nautanen deformation zone, Gällivare area, northern Sweden. Society of Economic Geology, 33, 149–160.
- Melezhik, V.A. & Hanski, E.J., 2012: Fennoscandia: the first 500 million years of the palaeoproterozoic. In V.A. Melezhik, A.R. Prave, E.J. Hanski, A.E. Fallick, A. Lepland, L.R. Kump, & H. Strauss (eds.): Reading the archive of Earth’s oxygenation: volume 1: the palaeoproterozoic of Fennoscandia as context for the fennoscandian Arctic Russia – drilling early Earth project (pp. 33–179). Berlin, Heidelberg: Springer. doi:10.1007/978-3-642-29682-6.
- Monro, D., 1988: The geology and genesis of the Aitik Cu-Au deposit, Arctic Sweden. Ph. D. thesis, University College, Cardiff, UK. 419 pp.
- Mutschler, F.E., Wright, E.G., Ludington, S. & Abbott, J.T., 1981: Granite molybdenite systems. Economic Geology, 76(4), 874–897. doi:10.2113/gsecongeo.76.4.874.
- Nash, T., 1976: Fluid-inclusion petrology– data from porphyry copper deposits and applications to exploration. US Geologial Survey, Professional Paper 907-D.
- Nironen, M., 1997: The Svecofennian Orogen: a tectonic model. Precambrian Research, 86(1–2), 21–44. doi:10.1016/S0301-9268(97)00039-9.
- Pearce, J.A., 1996: A User’s guide to Basalt Discrimination Diagrams. In D.A. Wyman (ed.): trace element geochemistry of volcanic rocks: applications for massive sulphide exploration. Geological association of Canada. Short Course Notes, 12, 79–113.
- Petrus, J.A. & Kamber, B.S., 2012: VizualAge: A novel approach to laser ablation ICP-MS U-Pb geochronology data reduction. Geostandards and Geoanalytical Research, 36(3), 247–270. doi:10.1111/j.1751-908X.2012.00158.x.
- Richards, J.P., 2011: Magmatic to hydrothermal metal fluxes in convergent and collided margins. Ore Geology Reviews, 40(1), 1–26. doi:10.1016/j.oregeorev.2011.05.006.
- Roedder, E., 1971: Fluid inclusion studies on the porphyry-type ore deposits at Bingham, Utah, Butte, Montana, and Climax, Colorado. Economic Geology, 66(1), 98–120. doi:10.2113/gsecongeo.66.1.98.
- Romer, R.L., Martinsson, O. & Perdahl, J.A., 1994: Geochronology of the Kiruna iron ores and hydrothermal alterations. Economic Geology, 89(6), 1249–1261. doi:10.2113/gsecongeo.89.6.1249.
- Romer, R.L. & Wright, J.E., 1992: U-Pb dating of columbites: A geochronologic tool to date magmatism and ore deposits. Geochimica et cosmochimica acta, 56(5), 2137–2142. doi:10.1016/0016-7037(92)90337-I.
- Sammelin, M., Broman, C., Wanhainen, C. & Martinsson, O., 2011: Origin of Au-rich mineralization in the Aitik Cu–Au deposit and its close surrounding: applications of fluid inclusions in exploration for gold-rich mineralization in the Gällivare area, northern Sweden. InM. Sammelin (ed.): The nature of gold in the Aitik Cu–Au deposit – implications for mineral processing and mine planning. Licentiate thesis, Luleå University of Technology, Luleå, Sweden, 25 pp.
- Sarlus, Z., Andersson, U.B., Bauer, T.E., Wanhainen, C., Martinsson, O., Nordin, R. & Andersson, J.B.H., 2018: Timing of plutonism in the Gällivare area: implications for proterozoic crustal development in the northern Norrbotten ore district, Sweden. Geological Magazine, 155(6), 1351–1376. doi:10.1017/S0016756817000280.
- Seedorf, E., Dilles, J.D., Proffett, J.M., Jr., Einaudi, M.T., Zurcher, L., Stavast, W.J.A., Johnson, D.A. & Barton, M.D., 2005: Porphyry deposits: characteristics and origin of hypogene features. In J.W. Hedenquist, J.F.H. Thompson, R.J. Goldfarb, & J.R. Richards (eds.): Economic geology 100th anniversary volume: society of economic geologists (pp. 251–298).Littleton.
- Stephens, M.B. & Bergman Weihed, J., 2020: About this title - Sweden: lithotectonic framework, tectonic evolution and mineral resources. Geological Society, London, Memoirs, 50(1), 620. doi:10.1144/M50
- Tollefsen, E., 2014: Thermal and chemical variations in metamorphic rocks in Nautanen, Gällivare, Sweden. Master’s thesis, Stockholm University, Stockholm, Sweden. 50 pp.
- Tooth, B., Brugger, J., Ciobanu, C. & Liu, W., 2008: Modeling of gold scavenging by bismuth melts coexisting with hydrothermal fluids. Geology, 36(10), 815–818. doi:10.1130/G25093A.1.
- Tooth, B., Ciobanu, C.L., Green, L., O’Neill, B. & Brugger, J., 2011: Bi-melt formation and gold scavenging from hydrothermal fluids: an experimental study. Geochimica et cosmochimica acta, 75(19), 5423–5443. doi:10.1016/j.gca.2011.07.020.
- Wagner, T., 2007: Thermodynamic modeling of Au–Bi–Te melt precipitation from high temperature hydrothermal fluids: preliminary results. In C.J. Andrew (ed.): Mineral Exploration and Research: Digging Deeper. Proceedings of the 9th Biennial SGA Meeting, Dublin, 20–23 August 2007, 769–772.
- Wanhainen, C., Billström, K. & Martinsson, O., 2006: Age, petrology and geochemistry of the porphyritic Aitik intrusion, and its relation to the disseminated Aitik Cu-Au-Ag deposit, northern Sweden. Geologiska Föreningens I Stockholm Förhandlingar, 128(4), 273–286. doi:10.1080/11035890601284273.
- Wanhainen, C., Billström, K., Martinsson, O., Stein, H., & Nordin, R., 2005: 160 Ma of magmatic/hydrothermal and metamorphic activity in the Gällivare area: Re–Os dating of molybdenite and U–Pb dating of titanite from the Aitik Cu–Au–Ag deposit, northern Sweden. Mineralium Deposita, 40(4), 435–447. doi:10.1007/s00126-005-0006-x.
- Wanhainen, C., Broman, C., Martinsson, O., & Magnor, B., 2012: Modification of a Palaeoproterozoic porphyry-like system: integration of structural, geochemical, petrographic, and fluid inclusion data from the Aitik Cu–Au–Ag deposit, northern Sweden. Ore Geology Reviews, 48, 306–331. doi:10.1016/j.oregeorev.2012.05.002.
- Weihed, P., Arndt, N., Billström, K., Duchesne, J.-C., Eilu, P., Martinsson, O., Papunen, H. & Lahtinen, R., 2005: 8: precambrian geodynamics and ore formation: the Fennoscandian shield. Ore Geology Reviews, 27(1–4), 273–322. doi:10.1016/j.oregeorev.2005.07.008.
- White, W.H., Bookstrom, A.A., Kamilli, R.J., Ganster, M.W., Smith, R., Ranta, D.E. & Steininger, R.C., 1981: Character and origin of Climax-type molybdenum deposits. Economic Geology, 75, 270–316.
- Wiedenbeck, M.A.P.C., Alle, P., Corfu, F., Griffin, W.L., Meier, M., Oberli, F.V., Quadt, A.V., Roddick, J.C. & Spiegel, W., 1995: Three natural zircon standards for U-Th-Pb, Lu-Hf, trace element and REE analyses. Geostandards and Geoanalytical Research, 19(1), 1–23. doi:10.1111/j.1751-908X.1995.tb00147.x.
- Winchester, J.A. & Floyd, P.A., 1977: Geochemical discrimination of different magma series and their differentiation products using immobile elements. Chemical Geology, 20, 325–343. doi:10.1016/0009-2541(77)90057-2.
- Witschard, F., 1984: The geological and tectonic evolution of the Precambrian of northern Sweden — a case for basement reactivation? Precambrian Research, 23(3–4), 273–315. doi:10.1016/0301-9268(84)90047-0.
- Xunfan, G., Yongqin, S., Jinghua, X., Shuzhao, L. & Jinmao, L., 1988: A new type of Tin deposit – the Yinyan porphyry Tin deposit in China. InC.S. Hutchison (ed.): Geology of tin deposits in Asia and the Pacific (pp. 487–494). Berlin, Heidelberg: Springer. doi:10.1007/978-3-642-72765-8_38.
- Zweifel, H., 1976: Aitik – geological documentation of a disseminated copper deposit: a preliminary investigation. Sveriges Geologiska Undersökning C, 720, 80.
Appendix
Table A1. Method of sample preparation and analysis for the various chemical data sets handled in this study. Abbreviations: AD – Acid Digestion, AR – Aqua Regia, ICP-(A)ES – Inductively Coupled Plasma (Atomic) Emission Spectroscopy, ICP-MS – Inductively Coupled Plasma Mass Spectrometry, FD – Fused Disc, LBF – Lithium borate fusion, MA – Multi Acid, XRF – X-ray fluorescence.