ABSTRACT
The Solstad copper deposit, located in SE Sweden, is hosted by a quartz-rich rock sliver surrounded by a granite belonging to the 1.8 Ga Transscandinavian Igneous Belt. Ore petrographic studies have revealed a number of previously unrecognized opaque phases, including several Co phases, selenides and tellurides. Based on an in situ U-Pb investigation of zircons from a mineralized sample, it is suggested that zircons have a detrital origin and that the quartz-rich host rock is a xenolith belonging to the c. 1.88–1.86 Ga Västervik quartzite formation. A low-radiogenic galena sample implies that the source for the metals in the ore has a primitive origin, probably the basaltic lavas (now amphibolites) that are intercalated in the Västervik quartzite. Fluid inclusion studies in quartz distinguish four distinct ore fluids: (1) a hypersaline halite-bearing aqueous fluid related to an early (1.85–1.86 Ga) chalcopyrite depositional stage, (2) a subsequent CO2-rich fluid, that deposited native gold, tellurides, selenides and bismuthinite, developed (at ≥1.8 Ga) as a result of a phase separation, (3), a moderate- to high-salinity aqueous fluid did also develop at this event and led to the deposition of bornite and (4) a concluding, low-salinity aqueous fluid stage (at ≤1.8 Ga) caused oxidation to covelline and digenite of previously formed phases. It is proposed that the Solstad deposit and other Cu ± Co-rich sulphide (± magnetite) occurrences in the Västervik region along the southernmost margin of the 1.9–1.8 Ga Svecofennian Domain, represent a distinct ore type associated with quartzites and amphibolites.
Introduction
The 1.9–1.8 Ga Bergslagen mining province in south-central Sweden is one of the most famous ore districts in Europe. Several huge deposits, such as the sulphide ores at Falun, Garpenberg, Sala and Zinkgruvan and the oxide ores at Grängesberg, Norberg and Långban, have produced vast quantities of copper, zinc, lead, silver, iron and manganese of major economic importance throughout the history. A common feature of all these ores is a close spatial, and genetic, association to Svecofennian (c. 1900 Ma) felsic metavolcanic rock and/or marble formations (Allen et al. Citation1996; Kumpulainen et al. Citation1996; Stephens et al. Citation2009).
A number of significant, but much smaller, mining operations have also been undertaken in a heterogeneous metallogenetic sub-province in south-eastern Sweden, immediately to the south of Bergslagen. These ores occur in a wide range of geological settings and include in particular copper and iron ores in 1.9 Ga amphibolites in the Åmmeberg-Valdemarsvik region and copper and cobalt ores in <1.9 Ga quartzites in the Västervik region. Some of the sites in these regions are famous for very early (although not the first) discoveries of cobalt and selenium and for being type localities for a number of mineral phases, such as berzelianite (Cu2Se), crookesite (Cu7(Tl,Ag)Se4) and gladite (PbCuBi5S9).
The Solstad copper deposit was a significant producer of copper ore already during the seventeenth century. It is located ca. 20 km south of Västervik and is the southernmost occurrence of this sub-province, located close to the contact between the Västervik quartzites and the 1.8–1.6 Ga Transscandinavian Igneous Belt (TIB; A). In spite of its historic importance and high Cu grades, the geological characteristics of the deposit are only briefly documented. The purpose of this contribution is to shed light on this deposit with respect to geological setting, ore mineral paragenesis, fluid inclusions and certain isotope aspects, in order to provide basic information on a key example of this poorly defined metallogenetic ore province.
Figure 1. A. Bedrock geology of the transition from the Svecofennian Domain to the Transscandinavian Igneous Belt. Town names referred to in the text are abbreviated as follows; Ask = Askersund, Eks = Eksjö, Frö = Fröderyd, Jön = Jönköping, Kal = Kalmar, Lin = Linköping, Lof = Loftahammar, Osk = Oskarshamn, Val = Valdemarsvik, Vet = Vetlanda, Väs = Västervik, and Åtv = Åtvidaberg. Ål = Ålatorp Zn-Pb-Cu occurrence and TIB = Transscandinavian Igneous Belt. Letters L (Långban), F (Falun) and Z (Zinkgruvan) refer to major ore deposits in the Bergslagen ore district (outlined with a dashed line on the inset map). B. Regional geology of the border zone between the Svecofennian domain and the TIB-dominated terrain. LLDZ is the Loftahammar-Linköping Deformation Zone. Stars denote certain significant copper-bearing mineralisations in the region; 1 = Skrikerum, 2 = Rumma, 3 = Gladhammar, 4 = Skälö. The cross symbol marks the location of the Solstad Cu deposit. C. The bedrock geology close to the Solstad copper ore deposit that is located just at the Baltic Sea coast-line. Also shown are local faults and lineaments and, in addition, some local Cu occurrences and showings are marked. Two linear trends (dotted), drawn to follow the general NW-SE fault/shear/ore strike direction, are tentatively connecting individual occurrences.
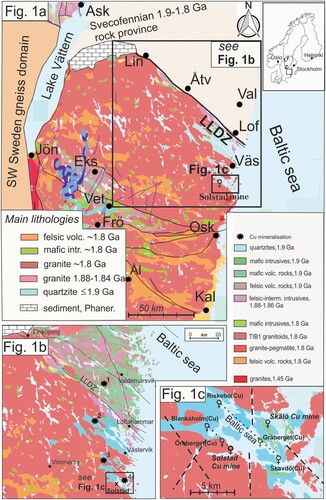
Geological and metallogenetic background
The southeastern part of the Fennoscandian Shield is composed of two main crustal components: the 1.9–1.8 Ga Svecofennian Domain and the 1.8–1.6 Ga TIB (Stephens et al., Citation2009). Some of the lithological associations in the Svecofennian Domain are endowed with a wealth of metal concentrations while only a few and economically insignificant metal concentrations are known in the TIB units.
The economically most important Svecofennian ore district is Bergslagen, where many hundreds of Fe-Cu-Zn-Pb-Ag sulphide ores and Fe oxide ores occur. They are hosted by metamorphosed Svecofennian (1.91–1.89 Ga) felsic volcanic rocks and limestones, which formed in a shallow-marine back-arc extensional basin developed on continental crust (Allen et al. Citation1996). The volcanic rocks are co-magmatic with early Svecofennian granitoids with a volcanic arc affinity (Stephens et al. Citation2009). Reviews of the formation of different types of sulphide and iron oxide ores have been presented by e.g., Allen et al. (Citation1996), Jonsson (Citation2013), Jansson et al. (Citation2017) and Kampmann et al. (Citation2018). The southernmost part of Bergslagen (the Zinkgruvan region) is in many ways similar to other parts of this ore district, particularly in the lower sections of the local stratigraphy, whereas the upper carry more abundant mafic igneous components and show a transition from a thick sequence of arkosic, deltaic sediments to turbidites indicative of a shift in environment from a back-arc environment to ocean opening (Kumpulainen et al. Citation1996).
The southernmost parts of the Svecofennian Domain are found in a belt extending from Tiveden (northwest of lake Vättern) via Åtvidaberg to Valdemarsvik at the Baltic Sea (A). Well-preserved pillow lava structures and mafic volcanoclastic rocks with basaltic to andesitic composition demonstrate a submarine primitive volcanic arc environment in the Tiveden area (Björklund & Weihed Citation1997). The metamorphic grade and deformation have obliterated almost all primary features in the basaltic to andesitic metavolcanic rocks in the Åtvidaberg-Valdemarsvik area, and the metavolcanic rocks are interfolded with early Svecofennian granitoids and 1.86–1.85 Ga TIB granitoids (Wikström & Persson Citation2002; Stephens & Andersson Citation2015).
The Svecofennian evolution in southern Sweden ended at c. 1.87–1.86 Ga with deposition in the Västervik area of extensive fluvial, tidal and turbiditic successions along a continental margin (Russell Citation1967; Sultan et al. Citation2005; Sultan & Plink-Björklund Citation2006; Vollbrecht & Leiss Citation2008; Nolte et al. Citation2011; Kleinhanns et al. Citation2012). These well-preserved quartzitic metasedimentary rocks show structures like cross-bedding and ripple marks (Russell Citation1967; Sultan & Plink-Björklund Citation2006). U-Pb investigations of detrital zircons show that the sediments were derived from a number of Archaean and Palaeoproterozoic sources and deposited from 1.88–1.86 Ga (Claesson et al. Citation1993; Sultan et al. Citation2005). Basaltic lava extrusions with well-preserved amygdules are interlayered with the sediments (Kresten Citation1972) and have a geochemical signature suggesting a continental environment (Wik et al. Citation2005; Sundblad et al. Citation2021). This mixed Västervik sedimentary-volcanic sequence is host to a number of Cu-(Co) rich sulphide (and oxide) mineralizations, e.g., at Gladhammar, Rumma and Skälö (Tegengren et al. Citation1924; Bruun et al. Citation1991). The most common sulphides in this ore type are pyrite and chalcopyrite, but bornite and chalcocite are locally abundant, e.g., at Skälö. The high Co contents at Gladhammar formed the base for one of the first cobalt production operations in the world in the eighteenth century.
The TIB is characterized by potassium-rich coarse-grained granitoids and co-magmatic terrestrial felsic volcanic rocks (Larsson & Berglund Citation1992; Högdahl et al. Citation2004). It can be subdivided into four chronological units, called TIB 0 (1855–1845 Ma), TIB 1a (1808–1794 Ma), TIB 1b (1793–1769 Ma) and TIB 2 (1700–1670 Ma) (Salin et al. Citation2019; Sundblad et al. Citation2021). The TIB 0 generation intruded along the entire SW (present day coordinates) margin of the Svecofennian Domain, from the Tiveden-Askersund to the Loftahammar regions (Wikström & Andersson Citation2004). It intruded into the amphibolites of the Valdemarsvik region and the Västervik quartzites, and an intrusion age of 1859 ± 9 Ma (Bergström et al. Citation2002) obtained for a deformed granite at Loftahammar can thus be considered as a minimum age for the Västervik quartzites. The TIB 1a generation forms a major batholith exposed between lake Vättern and the coast line of the Baltic Sea but it can also be followed under the Phanerozoic cover of northern Öland and southern Gotland (Salin et al. Citation2019). No major metallic mineralization is connected with the TIB units proper but several small-scale Zn-Pb-Cu rich magmatic-hydrothermal deposits are known, at e.g., Ramnebo in the TIB 1a granitoids (Ahl Citation1989) and Ålatorp in 1.80 Ga old volcanic TIB 1 successions.
A 1.85 Ga marine rifted arc environment with a number of small Cu-Zn-Pb mineralizations has been recognized at Fröderyd (Sundblad et al. Citation1997; Salin et al. Citation2021). Subsequent (1.83–1.82 Ga) formation of tonalitic crust (Mansfeld Citation1996; Åhäll et al. Citation2002) was followed by still later clastic sedimentation in the Eksjö-Vetlanda region (B). A major NW-SE shear zone (the Loftahammar-Linköping Deformation Zone; LLDZ), constituting a ca. 10 km wide plastic zone with brittle over-printing, runs along the contact between the Svecofennian units and the 1.86–1.85 Ga TIB 0 granitoids (A; Beunk & Page Citation2001; Beunk et al. Citation1996; Wik et al. Citation2005).
Small plutons of granites intruded into the TIB 1b granitoids in the Götemar-Figeholm region at ca. 1.45 Ga (Åhäll Citation2001).
The Precambrian crust in the southern Baltic Sea region is overlain by an extensive cover of Cambro-Silurian sedimentary rocks (Sopher et al. Citation2016). Cambrian sandstone dykes also occur at scattered localities in the Precambrian crystalline basement along the coast line of the Baltic Sea. The Precambrian crust and these sedimentary dykes were overprinted by Early Devonian fracturing and associated low-temperature hydrothermal activity resulting in galena-fluorite-calcite veins along the entire coastal region of southeastern Sweden (Alm & Sundblad Citation2002).
Ores at Solstad and its immediate surroundings
The first mining operations at Solstad ((B, C) took place during the seventeenth century, after which the mine was intermittently active before it finally was closed in 1919. The ore had an estimated average copper content of 9% and in total 89,400 metric tonnes copper ore were mined from ten different shafts (Tegengren et al. Citation1924). The ore zone was up to 6 m thick and mined to a depth of 356 m.
The Solstad area is located 500 m to the southwest of the contact between coarse-grained and megacrystic granitoids of the Vimmerby TIB 1a batholith and the Västervik quartzites (C; Tegengren et al. Citation1924). The local granitoid at Solstad is a barren, coarse-grained and essentially unmetamorphosed and megacrystic granite with a greyish-reddish colour. The ore zone occurs in a 30–40 m wide and ca. 140 m long NW-trending and SW-dipping disc-shaped body enclosed in the granite (). This body is dominated by a quartz-rich rock, which has been interpreted as a xenolith of hydrothermally altered Västervik quartzite (Nordenström Citation1876; Tegengren et al. Citation1924). Eklund (Citation1925) describes different varieties of the quartzite, where a dominant reddish, feldspar-rich, layered type shows a continuous gradation towards unaltered TIB granite. Occasionally, the feldspar is strongly altered to sericite. The ore-bearing quartzite is grey and does not contain feldspar and only minor quantities of mica and chlorite. In the northern part of the mined area, the copper grade gradually decreases and the quartzite gets darker due to a higher content of biotite.
Figure 2. A schematic vertical section, following an ESE-WNW direction, of the Solstad copper ore (modified from Nordenström Citation1876). The vertical and horisontal scales are approximately correct. The ore is cut by several faults, and chlorite gouges are often observed to follow faults and diabase dykes.
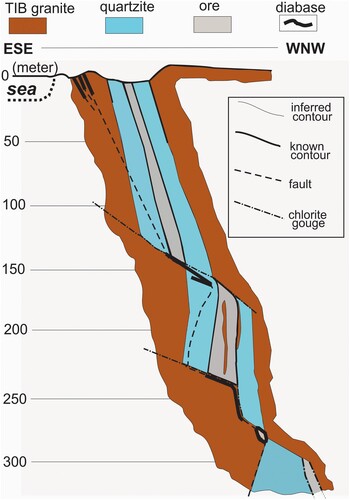
Nordenström (Citation1876) noted that chalcopyrite, together with smaller amounts of pyrite and pyrrhotite, were the main sulphide minerals, typically forming an even impregnation. Higher metal grades are found near fractures, and the ore has locally a more massive appearance with the ore minerals occuring together with chlorite, hornblende and biotite. Post-ore, brittle faults are also common in the ore zone and are interpreted as reverse faults. Diabases, enclosed by thin chlorite coatings, often occupy these faults ().
Field work, sampling and analytical techniques
The bedrock in the immediate surroundings to the Solstad mine is well exposed and these outcrops were briefly mapped. Systematic sampling of the ore types is, however, impossible due to the fact that the shafts are waterfilled. As a consequence, most of the samples were collected from the mine dumps and in three cases from the collections of the Swedish Museum of Natural History (NRM), Stockholm ().
Altogether twenty-one polished thin sections were studied in a reflected light microscope. Micro-chemical scanning electron microscope (SEM) analyses using an EG&G 5000 spectrometer attached to a Hitachi 4200 SEM microscope at Stockholm University, equipped with an energy-dispersive X-ray spectroscopy unit (EDS), were also utilized in the process of identifying minerals, as well as a Philips powder, X-ray diffractometer (XRD). A semi-quantitative estimate of the metal contents in the ore was obtained from emission spectral analysis performed at the Geological Survey of Estonia.
Isotope analysis
The rationale behind the U-Pb zircon work was to clarify the nature of the protolith of the quartz-rich host rock. However, the effects of hydrothermal alteration impose problems to find an ideal sample and, in this situation, a typical mineralized specimen (dump sample D, ) was selected. About 0.5 kg of this sample was crushed and processed with heavy liquids and magnetic techniques resulting in a high yield of zircons of which grains selected for isotopic analysis were hand-picked. The U-Pb in situ isotope work was carried out by secondary ion mass spectrometry (SIMS) using an ion microprobe (NORDSIM facility) at the Department of Geological Sciences at NRM, Stockholm. Zircons were mounted in epoxy resin and coated with gold. Cracks and inclusions of other minerals were avoided during analysis. The SIMS analyses, utilizing 35–40 µm spot sizes, followed well-established routines described in Whitehouse et al. (Citation1999) and Whitehouse & Kamber (Citation2005). Basically, negative ions were analysed on a Cameca 1270 using an oxygen flow to increase the yield. Tabulated errors on ratios and ages are 1σ and include propagated errors on the standard (Geostandards 91500; 1065 Ma, Wiedebeck et al. Citation1995) analyses.
The NORDSIM instrument was also used for doing in situ, spatially resolved Pb-Pb analyses of galena in two polished sections (C5 and G1 described in more detail below), previously prepared as epoxy mounts. The set-up for the Pb-Pb runs followed essentially the procedures described in Zetterström (Citation2001). The secondary ions were sputtered from the sample surface by a ca. 4 nA primary O2- beam focused to a 25 μm diameter spot. A single electron multiplier was used in ion counting mode to measure the secondary ion beam intensities in the mass-switching sequence; 204Pb, 204.2 (background), 206Pb, 207Pb and 208Pb. In an analysis generating data from an individual spot, each lead isotopic ratio was determined twelve times, and two or three spots per grain were analysed in this way. The magnitude of the mass bias affecting raw data was known through runs of an in-house galena standard with an isotopic composition accurately determined from TIMS analyses. A series of runs on this standard suggests that the mass bias on this instrument is amounting to 0.46 ± 0.01%/amu (Zetterström Citation2001) as defined from highly reproducible data collected during three different sessions. When estimating the typical analytical error, we cannot, however, exclude the possibility that there exists some isotopic variations between grains. Therefore, we choose to compare data from different spots in the same grain, and we find that the within-run error is consistently close to ca. 0.20 (Poisson % error). Combined with the uncertainty in the mass bias which is slightly more pronounced for ratios involving the less abundant 204Pb isotope, we estimate the total errors in the relevant isotope ratios to be less than ± 0.3% (1 sigma).
Fluid inclusion analysis
Quartz-rich parts of dump samples D, C and G (described in ), were prepared as 150 μm thick, doubly polished thin sections. The fluid inclusions were studied at the Department of Geological Sciences, Stockholm University by optical microscopy, microthermometry and Raman microspectroscopy. Detailed petrography and mapping of fluid inclusion assemblages in the quartz were performed to document the distribution of fluid inclusions and their association to different sulphide phases. Microthermometric analyses were carried out in the temperature range −196°C to 600°C on a Linkam THM 600 heating and cooling stage. The accuracy (by comparing with standard samples) is better than 0.5°C for the low-temperature range and better than 5°C at high temperatures. The stage was calibrated with synthetic fluid inclusion standards (SynFlinc).
Raman spectroscopic analyses were performed with a confocal laser Raman spectrometer (Horiba instrument LabRAM HR 800) at the Department of Geological Sciences, Stockholm University. Acquisitions were obtained with a multichannel air-cooled (−70°C) 1024 × 256 pixel CCD (charge-coupled device) array detector and with 1800 lines/mm grating. A 514 nm excitation of an Argon laser (Melles Griot 543) was used with a laser power at the sample surface of 1 mW. The laser beam was focused through an Olympus BX41 microscope with a 100x objective to obtain a spot size of about 1 µm. The spectral resolution was ∼0.4 cm−1/pixel. Analyses were made with detection accumulated 10 times with a measuring time of 10 s. The accuracy of the instrument was controlled by repeated use of a silicon wafer calibration standard with a characteristic Raman line at 520.7 cm−1. The Raman spectra were achieved with LabSpec 5 software.
Results
Setting and ore petrology
The outcrops adjacent to the mine are dominated by a K-rich, coarse-grained granitoid without any sign of regional deformation. However, a weak foliation along distinct WNW-trending local fracture planes could be identified, which relate to rather common NW-SE- and N-S-trending lineaments that are interpreted from geophysical data (stippled lines in C). Moderate deformation in the ore zone, involving strike-slip movements and some minor faults, was also reported by Tegengren et al. (Citation1924).
Almost all dump samples from the ore zone are dark, quartz-rich and variably mineralized. Many of these contain one essentially barren (either quartz-dominated or more granitic) part, and one more or less mineralized part, often with a relatively sharp contact. Some important features of the ore parageneses are given in and . A disseminated, low-grade mineralization is omnipresent in selected samples with richer ore parts connected to fissures and joints. shows microscopic images of a range of minerals typifying the identified ore assemblages.
Figure 3. Microphotos of typical ore minerals and parageneses. A. Subhedral Co-rich pyrite (py) adjacent to more anhedral Co-free pyrite. B. Clausthalite (cla) intergrown with native Te enclosed in bornite (bor). Bornite is typically containing lamellae of chalcopyrite (cpy). digenite (díg) is seen along cracks. C. Galena (gal) intergrown with pyrite (py) and subordinate amounts of chalcopyrite (cpy). D. Hessite (hes) is intergrown with wittichenite (wit) both associated with bornite and isolated grains in a silicate matrix. Digenite (dig) is seen along borders to bornite, whereas gold (Au) occurs partly in contact with wittichenite (wit). E. An aggregate of krennerite (kre) and sylvanite (syl) is seen associated with gold (Au) in a bornite-dominated paragenesis. Covelline (cov) and digenite (dig) are concentrated along cracks. F. Growth of covelline at the expense of bornite. The magnetite grain (mt) is partly martitisised.
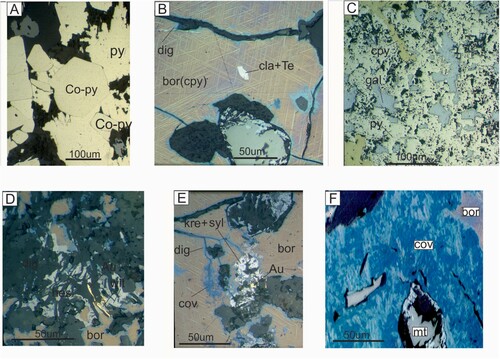
Table 1. Geological features and mineralogy of some selected samples including those utilized in the isotope study. Abundant ore phases are indicated in bold and common phases in italics. Sample locations are generally not specified, being taken either from dumps or the museum collection at the Swedish Museum of Natural History (NRM). The D sample was selected for U-Pb zircon analysis.
Table 2. Ore parageneses in a temporal frame-work and suggested relationships with the identified types of fluid inclusions.
Semi-quantitative estimates of the metal contents in the mineralized samples have confirmed high copper contents but also revealed anomalously high contents of a number of other metals. For instance, Co and Bi are significantly enriched in most of the copper-rich samples, while Cd and As are particularly enriched in the only sample that shows high zinc contents. Nickel shows some enrichment in a few samples while granite-related elements like Mo, Sn and In show constant and low background levels ().
Table 3. Semi-quantitative chemical analyses of dump samples.
All samples carry substantial amounts of chalcopyrite and locally bornite. Other abundant phases include Co-rich pyrite, and nickel-bearing linneite (Co3S4) (). Digenite (Cu9S5) and covelline (CuS) are locally replacing bornite and chalcopyrite. The bornite paragenesis contains a number of more unusual phases carrying Ag, Bi, Se and Te that have not been described previously from this deposit. Such phases include native bismuth and native tellurium, bismuthinite (Bi2S3), clausthalite (PbSe), wittichenite (Cu3BiS3), hessite (Ag2Te), sylvanite (Ag,AgTe2) and krennerite (AuTe2) (; ). From a textural point of view, the main mineral of economic interest, i.e., chalcopyrite, occurs as fracture fillings in quartz and disseminated in the more massive ore. Bornite, which is the dominating copper mineral in sample G2878, often shows a basket weave texture and is locally replaced by covelline and digenite along crystallographic planes and grain boundaries. The relative crystallization order of the copper minerals shows that chalcopyrite formed as the first phase, followed by bornite, and finally covelline and digenite. Co-free pyrite is abundant in practically all specimens and forms euhedral to subhedral (≤10 mm) grains, sometimes with goethite-filled fractures (as a result of recent weathering). Co-bearing pyrite is less common and is often fine-grained and sometimes replaced by chalcopyrite. Nickel-bearing linneite is also common and forms up to 30 mm large grains showing rather well-developed crystal surfaces. Galena is abundant in massive ore zones but also occurs sparsely as up to 0.3 mm anhedral grains or in cracks between pyrite grains.
Two galena-bearing samples (representing the C and the G dump samples, respectively) were analysed for lead isotopes. Both samples are dominated by chalcopyrite, pyrite and linneite. Sample G1 also contains a range of rare minerals such as native bismuth, bismuthinite, and native gold. The C5 galena occurs on a slicken-side surface and is found in contact with monazite and chalcopyrite, whereas the G1 galena is more coarse-grained (up to 0.3 mm) and occurs along with chalcopyrite and linneite.
Zircons, further described below, were observed in several samples, with quartz and chalcopyrite being the main host minerals. The presence of gold was never noted in the mining operations, but Söderhielm (Citation1997) showed that fine-grained (5–40 μm) gold occurs most typically as anhedral inclusions in linneite and as inclusions in bornite. Tellurides, ranging from 5–50 μm, are often associated with gold and wittichenite.
U-Pb data of zircon
The zircons in dump sample D, representing a border zone between a granitic and a strongly sulphide-mineralized quartz-rich rock, constitute a very heterogeneous population in terms of colours (from dark brown to colourless), morphologies (from anhedral to euhedral shapes) and sizes (). Moreover, both clear and more turbid grains were found. The grains were mounted in four different groups (A, B, C and D) showing a gradation in size and tendency for developing crystal faces. The grains belonging to group A are mainly colourless, relatively small (around 100 µm) and anhedral without cracks. The grains of group B are anhedral with brownish colours, occasionally slightly turbid, slightly larger and more roundish than the group A grains. The grains of group C are even (around 150–200 µm) and sub-euhedral, light brown, clear to turbid and have rather frequent cracks. Finally, the grains of group D are often colourless with similar sizes as the group C grains and are predominantly euhedral with frequent cracks. Cathodo-luminescence imaging show that xenocrystic cores occasionally occur (e.g., D08b in ), and that oscillatory and other types of zoning are characteristic in many of the crystals (e.g., D03, C03a, C09a in ). Besides, it is not uncommon to see complex internal structures and signs of dissolution-recrystallization features (e.g., C01, C10 in ). Cracks and inclusions of other minerals were avoided during analyses.
Figure 4. Selected zircon grains from dump sample D interpreted as an altered quartzite rock. These grains show variable grain shapes and internal (cathodo-luminescence) structures, as further discussed in the text. The ovals shown with a sample code correspond to SIMS spots in analysed grains (see ). Obtained 207Pb/206Pb ages (in Ma) are indicated with four digits.
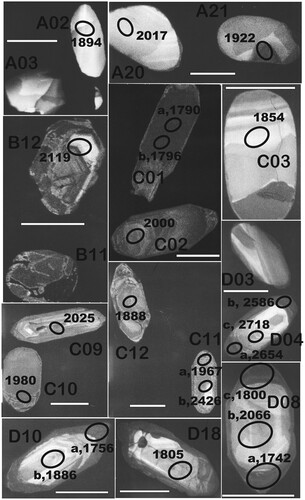
In total, 53 spots covering both inner parts and overgrowths were measured and the obtained ages show a large variation (, ). The degree of concordance varies substantially; some of the rounded grains in the group B population show extremely discordant data suggesting a Triassic lower intercept. Generally, but not always, U-rich zircons are the most discordant. The possibility of a multi-stage lead loss, the pronounced discordance, and the unfortunate circumstance that a set of data obtained during one session () were less precise, complicate the data interpretation. The least discordant zircons are likely to have the simplest post-crystallization history mimicking a more or less closed U-Pb system since crystallization. Although the 207Pb/206Pb ages correspond to minimum ages, data for spots showing a low discordance are likely to reflect real crystallization events and with such an approach, three age populations are distinguished. First, two spots in the tips of euhedral grains (D08a and D10a) yield 207Pb/206Pb ages (, inset) ranging from 1756 to 1742 Ma. Second, two other spots, in euhedral-subhedral grains (C01b, D08c and D18a), gave somewhat higher 207Pb/206Pb ages, between 1805 and 1796 Ma. Third, the dominant zircon age population, mainly derived from the A and B groups of rounded and anhedral zircons, yield consistently older 207Pb/206Pb ages mainly between ca 1.9 and 2.0 Ga. Six spots have 207Pb/206Pb ages between ca. 2.2 and 2.7 Ga.
Figure 5. U-Pb data of zircons separated from dump sample D. Data are displayed for different groups of zircons having different morphology and size, as further discussed in the text. In the main figure rounded grains are separated from sub- to euhedral grains; the inset map shows data for grains with more developed crystal faces (C and D types), excluding some data points with larger errors (cf. ).
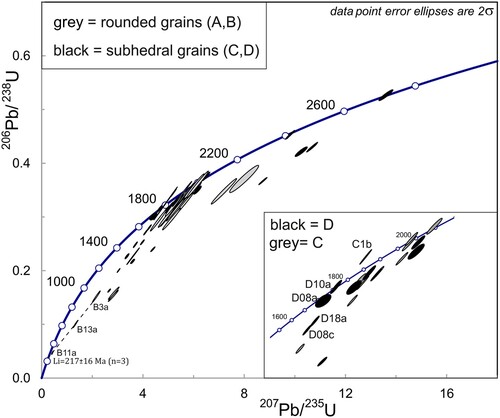
Table 4. Ion-microprobe (SIMS) U-Th-Pb data for zircon from the D dump sample at the Solstad copper mineralisation
SIMS-generated Pb-Pb data on galena
Altogether ten SIMS Pb-Pb isotope analyses were performed on coarse-grained galena from the G1 section (distributed among three individual grains), and a total of four analyses on slicken-side associated galena from the C5 section (three grains). Raw data representing a single grain (three to four intra-grain spots) were first averaged and then corrected for mass bias. The average lead isotope ratios for each grain are given in . The data for sample G1 do not vary beyond the analytical uncertainty and yield a low-radiogenic signature (206Pb/204Pb = 15.65–15.68, 207Pb/204Pb = 15.18–15.21, 208Pb/204Pb = 34.91–35.00 with averages of 206Pb/204Pb = 15.668, 207Pb/204Pb = 15.194 and 208Pb/204Pb = 34.962, respectively). Using these average values, a two-stage model age (Stacey & Kramers Citation1975) was calculated to ca. 1565 Ma with a corresponding µ (238U/204Pb) value for the second stage close to 9.0. In contrast, the ratios of the three grains in sample C5 are highly radiogenic (206Pb/204Pb = 21.13–21.48, 207Pb/204Pb = 15.74–16.03, 208Pb/204Pb = 40.51–41.18) and define a small-scale linear array suggesting a minor isotopic variability ().
Figure 6. The main 207Pb/204Pb versus 206Pb/204Pb diagram shows the isotopic composition of lead in galena in ore dump samples G1 and C5 from Solstad, as well as in galena in the Fröderyd (F) and Bergslagen (B) areas (Sundblad et al. Citation1997). A 1.8–0.4 Ga reference line is drawn to illustrate the possibility that C data represent lead being mobilized during a relatively young geological event. In the inset map the G1 composition is represented by a solid box, and letters F, Å and TÅV are used to depict three potential Bergslagen lead reservoirs (open squares): Falun, Åmmeberg and Tiveden /Åtvidaberg/Valdemarsvik, respectively (Sundblad Citation1994). The ore lead associated with TIB 1 magmatism is indicated by an open square (Sundblad Citation1997).
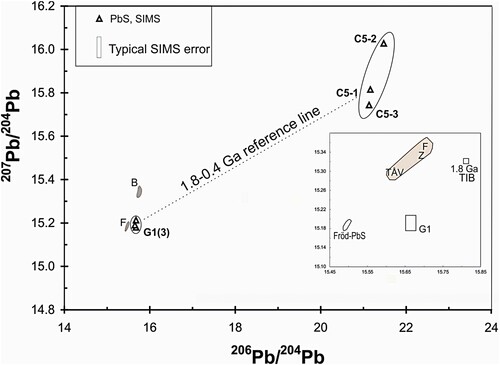
Table 5. SIMS-generated Pb isotope data for galena from dump samples G and C at the Solstad mineralization. Data represent analyses of n spots within discrete grains in polished mounts.
Fluid inclusion data
Four types of fluid inclusions were recognized in quartz hosting the sulphides (). The inclusions occur at random (type 1) or as trails (types 2, 3, 4) in the quartz and represent different stages of fluid evolution during the mineralizing processes. Type 1: This type occurs as primary inclusions in the quartz and is always associated with the chalcopyrite paragenesis. The inclusions consist of an aqueous liquid, a vapour phase (2 volume-%) and one or two solid phases. One of the latter was identified as halite by its cubic form, absence of detectable Raman signal and its behaviour on heating. The other solid phase is calcite as shown by its observed high relief and typical Raman spectrum. The size of the inclusions can be up to 20 µm. Upon heating, a partial homogenization, involving the vapour and the aqueous phase (vapour + liquid + NaCl = liquid + halite), occurred at temperatures between 135°C and 150°C (to liquid). Total homogenization (liquid + halite = liquid) was obtained at about 350°C when the NaCl crystal was observed to melt, suggesting a salinity of approximately 40 eq. mass-% NaCl.
Table 6. Microthermometric data from Solstad.
Type 2: This type has a size around 16 µm (or less) and consists of CO2 fluid inclusions that occur in bands or along trails in the quartz. They are abundant in the bornite-dominated paragenesis. Groups of fluid inclusions assemblages are either one- (liquid) or two-phase (liquid plus gas), typically indicating an entrapment at changed condition for an evolving fluid system. Melting of carbon dioxide takes place at −56.6°C and homogenization of the CO2 phases was measured in the temperature range 13.3–27.7°C to the liquid. Altogether, the measured temperatures for this inclusion type point at a pure CO2 fluid which was verified by Raman spectrometry. No other gases were detected. Using the data according to Van den Kerkhof & Thiéry (Citation2001) the melting and homogenization temperatures of the CO2 correspond to molar volumes of approximately 60 ± 5 cm3/mol. A transition of this type of CO2 fluid to type 3 inclusions was locally observed in a few healed fractures, but these decrepitated before total homogenization was reached.
Type 3: This type, which is found as trails in the grey quartz and within clear quartz along with bornite, represents a two-phase assemblage involving aqueous liquid and vapour with relatively uniform phase proportions exhibiting around 5 volume-%, large vapour bubbles. The sizes are up to 10 µm. Upon freezing and solidification, renewed heating was seen to initiate ice melting of inclusions at around −50°C, and final melting was completed in the interval −11.7°C to −25°C. These melting temperatures suggest (Davis et al. Citation1990) that the aqueous phase is composed of a CaCl2 and NaCl mixture, even though minor amounts of other salts may be present, and characterized by a salinity between 15 and 23 eq. mass-% (CaCl2 + NaCl). Homogenization temperatures fall between 145°C and 220°C (to liquid) and are regarded as minimum temperatures for this stage of ore deposition. In a few places, inclusion trails contain coexisting phases of this aqueous fluid and the CO2 fluid (), but in most cases they appear separately. The observation of co-existing aqueous salt-rich and CO2 fluid inclusions implies as a possibility that these inclusions were trapped during unmixing of an original H2O-CO2-salt fluid.
Figure 7. Photomicrograph of quartz from Solstad with one halite-bearing aqueous type 1 inclusion and a trail of aqueous carbon dioxide-bearing fluid inclusions trapped during unmixing.
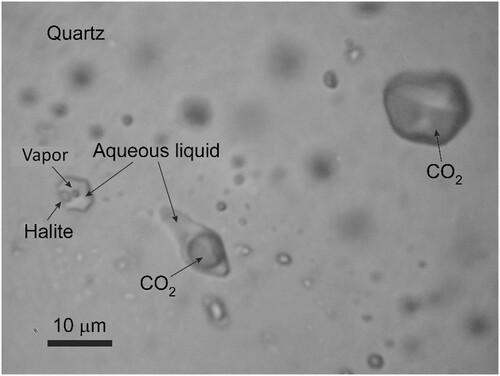
Type 4: This type represents a late aqueous fluid in healed microfractures in specimens representing both ore and wall rock. The inclusions are aqueous two-phase with a vapour bubble occupying between 2–5 volume-% in an aqueous liquid and occur in sizes up to 28 µm. After freezing and solidification, the initial ice melting was noted at about −25°C, which is just below the eutectic temperature for a saline solution composed of dominantly NaCl and KCl (Davis et al. Citation1990). Final melting occurred at −3°C to −0.3°C, which corresponds to a salinity of 0.5–5.0 eq. mass-% (NaCl + KCl). The homogenization temperatures spread between 109°C and 335°C (to liquid), with a mode between 160°C and 180°C. The latter can roughly be considered as minimum temperatures for the process associated with this type of inclusions.
Discussion
We will use field observations, paragenetical relationships, fluid inclusion and isotopic data to discuss the character of the host rock and the origin of ore fluids and metals in order to constrain a model of copper ore formation at Solstad, which stresses the importance of mafic magmatism.
U-Pb zircon data and the protolith to the altered quartz-rich host rock
The nature of the quartzitic host rock and its genetic relation to the Solstad ore is fundamental for the understanding of the geological history. Due to a general metamorphic over-print of the Västervik quartzite at amphibolite facies conditions (Gavelin Citation1984 and references therein), it is not self-evident if the high quartz content of the altered host rock reflects the presence of a quartzite of sedimentary origin or if this is due to an infiltration of hydrothermal quartz into an igneous body. The latter hypothesis could suggest that ore zones, at least partly, are spatially related to quartz veins. This is not seen at Solstad; instead the more massive mineralization is closely associated with small fissures, carrying chlorite, biotite and hornblende. However, a certain addition of hydrothermal quartz to the ore-bearing zones cannot be ruled out, but it is unlikely that such a process can also lead to a tens of metres wide zone of more or less barren quartz-rich rock surrounding the mineralization. Hence, the field relationships suggest that the quartz-rich rock is a lens of Västervik quartzite that got incorporated by a granite magma.
The U-Pb investigation of the zircons in the dump sample, showing a variety of colours, morphologies, sizes and ages, strengthens the view that the quartz-rich host rock constitutes a metamorphosed clastic rock. Although the discordant zircon age patterns are problematic to interpret and imply that the 207Pb/206Pb ages are minimum ages, it appears possible to distinguish several events of zircon crystallizing; a younger (207Pb/206Pb) population, close to 1.8 Ga, is indicated for euhedral-subhedral grains, whereas anhedral and rounded grains yielded two pre-Svecofennian age populations within the 2.1–1.9 Ga interval and exceeding ca. 2.4 Ga, respectively. The older age populations overlap the SIMS zircon data that Claesson et al. (Citation1993) and Sultan et al. (Citation2005) have published for the Västervik quartzite (B). They distinguished four age intervals: 2.12–1.84 Ga, 2.72–2.69 Ga, 3.03–2.95 Ga and ca. 3.64 Ga, respectively, with the majority of ages clustering around 2.0 Ga. This comparison leaves little doubt that the investigated Solstad sample represents a quartzite containing detrital zircons that have suffered partial hydrothermal over-printing in connection with an intruding 1.8 Ga granitic magma. The alternative hypothesis that the host rock is an altered granite finds no support from the U-Pb ages given that zircons in 1.80 Ga TIB 1 granites in southern Sweden show no signs of any significant inheritance (Mansfeld Citation1991; Jarl & Johansson Citation1988).
Altogether, field and U-Pb isotope data of zircon are consistent with the following history: (i) an input of detrital material (age modes at 2.1–1.9 and 2.7–2.4 Ga, respectively) to a sedimentary basin led to the emplacement of a sandstone that subsequently got transformed into a quartzite, and (ii) one or, possibly, two episodes in the ca. 1.80–1.77 Ga interval led to new zircon growth in the quartzite as a result of its incorporation as a xenolith in the TIB 1a magma and associated tectonic and hydrothermal processes.
Constraints from the fluid inclusion data
The fluid inclusion study shows that four different fluids (type 1 to type 4) were involved in the geological evolution of the Solstad ore zone () and that each fluid type is linked to a specific ore paragenetic stage: (1) the hypersaline halite-bearing aqueous fluid is linked to a chalcopyrite-dominated stage, (2) the CO2-rich fluid and (3) the moderate- to high-salinity aqueous fluids are linked to a bornite-dominated stage involving gold, tellurides, selenides and bismuthinite, and (4) the low-salinity aqueous fluid is associated with oxidation to covelline and digenite of previously formed phases. Different metals preferentially concentrate in the salt-rich aqueous liquid or in the CO2 phase depending on how they were transported in the fluid. The main copper ore (dominated by chalcopyrite) was deposited from the very salt-rich fluid (ca. 40 eq. wt-% of NaCl) at temperatures exceeding 350 °C (type 1 fluid). This was followed by the CO2 fluid with fluid inclusions (type 2) implying a decline in pressure. The role of the CO2-rich phase in sulphide-forming processes is still not well understood, but a possible relationship to gold has been proposed for many gold-rich deposits throughout the world (Phillips & Evans Citation2004). The CO2 phase at Solstad (together with the aqueous type 3 fluid inclusions) is present in the bornite-dominated paragenesis where also native gold as well as tellurides, selenides and bismuthinite are present. The local presence of associated type 2 and type 3 inclusions may indicate unmixing of an aqueous CO2-bearing fluid. The change in pressure, indicated by type 2 inclusions to a more surficial level, was accompanied by mixing of a highly saline aqueous fluid with a more Ca-rich and colder aqueous fluid as further suggested by the aqueous type 3 inclusions. This initiated deposition of the bornite-dominated phase from a NaCl-CaCl solution with a salinity of 15–23 eq. wt-% of NaCl at minimum temperatures between 145 and 220 °C.
There are two ways of interpreting the observed fluid inclusion styles: first, the temporarily first three fluid inclusion types (types 1–3) could reflect a continuous, and relatively short-lived, fluid history, followed by a low-salinity fluid (0.5–5 eq. wt-% of NaCl) that was genetically unrelated to the previous generations of ore-related inclusions. The latter affected fractures in both wall rocks and the ore which led to an oxidation of previously formed parageneses and the development of, e.g., covelline and digenite. The temperatures at this stage were probably not much higher than 160–180 °C. The second, and preferred model, which best fits the geological conditions as further discussed below, considers type 1 inclusions to characterize an early hypersaline fluid. Following this, an aqueous CO2 fluid underwent phase separation, most likely following a process of pressure decrease, thereby producing two specific fluids (represented by type 2 and 3 inclusions, respectively). The low-salinity fluid (type 4 inclusions) characterizes yet another fluid pulse that concluded the Proterozoic fluid history. It is worth noting that petrographic investigations suggest that the formation of magnetite preceded the deposition of chalcopyrite (stage 1), but no fluid inclusions remain from this stage.
Source of ore lead
The lead isotope data of the Solstad galenas define two populations, one homogeneous population with low-radiogenic compositions (on average 206Pb/204Pb = 15.65–15.68, 207Pb/204Pb = 15.18–15.21, 208Pb/204Pb = 34.91–35.00 and a Stacey-Kramers (Citation1975) µ value of ca. 9.0) and one heterogeneous population with 206Pb/204Pb ratios >21 (). The most plausible explanation for this bimodal composition is that galena crystallized at two different stages. It is suggested that the homogeneous low-radiogenic population represents lead that was available in the ore-forming fluid, while the heterogeneous, radiogenic population (206Pb/204Pb >20), represents lead that was added to the system during a much later event. In order to further constrain the source(s) of the two lead populations at Solstad, it is relevant to compare these data with lead isotope data for galena in other settings in southern Sweden.
In theory, it can be speculated on the possibility that the metals in the Solstad ore were derived by magmatic-hydrothermal fluids from TIB, but, in such a case, the initial lead composition of the TIB magma has to match that of the ore lead composition of Solstad. The first estimate of the initial lead composition of the TIB units was made by Jarl & Johansson (Citation1988) who determined the isotopic composition of lead in potassium feldspar in the Växjö granite, 120 km SW of Solstad to 206Pb/204Pb = 15.9, 207Pb/204Pb = 15.4, 208Pb/204Pb = 35.4. Ore lead data also exist for three granite-hosted, magmatic-hydrothermal Zn-Pb-Cu mineralizations at Ramnebo (Ahl Citation1989), 9 km SW of Solstad. The lead composition of the Ramnebo galena is on average 206Pb/204Pb = 15.78, 207Pb/204Pb = 15.32, 208Pb/204Pb = 35.34 and was considered to be a better estimate of the initial lead in the TIB units than estimates made on feldspar lead (Sundblad Citation1991). Furthermore, a hydrothermal Zn-Pb mineralization in 1.80 Ga TIB rhyolites at Ålatorp (ca. 97 km SW of Solstad) has a comparable ore lead composition to Ramnebo (Sundblad Citation1997). The corresponding µ values for Ramnebo are 9.72 and for Ålatorp 9.58, which are clearly different from the very primitive lead in the low-radiogenic population at Solstad (µ = 9.0). No support is thus provided for lead derivation from TIB 1 into the Solstad ore system.
The Solstad ore is hosted by Västervik quartzites implying that this rock unit must also be considered as a source of lead. However, no lead isotope data are available for this rock. Yet, as quartzites in general do not carry substantial amounts of any metal, the Västervik quartzite is not a feasible source for the metal supply in the Solstad ore.
Another hypothesis would be that the low-radiogenic lead at Solstad was derived from a 1.9 Ga Svecofennian source, but available data for the ore lead composition in the adjacent Åtvidaberg-Valdemarsvik region and the more remote Bergslagen region show significantly higher 207Pb/204Pb ratios (Sundblad Citation1994) compared to the low-radiogenic Solstad population (). The only ore lead composition which has a relatively close resemblance to the low-radiogenic population in Solstad is reported from the 1.85 Ga Fröderyd arc (Sundblad et al. Citation1997; Salin et al. Citation2021), which is host for a number of small Cu-Zn-Pb deposits, with a homogenous and low-radiogenic ore lead signature (on average 206Pb/204Pb = 15.486, 207Pb/204Pb = 15.184, 208Pb/204Pb = 35.090; µ = 9.2). This primitive ore lead composition was suggested to representative the initial lead in the Fröderyd arc rocks and is matched with very high εNd signatures (ca. + 5.0) in the host volcanic rocks (Mansfeld Citation1995). Although there is no perfect isotopic match between the ore lead composition at Fröderyd and Solstad, it appears that a 1.85 Ga mafic rock is the best candidate for being the source of the low-radiogenic lead population at Solstad.
Finally, the data for the heterogeneous, high-radiogenic population at Solstad are comparable with the isotopic composition of lead in fluorite-calcite-galena veins at Tindered, 30 km north of Västervik, recorded by Alm & Sundblad (Citation2002). These veins are hosted by TIB 0 granitoids but interfere with a Cambrian sandstone dyke and are considered to have formed by tectonic-hydrothermal remobilizing of lead from the TIB 0 granitoid during the Lower Devonian (Alm et al. Citation2005). A similar reworking of the Precambrian crust is envisaged also for the Solstad area.
Ore genetic aspects and an outlook towards other Cu±Co, Se ore deposits
The presence of several types of fluid inclusions in quartz argue for a multi-stage fluid evolution at Solstad but there is no radiometric evidence that allow for any temporal constraints on these stages. Nevertheless, it is suggested that most metals in the Solstad deposit, including Cu and Co, were introduced by hypersaline fluids (generated by heat from simultaneous mafic magmatism) into quartz-rich fluvial sediments at an early stage (ca. 1.88–1.86 Ga). As a result, predominantly chalcopyrite and Co-rich pyrite crystallized. Some metals, such as Se, Te, Bi and Au are associated with bornite and appear to over-print the chalcopyrite-dominated paragenesis. Minor dislocations of the ore-bearing zones took place in a significantly later and more surficial phase, associated with brittle tectonic conditions in a much cooler environment, when the primary ore minerals partly were oxidized to digenite and covelline.
Magnetite-chalcopyrite (±bornite and ± Co-minerals) mineralization has been recorded at many sites (e.g., Gladhammar, Skälö, Skavdö and Rumma) in the Västervik quartzites (Tegengren et al. Citation1924; Bruun et al. Citation1991), including also some minor occurrences close to Solstad (c). These metal concentrations are often close to, or locally even within, interlayered metabasalts, and it is noteworthy that TIB granites are rarely spatially connected to these copper deposits suggesting that granitic magmatism played a marginal, if any, role in the main metal deposition. This leads us to suggest a genetic concept where the ore-forming process is related to magmatic-hydrothermal activity governed by mafic igneous processes that occurred contemporaneously with the deltaic sedimentation in the Västervik basin. A new Cu-Co-Se metallogenetic province is thus on its way to be defined along the southern margin of the Svecofennian Domain.
Cu-Co-Au mineralizations in clastic metasedimentary rocks are also known at, e.g., Kuusamo in northern Finland in the Early Proterozoic pre-Svecofennian greenstone belts (Pankka & Vanhanen Citation1992) where the importance of shear zones and orogenic ore-forming processes evidently are more pronounced than in the Solstad area. In an even more global perspective, there are also a number of sulphide ores classified by Slack et al. (Citation2010) as “Cu-Co deposits hosted by metasedimentary rocks”, where the Solstad Cu ± Co deposit apparently should have a role to play.
Conclusions
The Solstad sulphide deposit has for a long time been known for high copper grades and the presence of chalcopyrite, but this investigation shows that the ore also is enriched in Pb, Zn, Se, Co, Ni and Bi and carries a number of previously unrecognized opaque phases (e.g., digenite, bornite, sphalerite, galena, several Co phases, selenides and tellurides).
An U-Pb investigation of zircons from a sulphide-bearing sample demonstrates that the host rock is a 1.88–1.86 Ga quartzite.
The lead isotopic composition of galena in the Solstad ore reveals two populations. The low-radiogenic population, with a 238U/204Pb of ca. 9.0, suggests that a primitive source rock was responsible for the metal supply, most probably the metabasalts that occur as layers in the Västervik quartzite. The high-radiogenic population indicates lead addition due to late reactivation of the Precambrian crust.
Four different types of fluid inclusions are recognized and can tentatively be linked with discrete ore paragenetic stages. A hypersaline, halite-bearing aqueous, fluid was linked to an early stage (at ca. 1.88–1.86 Ga) dominated by chalcopyrite deposition. Subsequently, a CO2-rich fluid and a moderate- to high-salinity aqueous fluid developed as a result of a phase separation process, and bornite, along with a paragenesis typified by native gold, tellurides, selenides and bismuthinite, crystallized from these fluids. Finally, a low-salinity, aqueous and oxidizing fluid active at ca. 1.76 Ga stabilized covelline and digenite at the expense of previously formed copper minerals.
Based on field, petrographic, geochemical and isotopic evidence, it is suggested that the Solstad deposit, together with other Cu ± Co ± Se rich sulphide occurrences in the Västervik quartzites, form a distinct ore type in the southernmost corner of the Svecofennian Domain.
Acknowledgements
This is NORDSIM contribution 712. We are indebted to N. Saintilan and an anonymous reviewer, as well as the journal subject editor, for constructive reviews of an earlier version of the manuscript.
Disclosure statement
No potential conflict of interest was reported by the author(s).
References
- Åhäll, K.-I., Connelly, J.N. & Brewer, T.S., 2002: Transitioning from Svecofennian to transscandinavian igneous belt (TIB) magmatism in SE Sweden: implications from the 1.82 Ga Eksjö tonalite. GFF 124, 217–224.
- Ahl, M., 1989: Zn-Pb-Cu-mineraliseringarna vid Ramnebo, sydöstra Sverige, och deras genetiska relation till Smålandsgraniten. Unpublished MSc thesis. Stockholm University, 26 pp.
- Allen, R.L., Lundström, I., Ripa, M., Simeonov, A. & Christofferson, H., 1996: Facies analysis of a 1.9 Ga, continental margin, back-arc, felsic caldera province with diverse Zn-Pb-Ag-(Cu-Au) sulfide and Fe oxide deposits, Bergslagen region, Sweden. Economic Geology 91, 979–1008.
- Alm, E. & Sundblad, K., 2002: Fluorite-calcite-galena-bearing fractures in the counties of Kalmar and Blekinge, Sweden. SKB report R-02-42, 116 pp.
- Alm, E., Huhma, H. & Sundblad, K., 2005: Preliminary Palaeozoic Sm-Nd ages of fluorite-calcite-galena veins in the southeastern part of the Fennoscandian Shield. SKB report R-04-27, 32 pp.
- Åhäll, K.-I., 2001: Åldersbestämning av svårdaterade bergarter i sydöstra Sverige. SKB report R-01-60, 28 pp.
- Bergström, U., Juhojuntti, N., Kero, L., Lundqvist, L., Stephens, M.B., Sukotjo, S., Wik, N.G. & Wikman, H., 2002: Projekt Småland, regionalt berg. In H. Delin (ed.): Regional berggrundsgeologisk undersökning. Sammanfattning av pågående undersökningar 2001, 65–83. Sveriges geologiska undersökning, Rapporter och meddelanden 110.
- Beunk, F.F. & Page, L.M., 2001: Structural evolution of the accretional continental margin of the Paleoproterozoic Svecofennian orogeny in southern Sweden. Tectonophysics 339, 67–92.
- Beunk, F.F., Page, L.M., Wijbrans, J.R. & Barling, J., 1996: Deformational, metamorphic and geochronological constraints from the Loftahammar-Linköping Deformation Zone (LLDZ) in SE Sweden: implications for the development of the Svecofennian orogeny. GFF Jubilee Issue A9.
- Björklund, L. & Weihed, P., 1997: Geochemistry and tectonic setting of the Orvar Hill mafic volcanic rocks of the Tiveden area, south-central Sweden. GFF 119, 127–134.
- Bruun, A., Kornfält, K.-A., Sundberg, A., Wik, N.-G., Wikman, H. & Wikström, A., 1991: Malmer, industriella mineral och bergarter i Kalmar län. Sveriges geologiska undersökning. Rapporter och meddelanden 65, 176, pp.
- Claesson, S., Huhma, H., Kinny, P.D. & Williams, I.S., 1993: Svecofennian detrital zircon ages – implications for the Precambrian evolution of the Baltic Shield. Precambrian Research 64, 109–130.
- Davis, D.W., Irwenstein, T.K. & Spencer, R.J., 1990: Melting behavior of fluid inclusions in laboratory-grown halite crystals in the systems sodium chloride-water, sodium chloride-potassium chloride-water, sodium chloride-magnesium chloride-water, and sodium chloride, calcium chloride-water. Geochimica et Cosmochimica Acta 54, 591–601.
- Eklund, A.J., 1925: Rapport över en undersökning av Solstads kopparmalmsfält (in Swedish) Unpublished report, 5 pp.
- Gavelin, S., 1984: The Västervik area in south-eastern Sweden. Studies in Proterozoic sedimentation, high-grade metamorphism and granitization. Sveriges geologiska undersökning Ba 32, 172 pp.
- Högdahl, K., Andersson, U.-B. & Eklund, O., 2004: The Transscandinavian Igneous Belt (TIB) in Sweden: a review of its character and evolution. Geological Survey Finland, 125 pp. Special paper 37.
- Jansson, N.F., Zetterqvist, A., Allen, R.L., Billström, K. & Malmström, L., 2017: Genesis of the Zinkgruvan stratiform Zn-Pb-Ag deposit and associated dolomite-hosted Cu ore, Bergslagen, Sweden. Ore Geology Reviews 82, 285–308.
- Jarl, L.-G. & Johansson, Å, 1988: U–Pb zircon ages of granitoids from the Småland-Värmland granite-porphyry belt, southern and central Sweden. Geologiska Föreningens i Stockholm Förhandlingar 110, 21–28.
- Jonsson, E., Troll, V.R., Högdahl, K., Harris, C., Weis, F., Nilsson, K.P. & Skelton, A., 2013: Magmatic origin of giant “Kiruna-type” apatite-iron oxide ores in Central Sweden. Nature, Scientific Reports 3, 8 pp.
- Kampmann, T.C., Jansson, N.F., Stephens, M.B., Olin, P.H., Gilbert, S. & Wanhainen, C., 2018: Syn-tectonic sulphide remobilization and trace element redistribution at the Falun pyritic Zn-Pb-Cu-(Au-Ag) sulphide deposit, Bergslagen, Sweden. Ore Geology Reviews 96, 48–71.
- Kleinhanns, I.C., Fischer-Gödde, M. & Hansen, B.T., 2012: Sr–Nd isotope and geochemical characterisation of the Paleoproterozoic Västervik formation (Baltic Shield, SE-Sweden): a southerly exposure of Svecofennian metasiliciclastic sediments. International Journal of Earth Sciences (Geologische Rundschau) 101, 39–55.
- Kresten, P., 1972: Der basische Magmatismus und seine Stellung in der geologischen Entwicklung des Västervik-Gebietes, Südostschweden. Geologiska Föreningens i Stockholm Förhandlingar 94, 91–109.
- Kumpulainen, R.A., Mansfeld, J. & Sundblad, K., 1996: Stratigraphy, Age, and Sm-Nd isotope systematics of the country rocks to Zn-Pb sulfide deposits, Åmmeberg District, Sweden. Economic Geology 91, 1009–1021.
- Larson, SÅ & Berglund, J., 1992: A chronological subdivision of the transscandinavian igneous belt - three magmatic episodes. Geologiska Föreningens i Stockholm Förhandlingar 114, 459–461.
- Mansfeld, J., 1991: U-Pb age determinations of Småland-Värmland granitoids in Småland, southeastern Sweden. Geologiska Föreningens i Stockholm Förhandlingar 113, 113–119.
- Mansfeld, J., 1995: Age and Nd-isotope geochemistry of the Fröderyd Group, southeastern Sweden. In J. Mansfeld (ed.): Crustal Evolution in the Southeastern Part of the Fennoscandian Shield, 289 pp. Meddelanden från Stockholms Universitet, Stockholm.
- Mansfeld, J., 1996: Geological, geochemical and geochronological evidence for a new Palaeoproterozoic terrane in southeastern Sweden. Precambrian Research 77, 91–103.
- Nolte, N., Kleinhanns, I.C., Baero, W. & Hansen, B.T., 2011: Petrography and whole-rock geochemical characteristics of Västervik granitoids to syenitoids, southeast Sweden: constraints on petrogene-sis and tectonic setting at the southern margin of the Svecofennian domain. GFF 133, 173–196.
- Nordenström, G., 1876: Ytterligare meddelanden om Solstads koppargrufva i Småland. Geologiska Föreningens i Stockholm Förhandlingar 2, 116–120.
- Pankka, H.S. & Vanhanen, E.J., 1992: Early Proterozoic Au-Co-U mineralization in the Kuusamo district, northeastern Finland. Precambrian Research 58, 387–400.
- Phillips, G.N. & Evans, K.A., 2004: Role of CO2 in the formation of gold deposits. Nature 429, 860–863.
- Russell, R.V., 1967: Paleocurrent analysis in the deltaic Precambrian meta-sedimentary rocks from Västervik, Sweden. Geologiska Föreningens i Stockholm Förhandlingar 89, 105–115.
- Salin, E., Sundblad, K. & Lahaye, Y., 2021: A 1.85 Ga volcanic arc offshore the proto-continent Fennoscandia in southern Sweden. Precambrian Research 356, https://doi.org/10.1016/j.precamres.2021.106134.
- Salin, E., Sundblad, K., Woodard, J. & ÓBrien, H., 2019: The extension of the transscandinavian igneous belt into the Baltic sea region. Precambrian Research 328, 287–308.
- Slack, J.F., 2010: Descriptive and geoenvironmental model for cobalt-copper-gold deposits in metasedimentary rocks (USGS report; J.F. Slack, editor) Scientific Investigations Report 2010–5070–G Chapter G of Mineral Deposits Models for Resource Assessment. 234 pp.
- Sopher, D., Erlström, M., Bell, N. & Juhlin, C., 2016: The structure and stratigraphy of the sedimentary succession in the Swedish sector of the Baltic Basin: New insights from vintage 2 D marine seismic data. Tectonophysics 676, 90–111.
- Söderhielm, J., 1997: Cu-Co-Au malmen i Solstad i sydöstra Sverige. Malmparageneser, geologi och sammanställning av gruvans historik. Unpublished M.Sc. thesis, in Swedish. Stockholm university, 57 pp.
- Stacey, J.S. & Kramers, J.D., 1975: Approximation of terrestrial lead isotope evolution by a two-stage model. Earth and Planetary Science Letters 26, 207–221.
- Stephens, M.B. & Andersson, J., 2015: Migmatization related to mafic underplating and intra- or back-arc spreading above a subduction boundary in a 2.0-1.8 Ga accretionary orogen, Sweden. Precambrian Research 264, 235–257.
- Stephens, M.B., Ripa, M., Lundström, I., Persson, L., Bergman, T., Ahl, M., Wahlgren, C.-H., Persson, P.-O. & Wickström, L., 2009: Synthesis of the bedrock geology in the Bergslagen region, Fennoscandian Shield, south-central Sweden. Sveriges geologiska undersökning Ba 58, 259 pp.
- Sultan, L., Claesson, S. & Plink-Björklund, P., 2005: Proterozoic and Archaean ages of detrital zircon from the Palaeoproterozoic Västervik Basin, SE Sweden: implications for provenance and timing of deposition. GFF 127, 17–24.
- Sultan, L. & Plink-Björklund, P., 2006: Depositional environments at a Palaeoproterozoic continental margin, Västervik Basin, SE Sweden. Precambrian Research 145, 243–271.
- Sundblad, K., 1991: Lead isotopic evidence for the origin of 1.8-1.4 Ga ores and granitoids in the southeastern part of the Fennoscandian Shield. Precambrian Research 51, 265–281.
- Sundblad, K., 1994: A genetic reinterpretation of the Falun and Åmmeberg ore types, Bergslagen, Sweden. Mineralium Deposita 29, 170–179.
- Sundblad, K., 1997: Syngenetic Pb-Zn-Cu sulfide mineralization in 1.8 Ga subaerial volcanic rocks, southeastern Sweden. GFF 119, 103–108.
- Sundblad, K., Mansfeld, J. & Särkinen, M., 1997: Palaeoproterozoic rifting and formation of sulphide deposits along the southwestern margin of the Svecofennian Domain, southern Sweden. Precambrian Research 82, 1–12.
- Sundblad, K., Salin, E., Claesson, S., Gyllencreutz, R. & Billström, K., 2021: The Precambrian of Gotland, a key for understanding the Proterozoic evolution in southern Fennoscandia. Precambrian Research 363. doi:10.1016/j.precamres.2021.106321
- Tegengren, F.R., 1924: Sveriges ädlare malmer och bergverk. Sveriges geologiska undersökning Ca 17, 406.
- Van den Kerkhof, A. & Thiéry, R., 2001: Carbonic inclusions. Lithos 55, 49–68.
- Vollbrecht, A. & Leiss, B., 2008: Complex fabric development in Paleoproterozoic meta-quartzites of the Västervik Basin, SE Sweden. GFF 130, 41–45.
- Whitehouse, M.J. & Kamber, B.S., 2005: Assigning dates to thin gneissic veins in high-grade metamorphic terranes: a cautionary tale from Akilia, southwest Greenland. Journal of Petrology 46, 291–318.
- Whitehouse, M.J., Kamber, B.S. & Moorbath, S., 1999: Age significance of U–Th–Pb zircon data from early Archaean rocks of west Greenland: a reassessment based on combined ion-microprobe and imaging studies. Chemical Geology (Isotope Geoscience Section) 160, 201–224.
- Wiedebeck, M., Allé, P., Corfu, F., Griffin, W., Meier, M., Oberu, F., von Quadt, A., Roddick, J.C. & Spiegl, W., 1995: Three natural zircon standards for U–Th–Pb, Lu–Hf, trace-element and REE analyses. Geostandards Newsletter 19, 1–23.
- Wik, N.-G., Bergström, U., Bruun, Å, Claeson, D., Jelinek, C., Juhojuntti, N., Kero, L., Lundqvist, L., Stephens, M.B., Sukotjo, S. & Wikman, H., 2005: Beskrivning till regional berggrundskarta över Kalmar län (in Swedish). Sveriges geologiska undersökning Ba 66, 50.
- Wikström, A. & Andersson, U.B., 2004: Geological features of the Småland-Värmland Belt along the Svecofennian margin, part I: from the Loftahammar to the Tiveden-Askersund areas. In K. Högdahl, U.B. Andersson & O. Eklund (eds.): The transscandinavian igneous belt (TIB) in Sweden: a review of its character and evolution, 22–39. Geological Survey of Finland Special Paper 37. Espoo 2004.
- Wikström, A. & Persson, P-O, 2002: A c. 1845 Ma (”Askersund”) age of the Hälla augen gneiss in south-eastern Östergötland, south-east Sweden. In S. Bergman (ed.): Radiometric dating results 5, 58–61. Sveriges Geologiska Undersökning C 834.
- Zetterström, L., 2001: U and Pb isotope analysis of uraninite and galena by ion microprobe, SKB Technical report TR-01-35, 49 pp.