Abstract
The crab Geryon longipes lives in muddy bottoms of bathyal environments. Its epibiontic assemblage was studied in a population from the Southern Adriatic Sea. Four species of invertebrates were observed on the crab exoskeleton. The most abundant was the hydroid Leuckartiara cf. octona; the bryozoan Triticella flava was also quite frequent, while another hydroid, Hydractinia sp., and a bryozoan, Arachnoidea sp. were only occasionally observed. The study of the abundance and the distribution of the two most common epibionts in relation to the crab revealed a different spatio‐temporal pattern of colonisation between the hydroid and the bryozoan.
Introduction
Geryon longipes A. Milne Edwards, 1881 is one of the most common deep‐sea brachyuran crabs inhabiting the bathyal muddy bottoms of the Mediterranean Sea (Mori & Belloni Citation1985). The species is often infested by the polychaetes Ophryotrocha spp. (Mori & Belloni Citation1985; Martin et al. Citation1991) living inside the branchial chambers but until now no data were available about the epibionts present on its external surface.
The benefit of epibiosis on crabs resides in them being one of the few hard substrates available in deep muddy habitats (Abellò et al. Citation1990). Moreover, epibionts are transported across the substrate improving their possibility of food collection, avoiding sedimentation and taking advantage of the food lost by the crab. On the other hand, the life on the crab exoskeleton is strongly constrained due to the reduced available space and particularly due to the temporal instability of the substratum determined by the moulting rate (Gili et al. Citation1993). Consequently, only small epibionts, characterised by fast life cycles, settle and reproduce on crabs and are more common on the adults.
Aim of this work is the description of the epibiontic assemblage recorded on the exoskeleton of G. longipes from Southern Adriatic Sea with particular attention to the most common species, the hydroid Leuckartiara cf. octona.
Several species of hydroids were until now observed on crabs exoskeleton. For example Clytia linearis (Thornely, 1899), Phialella quadrata (Forbes, 1848) and Turritopsis nutricula McCrady, 1856 were studied on the carapace of the homolid Paromola cuvieri (Risso, 1816) (Mori & Manconi Citation1990). Hydractinia carolinae Fraser Citation1912, was found on the legs of a crab dredged in North Carolina (Fraser Citation1912). Podocoryne borealis (Mayer, 1900) and Podocoryne carnea M. Sars, 1846 occurred on several crab species (Edwards Citation1972). Hydroids are often found on the exoskeleton of deep‐sea crabs (Abellò et al. Citation1990), in particular Stegopoma plicatile (Sars, 1863) was recorded on the portunid Bathynectes piperitus Manning & Holthuis, 1981 (Gili et al. Citation1993) while the Rosalindidae Rosalinda incrustans (Kramp, 1947) forms crust‐like expansion covering the carapace and the spines of Anamathia rissoana (Roux, 1828) (Vervoort Citation1966). On these deep‐sea crabs other small benthic organisms such as bryozoans, polychaetes, cirripeds and protozoans (Mori & Belloni Citation1985; Abellò et al. Citation1990; Mori & Manconi Citation1990; Martin et al. Citation1991; Gili et al. Citation1993; Gordon & Wear Citation1999; Fernandez‐Leborans & Gabilondo Citation2006) were frequently recorded.
Materials and methods
Eighteen specimens of G. longipes, 16 males and 2 females, were collected by fish traps on a muddy bottom, 1000 m depth, off Monopoli (Bari, Southern Adriatic Sea) in June 2003, and fixed in alcohol 80°. In the laboratory, each specimen was photographed, the carapace width (distance between base of posterior anterolateral spines) (Figure ) was measured and the specimens grouped in four size classes (3 cm, 4 cm, 5 cm, and ⩾6 cm). For each crab the epibionts were identified and the single zooids were counted on the following portions of the animal: carapace, A—anterior dorsal surface, B—posterior dorsal surface, C—antero‐lateral ventral surface, D—sternum and abdomen, E—junction between abdomen and cephalothorax; chelipeds, 1—junction between the mobile and fixed finger; walking legs, 2—proximal segments (coxa/ischium/basis), 3—medial segments (carpus/merus), 4—distal segments (propodus/dactylus) (Figure ).
Figure 1. Portions of the crab exoskeleton. Carapace: A, anterior dorsal surface; B, posterior dorsal surface; C, antero‐lateral ventral surface; D, sternum and abdomen; E, junction between abdomen and carapace. The black bar shows the distance between base of posterior anterolateral spines. Chelipeds: (1) chela, articulation of mobile finger; walking legs: (2) proximal segments coxa/ischium/basis; (3) central segments carpus/merus; (4) distal segments propodus/dactylus.
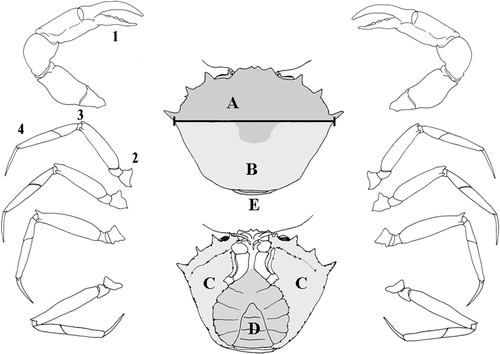
Flattened dark crusts covered eight crabs. Small portions of exoskeleton with this dark coloration were prepared for SEM observation. The EDAX analysis revealed that the dark coloration was due to deposits of manganese oxide, a mineral common in deep muds. Considering that the presence of these black areas might indicate a long time interval since the last moult, we compared, inside the same size classes, the number of epibionts on dark and clean crabs.
Results
The epibionts
Four species of epibionts were found on the carapace of G. longipes: two hydroids, Leuckartiara cf. octona and Hydractinia sp., and two bryozoans, Triticella flava and Arachnoidea sp. No polychaetes were observed inside branchial chambers.
Leuckartiara cf. octona growing on G. longipes forms stolonal colonies composed by unbranched or rarely branched hydrocauli (Figure ). The hydranths are large (200–260 µm in length and 220–270 in width), with a conical hypostome surrounded by 10–14 filiform tentacles, covered by a gelatinous and thin pseudohydrotheca extended up to the base of tentacles (Figure ). The pseudohydrotheca is wrinkled, encrusted by debris and often undulated in the distal part. When hydranths lack, the pseudohydrotheca is completely extended and smooth (Figure ). Pedicels are encrusted by foreign material and are composed by a perisarc thicker than that of pseudohydrothecae, sometimes 3–4 annuli are present in the proximal part.
Figure 2. Leuckartiara cf. octona: A, colony; B, hydranth; C, Pseudohydrotheca. Hydractinia sp.: D, colony; E, hydranth and medusa buds. F, Triticella flava. G, Arachnoidea sp. Scale bars: A, D, G, 500 µm; B, C, E, F, 200 µm.
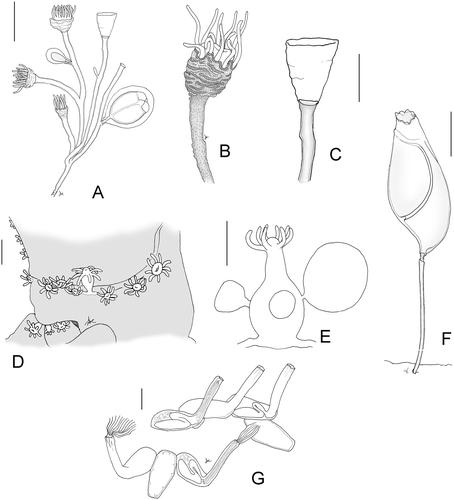
On fertile colonies medusa buds develop on stems and rarely on hydrorhiza. They are large, about 600 µm in length and 500 µm in width, enveloped by a perisarc sheet. A variable number of tentacular bulbs (2–4) are visible (Figure ).
The nematocysts both in hydranths and medusas are small microbasic euryteles (4–4.5×2–2.5 µm) and desmonemes (2.5×2.4 µm). The species determination is uncertain because we never observed free medusae.
Leuckartiara octona (Fleming, 1823) usually occurs on gastropods, but it was also found on crabs of the genus Halicarcinus (Millard Citation1975) and on shells inhabited by the hermit crab Pagurus bernhardus (Linnaeus, 1758) as well as on its chelipeds and pereopods (Fernandez‐Leborans & Gabilondo Citation2006).
Hydractinia sp. was observed only twice on G. longipes, one colony was fertile (Figure ). It was impossible to identify the species because only immature medusa buds were found. The hydroid forms stolonal colonies composed by hydranths measuring 450–600 µm. Medusa buds develop on the basal part of gonozoids and reach a diameter of 650 µm. The cnidome is represented by desmonemes (3.5–4 µm) and microbasic euriteles (2–3×7 µm). No spines were observed.
The bryozoan Triticella flava forms stolonal, unbranched colonies. The flattened zooid, characterized by a cuticular band called the frenaculum, is supported by a thin pedicel with an articulation on the distal end (Figure ).
Arachnoidea sp. forms colonies adherent to the substrate. The zooids are continuous with the lateral walls; the proximal part is tubular, elongated and directed upwards. The base of the zoecia presents short thickenings directed inwards similar to blunt teeth. Colonies are often covered by fine sediment (Figure ).
Distribution
The two most aboundant epibionts on G. longipes are Leuckartiara cf. octona and Triticella flava while Arachnoidea sp. and Hydractinia sp. were only occasionally observed. The number of zooids of Leuckartiara cf. octona and Triticella flava increases with the size of crabs, but the hydroid is totally absent on crabs belonging to the smallest size class (Figure ).
The different species appear to have a peculiar pattern of distribution on the crab exoskeleton (Figure ). Leuckartiara cf. octona is widely distributed both on the legs and on the ventral side of the body. The hydroid is more frequent on the proximal segments (coxa/ischium/basis) of walking legs, but is also abundant on central segments (carpus/merus), mostly concentrated on arthrodial membrane. Leuckartiara is also common on the chela at the articulation of the mobile finger, on articulation of abdomen with cephalotorax and on sternum and abdomen (Figure ). Triticella flava instead prefers the central segments (carpus/merus) of walking legs and is rare or totally absent on other parts of the crab surface (Figure ).
Figure 4. Average percentage(±SE) of epibionts in each portion of the crab exoskeleton. (A) Leuckartiara cf. octona. Epibionts are concentrated on junctions (arrows). (B) Triticella flava. The legend of the crab portions is explained in Material and methods. In the drawings, the parts colonised by the epibionts are showed in grey.
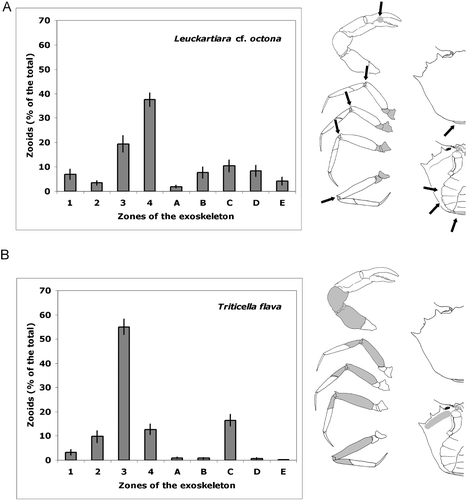
The presence of deposits of manganese oxide on the exoskeleton was positively related to crab sizes. All the crabs in the first size‐class were clean, in the two central classes the specimens with dark patches represented the 33% of the total, while in the class of the larger crabs their frequency increased to 75% (Figure ).
Figure 5. A, Percent of crabs with exoskeleton partly covered with manganese oxide deposits (dark bars) and with clean exoskeleton (white bars) in each size class. B, Example of a clean crab and of one with a manganese oxide cover, especially on the legs (arrows).
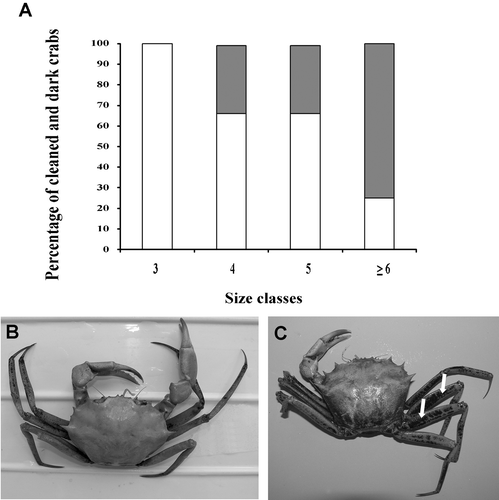
The number of Triticella flava on crabs with large cover of manganese oxide resulted to be higher than on clean crabs (P<0.005, t‐test) while no significant differences were observed for Leuckartiara cf. octona.
We observed that the zooids of Triticella flava are almost always oriented downwards, while the colonies of Leuckartiara cf. octona are always directed upwards.
Our samples contained only two female crabs, the smaller one (size‐class 3 cm) had very clean exoskeleton and few epibionts (45 zooids of Triticella flava), while the larger one (size‐class 4 cm) was very dark and was colonised by a high number of epibionts (118 zooids of Leuckartiara cf. octona; 158 of Triticella flava; and 93 of Arachnoidea sp.) comparable with that observed on male crabs of similar size.
Discussion
Our data suggest that the presence of epibionts is strongly dependent on the size of the crabs. Mori and Belloni (Citation1985), and Martin et al. (Citation1991) observed that the number of Ophryotrocha spp. infesting branchial chambers of G. longipes was directly proportional to the size of the crab. Abellò et al. (Citation1990) reported that most of smallest individuals of B. piperitus did not have epibionts and suggested that the abundance of epibionts is related to the time elapsed since the last moult. In fact, the moult frequency is higher in juvenile portunidae until puberty and epibionts are not able to settle and grow on them. On the contrary, post‐puberty crabs moult less frequently, and epibionts can develop on them.
Probably, the same phenomenon occurs in G. longipes. On our specimens Leuckartiara cf. octona was found only on crabs larger than 4 cm while Triticella flava is also observed on smaller hosts. This could be due to the ability of the bryozoan to settle earlier on the new exoskeleton following the moult, or to a faster grow, while Leuckartiara probably needs an older substratum.
There is a clear relationship between the crab sizes and the presence of crusts of manganese oxide on their exoskeleton (Figure ). This fact is in agreement with the hypothesis that the mineral crusts are due to a long permanence in the bottom sediments. This assumption allowed to establish a direct relationship between the age of the exoskeleton and the density of epibionts. In our specimens the number of zooids of Triticella flava was greater in dark crabs while no differences were shown by the number of Leuckartiara polyps in the two categories of crabs. This could be due to the different position of the two epibionts on the exoskeleton: both Leuckartiara cf. octona and Triticella flava predominate on legs, but the bryozoans settle on the exposed portions of the legs where they have a wide surface to colonise, while the hydroids live on the articular junctions between the segments, where they quickly saturate the available space. The hydroid is also abundant on coxa/ischium/basis, the most protected part. In one colony we found seven polyps on a scar present on a propodus, while the rest of the segment was not colonised. The hydroid could settle on sheltered areas to obtain protection, but we believe that Leuckartiara cf. octona prefers soft parts rather than hard ones and this is confirmed by the presence of some polyps on the scared, not yet calcified portions.
Triticella flava and Leuckartiara cf. octona are directed downwards and upwards, respectively; in this way, also when the two organisms occupy the same segment, they never compete for space. Hydroids direct their oral portion upwards, and this is probably due to their diet dominated by small zooplanktonic organisms (Gili & Hughes Citation1995). However, many small organisms living on sediment (for example, nematodes) could be suspended by the leg movements of the crab during feeding, representing a further food supply for the polyps (Christensen Citation1967).
Several Authors recorded a greater species richness and diversity of epibionts on male crabs (Mori & Belloni Citation1985; Martin et al. Citation1991; McGaw Citation2006) due to their larger size. The study of the two females present in our sample shows a cover comparable to that of the males of the same size but we have no conclusive evidences on this subject due to the paucity of specimens.
The life on a living substratum opens for the epibiontic organisms new ecological opportunities like the possibility to live on soft bottoms (Abellò et al. Citation1990). This is particularly evident for hydroids whose epibiontic aptitude is enhanced by the perisarc layer that isolates the living coenosarc from the host tissues (Puce et al. Citation2007). Probably for this reason hydrozoans represent the benthic group most involved in symbiotic relationships, with entire genera or families associated to specific groups (e.g. Puce et al. Citation2005, Citation2007). The crab exoskeleton is an inert and ephemeral substratum and for this reason it selects opportunistic species with limited relationships with the host. During the hydrozoan evolution, other groups, have established more specific cooperative relationships with their hosts giving rise to very intense speciation events.
Acknowledgements
We are very grateful to J. Winston and A. Balduzzi for their help with the identification of the bryozoan epibionts.
References
- Abellò , P. , Villanueva , R. and Gili , J. M. 1990 . Epibiosis in deep sea crab populations as indicator of biological and behavioural characteristics of the host. . Journal of Marine Biology Association UK , 70 : 687 – 695 .
- Christensen , H. E. 1967 . Ecology of Hydractinia echinata (Fleming) (Hydroidea, Athecata). 1. Feeding biology. . Ophelia , 4 : 245 – 275 .
- Edwards , C. 1972 . The hydroids and the medusae Podocoryne areolata, P. borealis and P. carnea. . Journal of Marine Biology Association UK , 52 : 97 – 144 .
- Fernandez‐Leborans , G. and Gabilondo , R. 2006 . Taxonomy and distribution of the hydrozoan and protozoan epibionts on Pagurus bernhardus (Linnaeus, 1758) (Crustacea, Decapoda) from Scotland. . Acta Zoologica (Stockholm) , 87 : 33 – 48 .
- Fraser , C. 1912 . Some hydroids of Beaufort, North Carolina. . Bulletin of the United States Bureau of Fisheries , 30 : 337 – 387 .
- Gili , J. M. , Abellò , P. and Villanueva , R. 1993 . Epibionts and intermoult duration in the crab Bathynectes piperitus. . Marine Ecology Progress Series , 98 : 107 – 113 .
- Gili , J. M. and Hughes , R. G. 1995 . The ecology of marine benthic hydroids. . Oceanography and Marine Biology: An Annual Review , 33 : 351 – 426 .
- Gordon , D. P. and Wear , R. G. 1999 . A new ctenostome bryozoan ectosymbiotic with terminal‐moult paddle crab (Portunidae) in New Zealand. . New Zealand Journal of Zoology , 26 : 373 – 380 .
- Martin , D. , Abellò , P. and Cartes , J. 1991 . A new species of Ophryotrocha (Polychaeta: Dorvilleidae) commensal in Geryon longipes (Crustacea: Brachyura) from the Western Mediterranean Sea. . Journal of Natural History , 25 : 279 – 292 .
- McGaw , J. 2006 . Epibionts of sympatric species of Cancer crabs in Barkley Sound, British Columbia. . Journal of Crustacean Biology , 26 : 85 – 93 .
- Millard , N. A. H. 1975 . Monograph on the Hydroida of Southern Africa. . Annals of the South African Museum , 68 : 1 – 513 .
- Mori , M. and Belloni , S. 1985 . Distribution, aboundance and infestation of Ophryotrocha geryonicola (Anellida: Dorvilleidae) in Geryon longipes (Crustacea: Decapoda: Geryonidae) of Ligurian bathyal bottoms. . Oebalia , 11 : 277 – 287 .
- Mori , M. and Manconi , R. 1990 . Macroepibiontes associated with Paramola cuvieri (Risso, 1816) (Decapoda, Homolidae) of the Ligurian Sea. . Crustaceana , 58 : 124 – 129 .
- Puce , S. , Calcinai , B. , Bavestrello , G. , Cerrano , C. , Gravili , C. and Boero , F. 2005 . Hydrozoa (Cnidaria) symbiotic with Porifera: a review. . P.S.Z.N.I. Marine Ecology , 26 : 73 – 81 .
- Puce , S. , Bavestrello , G. , Di Camillo , C. G. and Boero , F. 2007 . Symbiotic relationships between hydroids and bryozoans. . Symbiosis , 44 : 137 – 143 .
- Vervoort , W. 1966 . Skeletal structure in the Solanderiidae and its bearing on hydroid classification. . The Cnidaria and their Evolution , 16 : 373 – 396 .