ABSTRACT
The present marine Baltic Sea basin (BSB) occupies an eroded Proterozoic intra-cratonic basin on the Fennoscandian shield. Competing models propose a Neogene fluvial origin, with later modification by glacial erosion, or a much younger development, with overdeepening beneath the Fennoscandian Ice Sheet (FIS). We test these alternatives using a first order source to sink sediment budget for the catchment of the BSB. Best estimates derived from geomorphic and cosmogenic nuclide evidence suggest depths of erosion over the last 1 Ma of 20 m in basement and 40 m in sedimentary rocks that surround the BSB. As the BSB has been overdeepened below a regional base level provided by the shallow Darss Sill at the boundary with the Kattegat, erosion of the BSB may be interpreted as glacial in origin, without a fluvial component. The estimated total volume of source area erosion is 30,628 km3 of which 87% is derived from the present BSB. Sediment volumes in the sink area within the limits of maximum Pleistocene glaciation are estimated at a minimum of 37,629 km3, after correction for local erosion, porosity, and carbonate losses. Marine Isotope Stage 12 and younger sediments account for 87% of the total Pleistocene sediment volume in the sink in Poland. Although significant uncertainties remain, the sediment budget is consistent with erosion of the BSB entirely by the FIS, mainly when the ice sheet reached its maximum extent and thickness during the Middle and Late Pleistocene glaciations.
Introduction
The Baltic Sea, including the Bothnian Sea, the Gulf of Finland and the Kattegat, occupies a shallow basin on the surface of the Fennoscandian shield (). This Baltic Sea basin (BSB) is a major physiographic feature of Fennoscandia (Rosentau et al. Citation2017), but its origins and age have received little detailed attention. Competing models exist for the origin of the BSB that may be summarized as (i) a Neogene origin by fluvial erosion, followed by modification by glacial erosion (Ignatius et al. Citation1981; Tuuling & Flodén Citation2016) and (ii) a Pleistocene origin, with overdeepening beneath the Fennoscandian ice sheet (Puura et al. Citation2003; Amantov et al. Citation2011; Šliaupa & Hoth Citation2011). For the second model, three variants may be explored in which glacial erosion of the Baltic basin occurred (a) through all the cold stages of the Pleistocene, (b) mainly during and after the Middle Pleistocene Transition (MPT) towards large, extensive ice sheets after ~1.2 Ma or (c) mainly from the Elsterian (MIS 12) glaciation and after (Beckholmen & Tirén Citation2009). Here we present a first-order, source to sink, Quaternary sediment budget for the BSB. Comparison of estimated erosion depths in the BSB and across the surrounding basement with the volume of sediments found in depocentres to the south provides a test for competing hypotheses for the timing of erosion of the BSB.
Geological history of the Baltic Sea basin
The outline of the northern part of the present BSB corresponds roughly with that of three intra-cratonic sedimentary basins that today hold the Bothnian Bay, the Bothnian Sea and the Baltic Sea proper (). Crustal thinning and aborted rifting during the Mesoproterozoic was accompanied by rapakivi granite intrusion and the deposition of Jotnian arkosic sandstones of km thickness (Buntin et al. Citation2019). Only the southern Baltic basin was affected subsequently by Caledonian orogenesis and Mesozoic rifting (Van Balen & Heeremans Citation1998). Today, the Precambrian crystalline basement plunges to depths of >3 km below the southern shore of the present Baltic Sea (Šliaupa & Hoth Citation2011), whilst the sub-Cambrian basement unconformity remains close to present sea level further north ().
On Baltica, basement and cover had been deeply eroded to form extensive peneplains by the end of the Neoproterozoic (Lidmar-Bergström Citation1996). In the Early Palaeozoic, the basement was transgressed and sequences of sandstone, shales and limestone, a few hundred metres in total thickness, were deposited (Nielsen & Schovsbo Citation2006). Further sediment cover accumulated across the BSB area from the Silurian onwards as detritus was transported from the Caledonide mountain belt (Larson et al. Citation1999).
Palaeozoic sedimentary cover was thinned by erosion through the Mesozoic. The basement was re-exposed in southernmost Sweden by the Triassic (Ahlberg et al. Citation2003), and along the hilly western margin of the Bothnian Sea in the latest Jurassic (Mark et al. Citation2014). Deeply weathered basement in southernmost Sweden was reburied in the Late Cretaceous (Lidmar-Bergström Citation1995). Around much of the present BSB, however, the Early Palaeozoic cover persisted throughout this Era. Areas of geologically-recent re-exposure of basement around the BSB are represented by well-preserved parts of the sub-Cambrian unconformity; its distinctive peneplain form has not been erased by Neogene weathering and Quaternary glacial erosion (Hall et al. Citation2019a). Uplift of the Norwegian passive margin began in the Palaeogene (Sømme et al. Citation2019). During the later Paleogene, the southernmost Baltic region was occupied by the sea (Kramarska Citation2006). Epidote-rich heavy-mineral assemblages in sand beds in Poland (Gibbard & Lewin Citation2016) indicate derivation from land areas on the Devonian sandstones in Estonia and Latvia. A precursor river system, the Eridanos system, drained the present BSB area, carrying amber to deltas in the eastern Baltic in the late Eocene (Sadowski Citation2017). Uplift of Fennoscandia intensified in the Miocene (Rasmussen Citation2018), causing tilting of the backslope in eastern Sweden towards the BSB (Lidmar-Bergström & Olvmo Citation2015; Ebert et al. Citation2011) and the rise of epeirogenic domes (Lidmar-Bergström et al. Citation2012). Sediment supply to the eastern North Sea and southern Baltic increased, with transport via the successor Baltic River system, with major tributaries in the areas of the present Bothnian Sea and Gulf of Finland. The river system was equivalent in length, relief and hydrology to the present Orinoco drainage in South America (Overeem et al. Citation2001). Neogene marine sediments are absent from around the BSB (Gibbard & Lewin Citation2016), and continental sedimentation dominated to the S in Poland (Rasser et al. Citation2008); the BSB did not exist at this time.
Quaternary history of the Baltic Sea basin
Renewed Late Pliocene to Early Pleistocene uplift of southern Norway (Rasmussen et al. Citation2010) was accompanied after 2.7 Ma by phases of mountain glaciation in Fennoscandia. The first advance of the Fennoscandian ice sheet into the central North Sea (Patruno et al. Citation2020) was in the Menapian glaciation (MIS 32–36). The oldest tills recognised on the North European Plain date from MIS 22 (0.88 Ma) (Lindner et al. Citation2013; Ehlers et al. Citation2018). These events mark the start of the Early-Middle Pleistocene Transition towards 100 ka glacial cycles and development of thicker and more extensive ice sheets (Clark et al. Citation2006). The Baltic River continued to operate until ~1.2 Ma, when the drainage network was destroyed by erosion beneath the Menapian ice sheet (Bijlsma Citation1981).
The configuration and pattern of flow in the FIS in the BSB during the last glacial cycle is well established from patterns of striae (Glückert Citation1974), till lineations (Kleman et al. Citation1997) and directions of erratic carry (Donner Citation2005; Hoffmann & Meyer Citation1999; Czubla et al. Citation2017; Woźniak et al. Citation2009) and replicated in glaciological models (Patton et al. Citation2016) (). During ice sheet build-up and decay, the main ice shed lay towards the Scandic mountains, with fast flow directed southward along the Bothnian and Baltic depressions. At the Last Glacial Maximum (LGM), the ice shed moved to a position over the Bothnian depression but became cold-based and non-erosive. Hence, although LGM ice flow extended eastward into Finland, there is little evidence from glacial lineaments for strong erosion by ice moving against the eastern slope of the Bothnian basin. Instead, in Ostrobothnia, the presence of deeply weathered rock and multiple tills within thick Pleistocene sequences indicates negligible erosion (Pitkäranta Citation2013). An axial pattern of N-S ice flow developed, with carry of erratics of rapakivi granite from Åland to eastern Germany and Poland (Hoffmann & Meyer Citation1999; Woźniak & Czubla Citation2015). Axial flow was fed partly by ice moving south-east from that part of eastern Sweden lying N of the Åland sill. A southward ice flow developed across southern Sweden (Fredén Citation1994). Further west, a powerful flow of ice from the Oslo Graben into the Skagerrak and Norwegian Channel tended to block direct outflow to the eastern North Sea. Baltic ice was constrained to flow into Poland. When ice flow slackened from south-west Sweden, and during ice advance and retreat, a diffluent flow pattern developed in Denmark and N Germany, with ice flow westward towards the Norwegian trench (Houmark-Nielsen Citation2004).
Figure 2. The Baltic Sea basin and its bathymetry in relation to Pleistocene ice limits and ice flow lines. W Late Weichselian. Wa Warte. S1 Sanian 1, E Elsterian. S Saalian. Pleistocene ice limits are from Marks et al. (Citation2018). Bathymetric data from European Marine Observation and Data Network.
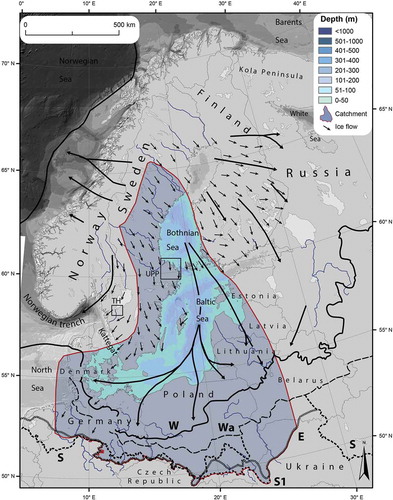
The build-up and decay of the FIS through the Quaternary has generated isostatic loading and unloading of the crust of the Fennoscandian shield. Glacial isostatic rebound from the last glacial cycle is not yet complete. The residual rebound in eastern Sweden has been modelled under the assumption that glaciation, and its associated loading, is not renewed (Näslund Citation2006). Residual rebound over the next 60 ka has been estimated at ~100 m in Ångermanland, ~70 m at Stockholm and ~20 m at the present southern Baltic coast (). Although these estimates include significant uncertainties (Näslund Citation2006), the fundamental effect of residual rebound is to tilt the BSB southwards, thereby reducing the area of the marine basin in the future, particularly in the Bothnian Sea.
Figure 3. Cross-section of the Baltic Sea basin today and at 60 ka in the future after residual glacial isostatic rebound is complete. Line of section indicated on . The long profile of the Orinoco River is added for comparison. Residual isostatic rebound at 60 ka after Näslund (Citation2006).
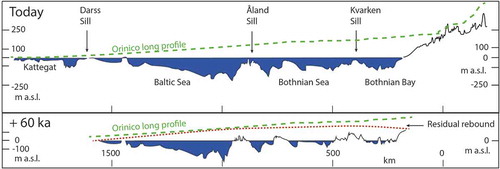
Landforms of glacial erosion in the BSB
The BSB has a threshold at the Darss Sill at the eastern boundary of the Kattegat (). The sill is presently at <20 m water depth, with the base of the Saalian and younger glacial sequence resting on Late Cretaceous chalk at 40–80 m depth (Lemke et al. Citation1994). Numerous, large glacial rafts of chalk occur in the surrounding Arkona Basin (Obst et al. Citation2017). These rafts indicate that the Darss Sill has been lowered by glacial erosion in the Middle and Late Pleistocene. Given the 550 m thickness of the chalk sequence to the S on Rügen (Flodén et al. Citation1996), it is likely that a chalk cuesta existed in the vicinity of the Darss Sill. Prior to glacial erosion, the Darss Sill probably stood at or above present sea level. Hence, fluvial erosion in sea level lowstands before or during the Pleistocene did not operate to elevations far below present day sea level.
Figure 4. Bathymetry and major landforms of the Baltic Sea. Bathymetric data from European Marine Observation and Data Network, EMODnet Digital Bathymetry (DTM 2018). EMODnet Digital Bathymetry (DTM) was accessed on 14 October 2019 at http://doi.org/10.12770/18ff0d48-b203-4a65-94a9-5fd8b0ec35f6. Major erosional basins, trenches and scarps are named. Eemian marine sediment localities from Miettinen et al. (Citation2002) and Funder et al. (Citation2002).
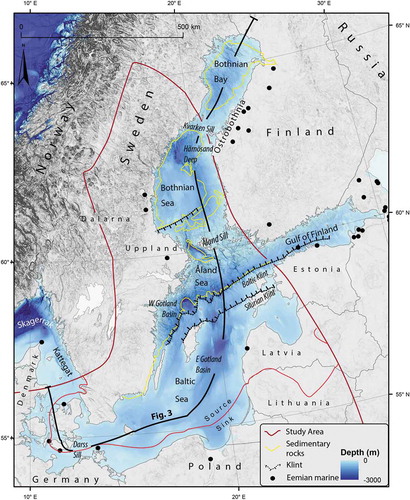
Superimposition of the long profile of the Orinoco, a river of equivalent length to the former Baltic River, indicates a plausible datum for Pleistocene glacial erosion (). Correction for residual isostatic rebound indicates that the present shoreline of the BSB will be tilted southward and warped at 60 ka in the future, when all rebound is complete. The rebound surface and the Orinoco profile lie at similar relative elevations, supporting the proposition that fluvial erosion had lowered rocks around the BSB close to the erosional level of the present shoreline prior to the onset of major glacial erosion. As rivers do not erode below base level, the presence of the BSB requires overdeepening by erosion from the FIS. This pattern and depth of erosion is the same for the present and +60 ka scenarios as the form of the BSB remains the same when it is tilted and warped.
Glacial erosion involved the formation of major glacial landforms (). The primary features are the topographic depressions that hold the Bothnian Bay, the Bothnian Sea and the Baltic Sea. The two Bothnian basins were formed mainly through the excavation of Mesoproterozoic and Early Palaeozoic sedimentary rocks (Amantov et al. Citation2011). Large secondary features occur as deeps, with basin and trench forms. The deeps in the Bothnian and Åland Seas reach −301 m; these features are excavated in weak Mesoproterozoic and Early Palaeozoic sedimentary units, not in basement. Some trenches run transverse to ice flow, as in the Gulf of Finland and its western continuation into the Baltic proper (Noormets & Flodén Citation2002). This latter trench is excavated through the Early Palaeozoic sequence, including soft early Cambrian clays (Kirsimae et al. Citation1999), and the deeply weathered basement beneath the sub-Ediacaran unconformity (Liivamägi et al. Citation2014). Other trenches run parallel to former ice flow, as in the eastern Gotland basin (- 249 m deep) and in the western Gotland basin (−459 metres in the Landsort Trench, where rockhead is at −518 m) (Flodén Citation1977). Long scarps, referred to as “klints” (Danish), mark the edges of resistant beds of Ordovician and Silurian limestone (Tuuling & Flodén Citation2001). Klints are conspicuous features on the sea floor from Gävle southwards into the Baltic. Other prominent scarps exist on the flanks of the Åland and Härnösand Deeps at the faulted contacts of basement and sedimentary rocks.
A first-order source-sink sediment budget for the BSB
The mapped source-sink area (~1.6 million km2) for the Baltic lobe of the FIS covers the territory from Denmark in the West to the Baltic states and Belarus in the East (~10° E to ~27° E) and is bounded by the Pleistocene glacial maximum limit in the South (~50° N) and the Kvarken Sill in the North (~64° N), see and . We attempt to constrain the timing of the excavation of the BSB by comparing the the rock volume missing in the source area with the total volume of Early, Middle and Late Pleistocene sediment found in the sink area south of the basin.
Data sources and manipulation
The surface topography model is a hybrid product based on the EU-DEM v1.0 (courtesy of Copernicus Program, https://land.copernicus.eu/imagery-in-situ/eu-dem, accessed September 2019) covering the countries within the European Union and the SRTM v3 (courtesy of NASA and National Geospatial-Intelligence Agency, https://lpdaac.usgs.gov/products/srtmgl1v003/, accessed September 2019) for Ukraine and Belarus. The hybrid surface model has an approximate spatial resolution of 30 m and a vertical accuracy of 2.9 m and 6.2 m, for the EU-DEM v1.0 and the SRTM v3 data respectively. The hybrid surface topography model was resampled to 100 m to match the spatial resolution of the Quaternary basin topography model.
The base-Quaternary topography model is a compilation of published and unpublished interpolated borehole data. For the North European Plain and Sweden, we used a depth to bedrock model as an approximation of the Quaternary sediment thickness. Local and national interpolated Quaternary borehole data of the Quaternary base topography were compiled for Denmark (courtesy of GEUS, https://www.geus.dk, accessed September 2019), the Germanic federal states (courtesy of LGB, LAGB, GPDN, LUNG, NIBIS, LLUR) and Poland (Nawrocki & Becker Citation2019), together with a depth to bedrock model for the eastern part of the North European Plain (Gorlach et al. Citation2015) and Sweden (courtesy of Lantmäteriet, https://maps.slu.se, accessed September 2019). All the datasets were resampled, if needed, to the dataset with the lowest spatial resolution (100 m). Often the interpolated borehole data was only given in contoured vector data which had to be processed into raster data using an iterative finite difference interpolation method. Narrow erosional tunnel valleys are not represented in our base-Quaternary topography model for Poland and the Baltic states due to the limited spatial resolution of the interpolated borehole data (Gorlach et al. Citation2015). A Quaternary sediment thickness map was calculated by subtracting accessible Quaternary basin topography models from a surface topography model. We ignore isostatic responses to rock unloading after erosion and water loading after submergence.
Defining source and sink
The source area for sediment () includes parts of northern Sweden E of the main ice shed () and those parts of eastern-southern Sweden with ice flow towards sink areas in Jutland, N Germany and Poland as indicated by patterns of striae and erratic carry (Overweel Citation1977). A major part of the source area is the BSB itself, but the Bothnian Bay, lying N of the Kvarken sill (), is excluded because ice flow was dominantly towards parts of Finland on the eastern shore of the Bothnian Gulf (Greenwood et al. Citation2017). Glacial erratics of Finnish rocks found in Poland come almost exclusively from rapakivi granites on Åland (Woźniak & Czubla Citation2015), reflecting dominant patterns of ice flow to the S and SE in southern Finland (Salonen & Glückert Citation1992). Carry of Ordovician erratics from the Bothnian Sea basin into SW Finland was restricted mainly to a 50 km wide coastal strip (Uutela Citation1991). On this basis, Finland, E of Åland, is excluded from both source and sink. Small numbers of erratic clasts in tills are derived from outside the source area in southern Sweden (Smed Citation2016; Smed & Ehlers Citation2002) and in Belarus (Karabanov & Matveyev Citation2011) but these gains are likely roughly equivalent to losses out of adjacent parts of the source area.
Figure 5. A. Source and sink areas for the Baltic Sea basin. Arrows indicate inputs and outputs of sediment outside the main source-sink area used for the sediment budget. B. Schematic representation along a NW-SE transect of the basement and sedimentary rock components removed from different terrains in the source area. Line of section indicated on Fig. 5A.
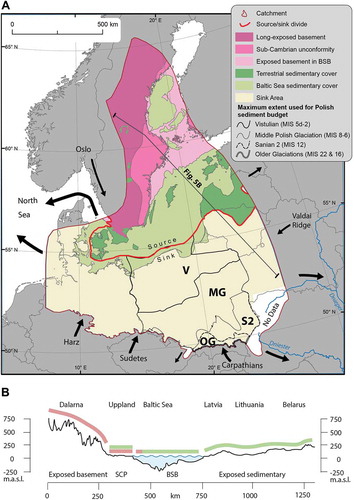
The boundary between source and sink is placed at the 50 m isopach for sediment depths in the southern Baltic, close to the present shoreline, in a belt where Pleistocene sediment thickness increases sharply.
The sink area lies on the North European Plain (). The presence of reverse slopes in Belarus towards the Valdai ridge, in Poland towards the Carpathian and Sudetes Mountains and in northern Germany towards the Harz massif make the sink area a widely effective trap for sediment released from the FIS margin (). However, sediment transfers occurred across the sink area boundaries. For example, at the Elsterian maximum, sediment was lost to the drainage systems of the Dnieper (Marks & Pavlovskaya Citation2003) and Dniester Rivers (Gozhik et al. Citation2012) (). Ice moving westward into the Kattegat and Skagerrak from the southern BSB fed sediment into the North Sea during the Middle and Late Pleistocene via the Norwegian Channel ice stream. Sediment loss by this route is difficult to quantify but was likely significant, as individual erratics from Åland are recorded on the western rim of the North Sea basin (Phemister Citation1926; Catt Citation1991; Hall et al. Citation2016). Transport of clastic debris from the Oslo Graben, outside the source area, is recorded by the presence of indicator rhomb-porphyry in tills within the sink area in northern Germany (Schuddebeurs Citation1987) and as traces in NW Poland (Górska Citation2003). Sediment was also transferred into the sink area by rivers flowing from the Carpathians (Lindner & Marks Citation2015) and the Sudetes (Krzyszkowski & Ibek Citation1996; Salamon Citation2009).
Source area erosion
The source area includes two main substrates: the Precambrian basement and overlying sedimentary cover (). The basement gneisses are very hard but unevenly fractured; the sedimentary cover varies in hardness from hard Mesoproterozoic sandstones to soft Early Cambrian clays and Late Cretaceous chalk, and generally has a relatively high fracture density. The basement and sedimentary substrates have provided fundamentally different resistance to erosion by the FIS, an important factor in the overdeepening of the BSB within its intra-cratonic basin setting.
The source area includes four main terrains (): (i) basement in Sweden exposed to weathering and erosion before the Pleistocene, (ii) basement in eastern and southern Sweden exhumed from beneath sedimentary cover during the Pleistocene. This includes terrain where the sub-Cambrian and sub-Mesozoic unconformity surfaces are inherited in the present basement topography, indicating limited erosion due to geologically-recent exhumation (Hall et al. Citation2019a, Citation2019b), (iii) the BSB, with its submarine floor of basement and Mesoproterozoic, early Palaeozoic and Mesozoic sedimentary rocks in the Bothnian and Baltic basins and (iv) the Palaeozoic sedimentary rock terrain on that part of the North European Plain that lies to the south of the Gulf of Finland and areas of Palaeozoic and Mesozoic sedimentary rock in southern Sweden.
In basement terrain in Sweden, estimated erosion depths for the last 1 Ma and 100 ka have been derived previously by using three separate methods: sediment budgets, erosion below a reference surface and Terrestrial Cosmogenic Nuclides (TCNs). An earlier source-sink sediment budget for Sweden and Denmark has yielded an estimate of an average 12 m Quaternary erosion of the bedrock, mainly basement, in Sweden (Påsse Citation2004); this estimate is too low as no account was taken of sediment transferred from Sweden into Germany and Poland. A second method is to use the near-planar sub-Cambrian unconformity as a reference surface for erosion of basement since its exhumation from beneath sedimentary cover. The timing of exhumation was diachronous, younging towards existing outliers, but the main phase of exhumation likely began at ~1.2 Ma, during and following the MPT (Hall et al. Citation2019b). In Uppland (), average depths of basement erosion below the former unconformity are estimated at 24 m (Hall et al. Citation2019a); at Trollhättan, estimated average depths are 16–20 m (Hall et al. Citation2019b). Most of the rock was excavated from trenches and basins. TCNs in basement rocks in Fennoscandia frequently occur at concentrations that indicate inheritance from before the last glaciation. Inheritance indicates that erosion beneath the last FIS was insufficient in depth (<2-3 m) to remove all existing TCNs. Under these circumstances, the depth of rock lost to erosion can be modelled. Around Forsmark, Sweden, mid-range estimates for erosion, mainly from resistant bedrock highs, over the last 100 ka and 1 Ma are 1.6–3.5 m and 13–27 m, respectively (Hall et al. Citation2019a; Heyman et al. Citation2019). An estimate of 20 m erosion depth is adopted for basement erosion over the last 1 Ma outside the BSB.
The depth of sedimentary cover removed is difficult to constrain. Sedimentary rock has been eroded from above basement unconformities in Sweden and above the surviving Palaeozoic sedimentary sequences from the Gulf of Finland southwards (). In the Öregrund archipelago, SE of Forsmark, small half grabens, with fills of Mesoproterozoic sandstone and Ordovician limestone, have been excavated by glacial erosion to depths of 30 to >45 m relative to the approximate position of the sub-Cambrian unconformity on adjacent rock blocks (Hall et al. Citation2019a). Glacial excavation below Neogene planation surfaces E of the Baltic Sea is estimated at 40 m (Amantov Citation1995). An estimate of 40 m erosion is adopted for the thickness of sedimentary rock removed by glacial erosion outside the BSB from above areas where the sub-Cambrian unconformity is well-preserved and from terrain south of the Gulf of Finland.
For the BSB, we assume that all erosion below present sea level is a result of glacial excavation. as rivers do not erode below base level and the regional base level for the BSB was provided by the shallow Darss Sill. The volume of the BSB below present sea level, however, represents a minimum value for glacial erosion. Along the present shoreline of the BSB, additional sedimentary rock has been removed from above sea level on the re-exposed sub-Cambrian unconformity. Hence sedimentary rock was likely also eroded above present sea level across the BSB, but this depth is not quantified here.
The total estimated volume of material eroded from the source area is estimated as 30,628 km3 (). Of this total, 67 % derives from erosion of the BSB.
Table 1. Source area erosion.
Sink area deposition
The sediment volume includes rock derived from erosion within the sink area. This local erosion is manifest in the presence of local rocks as clasts in tills (Czubla et al. Citation2010), by glacitectonic rafts of these rocks (Aleksa & Bitinas Citation2000; Obst et al. Citation2017) and by extensive networks of tunnel valleys cut by glacial meltwaters into bedrock (Ehlers Citation1981; Al Hseinat & Hübscher Citation2014). This local erosional contribution is large. Indicative components of local rocks in the fine gravel size fraction of tills from pre-Elsterian ice advances are 5–32 % local rocks at sites in the Poland-Belarus border zone (Lindner & Astapova Citation2000). Chalk and flint clasts locally dominate gravel fractions in Weichselian tills in Jutland (Houmark-Nielsen Citation2007) and Schleswig-Holstein (Van Der Wateren Citation1999). The contribution to local erosion by glacial rafting is potentially very large (Ruszczyńska-Szenajch Citation1987). Whilst rafts of Ordovician and Devonian rocks derived from the source area are present in the sink area in Poland and further east (Karabanov Citation2000), the predominant source of glacitectonic rafts is local bedrock. Glacitectonic effects beneath the FIS have mobilised and recycled soft Mesozoic rocks, notably the Late Cretaceous chalk (e.g. Dobrowolski Citation2009; Pedersen & Gravesen Citation2009; Lagerlund et al. Citation1995), and unconsolidated Palaeogene and Neogene sediments (e.g. Ruszczyńska-Szenajch Citation1985; Hoffmann & Piotrowski Citation2001). Reworking of Pleistocene sediment by glacitectonics is present across ~25% of the present landsurface of Lithuania. One glacitectonic structure is 30 km long and 8–11 km wide (Aleksa & Bitinas Citation2000). Glacial rafts are up to 1.5 km long and 20–25 m high in Belarus (Karabanov Citation2000). Glacitectonic structures and its associated disturbance occur to depths of 100–240 m in NE Poland (Słodkowska & Gałązka Citation2015). In Jutland, glacitectonised pre-Pleistocene and Pleistocene sedimentary megablocks may form the entire thickness of the Pleistocene sediment (Jakobsen Citation1996). Where tunnel valleys occur in the southern Baltic, they occupy 10–30% of the seabed and are commonly 25–50 m deep (Flodén et al. Citation1997). In Schleswig-Holstein, tunnel valley floors reach depths of 100–200 m (Piotrowski Citation1994). We adopt 25 % as a rough estimate for the contribution of local erosion in the sink area to Pleistocene sediment volumes.
The BSB and its southern margins includes large areas of carbonate rock (). Glacial crushing and comminution of soft carbonates during transport renders them susceptible to solution (Fairchild et al. Citation1994). Solute load is lost to meltwater and so represents a missing part of the material eroded from the source area where meltwater can escape from the sink area. Losses to solution in soils are evident from the Geological Atlas of Poland (Nawrocki & Becker Citation2019) which gives mean Ca concentrations of 0.5 % in top soil and 1.67% in surface sediments that compare to Ca concentrations of 5–10 % at depth in the matrix of Saalian and Elsterian tills (Czubla et al. Citation2010). We adopt 10% as a conservative estimate for total losses of carbonate in solution from the sink area.
Porosity of Pleistocene sediments in Poland approximates to 20 % for clay, 25–30 % for sand and 40 % for gravel (Carcione et al. Citation2000). These estimates are similar to those of 20–30 % based on sediment density data for Pleistocene sediments in well logs offshore from western Norway (Dowdeswell et al. Citation2010; Knutsen Citation2018). An estimate of 25 % is adopted here for average porosity. Sediment volumes were calculated using sediment isopachs in the sink area (), with correction for local erosion, pore space and carbonate loss. Our compilation indicates that the total volume of sediment present in the sink area is equivalent to a former rock volume of 37,629 km3 ().
Table 2. Sink area sediment volume.
Figure 6. Surface elevation, base Quaternary elevation and Quaternary sediment isopachs in the sink area. See text for data sources.
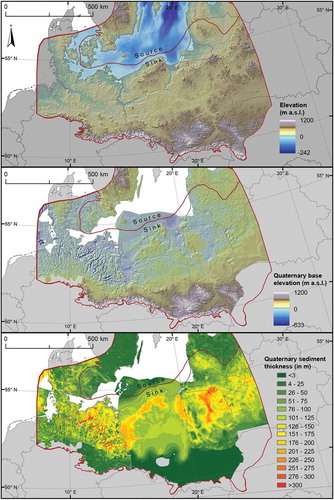
To constrain the timing of erosion, we examine the volumes of sediment of different ages along three 600–800 km long transects that connect critical stratigraphic sections in Poland and westernmost Belarus that extend through thick Quaternary sequences into bedrock. Poland lies along the continuation of the axial ice flow line for the FIS through the BSB () and so the stratigraphy can be regarded as typical of the wider sink area. We employ a four-part division of the Polish Quaternary stratigraphy into Older Glaciations (OG: MIS 22 and 16) (Marks et al. Citation2016), Sanian 2 (S2: MIS 12). Middle Polish Glaciation (Liviecian, Krznanian and Odranian glaciations) (MP: MIS 8–6) and Vistulian (V: MIS 5d-2) that corresponds with the pre-Elsterian, Elsterian, Saalian and Weichselian glaciations in the NW European stratigraphic framework. Reworking of pre-existing sediment is common throughout the Quaternary stratigraphy of the sink area.
The stratigraphic transects in Poland suggest that ~69 % of the sediment is found in Middle Pleistocene glacial deposits from the MIS 12 to 6 glaciations (). Sediment volumes dating back to MIS 22 are small (~13 %), but existing sediment may have been reworked by the MIS 12 ice sheet. OG sediments are widely found in deep depressions likely eroded by meltwater from pre-MIS 12 ice sheets (). Sediment volumes from the last glacial cycle are also small (~18 %) and must include material recycled from earlier glacial phases and so not eroded from source bedrock by the last ice sheet. However, significant additional thicknesses of Late Weichselian to Holocene sediments exist in the source area on the floor of the southern Baltic Sea (18.7 m), and also in the Kattegat (63.8 m) (Påsse Citation2004). Average thicknesses of Late Weichselian glacial deposits that rest on the basement in eastern Sweden are lower, at <6 m (Påsse Citation2004).
Table 3. Sediment in Poland.
Figure 7. Pleistocene sediment in boreholes along three N-S transects in Poland and westernmost Belarus. Pleistocene ice limits are from Marks et al. (Citation2018).
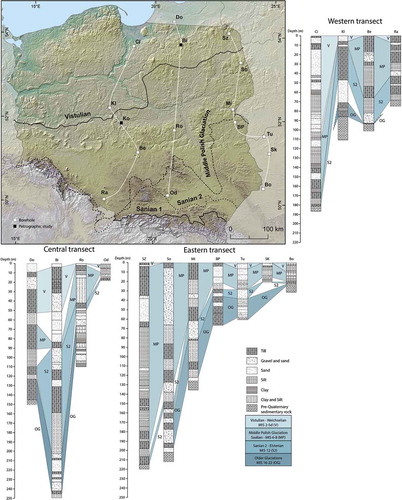
Discussion
A fluvial drainage basin of sub-continental scale, drained by the Baltic River, existed in the area of the BSB in the Neogene, with major tributaries following routes along what are now the Gulfs of Bothnia and Finland. Superimposition of the profile of the comparable Orinoco River onto the post-rebound profile of the present Baltic shoreline indicates that, prior to glacial erosion, the erosional level lay within a few tens of metres of that of the present (). Differential erosion of sequences of Early Palaeozoic sedimentary rocks, with unconsolidated Cambrian clays, fissile shales and more massive Ordovician and Silurian limestones, that dip gently southwards (Tuuling Citation2017) likely led to development of north-facing homoclinal scarps along the receding edge of the sedimentary cover. A cuesta may have existed on the thick chalk at the site of the present Darss Sill.
The present marine basins of the Bothnian, Åland and Baltic Seas fill depressions overdeepened after erosion by the FIS. Overdeepening exploited differences in rock resistance between basement and the sedimentary infill of intra-cratonic basins in the Bothnian and Åland Seas (Amantov et al. Citation2011). The contributions of different processes of glacial erosion also differed across the two substrates. Abrasion, plucking and ripping were dominant on the basement rocks (Hall et al. Citationin Press); thrusting, rafting and tunnelling were much more significant on sedimentary rocks. The main deeps in the BSB are developed in sedimentary rocks, with 100–200 m high fault-line scarps at basement-sedimentary contacts (). The present submarine Baltic klints are of similar height and stand at similar depths to the BSB deeps. Hence the present-day Baltic klints cannot be inherited pre-glacial structural landforms, modified by glacial erosion (Puura et al. Citation2003; Tuuling & Flodén Citation2016), as the bases of the klints lie at depths of up to −150 m below sea level in the East Gotland Basin, far below the reach of fluvial erosion. Instead, the klints are largely glacial features (Amantov et al. Citation2011), formed as the FIS moved against prominent edges developed in the more massive Ordovician and Silurian sedimentary units in the Early Palaeozoic sequence, as documented by Tuuling & Flodén (Citation2016). On land in northern Estonia, glacitectonic thrusting acting on the Baltic klint led to extensive and deep deformation and faulting, and to the detachment and transport of large rafts of limestone (Rattas & Kalm Citation2004). That similar processes likely operated beneath the FIS in the present offshore area of the BSB is consistent with widespread evidence for glacitectonic disturbance of pre-existing faults across the scarp and dip slopes of the submerged Baltic and Silurian klints (Tuuling & Flodén Citation2001). Across wide areas further S on the bed of the Baltic Sea, fault movements occurred in response to regional stress fields and ice loading (Al Hseinat & Hübscher Citation2017). Here the sub Quaternary surface generally lies >100 m below sea level, a depth that can be attributed to the removal of Palaeozoic to Palaeogene strata by Pleistocene glacial erosion (Uscinowicz Citation2003).
The estimated volume of rock derived from the source area (30,628 km3) is similar to that estimated for the sink area (37,629 km3). When the sediment that has accumulated over the last 1.2 Ma in the sink area is back-stacked, it refills the BSB and allows for erosion depths over the last 1 Ma of 20 m and 40 m on the surrounding areas of basement and sedimentary cover, respectively. This finding suggests that the budget represents a valid first order representation of erosion and deposition.
The similarity is potentially misleading because significant uncertainties exist. The extent of the source area and the depth of erosion below present sea level in the BSB are well constrained. The depths of erosion estimated in basement and sedimentary cover, however, are based on case studies of small areas and may have errors. The minor difference between source and sink volume suggests, however, that errors in source erosion depths are small. For example, the removal of an additional 20 m of rock across the BSB, representing rock eroded from above present sea level, would eliminate the difference between source erosion and sink sediment volumes. The extent of the sink area is less well constrained, with leakage of sediment to the eastern North Sea and central European river systems (). Small areas in the sink area lack data (). Nonetheless the volume of sediment present in the sink area is generally well constrained and porosity likely falls in the range of 20–30%, close to the value of 25% used here. The estimated 25% contribution from erosion of local bedrock within the sink area is more uncertain and requires further testing. The carbonate content of tills in Poland is highly variable (Woźniak et al. Citation2009; Morawski Citation2009; Czubla et al. Citation2010) and the leakage losses of carbonate in solution may be too low. On balance, the volume of sediment eroded from the BSB is likely underestimated. If so, this requires greater erosion depths across basement and cover rocks across the entire source area and, more specifically, across carbonate rocks in and around the BSB. Further work is needed to refine the source-sink model and its parameter values in order to reduce these significant uncertainties. Nonetheless the adoption of current best-estimate values based on available evidence leads to an approximate balance of the source-sink sediment budget for the BSB and its surroundings. The pioneer Norwegian glacial geologist, Amund Helland, recognised the fundamentals of this source-sink balance as early as the 1870’s (Hansen Citation1894).
Basal Pleistocene sequences in Belarus and Poland include tills formerly regarded as MIS 32 in age (Ber Citation2006; Lindner et al. Citation2006), but more recently attributed to MIS 22 (Lindner et al. Citation2013). Hence, the sediment in the sink accumulated after 1.2 Ma, and may be almost entirely of post-0.88 Ma age. Erosion of the BSB must date mainly from the late Early Pleistocene and later. This is consistent with (i) the restricted size of the FIS in the Early Pleistocene (Kleman et al. Citation2008; Ehlers et al. Citation2011), (ii) an absence of Early Pleistocene marine sediment from the BSB (Gibbard & Lewin Citation2016), either in situ or in glacial rafts, (iii) first excavation of the Skagerrak and Norwegian Channel overdeepenings at ~1.2 Ma (Sejrup et al. Citation1995) or ~0.8 Ma (Ottesen et al. Citation2014), (iv) the destruction of the Baltic River system in the Menapian, signified by the disappearance of the Baltic Gravel Assemblage (Overeem et al. Citation2001), (v) the first appearance of clasts from central and eastern Sweden, and Åland, in gravel beds in sediments of Menapian age in the Netherlands (Zandstra Citation1971, Citation1983) and (vi) regional average post-MIS 32 erosion depths of 49 m estimated from Pleistocene sediment volumes in platform areas that lie south-east of the Baltic Sea and extend into western Russia (Kalm & Gorlach Citation2014). Initiation of overdeepening in the BSB at 1.2 Ma is also consistent with evidence for the timing of the onset of major glacial erosion on inner shelves across NW Europe (Patruno et al. Citation2020).
Sediment volumes of different ages indicated by transects in Poland provide constraints on the timing of erosion of the BSB ( and ). Each ice sheet tends to erode and rework pre-existing sediment and so the sediment volumes do not convert simply to erosion depths in different time slices. Nonetheless the bulk of the sediment (87 %) was deposited in the Middle and Late Pleistocene glaciations in MISs 12, 8, 6 and 2 when the FIS reached its greatest thickness and extent. Most of the erosion in the BSB occurred during and after MIS 12.
Deep erosion of the BSB in the Middle and Late Pleistocene is consistent with other evidence. The Elsterian ice sheet was associated with major erosion across NW Europe, with examples including the development of a prominent glacigenic unconformity in the southern North Sea (Moreau et al. Citation2012), the cutting of the English Channel by meltwater (Gupta et al. Citation2017) and the incision of dense networks of tunnel valleys on the North European Plain (Ehlers et al. Citation2011). In the southern BSB, the axial flow beneath the FIS was across the ~150-300 km wide outcrops of the Ordovician and Silurian limestones ( and ). Middle and Late Pleistocene tills in Poland often include >50% limestone clasts over a distance of 400 km southwards from the present coast (Lindner & Astapova Citation2000; Czubla et al. Citation2010, Citation2017; Woźniak & Czubla Citation2015; Woźniak et al. Citation2009). Whilst the limestone clasts may range from Ordovician to Late Cretaceous in age, sustained and deep erosion of the Ordovician and Silurian outcrop in the BSB is indicated. It is also significant that the earliest marine sediments found around the south-eastern Baltic are Holsteinian in age (MIS 11) (Marks & Pavlovskaya Citation2003); if an extensive marine basin had existed before the preceding Elsterian glaciation then traces of its marine sediments should remain in the BSB. The Elsterian glaciation likely led to the breaching of the Darss Sill, allowing the first ingress of marine waters in MIS 11 and, later, in MIS 5 (). In terms of competing hypotheses, available evidence points to an origin for the BSB almost entirely by glacial erosion after 1.2 Ma, with most erosion occurring after 0.5 Ma.
The BSB can be compared in its development to the glacial basins that hold the Great Lakes in North America. Ice flow in the Laurentide Ice Sheet was channelled along pre-existing valley systems (Eyles et al. Citation1985). The southern Great Lakes were excavated in gently-dipping Palaeozoic sedimentary rocks, including easily erodible shales and limestones. Large parts of Lake Superior were excavated in unmetamorphosed Mesoproterozoic and Neoproterozoic sandstones (Larson & Schaetzl Citation2001). Cover rocks have been stripped from wide areas of the sub-Ordovician basement unconformity at Georgian Bay (Eyles et al. Citation1993). Glacial overdeepening extends over a range of depths, from >210 m in Lake Superior and >64 m in Lake Huron, and dates mainly from the last 0.8 Ma (Larson & Schaetzl Citation2001). The BSB and the Great Lakes show strong similarities in their development that include geological setting, the pattern and depth of glacial erosion and a timing for peak erosion that coincides with the build-up of large ice sheets through the Middle and Late Pleistocene.
Conclusion
A first-order source to sink Quaternary sediment budget for the marine basin of the Baltic Sea indicates that 30,628 km3 rock has been removed from source areas compared to the 37,629 km3 which remains in sink areas within Pleistocene maximum limits. Sink area sediment dates, at earliest, from the Menapian (MIS 32–36) glaciation at ~1.2 Ma, and an estimated 87% of the sediment in Poland was deposited during and after MIS 12. Approximately 67% of the erosion volume comes from the BSB; the basin has been overdeepened by erosion beneath the Fennoscandian Ice Sheet. The major landforms of the Baltic Sea floor, its sub-basins, trenches, deeps and klints, can be interpreted as features of differential glacial erosion in basement and sedimentary rocks. Formation of the BSB in its present configuration during the Middle and Late Pleistocene is consistent with evidence for major transformation of inner shelves across NW Europe and with overdeepening of the Great Lakes in North America beneath large and extensive ice sheets.
Acknowledgments
The interesting problem of the origin and age of the BSB became apparent during SKB-funded research on glacial erosion in lowland Fennoscandia. Professor Johan Kleman provided insightful commentary on a draft of this paper. The paper was further improved by helpful suggestions and corrections from two anonymous referees and the Editor.
Disclosure statement
No potential conflict of interest was reported by the authors.
References
- Ahlberg, A., Olsson, I. & Simkevicius, P. 2003: Triassic-Jurassic weathering and clay mineral dispersal in basement areas and sedimentary basins of southern Sweden. Sedimentary Geology 161, 15–29. doi:10.1016/S0037-0738(02)00381-0.
- Al Hseinat, M. & Hübscher, C., 2014: Ice-load induced tectonics controlled tunnel valley evolution–instances from the southwestern Baltic Sea. Quaternary Science Reviews 97, 121–135. doi:10.1016/j.quascirev.2014.05.011.
- Al Hseinat, M. & Hübscher, C., 2017: Late Cretaceous to recent tectonic evolution of the North German Basin and the transition zone to the Baltic Shield/southwest Baltic Sea. Tectonophysics 708, 28–55. doi:10.1016/j.tecto.2017.04.021.
- Aleksa, P. & Bitinas, A., 2000: Glaciotectonic features in Lithuania. Geological Quarterly 44, 9–14.
- Amantov, A., 1995: Plio Pleistocene Erosion of Fennoscandia and its Implications for Baltic Area. Proceedings of the Third Marine Geological Coonference “The Baltic”. Prace Panstwowego Instytutu Geologicznego, Warsaw.
- Amantov, A., Fjeldskaar, W. & Cathles, L., 2011: Glacial Erosion/Sedimentation of the Baltic Region and the Effect on the Postglacial Uplift. In J. Harff, S. Björck, & P. Hoth (eds.): The Baltic Sea Basin, 53–71. Springer, Berlin Heidelberg.
- Beckholmen, M. & Tirén, S.A., 2009: The geological history of the Baltic Sea, a review of the literature and investigation tools. SSM report. 2009: 17. Swedish Radiation Safety Authority Report, Stockholm.
- Ber, A., 1989: Stratigraphy of the Quaternary of the Suwałki Lakeland and its substrate based on recent data. Geological Quarterly 33, 463–478.
- Ber, A., 2006: Pleistocene interglacials and glaciations of northeastern Poland compared to neighbouring areas. Quaternary International 149, 12–23. doi:10.1016/j.quaint.2005.11.014.
- Bijlsma, S., 1981: Fluvial sedimentation from the Fennoscandian area into the north-west European Basin during the Late Cenozoic. Geologie En Mijnbouw 60, 337–345.
- Buntin, S., Malehmir, A., Koyi, H., Hogdahl, K., Malinowski, M., Larsson, S.A., Thybo, H., Juhlin, C., Korja, A. & Gorszczyk, A., 2019: Emplacement and 3D geometry of crustal-scale saucer-shaped intrusions in the Fennoscandian Shield. Scientific Reports 9, 10498. doi:10.1038/s41598-019-46837-x.
- Carcione, J.M., Marcak, H., Seriani, G. & Padoan, G., 2000: GPR modeling study in a contaminated area of Krzywa Air Base (Poland). Geophysics 65, 521–525. doi:10.1190/1.1444746.
- Catt, J.A., 1991: The Quaternary history and glacial deposits of East Yorkshore. In J. Ehlers, P. Gibbard, & J. Rose (eds.): Glacial deposits of Great Britain and Ireland, 185–191 p. Balkema, Rotterdam.
- Clark, P.U., Archer, D., Pollard, D., Blum, J.D., Rial, J.A., Brovkin, V., Mix, A.C., Pisias, N.G. & Roy, M., 2006: The middle Pleistocene transition: characteristics, mechanisms, and implications for long-term changes in atmospheric CO2. Quaternary Science Reviews 25, 3150–3184. doi:10.1016/j.quascirev.2006.07.008.
- Czubla, P., Forysiak, J. & Petera-Zganiacz, J., 2010: Lithological and petrographic features of tills in the Koźmin region and their value for stratigraphical interpretation of glacial Lake Koźmin deposits, Central Poland. Geologija 52, 1–8. doi:10.2478/v10056-010-0001-5.
- Czubla, P., Terpiłowski, S., Orłowska, A., Zieliński, P., Zieliński, T. & Pidek, I.A., 2017: Petrographic features of tills as a tool in solving stratigraphical and palaeogeographical problems–a case study from Central-Eastern Poland. Quaternary International 501, 45–58. doi:10.1016/j.quaint.2017.08.028.
- Dobrowolski, R., 2009: Glaciotectonic deformations of the Upper Cretaceous rocks: evidence from the chalk quarry in Chełm (Lublin region, Eastern Poland). Geologija 51, 70–75.
- Donner, J., 2005: The Quaternary History of Scandinavia. Cambridge University Press, Cambridge.
- Dowdeswell, J.A., Ottesen, D. & Rise, L., 2010: Rates of sediment delivery from the Fennoscandian Ice Sheet through an ice age. Geology 38, 3–6. doi:10.1130/G25523.1.
- Ebert, K., Hättestrand, C., Hall, A.M. & Alm, G., 2011: DEM identification of macro-scale stepped relief in arctic northern Sweden. Geomorphology 132, 339–350. doi:10.1016/j.geomorph.2011.05.021.
- Ehlers, J., 1981: Some aspects of glacial erosion and deposition in north Germany. Annals of Glaciology 2, 143–146. doi:10.3189/172756481794352513.
- Ehlers, J., Gibbard, P.L. & Hughes, P.D., 2018: Chapter 4 - Quaternary glaciations and chronology. In J. Menzies & J.J.M. van der Meer (eds.): Past Glacial Environments (Second Edition), 77–101. Elsevier, Amsterdam. ISBN 9780081005248. https://doi.org/10.1016/B978-0-08-100524-8.00003-8.
- Ehlers, J., Grube, A., Stephan, H.J. & Wansa, S., 2011: Chapter 13 - Pleistocene Glaciations of North Germany—New Results. In J. Ehlers, P.L. Gibbard, & P.D. Hughes (eds.): Developments in Quaternary Sciences, 149–162. Elsevier, Amsterdam.
- Eyles, N., Boyce, J. & Mohajer, A., 1993: The bedrock surface of the western Lake Ontario region: evidence of reactivated basement structures? Géographie physique et Quaternaire 47, 269–283. doi:10.7202/032957ar.
- Eyles, N., Clark, B.M., Kaye, B.G., Howard, K.W.F. & Eyles, C.H., 1985: The application of basin analysis techniques to glaciated terrains: an example from the Lake Ontario Basin, Canada. Geoscience Canada 12, 22–32.
- Fairchild, I.J., Bradby, L. & Spiro, B., 1994: Reactive carbonate in glacial systems: a preliminary synthesis of its creation, dissolution and reincarnation. In M. Deynoux, J. Miller, E. Domack, N. EYLES, I. Fairchild, & G. Young (eds.): Earth’s Glacial Record, 176–192. Cambridge University Press, Cambridge.
- Flodén, T., 1977: Tectonic lineaments in the Baltic from Gävle to Simrishamn. KBS Technical Report. 59. Kärnbränslesäkerhet, Stockholm.
- Flodén, T., Bjerkéus, M., Endler, R. & Lemke, W., 1996: Cretaceous sedimentary bedrock of the Darss Sill area, southern Baltic Sea. GFF 118, 80–80. doi:10.1080/11035899609546381.
- Flodén, T., Bjerkéus, M., Sturkell, E., Gelumbauskaitė, Ž., Grigelis, A., Endler, R., Lemke, W., Flodén, T., Bjerkéus, M. & Sturkell, E., 1997: Distribution and seismic stratigraphy of glacially incised valleys in the southern part of the Baltic. Proceedings of the Fourth Marine Geological Conference–the Baltic, Sveriges Geologiska Undersökning, Ser. Ca, 43–49.
- Fredén, C. (ed), 1994: Geology. National Atlas of Sweden. Geological Survey of Sweden, Stockholm, PN Publishing. 208.
- Funder, S., Demidov, I. & Yelovicheva, Y., 2002: Hydrography and mollusc faunas of the Baltic and the White Sea–North Sea seaway in the Eemian. Palaeogeography, Palaeoclimatology, Palaeoecology 184, 275–304. doi:10.1016/S0031-0182(02)00256-0.
- Gibbard, P. & Lewin, J., 2016: Filling the North Sea Basin: Cenozoic sediment sources and river styles. Geologica Belgica 19, 201–217. doi:10.20341/gb.2015.017.
- Glückert, G., 1974: Map of glacial striation of the Scandinavian ice sheet during the last (Weichsel) glaciation in northern Europe. Bulletin of the Geological Society of Finland 46, 1–8. doi:10.17741/bgsf/46.1.001.
- Gorlach, A., Kalm, V. & Hang, T., 2015: Thickness distribution of Quaternary deposits in the formerly glaciated part of the East European plain. Journal of Maps 11, 625–635. doi:10.1080/17445647.2014.954646.
- Górska, M., 2003: Nowe znaleziska narzutniaków porfiru rombowego z Oslo na terenie północno-zachodniej Polski. Przeglad Geologiczny 51, 580–585.
- Gozhik, P., Lindner, L. & Marks, L., 2012: Late Early and early Middle Pleistocene limits of Scandinavian glaciations in Poland and Ukraine. Quaternary International 271, 31–37. doi:10.1016/j.quaint.2010.07.027.
- Greenwood, S.L., Clason, C.C., Nyberg, J., Jakobsson, M. & Holmlund, P., 2017: The Bothnian Sea ice stream: early Holocene retreat dynamics of the south-central Fennoscandian Ice Sheet. Boreas 46, 346–362. doi:10.1111/bor.12217.
- Gupta, S., Collier, J.S., Garcia-Moreno, D., Oggioni, F., Trentesaux, A., Vanneste, K., De Batist, M., Camelbeeck, T., Potter, G. & Van Vliet-Lanoë, B., 2017: Two-stage opening of the Dover Strait and the origin of island Britain. Nature Communications 8, 1–12. doi:10.1038/ncomms15101.
- Hall, A.M., Ebert, K., Goodfellow, B.W., Hättestrand, C., Heyman, J., Krabbendam, M., Moon, S. & Stroeven, A.P., 2019a: Past and future impact of glacial erosion in Forsmark and Uppland. SKB TR-19-07. Svensk Kärnbränslehantering AB, Stockholm.
- Hall, A.M., Krabbendam, M., Goodfellow, B.W., Hätttestrand, C., Heyman, J., Palamakumbura, R.N., Stroeven, A. & Näslund, J.-O., in Press: Glacial ripping: geomorphological evidence from Sweden for a new process of glacial erosion. Geografiska Annaler. https://doi.org/10.1080/04353676.2020.1774244
- Hall, A.M., Krabbendam, M., Van Boeckel, M., Ebert, K., Hättestrand, C. & Heyman, J., 2019b: The sub-Cambrian unconformity in Västergötland: reference surface for glacial erosion of basement. Technical Report. SKB TR-19-21. Svensk Kärnbränslehantering AB, Stockholm.
- Hall, A.M., Riding, J. & Brown, J.F., 2016: The last glaciation in Orkney, Scotland: glacial stratigraphy, event sequence and flow paths. Scottish Journal of Geology 52, 90–102. doi:10.1144/sjg2016-002.
- Hansen, A. M., 1894: The glacial succession in Norway. The Journal of Geology, 2, 123–144.
- Heyman, J., Goodfellow, B.W., Stroeven, A.P., Hall, A.M., Caffee, M., Hättestrand, C., Ebert, K., Näslund, J.O., Hippe, K., Martel, S.J., Moon, S., Perron, J.T. & Stuart, A.J., 2019: Erosion of low-relief basement by the Fennoscandian ice sheet based on bedrock 10Be and 26Al. INQUA, Dublin.
- Hoffmann, K. & Meyer, K.-D., 1999: Indicator stone counts on Elsterian and Saalian sediments from eastern Germany. Geological Quarterly 43, 233–240.
- Hoffmann, K. & Piotrowski, J.A., 2001: Till mélange at Amsdorf, central Germany: sediment erosion, transport and deposition in a complex, soft-bedded subglacial system. Sedimentary Geology 140, 215–234. doi:10.1016/S0037-0738(00)00184-6.
- Houmark-Nielsen, M., 2004: The Pleistocene of Denmark: areview of stratigraphy and glaciation history. In J. Ehlers & P.L. Gibbard (eds.): Developments in Quaternary Sciences, 35–46, Volume 2, Part 1. Elsevier. ISBN 9780444514622, https://doi.org/10.1016/S1571-0866(04)80055-1.
- Houmark-Nielsen, M., 2007: Extent and age of Middle and Late Pleistocene glaciations and periglacial episodes in southern Jylland, Denmark. Bulletin of the Geological Society of Denmark 55, 9–35.
- Ignatius, H., Axberg, S., Niemistö, L. & Winterhalter, B., 1981: Quaternary geology of the Baltic Sea. In A. Voipio (ed.): The Baltic Sea, (54–121. Elsevier Oceanography, Amsterdam.
- Jakobsen, P.R., 1996: Distribution and intensity of glaciotectonic deformation in Denmark. Bulletin of the Geological Society of Denmark 42, 175–185.
- Kalm, V. & Gorlach, A., 2014: Impact of bedrock surface topography on spatial distribution of Quaternary sediments and on the flow pattern of late Weichselian glaciers on the East European Craton (Russian Plain). Geomorphology 207, 1–9. doi:10.1016/j.geomorph.2013.10.022.
- Karabanov, A.K., 2000: Glaciotectonics of Belarus. Geological Quarterly 44, 1–7.
- Karabanov, A.K. & Matveyev, A.V., 2011: Chapter 3 - The Pleistocene Glaciations in Belarus. In J. Ehlers, P.L. Gibbard, & P.D. Hughes (eds.): Quaternary Glaciations Extent and Chronology, 29–35. Elsevier, Amsterdam.
- Kirsimae, K., Jørgensen, P. & Kalm, V., 1999: Low-temperature diagenetic illite-smectite in Lower Cambrian clays in North Estonia. Clay Minerals 34, 151–163. doi:10.1180/000985599546000.
- Kleman, J., Hättestrand, C., Borgström, I. & Stroeven, A., 1997: Fennoscandian palaeoglaciology reconstructed using a glacial geological inversion model. Journal of Glaciology 43, 283–299. doi:10.1017/S0022143000003233.
- Kleman, J., Stroeven, A.P. & Lundqvist, J., 2008: Patterns of Quaternary ice sheet erosion and deposition in Fennoscandia and a theoretical framework for explanation. Geomorphology 97, 73–90. doi:10.1016/j.geomorph.2007.02.049.
- Knutsen, Å.R., 2018: Quantitative Comparison of Offshore Sediment Volumes and Onshore Erosion Potential in Norway in the Neogene and Quaternary. The University of Bergen, 65 p.
- Kramarska, R., 2006: Paleogen i neogen południowego Bałtyku i jego wybrzeża. Thesis, Central Geological Archives of the Polish Geological Institute, Warszawa. 139 p.
- Krzyszkowski, D., 1991: Saalian sediments of the Belchatow outcrop, central Poland. Boreas 20, 29–46. doi:10.1111/j.1502-3885.1991.tb00457.x.
- Krzyszkowski, D. & Ibek, M., 1996: Middle Pleistocene sedimentation and palaeogeography of the Dzierżoniów Basin, Sudetic Foreland, Southwestern Poland. Annales Societatis Geologorum Poloniae 66, 35–58.
- Lagerlund, E., Persson, K.M., Krzyszkowski, D., Johansson, P., Dobracka, E., Dobracki, R. & Panzig, W.-A., 1995: Unexpected ice flow directions during the Late Weichselian deglaciation of the South Baltic area indicated by a new lithostratigraphy in NW Poland and NE Germany. Quaternary International 28, 127–144. doi:10.1016/1040-6182(95)00028-H.
- Larson, G. & Schaetzl, R., 2001: Origin and evolution of the Great Lakes. Journal of Great Lakes Research 27, 518–546. doi:10.1016/S0380-1330(01)70665-X.
- Larson, S.-A., Tullborg, E.-L., Cederbom, C. & Stiberg, J.-P., 1999: Sveconorwegian and Caledonian foreland basins in the Baltic Shield revealed by fission-track thermochronology. Terra Nova-Oxford 11, 210–215. doi:10.1046/j.1365-3121.1999.00249.x.
- Lemke, W., Kuijpers, A., Hoffmann, G., Milkert, D. & Atzler, R., 1994: The Darss Sill, hydrographic threshold in the southwestern Baltic: late Quaternary geology and recent sediment dynamics. Continental Shelf Research 14, 847–870. doi:10.1016/0278-4343(94)90076-0.
- Lewandowski, J., 1988: Plejstocen środkowy w strefie doliny górnej Odry: brama Morawska-Kotlina Raciborska (próba syntezy). Przeglad Geologiczny 36, 465–474.
- Lidmar-Bergström, K., 1995: Relief and saprolites through time on the Baltic Shield. Geomorphology 12, 45–61. doi:10.1016/0169-555X(94)00076-4.
- Lidmar-Bergström, K., 1996: Long term morphotectonic evolution in Sweden. Geomorphology 16, 33–59. doi:10.1016/0169-555X(95)00083-H.
- Lidmar-Bergström, K., Bonow, J.M. & Japsen, P., 2012: Stratigraphic Landscape Analysis and geomorphological paradigms: scandinavia as an example of Phanerozoic uplift and subsidence. Global and Planetary Change 100, 153–171. doi:10.1016/j.gloplacha.2012.10.015.
- Lidmar-Bergström, K. & Olvmo, M., 2015: Plains, steps, hilly relief and valleys in northern Sweden – review, interpretations and implications for conclusions on Phanerozoic tectonics. SGU Research Paper, C 838, 1–42.
- Liivamägi, S., Somelar, P., Mahaney, W.C., Kirs, J., Vircava, I. & Kirsimäe, K., 2014: Late Neoproterozoic Baltic paleosol: intense weathering at high latitude? Geology 42, 323–326. doi:10.1130/G35209.1.
- Lindner, L. & Astapova, S.D., 2000: The age and geological setting of Pleistocene glacigenic beds around the border between Poland and Belarus. Geological Quarterly 44, 187–198.
- Lindner, L., Bogutsky, A., Gozhik, P., Marks, L., Łanczont, M. & Wojtanowicz, J., 2006: Correlation of Pleistocene deposits in the area between the Baltic and Black Sea, Central Europe. Geological Quarterly 50, 195–210.
- Lindner, L., Gozhik, P., Marciniak, B., Marks, L. & Yelovicheva, Y., 2004: Main climatic changes in the Quaternary of Poland, Belarus and Ukraine. Geological Quarterly 48, 97–114.
- Lindner, L. & Marks, L., 2008: Pleistocene stratigraphy of Poland and its correlation with stratotype sections in the Volhynian Upland (Ukraine). Geochronometria 31, 31–37. doi:10.2478/v10003-008-0014-9.
- Lindner, L. & Marks, L., 2015: Early and Middle Pleistocene fluvial series in northern foreland of the Carpathians (Poland and Ukraine) and their relation to Dnistr River terraces. Quaternary International 357, 22–32. doi:10.1016/j.quaint.2014.09.053.
- Lindner, L., Marks, L. & Nita, M., 2013: Climatostratigraphy of interglacials in Poland: middle and Upper Pleistocene lower boundaries from a Polish perspective. Quaternary International 292, 113–123. doi:10.1016/j.quaint.2012.11.018.
- Mark, D.F., Lindgren, P. & Fallick, A.E., 2014: A high-precision 40Ar/39Ar age for hydrated impact glass from the Dellen impact, Sweden. Geological Society, London, Special Publications 378, 349–366. doi:10.1144/SP378.22.
- Marks, L., Dzierżek, J., Janiszewski, R., Kaczorowski, J., Lindner, L., Majecka, A., Makos, M., Szymanek, M., Tołoczko-Pasek, A. & Woronko, B., 2016: Quaternary stratigraphy and palaeogeography of Poland. Acta Geologica Polonica 66, 410–434. doi:10.1515/agp-2016-0018.
- Marks, L., Gałązka, D., Krzymińska, J., Nita, M., Stachowicz-Rybka, R., Witkowski, A., Woronko, B. & Dobosz, S., 2014: Marine transgressions during Eemian in northern Poland: A high resolution record from the type section at Cierpięta. Quaternary International 328, 45–59. doi:10.1016/j.quaint.2013.12.007.
- Marks, L., Karabanov, A., Nitychoruk, J., Bahdasarau, M., Krzywicki, T., Majecka, A., Pochocka-Szwarc, K., Rychel, J., Woronko, B., Zbucki, Ł., Hradunova, A., Hrychanik, M., Mamchyk, S., Rylova, T., Nowacki, Ł. & Pielach, M., 2018: Revised limit of the Saalian ice sheet in central Europe. Quaternary International 478, 59–74. doi:10.1016/j.quaint.2016.07.043.
- Marks, L. & Pavlovskaya, I.E., 2003: The Holsteinian Interglacial river network of mid‐eastern Poland and western Belarus. Boreas 32, 337–346. doi:10.1080/03009480310001975.
- Miettinen, A., Rinne, K., Haila, H., Hyvarinen, H., Eronen, M., Delusina, I., Kadastik, E., Kalm, V. & Gibbard, P., 2002: The marine Eemian of the Baltic: new pollen and diatom data from Peski, Russia, and Pohja-Uhtju, Estonia. Journal of Quaternary Science 17, 445–458. doi:10.1002/jqs.706.
- Morawski, W., 2009: Differences in the regional stratigraphy of NE Poland caused by vertical movements due to glacioisostasy. Geologos 15, 235–250. doi:10.2478/v10118-009-0006-x.
- Moreau, J., Huuse, M., Janszen, A., Van Der Vegt, P., Gibbard, P.L. & Moscariello, A., 2012: The glaciogenic unconformity of the southern North Sea. Geological Society, London, Special Publications 368, 99–110. doi:10.1144/SP368.5.
- Näslund, J.O., 2006: Climate and climate-related issues for the safety assessment SR-Can. SKB TR-06-23. Svensk Kärnbränslehantering AB, Stockholm.
- Nawrocki, J. & Becker, A., 2019: Geological Atlas of Poland. Polish Geological Institute – National Research Institute, Warszawa.
- Nielsen, A.T. & Schovsbo, N.H., 2006: Cambrian to basal Ordovician lithostratigraphy in southern Scandinavia. Bulletin of the Geological Society of Denmark 53, 47–92.
- Noormets, R. & Flodén, T., 2002: Glacial deposits and ice-sheet dynamics in the north-central Baltic Sea during the last deglaciation. Boreas 31, 362–377. doi:10.1080/030094802320942572.
- Obst, K., Nachtweide, C. & Müller, U., 2017: Late Saalian and Weichselian glaciations in the German Baltic Sea documented by Pleistocene successions at the southeastern margin of the Arkona Basin. Boreas 46, 18–33. doi:10.1111/bor.12212.
- Ottesen, D., Dowdeswell, J.A. & Bugge, T., 2014: Morphology, sedimentary infill and depositional environments of the Early Quaternary North Sea Basin (56°–62°N). Marine and Petroleum Geology 56, 123–146. doi:10.1016/j.marpetgeo.2014.04.007.
- Overeem, I., Weltje, G., Bishop-Kay, C. & Kroonenberg, S., 2001: The Late Cenozoic Eridanos delta system in the Southern North Sea Basin: a climate signal in sediment supply? Basin Research 13, 293–312. doi:10.1046/j.1365-2117.2001.00151.x.
- Overweel, C.J., 1977: Distribution and transport of Fennoscandinavian indicators: A synthesis of data from the literature leading to a reconstruction of a pattern of flowlines and ice margins of the Scandinavian ice sheets. Scripta Geologica 43, 1–117.
- Påsse, T., 2004: The amount of glacial erosion of the bedrock. SKB TR-04-25. Svensk kärnbränslehantering AB, Stockholm.
- Patruno, S., Scisciani, V., Helland-Hansen, W., D’intino, N., Reid, W. & Pellegrini, C., 2020: Upslope‐climbing shelf‐edge clinoforms and the stepwise evolution of the northern European glaciation (lower Pleistocene Eridanos Delta system, U.K. North Sea): when sediment supply overwhelms accommodation. Basin Research 32, 224–239. doi:10.1111/bre.12379.
- Patton, H., Hubbard, A., Andreassen, K., Winsborrow, M. & Stroeven, A.P., 2016: The build-up, configuration, and dynamical sensitivity of the Eurasian ice-sheet complex to Late Weichselian climatic and oceanic forcing. Quaternary Science Reviews 153, 97–121. doi:10.1016/j.quascirev.2016.10.009.
- Pedersen, S.A.S. & Gravesen, P., 2009: Structural development of Maglevandsfald: a key to understanding the glaciotectonic architecture of Møns Klint, SE Denmark. Geological Survey of Denmark and Greenland Bulletin 17, 29–32. doi:10.34194/geusb.v17.5007.
- Phemister, J., 1926: The distribution of Scandinavian boulders in Britain. Geological Magazine 63, 433–454. doi:10.1017/S0016756800085393.
- Piotrowski, J.A., 1994: Tunnel-valley formation in northwest Germany—geology, mechanisms of formation and subglacial bed conditions for the Bornhöved tunnel valley. Sedimentary Geology 89, 107–141. doi:10.1016/0037-0738(94)90086-8.
- Pitkäranta, R., 2013: Lithostratigraphy and Age of Pre-Late Weichselian Sediments in the Suupohja Area, Western Finland. Ph.D. thesis. University of Turku, Turku. 66 p.
- Puura, V., Flodén, T. & Mokrik, R., 2003: The Baltic Sea basin in the geology of Fennoscandia and Baltic region. Litosfera 7, 134–137.
- Rasmussen, E.S., 2018: Discussion of “Eocene to mid-Pliocene landscape evolution in Scandinavia inferred from offshore sediment volumes and pre-glacial topography using inverse modelling”(Pedersen et al. 2018. Geomorphology, 303: 467–485). Geomorphology 328, 222–224. doi:10.1016/j.geomorph.2018.06.022.
- Rasmussen, E.S., Dybkjær, K. & Piasecki, S., 2010: Lithostratigraphy of the upper Oligocene–Miocene succession of Denmark. Geological Survey of Denmark and Greenland Bulletin 22, 1–92. doi:10.34194/geusb.v22.4733.
- Rasser, M.W., Harzhauser, M., Anistratenko, O.Y., Anistratenko, V.V., Bassi, D., Belak, M., Berger, J.P., Bianchini, G., Čičić, S. & Ćosović, V., 2008: Palaeogene and Neogene. The Geology of Central Europe 2, 1031–1139.
- Rattas, M. & Kalm, V., 2004: Glaciotectonic deformation patterns in Estonia. Geological Quarterly 48, 15–22.
- Rosentau, A., Bennike, O., Uscinowicz, S. & Miotk-Szpiganowicz, G., 2017: The Baltic Sea Basin. In N.C. Flemming, J. Harff, D. Moura, A. Burgess, & G.N. Bailey (eds.): Submerged Landscapes of the European Continental Shelf: Quaternary Paleoenvironments, 103–134. John Wiley & Sons, Chichester.
- Ruszczyńska-Szenajch, H., 1985: Origin and age of the large-scale glaciotectonic structures in central and eastern Poland. Annales Societatis Geologorum Poloniae 55, 307–332.
- Ruszczyńska-Szenajch, H., 1987: The origin of glacial rafts: detachment, transport, deposition. Boreas 16, 101–112. doi:10.1111/j.1502-3885.1987.tb00761.x.
- Sadowski, E.-M., 2017: Towards a New Picture of the ’Baltic Amber Forest’-Flora, Habitat Types, and Palaeoecology. Georg-August-Universität Göttingen.
- Salamon, T., 2009: Origin of Pleistocene outwash plains in various topographic settings, southern Poland. Boreas 38, 362–378. doi:10.1111/j.1502-3885.2008.00049.x.
- Salonen, V.-P. & Glückert, G., 1992: Late-Weichselian glacial activity and sediments in southwestern Finland. Sveriges Geologiska Undersökning Ca Research Paper 81, 313–318.
- Schuddebeurs, A., 1987: De verspreiding over Europa van gidsgesteenten uit het Oslogebied en begeleidende zwerfstenen. Grondboor & Hamer 41, 114–144.
- Sejrup, H.P., Aarseth, I., Haflidason, H., Løvlie, R., Bratten, Å., Tjøstheim, G., Forsberg, C.F. & Ellingsen, K.L., 1995: Quaternary of the Norwegian Channel: glaciation history and palaeoceanography. Norsk Geologisk Tidsskrift 75, 65–87.
- Šliaupa, S. & Hoth, P., 2011: Geological Evolution and Resources of the Baltic Sea Area from the Precambrian to the Quaternary. In J. Harff, S. Björck, & P. Hoth (eds.): The Baltic Sea Basin, 13–51. Springer, Berlin Heidelberg.
- Słodkowska, B. & Gałązka, D., 2015: Osady paleogenu i neogenu w wielkoskalowych strukturach glacitektonicznych Wzgórz Dylewskich. Biuletyn Panstwowego Instytutu Geologicznego 461, 251–293. doi:10.5604/08676143.1142101.
- Smed, P., 2016: Sten i det danske landskab, 271 p. Højers Forlag, Aalborg.
- Smed, P. & Ehlers, J., 2002: Steine aus dem Norden: geschiebe als Zeugen der Eiszeit in Norddeutschland. Borntraeger, Berlin. 198 p.
- Sømme, T.O., Skogseid, J., Embry, P. & Løseth, H., 2019: Manifestation of Tectonic and Climatic Perturbations in Deep-Time Stratigraphy – an Example From the Paleocene Succession Offshore Western Norway. Frontiers in Earth Science 7, 303. doi:10.3389/feart.2019.00303.
- Tuuling, I., 2017: Paleozoic rocks structure versus Cenozoic cuesta relief along the Baltic Shield–East European Platform transect. Geological Quarterly 61, 396–412. doi:10.7306/gq.1342.
- Tuuling, I. & Flodén, T., 2001: The structure and relief of the bedrock sequence in the Gotland-Hiiumaa area, northern Baltic Sea. GFF 123, 35–49. doi:10.1080/11035890101231035.
- Tuuling, I. & Flodén, T., 2016: The Baltic Klint beneath the central Baltic Sea and its comparison with the North Estonian Klint. Geomorphology 263, 1–18. doi:10.1016/j.geomorph.2016.03.030.
- Uscinowicz, S., 2003: Relative sea level changes, glacio-isostatic rebound and shoreline displacement in the Southern Baltic. Polish Geological Institute Special Papers 10, 1–79.
- Uutela, S.A., 1991: Age and dispersal of sedimentary erratics on the coast of southwestern Finland. Bulletin of the Geological Society of Finland 349, 1–100.
- Van Balen, R.T. & Heeremans, M., 1998: Middle Proterozoic-early Palaeozoic evolution of central Baltoscandian intracratonic basins: evidence for asthenospheric diapirs. Tectonophysics 300, 131–142. doi:10.1016/S0040-1951(98)00237-6.
- Van Der Wateren, F.M., 1999: Structural geology and sedimentology of the Heiligenhafen till section, Northern Germany. Quaternary Science Reviews 18, 1625–1639. doi:10.1016/S0277-3791(98)00120-6.
- Widera, M., 2000: Stratigraphy and lithology of Quaternary sediments in the Kleczew region and in key sections of the eastern Wielkopolska Lowland, central Poland. Geological Quarterly 44, 211–220.
- Woźniak, P.P. & Czubla, P., 2015: The Late Weichselian glacial record in northern Poland: A new look at debris transport routes by the Fennoscandian Ice Sheet. Quaternary International 386, 3–17. doi:10.1016/j.quaint.2015.01.014.
- Woźniak, P.P., Czubla, P., Wysiecka, G. & Drapella, M., 2009: Petrographic composition and directional properties of tills on the NW surroundings of Gdańsk Bay, Northern Poland. Geologija 51, 59–67. doi:10.2478/v10056-009-0007-z.
- Zandstra, J.G., 1971: Geologisch onderzoek in de stuwwal van de oostelijke Veluwe bij Hattem en Wapenveld. Mededelingen Rijks Geologische Dienst, NS 22, 215–260.
- Zandstra, J.G., 1983: Fine gravel, heavy mineral and grain-size analyses of Pleistocene, mainly glacigenic deposits in the Netherlands. In J. Ehlers (ed.): Glacial Deposits in North-West Europe, 361–377 p. Balkema, Rotterdam.