Abstract
The study aimed to investigate the anti-tumor effects and underlying mechanisms of Enzalutamide (ENZ) and Arsenic trioxide (ATO) co-treatment on castration-resistant prostate cancer (CRPC). The effects on C4-2B cells were initially evaluated by colony formation assay, FACS analysis, and DNA fragmentation detection. Bioinformatics methods including mRNA-sequencing and gene enrichment analysis were used to screen the underlying target genes and pathways related to their actions. Western blot was employed to assess the expression levels of protein-related angiogenesis, apoptosis, DNA repair, and the screened genes. Finally, the effects were further verified in subcutaneous tumor models and tissue sections from the xenografts. It was found that not only could ENZ combination with ATO significantly inhibit cell proliferation and angiogenesis, but also induce cell arrest and apoptosis in C4-2B cells. In addition, interruption of the DNA damage repair-related pathways also occurred as a result of their combined effects. Western blot analysis further suggested that proteins involved in these pathways, especially P-ATR and P-CHEK1 were significantly reduced. In addition, their combination also inhibited the tumor growth of xenografts. Altogether, ENZ combination with ATO synergistically improved the therapeutic effects and suppressed CRPC progression through regulation of the ATR-CHEK1-CDC25C pathway.
Introduction
Prostate cancer (PCa) is a common malignancy in men worldwide, which is characterized by a high recurrence rate and high mortality [Citation1]. Currently, androgen deprivation therapy (ADT) is considered the gold standard treatment for advanced PCa [Citation2]. Nevertheless, approximately 80-90% PCa patients progress to castration-resistant prostate cancer (CRPC) that occurs within 2-3 years of initiation of ADT [Citation3]. Unfortunately, there are still no effective drugs for the treatment of CRPC up to now because of acquired resistance. Hence, it is necessary to understand the underlying molecular mechanism of CRPC progression, so as to provide important insights into more effective therapeutic approaches.
Enzalutamide (ENZ), an effective androgen receptor (AR) signaling inhibitor, has demonstrated significant efficacy in the treatment of CRPC and prolongs the overall survival (OS) [Citation4, Citation5]. Despite these encouraging results, development of resistance to ENZ amplifies the difficulty of treatment [Citation6, Citation7]. Therefore, it is urgently needed for the development of novel anticancer agents combined with ENZ to improve the treatment efficacy for CRPC. Arsenic trioxide (ATO) is a part of traditional Chinese medicine, which induces significant clinical remission in patients with acute promyelocytic leukemia (APL) [Citation8]. Recently, accumulated evidence has demonstrated that ATO can be used as a novel effective anticancer agent for the treatment of various types of cancers [Citation9, Citation10]. For instance, it has been shown that ATO regulates gastric cancer cell apoptosis by mediating cAMP [Citation9], and inhibits bladder cancer cell and tumor growth via downregulating specificity protein (Sp) transcription factors [Citation10]. More notably, ATO has also been indicated to play a suppressive role in the progression of PCa by inducing apoptosis and inhibiting tumor growth [Citation11, Citation12].
A recent study has found that ATO exhibits significant anti-angiogenic ability of PCa cells through inhibitions of the TGF-β/SMAD2 signaling, the vascular endothelial growth factor secretion and angiogenesis [Citation13]. Moreover, it has also been reported that ATO can inhibit AR transcriptional activity by disrupting AR recruitment to AR target gene promoters in the presence of very low androgens [Citation14]. Additionally, the effect was additive when ATO was used with Bicalutamide (an AR inhibitor) in vitro. Nevertheless, the effects of ENZ and ATO in combination for the treatment of CRPC and the molecular mechanism of their synergy remain unclear.
In the present study, we mainly investigated the anti-tumor effects of ENZ combination with ATO on the CRPC progression by regulation of cell proliferation, apoptosis and angiogenesis. Altogether, their combination may be essential for achieving enhanced efficacy and reduced side effects in advanced prostate cancer.
Materials and methods
Cell lines
The human PCa cell line C4-2B was obtained from the American Type Culture Collection (Manassas, VA, USA). All cells were cultured in RPMI-1640 medium supplemented with 10% complete fetal bovine serum (FBS) and maintained at 37 °C in a humidified incubator with 5% CO2.
In vitro ENZ and ATO treatment
ENZ (MDV3100) was purchased from SellekChem (Houston, TX, USA) and diluted in dimethyl sulfoxide (DMSO) at 1 μM for in vitro experiments. ATO was purchased from Sigma (St. Louis, MO, USA) and diluted in DMSO at 5 μM for in vitro experiments.
C4-2B cells were pretreated with 5% DMSO vehicle control for 24 h. On the following days, added additional drug combinations into cells and then incubated for 48 h as follows: 1 μM ENZ, 5 μM ATO, or their combination. The cells were collected and prepared for fluorescence-activated cell sorting (FACS), DNA fragmentation detection, and western blot (WB) analysis.
Colony formation assay
C4-2B cells were seeded into 6-well plates at the density of 1 × 103 cells/well and pretreated with 5% DMSO vehicle control for 24 h. Subsequently, the cells were treated with 1 μM ENZ [Citation15], 5 μM ATO [Citation16], and their combination [Citation12]. Following 48 h of incubation, the media were replaced with fresh drug-free media, and the plate was incubated at 37 °C until the colonies were visible. Following washing with phosphate buffer solution (PBS), the colonies were fixed with cold methanol for 30 min, stained with 0.5% crystal violet in 25% methanol for 30 min, counted, and imaged with a camera (Nikon Instruments Inc., Shanghai, CHN). Finally, the colony formation rate was calculated as the number of colonies formed/number of inoculated cells. Three independent measurements were performed.
Fluorescence-activated cell sorting analysis
The collected cells were stained with propidium iodide (PI) and subsequently analyzed on a FACS Canto II (BD Biosciences, NJ, USA) flow cytometer. The cell cycle distribution and quantitative data were obtained using FlowJoTM software (BD Biosciences, NJ, USA). Three independent measurements were performed in triplicate.
DNA fragmentation detection
A Cell Death Detection ELISA Kit (Roche, Mannheim, GER) was used for DNA fragmentation detection. The cytoplasmic histone-associated DNA fragments (mono- and oligonucleosomes) of cells were extracted and assayed according to the manufacturer’s instructions. The absorbance was measured at 405 nm using a microplate reader (BioTek, VT, USA). Three independent experiments were performed in triplicate.
Screening of the underlying target genes
To assess the potential molecular mechanism of the effects caused by the combined treatment of ENZ and ATO, the gene expression microarray dataset GSE32269, which included 22 primary PCa specimens (hormone-dependent) and 29 metastatic PCa specimens (CRPC), was downloaded from the GEO database (https://www.ncbi.nlm.nih.gov/geo/). The differentially expressed genes (DEGs) of DNA damage repair pathways and the targets of ATO derived from Drugbank (https://go.drugbank.com/drugs/DB01169) were assessed in the GSE32269. Subsequently, these genes were further assessed in the mRNA-sequencing (RNA-seq) library established by our previous study, which included DEGs between C4-2B-ENZ (ENZ resistance) and C4-2B cells. In addition, enriched pathways of these genes were examined by gene set enrichment analysis (GSEA) [Citation17]. The heatmap and the volcano plot were generated by TB tools software (CJ-Chen, South China Agricultural University, CHN) and GraphPad Prism 8.4.3 (GraphPad Software Inc., San Diego, CA), respectively, and then data of the DEGs related to DNA damage repair and the targets of ATO were visualized. The box plot was performed by the R package ‘ggplot2’, whereas the R package ‘wordcloud2’ was used to visualize the frequency of the aforementioned genes in the enriched pathways.
Western blot analysis
The primary antibodies were detected by horseradish peroxidase-conjugated antibody (1:2,000). Densitometry analysis for WB was performed using Image J software (NIH, MD, USA). The expression levels of phosphorylated proteins were normalized to those of total proteins, whereas the expression levels of other related proteins were normalized to those of GAPDH. Values of each drug-treated group were further normalized to those of the control, and the control was set as one. Assays were performed at least three times and data were shown by the mean of these replicates. Antibodies against ATR, P-ATR (S428), CHEK1, P-CHEK1 (S317), CDC25C, and P-CDC25C (S216) were purchased from Cell Signaling Technology (Danvers, MA, USA). Antibodies against cleaved PARP (C-PARP), BCL-2, and γH2AX were purchased from Santa Cruz Biotechnology (Dallas, TX, USA). Other antibodies against EGFR, P-EGFR (T1068), AKT, P-AKT (T308), VEGFR2, and GAPDH were obtained from Abcam (Cambridge, MA, USA).
Subcutaneous C4-2B xenografts
Pre-castrated severe combined immunodeficiency (SCID) mice were purchased from Charles River (Wilmington, MA, USA). C4-2B cells (1.5 × 106 cells/mouse) were injected subcutaneously into the left flanks of SCID mice to induce subcutaneous tumors. The tumors were grown for 14 days before treatment and surviving tumor-bearing mice were randomly and equally divided into four experimental groups with 15 mice in each group. After that, treatments were implemented as described above for 27 days. Tumor growth was monitored by calipers, and tumor volumes were calculated using the following formula: length × width2/2. Finally, mice were euthanized and tumors were collected.
Immunohistochemical analysis
Immunohistochemistry (IHC) staining was performed on formalin-fixed and paraffin-embedded tissue sections from the C4-2B xenografts to analyze the following aspects of tumor pathophysiology, including apoptosis (TUNEL), proliferation (Ki-67), VEGFR2 expression, and homologous recombination (HR) repair (BRAC1). Briefly, tissue sections were deparaffinized and rehydrated using a graded alcohol series, microwaved in 0.01 M citrate buffer (pH = 6.0) for 10 min to retrieve the antigen, and then incubated in 1% H2O2 for an additional 10 min to inactivate endogenous horseradish peroxidase (HRP). Following a 30-min incubation in a protein block (Dako, Hamburg, GER), the tissue sections were incubated with the aforementioned antibodies (VEGFR2, 1:50; Ki-67, 1:100; BRAC1, 1:100) for 90 min, followed by incubation in an HRP polymer conjugated secondary antibody (Dako, cat#K4061) for 40 min. The immunofluorescence reactions were visualized using DAB/H2O2. The VEGFR2+ microvessels and the labeling rates of Ki-67 and BRCA1 were evaluated by the average value of 10-15 random fields (at 200×) for each specimen. Specific primary antibodies were used for the TUNEL kit (Roche, Mannheim, GER) and the detection of VEGFR2 (Cell Signalling, Cambridge, UK), Ki-67 (Abcam, Cambridge, UK), and BRAC1 (Abcam, Cambridge, UK).
TUNEL assay
Terminal deoxynucleotidyl transferase dUTP nick end labeling (TUNEL) staining was performed as previously described [Citation18], using in situ Apoptosis Detection Kit (Roche, Mannheim, GER) according to the manufacturer’s instructions.
Statistical analysis
All statistical analyses were performed using GraphPad Prism 8.4.3 or R version 4.0.2. The experimental data are presented as mean ± SD. Comparisons between the treatment groups were performed by unpaired Student’s t-test, ANOVA, or the Wilcoxon rank-sum test when appropriate. P-value < 0.05 was considered to indicate a statistically significant difference.
Results
The combination of ENZ and ATO attenuates cell proliferation by arresting the cells in the G2/M phase and induces enhanced apoptosis
The enhanced antitumor effects of ENZ and ATO in combination on C4-2B cells were examined by colony formation, cell cycle, and DNA fragmentation assays. The colony formation assay suggested that treatment with ENZ or ATO only could moderately restrain the proliferation of C4-2B cells, and their combined treatment enhanced this inhibitory effect compared with that of DMSO (p < 0.001) (). More importantly, the combination of ENZ and ATO significantly inhibited the proliferation of C4-2B cells compared with those of ENZ or ATO alone (p < 0.001 for both). Cell cycle analysis indicated that ENZ or ATO induced cell cycle arrest at the G2/M phase (ENZ: 56%; ATO: 50%), whereas increased the sub-G1 cell population accompanied by a reduction of the G0/G1 cell population compared with that of DMSO-treated cells (). A significant increase in the sub-G1 cell population when cells were treated with the combination of ENZ and ATO compared with the effects of ENZ or ATO alone (p < 0.001 for both), which was accompanied by a decrease in the G2/M cell population (). In addition, ENZ or ATO treatment could induce moderate apoptosis compared with DMSO treatment, but the combination of ENZ and ATO induced higher levels of apoptosis than ENZ or ATO alone (p < 0.01 for both) (). The aforementioned data suggested that ATO synergistically enhanced the antitumor effects of ENZ on CRPC by attenuation of cell proliferation and induction of enhanced apoptosis.
Figure 1. The effects of combined ENZ and ATO on the proliferation of CRPC cells and inducing enhanced apoptosis. A-B. The antiproliferative effect of ENZ and ATO in combination was evaluated by colony formation assay. C-E. FACS analysis of C4-2B cells with different treatments and quantification of cell cycle distribution. F. DNA fragmentation was analyzed using ELISA detection. The data are presented as mean ± SD of three independent measurements performed in triplicate. **** p < 0.0001, *** p < 0.001, ** p < 0.01.
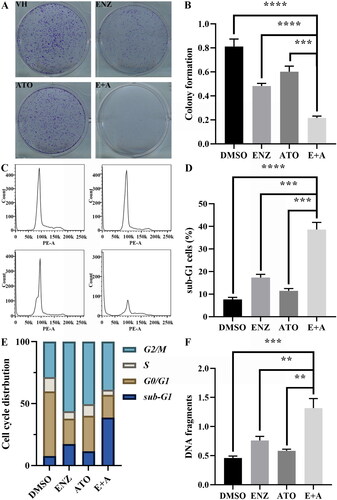
Involvement of DNA damage repair signaling in the combined effect of ENZ and ATO on the induction of cell death
Abnormal activation of DNA damage repair pathways has been shown to play an important role in the occurrence and progression of PCa [Citation15, Citation19–21], which was also demonstrated in our previous study by analysis of the independently published gene expression microarray (GSE32269) from the GEO database [Citation17]. As shown in , expression levels of the DNA damage repair-related genes, notably TOPBP1, ATR, CHEK1, and CDC25C were significantly upregulated in metastatic CRPC compared with those noted in primary PCa. Following drug resistance to ENZ, the aforementioned genes were also increased (), suggesting that the DNA damage repair pathways may also play important roles in the process of CRPC resistance to ENZ.
Figure 2. Abnormal upregulation of DDR-related pathways was involved in the progression of PCa. A. The DEGs of DDR-related pathways and the targets of ATO were derived from the Drugbank database, and visualized in the heatmap with GSE32269 data. B. Significant upregulation of the TOPBP1-ATR-CHEK1-CDC25C signaling in metastatic CRPC compared to primary PCa. **** p < 0.0001, ** p < 0.01.
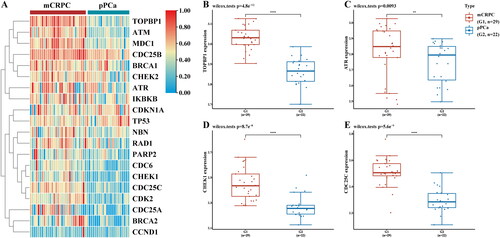
Figure 3. Involvement of DDR-related signalings in the effect of ENZ combination with ATO on CRPC cells. A. The DEGs of the DDR-related pathways and the targets of ATO were derived from the Drugbank database, and visualized by the heatmap with the RNA-seq library data. B. The frequency of the genes was visualized with the R package "wordcloud2". The larger the sample size of the word, the higher the frequency. C. The fold change of the expression levels of genes was visualized with the volcano plot. D-E. The expression levels of DNA damage and apoptosis-related genes of C4-2B cells following different treatments. ****p < 0.0001, ns p > = 0.05.
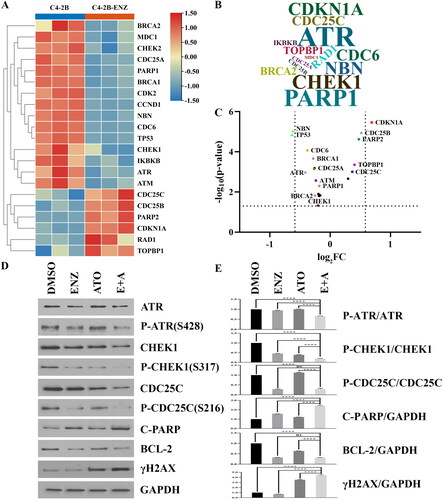
To investigate underlying mechanism of the synergistic interaction between the two agents, genes related to DNA damage repair from enriched pathways were explored by GSEA. The results indicated that ATR, CHEK1 and CDC25C had higher frequencies among these genes in the enriched pathways (). Western blot was employed to further assess the protein expression levels of the three genes, cell apoptosis marker C-PARP, anti-apoptotic marker BCL-2, and DNA damage marker γH2AX following single or combined treatment. And data indicated that the phosphorylation levels of ATR and CHEK1 (P-ATR and P-CHEK1) were significantly reduced when ENZ combination with ATO compared with ENZ or ATO treatment alone (p < 0.0001 for all) (). The expression level of phosphorylated CDC25C (P-CDC25C) was decreased by ATO alone and the combination of ENZ and ATO (p < 0.0001 for both), but not ENZ alone (p = 0.6171) (). Moreover, ENZ combination with ATO notably reduced the phosphorylation levels of BCL-2 compared with those of ATO alone (p < 0.0001), whereas caused a relative increase in the expression levels of phosphorylated C-PARP and γH2AX compared with ENZ or ATO treatment alone (p < 0.0001 for all) (). These data indicated that ENZ and ATO in combination could result in DNA double-strand breaks and induction of apoptosis in CRPC cells by affecting the TOPBP1-ATR-CHEK1-CDC25C signaling pathway.
Repression of the angiogenic activities of CRPC cells following combination treatment of ENZ and ATO
It has been widely accepted that angiogenesis plays a crucial role in the induction of metastasis and recurrence of PCa, which primarily results in malignant progression. To further explore the biological effects of ENZ and ATO co-treatment on angiogenesis, the expression levels of phosphorylated EGFR, AKT (P-EGFR, P-AKT), and VEGFR2 were evaluated by WB. It was found ATO treatment could reduce the expression levels of P-AKT and VEGFR2 compared with those of DMSO-treated C4-2B cells (). Moreover, the combination of ENZ and ATO resulted in a reduction in the expression levels of P-EGFR, P-AKT and VEGFR2 compared with DMSO, ENZ or ATO alone (). These results suggested that the angiogenesis activities of CRPC cells can be significantly inhibited by the combined treatment of ENZ and ATO.
Tumor growth inhibition of C4-2B xenografts treated with the combination of ENZ and ATO
To further evaluate the synergistic inhibitory effects of ENZ and ATO on CRPC, subcutaneous tumor models were established and treated with different treatments according to the described protocol (). The tumor growth curves revealed that single or combined treatment could moderately suppress the growth of C4-2B xenografts compared with the DMSO-treated groups (). More notably, compared to ENZ or ATO alone, ENZ and ATO co-treatment indicated significant advantages in reducing the tumor volume in the later stage of the experiment (Day 27: p < 0.0001) (). In addition, their combination could further inhibit the tumor wet weight compared with single-agent treatment (p < 0.0001 for both) (). It suggested that the combination of ENZ and ATO was an effective treatment to prevent the malignant progression of CRPC.
Figure 5. The combination of ENZ and ATO inhibited the growth of C4-2B xenografts. A. The in vivo protocol of the experimental design and treatment groups. B-D. Significant differences were noted in the volume and wet weight of the C4-2B xenografts with different groups. The data are presented as mean ± SD, ****p < 0.0001.
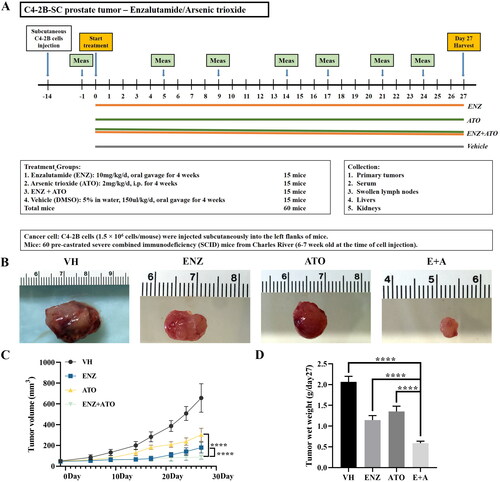
Effects on biological function and HR repair signaling of ENZ and ATO co-treated C4-2B xenografts
To further analyze the specific biological effects of the combined treatment of ENZ and ATO, the tissue sections from xenografts harvested on day 27 were immunostained with different antibodies. TUNEL staining indicated that ENZ and ATO co-treatment induced apoptosis of tumor cells compared with single-agent treatment (p < 0.001 for both) (). Moreover, their combination significantly reduced neo-angiogenesis of C4-2B xenografts compared with ENZ (p < 0.01) or ATO (p < 0.001) alone (). Ki-67 staining revealed that combined treatment of ENZ and ATO demonstrated an improved inhibitory effect on the proliferation of tumor cells compared with ATO (p < 0.05) or DMSO (p < 0.001) alone, but did not significantly suppress when compared with ENZ alone ().
Figure 6. The effects of ENZ and ATO co-treatment on the angiogenesis, apoptosis and DNA repair processes in vivo. A-D. These effects were monitored on C4-2B xenografts following different treatments and evaluated with immunohistochemical analysis. A. Apoptosis was evaluated with TUNEL staining. B. VEGFR2 expression visualized with IHC staining. C. Ki-67 expression visualized with IHC staining. D. HR repair was assessed for BRAC1. **** p < 0.0001, ***p < 0.001, **p < 0.01, *p < 0.05, ns p > = 0.05.
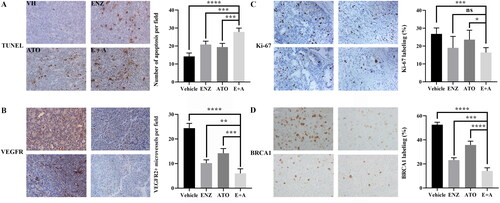
ENZ has been shown to reduce the expression levels of BRAC1, which results in impairment of the DDR-related pathway called homologous recombination (HR) repair [Citation15]. Considering the special role of ATO in inducing DNA damage, immunostaining was used to calculate the expression levels of BRAC1 when ATO was used alone or in combination with ENZ. As shown in , ENZ or ATO treatment could moderately reduce the expression levels of BRCA1 compared with DMSO, whereas their combination could significantly decrease the levels compared to the treatment of ENZ (p < 0.001) or ATO (p < 0.0001) alone. Taken together, these results indicated that ENZ and ATO co-treatment could significantly suppress proliferation, inhibit angiogenesis, and impair HR repair signaling partly by inducing enhanced apoptosis of C4-2B xenografts.
Discussion
In the present study, the synergistic antitumor effects of ENZ and ATO on CRPC cells were explored by in vitro and in vivo assays. It was found that not only could ENZ combination with ATO significantly inhibit cell proliferation and angiogenesis, but also induce cell arrest and apoptosis in C4-2B cells. In addition, interruption of the DDR-related pathways also occurred as a result of their combined effects. Furthermore, the synergistic inhibition of tumor growth was further confirmed in xenograft tissues. Altogether, these results suggested that ENZ combination with ATO inhibited CRPC progression by affecting the TOPBP1-ATR-CHEK1-CDC25C signaling pathway.
ENZ is an effective AR-targeting agent that would induce the cellular hypoxia microenvironment and activation of hypoxia signaling in CRPC [Citation22]. In this study, ENZ also showed an inhibitory effect on the malignant phenotype of cells and tumor growth. Regrettably, the majority of CRPC patients who received ENZ may develop drug resistance [Citation6]. Previous studies have shown that a high concentration of ATO can induce apoptosis by enhancing the generation of reactive oxygen species (ROS). Whereas, at low doses, ATO could inhibit tumor growth by inducing CRPC cells arresting in the G2/M phase [Citation11, Citation12]. These above findings were further confirmed by the present results. As expected, combination treatment exhibited a synergistic effect compared with the application of ENZ or ATO alone.
It has been shown that ROS is the most important of the three signaling pathways involved in the induction of apoptosis on PCa [Citation12], which could induce DNA damage leading to the activation of DNA damage repair (DDR) [Citation23]. Previous studies suggested that aberrant activation of DDR-related pathways plays a crucial role in PCa development and progression [Citation15, Citation19–21]. Besides, the expression levels of DDR-related genes were significantly upregulated in metastatic CRPC compared to primary PCa samples. To further explore the mechanism of the regulation of these genes, we focused on three main DDR-related pathways, namely TOPBP1-ATR-CHEK1-CDC25C, NBN-ATM-CHEK2-P53, and PARP-1 signaling pathways. Previous studies reveal that AR could promote CRPC survival via activation of the ATR-CHEK1 signaling pathway for initiating DDR [Citation20, Citation24], and regulation of the function of PARP-1 [Citation25]. Furthermore, associated genes of the three pathways were frequently expressed in the enriched DDR-related pathways, which were identified following ENZ resistance of CRPC cells in our previous study [Citation17]. When compared with those treated with ENZ or ATO alone, the expression levels of preliminarily identified signaling-related genes (ATR and CHEK1) and anti-apoptotic marker BCL-2 were significantly decreased in co-treated cells, while the DNA damage marker γH2AX and apoptotic marker C-PARP were up-regulated. Therefore, ENZ combination with ATO can further exacerbate DNA damage and induce enhanced cell apoptosis.
VEGFR2, a VEGF receptor, is generally acknowledged as the major mediator of VEGF-driven responses in tumor progression [Citation26]. Accumulating evidence has shown that ATO exerts antitumor activities by regulating the expression levels of key signaling molecules involved in the processes of tumor angiogenesis, such as VEGF and VEGFR2 [Citation27–30]. Our previous study highlighted that ENZ could suppress VEGF-VEGFR2 signaling in CRPC cells [Citation31]. In the current study, the expression level of VEGFR2 was modestly reduced following ENZ treatment, and this effect was potentiated by ATO treatment in vitro and confirmed by in vivo experiments. These data suggested that the combination of ENZ and ATO could synergistically dampen VEGF-VEGFR2 signaling-induced angiogenesis and thereby inhibited the progression of CRPC.
Taken together, our study demonstrated that ENZ and ATO in combination synergistically suppressed CRPC progression by inhibition of cell growth, exacerbation of DNA damage, and promotion of cell apoptosis through blockade of the AR-TOPBP1-ATR-CHEK1-CDC25C signaling pathway. In brief, their combination can improve anti-tumor efficacy, and consequently delay the occurrence of drug resistance.
Abbreviation index | ||
AKT/PKB | = | Protein kinase B |
APL | = | Acute promyelocytic leukemia |
AR | = | Androgen receptor |
ATM | = | Ataxia telangiectasia mutated gene |
ATO | = | Arsenic trioxide |
ATR | = | Ataxia-telangiectasia-mutated-and-Rad3-related kinase |
BCL-2 | = | B cell lymphoma 2 |
BRAC1 | = | Breast cancer susceptibility gene 1 |
cAMP | = | Cyclic adenosine monophosphate |
CDC25C | = | Cell division cycle 25c |
CHEK1/2 | = | Checkpoint kinase 1/2 |
C-PARP | = | Cleaved poly (ADP-ribose) polymerase |
CRPC | = | Castration-resistant prostate cancer |
DDR | = | DNA damage repair |
DEGs | = | Differentially expressed genes |
DMSO | = | Dimethyl sulfoxide |
EGFR | = | Epidermal growth factor receptor |
ELISA | = | Enzyme-linked immunosorbent assay |
ENZ | = | Enzalutamide |
FACS | = | Fluorescence-activated cell sorting |
FBS | = | Fetal bovine serum |
GAPDH | = | Glyceraldehyde-3-phosphate dehydrogenase |
GSEA | = | Gene set enrichment analysis |
HR | = | Homologous recombination |
HRP | = | Horseradish peroxidase |
IHC | = | Immunohistochemistry |
NBN | = | Nibrin |
OS | = | Overall survival |
P53 | = | Protein 53 |
PARP-1 | = | Poly (ADP-ribose) polymerase 1 |
PBS | = | Phosphate buffer solution |
PCa | = | Prostate cancer |
PI | = | Propidium iodide |
ROS | = | Reactive oxygen species |
RPMI-1640 | = | Roswell Park Memorial Institute-1640 |
SCID | = | Severe combined immunodeficiency |
SMAD2 | = | SMAD family member 2 |
SP | = | Specificity protein |
TGF-β | = | Transforming growth factor-β |
TUNEL | = | Terminal deoxynucleotidyl transferase dUTP nick-end labeling |
VEGFR2 | = | Vascular endothelial growth factor receptor 2 |
WB | = | Western blot |
γH2AX | = | γ-H2A histone family member X |
Disclosure statement
No potential conflict of interest was reported by the authors.
Data availability statement
The data that support the findings of this study are available from the corresponding author, Luo Y, upon reasonable request.
Additional information
Funding
References
- Bray F, Ferlay J, Soerjomataram I, et al. Global cancer statistics 2018: GLOBOCAN estimates of incidence and mortality worldwide for 36 cancers in 185 countries. CA Cancer J Clin. 2018;68(6):394–424.
- Sooreshjani MA, Nikhil K, Kamra M, et al. LIMK2-NKX3.1 engagement promotes castration-resistant prostate cancer. Cancers (Basel). 2021;13(10):2324.
- Chandrasekar T, Yang JC, Gao AC, et al. Mechanisms of resistance in castration-resistant prostate cancer (CRPC). Transl Androl Urol. 2015;4(3):365–380.
- Guerrero J, Alfaro IE, Gómez F, et al. Enzalutamide, an androgen receptor signaling inhibitor, induces tumor regression in a mouse model of castration-resistant prostate cancer. Prostate. 2013;73(12):1291–1305.
- Shah N, Wang P, Wongvipat J, et al. Regulation of the glucocorticoid receptor via a BET-dependent enhancer drives antiandrogen resistance in prostate cancer. Elife. 2017;6:e27861.
- Chou FJ, Lin C, Tian H, et al. Preclinical studies using cisplatin/carboplatin to restore the enzalutamide sensitivity via degrading the androgen receptor splicing variant 7 (ARv7) to further suppress enzalutamide resistant prostate cancer. Cell Death Dis. 2020;11(11):942.
- Antonarakis ES, Lu C, Wang H, et al. AR-V7 and resistance to enzalutamide and abiraterone in prostate cancer. N Engl J Med. 2014;371(11):1028–1038.
- Jin Z, Yi X, Yang J, et al. Liposome-coated Arsenic-manganese complex for magnetic resonance Imaging-Guided synergistic therapy against carcinoma. Int J Nanomedicine. 2021;16:3775–3788.
- Abudoureyimu A, Muhemaitibake A. Arsenic trioxide regulates gastric cancer cell apoptosis by mediating cAMP. Eur Rev Med Pharmacol Sci. 2017;21(3):612–617.
- Jutooru I, Chadalapaka G, Sreevalsan S, et al. Arsenic trioxide downregulates specificity protein (Sp) transcription factors and inhibits bladder cancer cell and tumor growth. Exp Cell Res. 2010;316(13):2174–2188.
- Lu M, Xia L, Luo D, et al. Dual effects of glutathione-S-transferase pi on As2O3 action in prostate cancer cells: enhancement of growth inhibition and inhibition of apoptosis. Oncogene. 2004;23(22):3945–3952.
- Maeda H, Hori S, Nishitoh H, et al. Tumor growth inhibition by arsenic trioxide (As2O3) in the orthotopic metastasis model of androgen-independent prostate cancer. Cancer Res. 2001;61(14):5432–5440.
- Ji H, Li Y, Jiang F, et al. Inhibition of transforming growth factor beta/SMAD signal by MiR-155 is involved in arsenic trioxide-induced anti-angiogenesis in prostate cancer. Cancer Sci. 2014;105(12):1541–1549.
- Rosenblatt AE, Burnstein KL. Inhibition of androgen receptor transcriptional activity as a novel mechanism of action of arsenic. Mol Endocrinol. 2009;23(3):412–421.
- Li L, Karanika S, Yang G, et al. Androgen receptor inhibitor-induced "BRCAness" and PARP inhibition are synthetically lethal for castration-resistant prostate cancer. Sci Signal. 2017;10(480):eaam7479.
- Mirzaei A, Rashedi S, Akbari M, et al. Combined anticancer effects of simvastatin and arsenic trioxide on prostate cancer cell lines via downregulation of the VEGF and OPN isoforms genes. J Cell Mol Med. 2022;26(9):2728–2740.
- Feng T, Wei D, Zhao J, et al. Construction of enzalutamide-resistant cell model of prostate cancer and preliminary screening of potential drug-resistant genes. Exp Biol Med. 2021;246(15):1776–1787.
- Li C, Wang Y, Zhang H, et al. An investigation on the cytotoxicity and caspase-mediated apoptotic effect of biologically synthesized gold nanoparticles using cardiospermum halicacabum on AGS gastric carcinoma cells. Int J Nanomedicine. 2019;14:951–962.
- Karanika S, Karantanos T, Li L, et al. Targeting DNA damage response in prostate cancer by inhibiting androgen receptor-CDC6ATR-Chk1 signaling. Cell Rep. 2017;18(8):1970–1981.
- Li L, Chang W, Yang G, et al. Targeting poly(ADP-ribose) polymerase and the c-Myb-regulated DNA damage response pathway in castration-resistant prostate cancer. Sci Signal. 2014;7(326):ra47.
- Luo Y, Li M, Zuo X, et al. β‑Catenin nuclear translocation induced by HIF‑1α overexpression leads to the radioresistance of prostate cancer. Int J Oncol. 2018;52(6):1827–1840.
- Liu B, Sun Y, Tang M, et al. The miR-361-3p increases enzalutamide (enz) sensitivity via targeting the ARv7 and MKNK2 to better suppress the enz-resistant prostate cancer. Cell Death Dis. 2020;11(9):807.
- Srinivas US, Tan BWQ, Vellayappan BA, et al. ROS and the DNA damage response in cancer. Redox Biol. 2019;25:101084.
- Ide H, Lu Y, Yu J, et al. Testosterone promotes DNA damage response under oxidative stress in prostate cancer cell lines. Prostate. 2012;72(13):1407–1411.
- Schiewer MJ, Goodwin JF, Han S, et al. Dual roles of PARP-1 promote cancer growth and progression. Cancer Discov. 2012;2(12):1134–1149.
- Melincovici CS, Boşca AB, Şuşman S, et al. Vascular endothelial growth factor (VEGF) - key factor in normal and pathological angiogenesis. Rom J Morphol Embryol. 2018;59(2):455–467.
- Xiao YF, Liu SX, Wu DD, et al. Inhibitory effect of arsenic trioxide on angiogenesis and expression of vascular endothelial growth factor in gastric cancer. World J Gastroenterol. 2006;12(36):5780–5786.
- Yang MH, Chang KJ, Zheng JC, et al. Anti-angiogenic effect of arsenic trioxide in lung cancer via inhibition of endothelial cell migration, proliferation and tube formation. Oncol Lett. 2017;14(3):3103–3109.
- Yang MH, Zang YS, Huang H, et al. Arsenic trioxide exerts anti-lung cancer activity by inhibiting angiogenesis. Curr Cancer Drug Targets. 2014;14(6):557–566.
- Carney DA. Arsenic trioxide mechanisms of action–looking beyond acute promyelocytic leukemia. Leuk Lymphoma. 2008;49(10):1846–1851.
- Luo Y, Azad AK, Karanika S, et al. Enzalutamide and CXCR7 inhibitor combination treatment suppresses cell growth and angiogenic signaling in castration-resistant prostate cancer models. Int J Cancer. 2018;142(10):2163–2174.