Abstract
Polychaetes are a well-represented group in most marine and estuarine environments, both in terms of number of individuals and species; they typically constitute a significant percentage of the total macrofaunal diversity. The aim of our analyses was to characterise the biodiversity and regional distribution patterns of polychaete assemblages from the continental shelf (0–200 m) of the North Sea, English Channel and Irish Sea. Variations in depth and local distribution of sediment types, amongst other environmental factors, are the main factors affecting the pattern of distribution of benthic polychaetes. Here we applied and compared different approaches to draw the diversity pattern of this marine invertebrate group on a regional biogeographical scale, and to identify the main factors structuring these patterns.
Introduction
Among marine species, macrobenthos inhabiting marine soft-bottom habitats is especially important from an ecological standpoint because: (1) it plays a significant role in a large variety of ecological processes such as nutrient and pollutant cycling (Gilek et al. Citation1997; Gunnarsson et al. Citation2000), or sediment transport (Aller & Yingst Citation1985), and (2) its (specific) composition influences ecosystem functions (Snelgrove et al. Citation1997) and can be used to assess the quality of benthic habitats (Pearson & Rosenberg Citation1978; Borja et al. Citation2000; Simboura & Zenetos Citation2002; Rosenberg et al. Citation2004; Dauvin & Ruellet Citation2007).
Annelid polychaetes are one of the most characteristic groups of soft-bottom benthic communities (Knox Citation1977) and one of the richest invertebrate benthic taxa with regard to species number (Arvanitidis et al. Citation2002). This group, which often dominates benthic macrofauna (Grémare et al. Citation1998), is known to comprise mainly 60–70% of macrozoobenthic individuals on soft bottoms (Knox Citation1977; Dogan et al. Citation2005), and can be used as ‘markers’ of different ecological conditions (Gambi & Giangrande Citation1986). Polychaetes, as surrogates for benthic biodiversity in marine sediments, can be extremely informative in assessing the condition or health of benthic environments (Reish Citation1955; Pearson & Rosenberg Citation1978; Bellan et al. Citation1988; Pocklington & Wells Citation1992; Olsgard & Somerfield Citation2000; Belan Citation2003; Ergen et al. Citation2006; Mutlu et al. Citation2010).
Depth, latitude and longitude are known to influence spatial structure of marine benthic assemblages (Nybakken Citation1982). Growth, reproduction and abundance of a large number of marine soft-bottom faunal species are influenced by great environmental variability in littoral ecosystems (Rhoads & Young Citation1970). Depth-related sedimentary, hydrographical and biological variables are most likely to be the temporal controlling factors of the shallow water communities (Gray Citation1981; Tsutsumi et al. Citation1990; Mcpherson Citation1991; Libes Citation1992; Karakassis & Eleftheriou Citation1997; Dernie et al. Citation2003). In marine soft sediments, spatial and temporal distribution of species are important for the understanding of interactions of species with each other and with the environment (Dauvin et al. Citation2004).
An important focus for biodiversity studies has been to test for the existence of latitudinal or bathymetric gradients in the diversity of soft sediment biotas (Clarke Citation1992; Kendall & Aschan Citation1993; Rex et al. Citation1993; Dauvin et al. Citation1994). There have been many studies investigating diversity patterns, with special emphasis on how biodiversity varies with depth and latitude (Mokievsky & Azovsky Citation2002; Gage et al. Citation2004; Renaud et al. Citation2009), most of which have identified an increase in diversity with water depth through the bathyal zone (Rex Citation1981; Levin et al. Citation2001). On the other hand, the latitudinal gradient in species richness is a well-known biogeographical pattern in terrestrial ecology, where there is an observed increase in species diversity from high to low latitudes (from the poles to the tropics) (Pianka Citation1966; Gray et al. Citation1997; Gaston & Blackburn Citation2000). In general, the diversity of benthic communities has been shown to decline with increasing latitude in the northern hemisphere, and although this pattern has been described for most major terrestrial and some marine groups (Mcpherson Citation2002), the latitudinal gradient appears to be less consistent in marine environments (Clarke Citation1992; Roy et al. Citation2000).
In the eastern part of the English Channel and southern part of the North Sea, most studies referring to benthic communities have focused on some restricted areas (Davoult et al. Citation1988; Desroy et al. Citation2002; Sanvicente-Añorve et al. Citation2002; Dauvin et al. Citation2004; Van Hoey et al. Citation2004). Rees et al. (Citation1999) compared benthic diversity in the English Channel with fauna from the North and Celtic Seas at several study sites. Dauvin et al. (Citation1994) showed that there was no evidence of general gradients of diversity with depth and latitude in the polychaete fauna from the north-eastern Atlantic Ocean. They also mentioned the presence of a west to east gradient of impoverishment of the diversity of polychaetes, similar to the one shown by Cabioch et al. (Citation1977).
The objectives of this article were to identify the latitudinal, longitudinal and bathymetric patterns of the polychaete fauna from the North-eastern Atlantic continental shelf, i.e. from the North Sea, English Channel, Irish Sea and Outer Bristol Channel, where quantitative databases are available, or where recent data were obtained (i.e. the eastern part of the English Channel). Using a large number of data collected from different databases, we tried to identify patterns of biodiversity at a regional scale within regions under the influence of Atlantic waters in the western part of the English Channel, the Irish Sea and the Outer Bristol Channel, and the northern part of the North Sea. In addition, those patterns under the influence of more continental conditions in the eastern part of the English Channel and the southern part of the North Sea, which were influenced by large inputs of freshwater from important estuarine systems such as the Seine, the Thames, the Rhine–Meuse system and the Scheldt.
Materials and methods
Study zones
The Greater North Sea is defined (OSPAR Citation2000) by coordinates from 48° to 62°N, and 5°W to 12°E. To the south, it embraces the entire English Channel bordered by England, France and Belgium, and to the east the waters of the Skagerrak and Kattegat, bounded by Denmark, Norway and Sweden. Exchange occurs principally through the influx of Atlantic water to the north and to a lesser extent via the English Channel, and from the Baltic to the east, along with northward efflux, mainly along the Norwegian coast. The North Sea is relatively shallow, with depths generally not exceeding 100 m, except in the northern North Sea and in the Norwegian Trench (Rees et al. Citation2007). Water depths generally increase from south to north, with coarser sediments to the south and to the east of the study area and finer sediments to the north. This pattern can be broadly linked to changes in bathymetry and tidal current velocities (Rees et al. Citation2007).
The English Channel is located in the temperate zone of the northeast Atlantic continental shelf. It is an epicontinental sea bordered by the UK to the north and France to the south. It is influenced by the Atlantic Ocean to the west and is connected to the North Sea through the Dover Strait; it covers an approximate surface of 77,000 km2. Bathymetry reaches approximately 174 m at its maximum and becomes progressively shallow towards the east and with distance from land. Depth is about 40 m in the centre of the Dover Strait (Carpentier et al. Citation2009; Foveau Citation2009). Because of high hydrodynamics, mainly due to tidal currents, there is a bio-sedimentary gradient from pebbles and coarse sand sediments in the regions dominated by high currents, to coarse, fine and muddy fine sand sediments in the bays and estuaries, and in the regions dominated by weaker currents. The English Channel, unlike the North Sea where sand and muddy sand dominate, is characterized by a large quantity of coarse sediments.
Ireland and Great Britain, the two largest islands on the northwest European continental shelf, are separated from each other by a channel which is loosely referred to as the Irish Sea. This channel is about 330 km in length and typically reaches 80–110 m in depth along the main north–south axis. The Outer Bristol Channel lies at the western end of the Bristol Channel from where it merges into the Celtic Sea (Mackie et al. Citation1995, 2006). The southern Irish Sea area is dominated by sandy gravel, with a distinct south-westerly strip of gravely sand (Mackie et al. Citation1995) while the Outer Bristol Channel is mainly covered by sandy sediments (Mackie et al. Citation2006).
Databases
The North Sea Benthos Project 2000 (NSBP), former North Sea Benthos Survey 1986 (NSBS) is an activity of the ICES (Rees et al. Citation2007). In the NSBP initiative, data from the North Sea from various sources were brought together and analysed jointly. The data management for the NSBP was described in Vanden Berghe et al. (Citation2009); the database contains distribution records, taxonomic information, geo-referenced sampling stations and abiotic data (Vanden Berghe et al. Citation2009). Most sampling was done by collecting infauna with grabs of the van Veen type (mostly two grabs of 0.1 m2 at each station; Rees et al. Citation2007).
Sources for the eastern English Channel database were threefold, and are described in detail by Carpentier et al. (Citation2009). First, a characterization of the macrobenthic communities of the eastern English Channel was initiated at the LOG (Marine Station in Wimereux) within the framework of the MABEMONO programme (MAcroBEnthos of the eastern English Channel and the south of the North Sea 2006–2007, CHARM 2 programme) (Foveau Citation2009). Second, results obtained during the Copale-Authie surveys (Desroy et al. Citation2002) were added to the data set, and third, the results of a detailed survey carried out in the Bay of Seine by Ghertsos (Citation2002), were also added to the database. In each campaign, two replicates (0.25 m2) with a Hamon grab were used covering a total surface area of 0.5 m2.
In the context of the BIOMÔR project, biodiversity was investigated for both organism and ecosystem categories in the southern Irish Sea (BIOMÔR 1) and the Outer Bristol Channel (BIOMÔR 4), as two of their planned reports on ‘Studies in Marine Biodiversity and Systematics from the National Museum of Wales’. The BIOMÔR 1 report sought to make an overall assessment of biodiversity in the southern Irish Sea, whereas the Outer Bristol Channel Marine Habitat Study (OBCMHS-BIOMÔR 4) report, sought to address the lack of recent broad-scale biological and geological data for the Outer Bristol Channel. In each campaign, two replicates (0.112 m2) with a van Veen grab were used for a total surface area of 0.224 m2 (Mackie et al. Citation1995, 2006).
An effort was made to harmonize the taxonomy across the different data sets used in this work. All names were matched both for spelling and for synonymy with the European Register of Marine Species (ERMS, http://www.marbef.org/data/erms.php).
Data analysis
Species diversity indices. For each site, univariate measures of diversity such as species richness (S), Shannon–Weiner index (exp Hʹ) log base 2 were calculated. As sample size is known to influence diversity measures in different ways, species richness was also calculated for a standard sample size (Heip et al. Citation1992) by using Hurlbert's (Citation1971) modification of Sanders (Citation1968) rarefaction methodology (Dauvin et al. Citation1994); rarefaction compensates for different sample sizes between stations (e.g. ES(100) = expected number of species in a hypothetical sample of 100 individuals) (Rees et al. Citation2007). Rarefaction is based on drawing random samples from the distributional pattern of individuals among species in the total sample, to obtain an estimate of the total number of species in a smaller sample of individuals. This method has become the standard way to compare samples of different size (Gray Citation2002). Species richness performs better than equitability indices in the study of large-scale patterns, and E(Sn ) is robust to sample size variations (Boucher & Lambshead Citation1995).
Multivariate analyses were undertaken using the PRIMER (Plymouth routines in multivariate ecological research) software package (Clarke & Warwick Citation2001; Clarke & Gorley Citation2006). A similarity matrix was constructed using the Bray–Curtis coefficient (Bray & Curtis Citation1957), and the relationship between community structure and environmental variables was examined using the BIOENV routine (Clarke & Ainsworth Citation1993). BIOENV uses a Spearman's rank correlation to test for significant correlations between similarity matrices of polychaete abundance and of environmental variables. Latitude, longitude, depth and sediment composition (percentage silt–clay fraction < 0.063 mm) were the abiotic variables analysed. These analyses were undertaken for all three databases separately.
Diversity indices were plotted against latitude, longitude and water depth to determine the extent to which polychaete patterns across latitudinal, longitudinal and bathymetric gradients were consistent with those reported in the literature.
Co-variation using a local linear kernel regression. Kernel smoothing belongs to a class of weighted moving averages that consists of applying a function known as the kernel to each data point in a database. In practice, this means that all the points in the database are weighted using the results of the computation of the kernel function as weights.
To do so, we considered the relationship between two variables X and Y, which are produced by simultaneous measurements. Regression analysis concerns the knowledge of how X can explain the variations of Y. Denoting (xi ,yi ) the measurements, we then assumed a relation of the form:
Species–area relationships. The method to estimate the species–area relationship (SAR) consisted of covering an object with a set of boxes of linear size (l), and calculating the mean species richness of boxes containing at least one point. This procedure was then repeated for boxes with different sizes until the whole study area fitted into a single box.
The relationship between the number of species and the area sampled is one of the best documented patterns in community ecology (Williamson Citation1988; Durret & Levin Citation1996). Although the fact that larger areas contain more species than smaller ones is obvious, there has been no consensus about the exact form of the SAR, and the shape and slope of the SAR have remained largely unexplained. The relationship is mostly linear under a log–log transformation, following the power equation
where S is the number of species, A is the area, and c and z are constants (Arrhenius Citation1921; Rosenzweig Citation1995; Durret & Levin Citation1996; Storch et al. Citation2003). Both values c and z are important to characterize this relationship. In past studies, the parameter z has been found to lie mostly in the range 0.2–0.5 (Rosenzweig Citation1995; Durret & Levin Citation1996; Lomolino Citation2000; Chave & Levin Citation2003; Begon et al. Citation2005).
Results
A total of 428 polychaete species from 794 sampling stations in the North Sea, 197 species from 398 sampling stations in the eastern English Channel, and 336 from 187 sampling stations in the Irish Sea and the Outer Bristol Channel were identified from a total of 1380 sampling stations and 580 polychaete species.
The SARs estimated by mean species number for each sample area were linear on a log–log scale as shown in . The estimated slope of the SAR for the North Sea was z = 0.4, for the English Channel it was z = 0.35, and for the Irish Sea–Outer Bristol Channel it was z = 0.32. These values were very close to each other and to typical values of z reported throughout the literature.
Figure 1. Species–area relationship log–log plot for the North Sea, English Channel and Irish Sea–Outer Bristol Channel data sets: S(A)=1.5A–0.4 for the North Sea, S(A)=4.2(A)0.35 for the English Channel and S(A)=7(A)0.32 for the Irish Sea–Outer Bristol Channel.
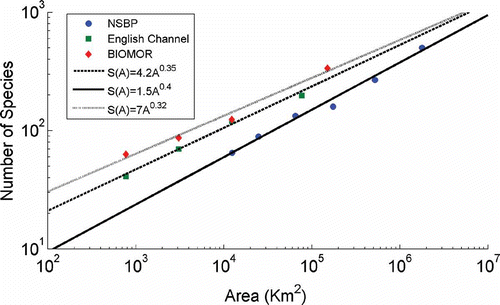
shows an increased number of individuals from the southern to the northern North Sea, while shows slight increases in abundance from the Irish Sea to the south-eastern North Sea.
Figure 2. Relationship between log abundance and increasing latitude for all samples. Conditional average (coloured line) was estimated using a Gaussian kernel with basic parameter h=2°.
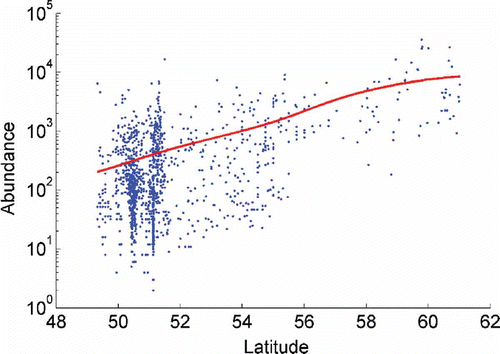
Figure 3. Relationship between log abundance and longitude for all samples. Conditional average (coloured line) was estimated using a Gaussian kernel with basic parameter h=2°.
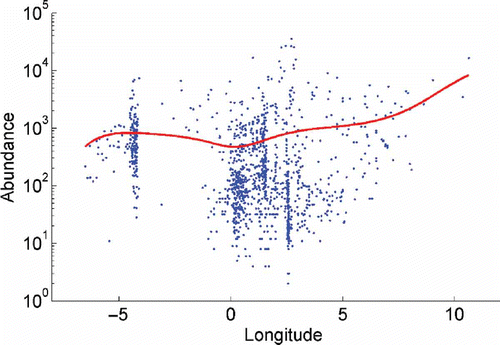
Shannon Diversity (figure not shown) and rarefaction indices (ES(100), ) showed increasing values with latitude, whereas a longitudinal trend in diversity also exists; with a decrease towards the eastern North Sea (), with the lowest values found in the estuaries region on the eastern North Sea.
Figure 4. Relationship between rarefaction ES(100) and latitude for all samples. Conditional average (coloured line) was estimated using a Gaussian kernel with basic parameter h=2°.
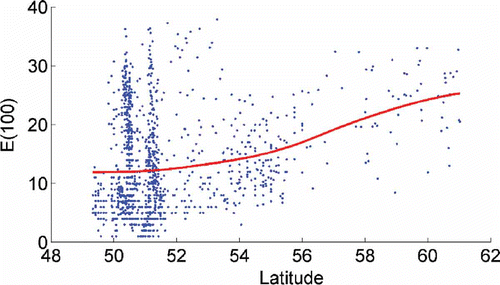
Figure 5. Relationship between rarefaction ES(100) and longitude for all samples. Conditional average (coloured line) was estimated using a Gaussian kernel with basic parameter h=2°.
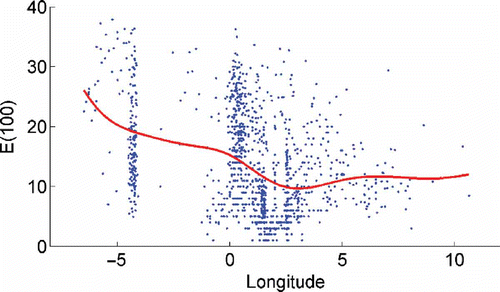
Multivariate patterns of community composition were related to latitude, longitude, depth and silt–clay percentage. The Pairwise Spearman rank correlation between environmental variables and diversity was estimated for all data () and separately for all data sets (tables not shown). This showed that no variables were highly correlated (all correlations < 0.95). All variables were used in the BIO-ENV analysis; the results are summarized in . The relations between single environmental factors and square-root transformed abundance data were generally weak (range of rs = 0.70 to –0.39). Longitude and depth showed higher degrees of correlation with the faunal composition than the other single factors and ‘explained’ the faunal patterns better than any subset of environmental variables.
Table I. Pairwise Spearman rank correlations between environmental variables and biotic diversity for the whole study area. Bold and italic are for statistically significant correlations
Table II. Relationship between environmental variables and polychaete data using BIOENV analysis. Up to three environmental variables, best explain these faunal patterns. Resulting values are weighted Spearman rank correlation coefficients (r s )
BIO-ENV analysis was first conducted on the overall data set and the resulting maximum correlation was rs = 0.382 for up to three variables (latitude, longitude and depth).
Discussion
Increasing areas can support more species both because the increasing habitat heterogeneity allows greater potential for specialization and because even in a homogenous environment smaller areas are, to some extent, random samples drawn from a larger population of species not all of the same size (Preston Citation1962; Begon et al. Citation2005). The species–area relationship (SAR) is one of the pillars of the study of spatial patterns of species diversity (Connor & McCoy Citation1979; Rosenzweig Citation1995; Durret & Levin Citation1996; Ney-Nifle & Mangel Citation1999; Lomolino Citation2000). Although alternative models have been proposed, the most commonly used form of the SAR is the power-function relationship, which produces straight lines when plotting the log values of species number and area. The slope of such lines, called z, is related to species turnover (Caswell & Cohen Citation1993; Westoby Citation1993; Rosenzweig Citation1995). The exponent z has been reported in a large variety of systems and its values have been found ranging between 0.1 and 0.4 (Connor & McCoy Citation1979; Rosenzweig Citation1995; Chave & Levin Citation2003; García Martín & Goldenfeld Citation2006). A larger value of z corresponds to a faster accumulation of species as area increases, indicating higher rates of species turnover among the sites that compose the larger region. The slope of a species–area curve can provide a measure of differentiation diversity, which is usually called β diversity; this is the diversity of a sample based on the way a species is grouped into subunits. That is, β diversity characterizes the difference in species composition from one place to another (Scheiner Citation2004). A power-law function like the SAR indicates that β diversity is scale-invariant: if we consider a larger or smaller scale, a change of scale does not modify its basic statistical behaviour (Solé et al. Citation1999). A SAR with a power-law form implies a self-similarity in the scaling patterns of species richness (Marquet et al. Citation2007).
The decrease in diversity with increasing latitude and depth is a well-documented pattern that has been described for some marine groups such as copepods, gastropods and bivalves (Rex et al. Citation1993; Rombouts et al. Citation2009), although an increasing number of researchers have shown that this pattern is not as universal as it was thought to be (Clarke Citation1992; Heip et al. Citation1992; Roy et al. Citation2000; Kindlmann et al. Citation2007; Rees et al. Citation2007). Dauvin et al. (Citation1994), using a smaller database, had already shown this for polychaetes of the northeastern Atlantic. In our work, species diversity and rarefaction indices (using 1380 sampling stations) showed increasing values with latitude north of 51°N, which is in accordance with the results of Heip et al. (Citation1992: 200 sampling stations) and Rees et al. (Citation2007: 950 sampling stations), for macro-zoobenthos of the North Sea and the English Channel. These patterns coincide with gradients in depth, bottom temperature and salinity (Renaud et al. Citation2009). South of 53°N, elevated diversities in the eastern English Channel, and also along parts of the southeast English coast, can be explained by the generally coarser substrata, which presents greater small-scale heterogeneity (Rees et al. Citation2007). It can also be explained by the influence of Atlantic waters and from the climatic gradient (a more severe range of temperatures in the eastern part of the channel than in the western part) that appears to be favourable for polychaete species. Künitzer et al. (Citation1992) showed that the bottom fauna of the North Sea is composed of northern elements that do not extend further south than the Dogger Bank and of southern elements; the shallow southern North Sea is, in contrast to deeper northern areas, influenced by the English Channel inflow which extends up to the Dogger Bank. The northern North Sea and part of the central North Sea is influenced by a different type of water than the rest of the North Sea (Künitzer et al. Citation1992). The general circulation in the North Sea varies little with seasons and is in general cyclonic; Atlantic waters entering the North Sea from the north do not reach the continental coastline but turn gradually eastward towards the Jutland coast and the Skagerrak (Heip et al. Citation1992). The lack of a decline in diversity of polychaetes and other macrobenthic groups with latitude has also been documented by several authors (Kendall & Aschan Citation1993; Dauvin et al. Citation1994; Kendall Citation1996; Ellingsen & Gray Citation2002; Kindlmann et al. Citation2007; Renaud et al. Citation2009), and as stated by the latter it could also be a consequence of the limited latitudinal range that only spans about 12° of latitude considered in this study. Nonetheless, the influence of the estuarine systems to the south of the study area should not to be ruled out.
We also observed a west to east gradient in the impoverishment of polychaete diversity, consistent with the results reported for the English Channel by Cabioch et al. (Citation1977) and Dauvin et al. (Citation1994), with a decrease towards the eastern North Sea and with a west to east transition from deeper, stratified to shallow, mixed waters (Rees et al. Citation1999). This is a region where less saline water is accounted for by the estuarine influences of the Rhine, Scheldt and Meuse systems, more continental influences prevail and species adapted to warmer waters are rare. As expected, the lowest values were found in the estuaries and on the homogenous fine sand sediments occurring in the southern part of the North Sea (less diverse in terms of sediment types). This may also be related to not only the reduced salinities there, but also the high climatic and hydrological variability and disturbing human influences, including pollution and eutrophication (Rees et al. Citation2007). According to Heip et al (Citation1992), the effect of longitude on diversity possibly shows the importance of current patterns on species distribution. These seemingly west-to-east trends shown with univariate methods could indicate that the eastern North Sea is generally impoverished in diversity. This part of the North Sea is the most remote and biogeographically apart from the species-rich Atlantic Ocean, and most strongly under the natural and anthropogenic ‘continental’ (mainland) influences (Rees et al. Citation2007), and less influenced by Atlantic waters. It is well known that coastal areas with high physical variability have low diversity (Gray et al. Citation1997). The great diversity of habitats in the English Channel and Irish Sea–Outer Bristol channel, due to a greater sedimentary and depth heterogeneities, could also factor in this decline in diversity.
Acknowledgements
This work was supported by Consejo Nacional de Ciencia y Tecnología (CONACyT), México. We thank Sylvie Zongo (LOG) for her help with the kernel smoothing methodology. The authors would also like to thank the North Sea Benthos Project (NSBP 2000) (VLIZ, ICES), Andrew S.Y. Mackie (National Museum Wales) and BIOMÔR project participants, INTERREG-CHARM 2 project participants and we also thank all the persons (researchers, engineers, technicians, ship crew) who collected and sorted the specimens used in this work. Peter Magee (www.anglais.webs.com) is acknowledged for polishing the English.
References
- Aller , RC and Yingst , JY. 1985 . Effects of the marine deposit-feeders Heteromastus filiformis (Polychaeta), Macoma balthica (Bivalvia), and Tellina texana (Bivalvia) on averaged sedimentary solute transport, reaction rates, and microbial distributions . Journal of Marine Research , 43 : 615 – 645 .
- Arrhenius , O. 1921 . Species and area . Journal of Ecology , 9 : 95 – 99 .
- Arvanitidis , C , Bellan , G , Drakopoulos , P , Valavanis , V , Dounas , C , Koukouras , A and Eleftheriou , A. 2002 . Seascape biodiversity patterns along the Mediterranean and the Black Sea: Lessons from the biogeography of benthic polychaetes . Marine Ecology Progress Series , 244 : 139 – 152 .
- Begon , M , Harper , JL and Townsend , CR. 2005 . Ecology: Individuals, populations and communities , Oxford : Blackwell Scientific Publications .
- Belan , TA. 2003 . Marine environmental quality assessement using polychaete taxocene characteristics in Vancouver harbour . Marine Environmental Research , 57 : 89 – 101 .
- Bellan , G , Desrosiers , G and Willsie , A. 1988 . Use of annelid pollution index for monitoring a moderately polluted littoral zone . Marine Pollution Bulletin , 19 : 662 – 665 .
- Borja , A , Franco , J and Pérez , V. 2000 . A marine biotic index to establish the ecological quality of soft-bottom benthos within European estuarine and coastal environments . Marine Pollution Bulletin , 40 : 1100 – 1114 .
- Boucher , J and Lambshead , P. 1995 . Ecological biodiversity of marine nematodes in samples from temperate, tropical, and deep-sea regions . Conservation Biology , 9 : 1596 – 1604 .
- Bray , JR and Curtis , JT. 1957 . An ordination of the upland forest communities in Southern Wisconsin . Ecological Monographs , 27 : 325 – 349 .
- Cabioch , L , Gentil , F , Glaçon , R and Retière , C. Le macrobenthos des fonds meubles de la Manche: Distribution générale et écologie générale . Proceedings of the 11th European Symposium on Marine Biology, Galway . October 1976 , Oxford. pp. 115 – 128 . Oxford : Pergamon Press .
- Carpentier , A , Martin , CS and Vaz , S. 2009 . Channel habitat atlas for marine resource management, final report/ Atlas des Habitats des Ressources Marines de la Manche Orientale , Boulogne sur Mer, France : IFREMER .
- Caswell , H and Cohen , JE. 1993 . “ Local and regional regulation of species–area relations: A patch-occupancy model ” . In Species diversity in ecological communities: Historical and geographical perspectives , Edited by: Ricklefs , RE and Schulter , D . 99 – 107 . Chicago, IL : University of Chicago Press .
- Chave , J and Levin , SA. 2003 . Scale and scaling in ecological and economic systems . Environmental and Resource Economics , 26 : 527 – 557 .
- Clarke , A. 1992 . Is there a latitudinal diversity cline in the sea? . Trends in Ecology & Evolution , 7 : 286 – 287 .
- Clarke , KR and Ainsworth , M. 1993 . A method of linking multivariate community structure to environmental variables . Marine Ecology Progress Series , 92 : 213 – 226 .
- Clarke , KR and Gorley , RN. 2006 . Primer v6: user manual/tutorial , Plymouth : PRIMER-E .
- Clarke , KR and Warwick , RM. 2001 . Change in marine communities: An approach to statistical analysis and interpretation , Plymouth : PRIMER-E .
- Connor , EF and McCoy , ED. 1979 . The statistics and biology of the species–area relationship . The American Naturalist , 113 : 791 – 833 .
- Dauvin , JC , Kendall , M , Paterson , G , Gentil , F , Jirkov , I , Sheader , M and De Lange , M. 1994 . An initial assessment of polychaete diversity in the northeastern Atlantic Ocean . Biodiversity Letters , 2 : 171 – 181 .
- Dauvin , JC and Ruellet , T. 2007 . Polychaete/amphipod ratio revisited . Marine Pollution Bulletin , 55 : 215 – 224 .
- Dauvin , JC , Thiébaut , E , Gomez-Gesteira , JL , Ghertsos , K , Gentil , F , Ropert , M and Sylvand , B. 2004 . Spatial structure of subtidal macrobenthic community in the Bay of Veys (western Bay of Seine, English Channel) . Journal of Experimental Marine Biology and Ecology , 3007 : 217 – 235 .
- Davoult , D , Dewarumez , JM , Prygiel , J and Richard , A. 1988 . Carte des peuplements benthiques de la partie française de la mer du Nord , Pas de Calais : IFREMER/Région Nord .
- Dernie , KM , Kaiser , MJ , Richardson , EA and Warwick , RM. 2003 . Recovery of soft sediment communities and habitats following physical disturbance . Journal of Experimental Marine Biology and Ecology , 285–286 : 415 – 434 .
- Desroy , N , Warembourg , C , Dewarumez , JM and Dauvin , JC. 2002 . Macrobenthic resources of the shallow soft-bottom sediments in the eastern English Channel and southern North Sea . ICES Journal of Marine Science , 60 : 120 – 131 .
- Dogan , A , Çinar , ME , Onen , M , Ergen , Z and Katagan , T. 2005 . Seasonal dynamic of soft-bottom zoobenthic communities in polluted and unpolluted areas of Izmir Bay (Aegean Sea) . Senckenbergiana Maritima , 35 : 133 – 145 .
- Durret , R and Levin , SA. 1996 . Spatial models for species–area curves . Journal of Theoretical Biology , 179 : 119 – 127 .
- Ellingsen , KE and Gray , JS. 2002 . Spatial patterns of benthic diversity: Is there a latitudinal gradient along the Norwegian continental shelf? . Journal of Animal Ecology , 71 : 373 – 389 .
- Ergen , Z , Çinar , ME , Dagli , E and Kurt , G. 2006 . Seasonal dynamics of soft-bottom polychaetes in Izmir Bay (Aegean Sea, Eastern Mediterranean) . Scientia Marina , 70S3 : 197 – 207 .
- Foveau , A. 2009 . Habitats et communautés du bassin oriental de la Manche Etat des lieux au début du XXIème siècle . Thèse de Doctorat de l'Université de Lille , 1 : 288
- Gage , JD , Lambshead , P , Bishop , JDD , Stuart , CT and Jones , NS. 2004 . Large-scale biodiversity pattern of Cumacea (Peracarida: Crustacea) . Marine Ecology Progress Series , 277 : 181 – 196 .
- Gambi , MC and Giangrande , A. 1986 . Distribution of soft-bottom polychaetes in two coastal areas of the Tyrrhenian Sea (Italy): Structural analysis . Estuarine, Coastal and Shelf Science , 23 : 847 – 862 .
- García Martín , H and Goldenfeld , N. 2006 . On the origin and robustness of power-law species–area relationships in ecology . Proceedings of the National Academy of Sciences , 103 : 10,310 – 10,315 .
- Gaston , KJ and Blackburn , T. 2000 . Patterns and process in macroecology , Oxford : Blackwell Science .
- Ghertsos , K. 2002 . Structure spatio-temporelle des peuplements macrobenthiques de la baie de Seine à plusieurs échelles d'observation . Thèse de Doctorat de l'Université de Lille , 1 : 181
- Gilek , M , Björk , M , Broman , D , Kautsky , N , Kautsky , U and Näf , C. 1997 . The role of the blue mussel, Mytilus edulis, in the cycling of hydrophobic organic contaminants in the Baltic Proper . Ambio , 26 : 202 – 209 .
- Gray , JS. 1981 . The ecology of marine sediments. An introduction to the structure and function of benthic communities , Cambridge : Cambridge University Press .
- Gray , JS. 2002 . Species richness of marine soft sediments . Marine Ecology Progress Series , 244 : 285 – 297 .
- Gray , JS , Poore , GCB , Ugland , KI , Wilson , RS , Olsgard , F and Johannessen , Ø. 1997 . Coastal and deep-sea benthic diversities compared . Marine Ecology Progress Series , 159 : 97 – 103 .
- Grémare , A , Amouroux , JM and Vetion , G. 1998 . Long-term comparison of macrobenthos within the soft bottoms of the Bay of Banyuls-sur-mer (Northwestern Mediterranean Sea) . Journal of Sea Research , 40 : 281 – 302 .
- Gunnarsson , J , Björk , M , Gilek , M , Granberg , M and Rosenberg , R. 2000 . Effects of eutrophication on contaminant cycling in marine benthic systems . Ambio , 29 : 252 – 259 .
- Heip , CHR , Basford , D , Craeymeersch , JA , Dewarumez , JM , Dörjes , J , de Wilde , P , Duineveld , GCA , Eleftheriou , A , Herman , PMJ Niermann , U . 1992 . Trends in biomass, density and diversity of North Sea macrofauna . ICES Journal of Marine Sciences , 49 : 13 – 22 .
- Hurlbert , SH. 1971 . The non-concept of species diversity: A critique and alternative parameters . Ecology , 52 : 577 – 586 .
- Karakassis , I and Eleftheriou , A. 1997 . The continental shelf of Crete: Structure of macrobenthic communities . Marine Ecology Progress Series , 160 : 185 – 196 .
- Kendall , MA. 1996 . Are Arctic soft-sediment macrobenthic communities impoverished? . Polar Biology , 16 : 393 – 399 .
- Kendall , MA and Aschan , M. 1993 . Latitudinal gradients in the structure of macrobenthic communities: A comparison of Arctic, temperate and tropical sites . Journal of Experimental Marine Biology and Ecology , 172 : 157 – 169 .
- Kindlmann , P , Schödelbauerová , I and Dixon , AFG. 2007 . “ Inverse latitudinal gradients in species diversity ” . In Scaling biodiversity , Edited by: Storch , D , Marquet , PA and Brown , JH . Cambridge : Cambridge University Press .
- Knox , G. 1977 . “ The role of polychaetes in benthic soft-bottom communities ” . In Essays on polychaetous annelids in memory of Dr Olga Hartman , Edited by: Reish , DJ and Fauchald , K . 547 – 604 . California : Allan Hancock Foundation, University of Southern California .
- Künitzer , A , Basford , D , Craeymeersch , JA , Dewarumez , JM , Dörges , J , Duineveld , GCA , Eleftheriou , A , Heip , CHR , Herman , PMJ Kingston , P . 1992 . The benthic infauna of the North Sea: Species distribution and assemblages . ICES Journal of Marine Science , 49 : 127 – 143 .
- Levin , LA , Etter , RJ , Rex , MA and Gooday , AJ. 2001 . Environmental influences of regional deep-sea species diversity The Annual Review of Ecology . Evolution, and Systematics , 32 : 51 – 93 .
- Libes , SA. 1992 . An introduction to marine biogeochemistry , New York, NY : Wiley .
- Lomolino , MV. 2000 . Ecology's most general, yet protean pattern: The species–area relationship . Journal of Biogeography , 27 : 17 – 26 .
- Mackie , ASY , James , JWC , Rees , EIS , Darbyshire , T , Philipott , SL , Mortimer , K , Jenkins , GO and Morando , A. 2006 . “ The Outer Bristol Channel Marine Habitat Study ” . In Studies in Marine Biodiversity and Systematics for the National Museum of Wales. BIOMÔR 4
- Mackie , ASY , Oliver , PG and Rees , EIS. 1995 . “ Benthic biodiversity in the southern Irish Sea ” . In Studies in Marine Biodiversity and Systematics from the National Museum of Wales. BIOMOR Reports 1
- Marquet , PA , Abades , SR and Labra , FA. 2007 . “ Biodiversity power-laws ” . In Scaling biodiversity , Edited by: Storch , D , Marquet , PA and Brown , JH . 470 Cambridge : Cambridge University Press .
- Mcpherson , E. 1991 . Biogeography and community structure of the decapod crustacean fauna off Namibia (southeast Atlantic) . Journal of Crustacean Biology , 11 : 401 – 415 .
- Mcpherson , E. 2002 . Large-scale species-richness gradients in the Atlantic Ocean . Proceedings of the Royal Society B , 269 : 1715 – 1720 .
- Mokievsky , V and Azovsky , A. 2002 . Re-evaluation of species diversity patterns of free-living nematodes . Marine Ecology Progress Series , 238 : 101 – 108 .
- Mutlu , E , Çinar , ME and Ergev , MB. 2010 . Distribution of soft-bottom polychaetes of the Levantine coast of Turkey, eastern Mediterranean Sea . Journal of Marine Systems , 79 : 23 – 35 .
- Ney-Nifle , M and Mangel , M. 1999 . Species–area curves based on geographic range and occupancy . Journal of Theoretical Biology , 196 : 327 – 342 .
- Nybakken , JW. 1982 . Marine biology. An ecological approach , New York, NY : Harper & Row .
- Olsgard , F and Somerfield , PJ. 2000 . Surrogates in marine benthic investigations – which taxonomic unit to target? . Journal of Aquatic Ecosystem Stress and Recovery , 7 : 25 – 42 .
- OSPAR . 2000 . Quality Status Report 2000, Region II – Greater North Sea , London : OSPAR Commission .
- Pearson , TH and Rosenberg , R. 1978 . Macrobenthic succession in relation to organic enrichment and pollution of the marine environment . Oceanography and Marine Biology Annual Review , 16 : 229 – 311 .
- Pianka , ER. 1966 . Latitudinal gradients in species diversity: A review of concepts . The American Naturalist , 100 : 33 – 46 .
- Pocklington , P and Wells , PG. 1992 . Polychaetes: Key taxa for marine environmental quality monitoring . Marine Pollution Bulletin , 24 : 593 – 598 .
- Preston , FW. 1962 . The canonical distribution of commonness and rarity . Ecology I: 185–215 ,
- Rees , HL , Eggleton , JD , Rachor , E and Vanden Berghe , E . Structure and dynamics of the North Sea benthos . ICES Cooperative Research Report No. 288 . 2007 . 258
- Rees , HL , Pendle , MA , Wadlock , R , Limpenny , DS and Boyd , SE. 1999 . A comparison of benthic biodiversity in the North Sea, English Channel, and Celtic Seas . ICES Journal of Marine Science , 56 : 228 – 246 .
- Reish , DJ. 1955 . The relation of polychaetous annelids to harbor pollution . US Public Health Report , 70 : 1168 – 1174 .
- Renaud , PE , Webb , TJ , Bjørgesæter , A , Karakassis , I , Kędra , M , Kendall , MA , Labrune , C , Lampadariou , N , Somerfield , PJ Włodarska-Kowalczuck , M . 2009 . Continental-scale patterns in benthic invertebrate diversity: Insights from MacroBen database . Marine Ecology Progress Series , 382 : 239 – 252 .
- Rex , MA. 1981 . Community structure in the deep-sea benthos . The Annual Review of Ecology, Evolution, and Systematics , 12 : 331 – 353 .
- Rex , MA , Stuart , CT , Hessler , RR , Allen , JA , Sanders , HL and Wilson , GDF. 1993 . Global-scale latitudinal patterns of species diversity in the deep-sea benthos . Nature , 365 : 636 – 639 .
- Rhoads , DC and Young , DK. 1970 . The influence of deposit-feeding organisms on sediment stability and community trophic structure . Journal of Marine Research , 28 : 150 – 178 .
- Rombouts , I , Beaugrand , G , Ibañez , F , Gasparini , S , Chiba , S and Legendre , L. 2009 . Global latitudinal variations in marine copepod diversity and environmental factors . Proceedings of the Royal Society B , 276 : 3053 – 3062 .
- Rosenberg , R , Blomqvist , M , Nilsson , HC , Cederwall , H and Dimming , A. 2004 . Marine quality assessment by use of benthic species-abundance distributions: A proposed new protocol within the European Union Water Framework Directive . Marine Pollution Bulletin , 49 : 728 – 739 .
- Rosenzweig , ML. 1995 . Species diversity in space and time , Cambridge : Cambridge University Press .
- Roy , K , Jablonski , D and Valentine , JW. 2000 . Dissecting latitudinal diversity gradients: Functional groups and clades of marine bivalves . Proceedings of the Royal Society B , 267 : 293 – 299 .
- Sanders , HL. 1968 . Marine benthic diversity: A comparative study . The American Naturalist , 102 : 243 – 282 .
- Sanvicente-Añorve , L , Leprêtre , A and Davoult , D. 2002 . Diversity of benthic macrofauna in the eastern English Channel: Comparison among and within communities . Biodiversity and Conservation , 11 : 265 – 282 .
- Scheiner , SM. 2004 . A melange of curves – further dialogue about species–area relationships . Global Ecology and Biogeography , 13 : 479 – 484 .
- Simboura , N and Zenetos , A. 2002 . Benthic indicators to use in the Ecological Quality classification of Mediterranean soft bottom marine ecosystems, including a new Biotic Index . Mediterranean Marine Science , 3 : 77 – 111 .
- Snelgrove , P , Blackburn , T , Hutchings , P , Alongi , A , Grassle , J , Hummel , H , King , G , Koike , I , Lambshead , P , Ramsing , N and Solis-Weiss , V. 1997 . The importance of marine sediment diversity in ecosystem processes . Ambio , 26 : 578 – 583 .
- Solé , RV , Manrubia , SC , Benton , M , Kauffman , S and Bak , P. 1999 . Criticality and scaling in evolutionary ecology . Trends in Ecology & Evolution , 14 : 156 – 160 .
- Storch , D , Šizling , AL and Gaston , KJ. 2003 . Geometry of the species–area relationship in central European birds: testing the mechanism . Journal of Animal Ecology , 72 : 509 – 519 .
- Tsutsumi , H , Fukunaga , S , Fujita , N and Sumida , M. 1990 . Relationship between growth of Capitella sp . and organic enrichment of the sediment. Marine Ecology Progress Series , 63 : 157 – 162 .
- Van Hoey , G , Degraer , S and Vincx , M. 2004 . Macrobenthic community structure of soft-bottom sediments at the Belgian Continental Shelf . Estuarine, Coastal and Shelf Science , 59 : 599 – 613 .
- Vanden Berghe , E , Claus , S , Appeltans , W , Faulwetter , S , Arvanitidis , C , Somerfield , PJ , Aleffi , IF , Amouroux , JM , Anisimova , N Bachelet , G . 2009 . MacroBen integrated database on benthic invertebrates of European continental shelves: A tool for large-scale analysis across Europe . Marine Ecology Progress Series , 382 : 225 – 238 .
- Wand , MR and Jones , MC. 1995 . Kernel smoothing , 1st , New York, NY : Chapman & Hall/CRC .
- Westoby , M. 1993 . “ Biodiversity in Australia compared with other continents ” . In Species diversity in ecological communities: Historical and geographical perspectives , Edited by: Ricklefs , RE and Schulter , D . 170 – 177 . Chicago, IL : University of Chicago Press .
- Williamson , MH. 1988 . “ Relationship of species number to area, distance and other variables ” . In Analytical biogeography , Edited by: Myers , AA and Giller , PS . 91 – 115 . London : Chapman & Hall .