Abstract
Postnatal growth of nestling great cormorants Phalacrocorax carbo sinensis was studied at two northeastern Mediterranean wetlands, the Lakes Kerkini and Mikri Prespa, northern Greece. A method for constructing growth curves from only two visits to bird colonies was used. Logistic growth parameters re-calculated from the logarithmic equation fitted well to the actual growth data of initial-final measurements of body mass, bill length, bill + head length, and tarsus length. Growth rates (K) did not significantly differ between colonies for all variables: body mass (0.205 and 0.206 day−1 at Lakes Kerkini and Mikri Prespa respectively), bill length (0.131 and 0.147 day−1), bill + head length (0.109 and 0.121 day−1) and tarsus length (0.201 and 0.215 day−1). Asymptotic values (A) did not significantly differ between colonies for body mass (2126.7 g and 2128.95 g) and bill length (70.57 and 68.83 mm) but did so for bill + head (147.89 and 151.51 mm) and tarsus length (75.42 and 71.31 mm). The inflection point (T) was similar for body mass (16.6 and 16.4 days) and tarsus length (7.9 and 7.1 days) but significantly different for bill (13 and 11.2 days) and bill + head length (12.2 and 11.1 days). Climatic conditions and food availability were not considered as factors greatly affecting the observed intercolony variation, and therefore other proximate and ultimate factors should be further examined. The proposed method is very helpful because growth curves can be constructed with the allocation of the smallest amount of time and effort, while at the same time keeping the disturbance of vulnerable breeding avian populations to the minimum level. Furthermore, and provided that other factors are controlled, this method could be used for the monitoring of the influence of spatial and temporal variation of ecological conditions on nestling growth.
Introduction
The great cormorant Phalacrocorax carbo is a top predator of aquatic ecosystems that feeds almost entirely on fish and is found in both inland and coastal waters throughout large parts of the world (Cramp & Simmons Citation1977). The subspecies P. c. sinensis is found in continental Europe and Asia, and breeds in colonies mainly located in trees near fresh, brackish or salt water. During the first half of the 20th century its status was threatened, but since the 1970s, its populations have rapidly increased throughout its European range (Debout et al. Citation1995; van Eerden & Gregersen Citation1995; Handrinos & Akriotis Citation1997; Røv et al. Citation2003; Volponi & Addis Citation2003; Liordos & Goutner Citation2008a), due mainly to legal protection by the European Community and eutrophication of water bodies that led to increase in fish productivity (van Eerden et al. Citation1995).
The large populations combined with the fish-eating habits of this bird have caused considerable conflicts with fishermen and the fisheries industry in many countries (Kirby et al. Citation1996; van Dam & Asbirk Citation1997), which consequently resulted to a large number of studies on the bird's diet, energetics, and possible impact on fish populations and economic activities (see Baccetti & Cherubini Citation1997; Cowx Citation2003; and Keller et al. Citation2003 for reviews). In contrast, other aspects of the bird's biology and ecology, such as nestling growth and development received relatively little attention. We know of three studies on the growth of wild populations of continental great cormorants (Platteeau et al. Citation1995; Goutner et al. Citation1997; Barati Citation2009) and another one of P. c. sinensis chicks kept in captivity (Shmueli et al. Citation2003).
Avian postnatal growth is a non-linear function of age (Ricklefs Citation1967, 1968, 1973). Each species has a characteristic and inherent growth pattern (Ricklefs Citation1967) and nestling growth provides valuable information on the behaviour and ecology of individuals and populations (Ricklefs Citation1968; Starck & Ricklefs Citation1998a). However, the study of growth is labour-intensive because it requires numerous data and many visits to the colony. To resolve this Ricklefs & White (Citation1975) provided a method for constructing average growth curves from only two visits to bird colonies. In doing so, time and effort could be saved and the disturbance of birds could be minimised. The analysis of growth can then be achieved by fitting different types of equation to growth data (Ricklefs Citation1967). Three equations are most commonly used to describe avian growth and are known as the logistic, Gompertz and von Bertalanffy sigmoid curves (Ricklefs Citation1968). The comparison of average growth curves can reveal interspecific patterns of reproductive strategy and intraspecific variations in the feeding conditions of nestlings (Ricklefs Citation1967).
The aims of the present study were thus to describe and compare the growth of great cormorant nestlings in two major lacustrine colonies in Greece, by fitting average curves to growth data from two successive visits.
Materials and methods
The study was conducted in two breeding colonies located in northern Greece (), the Lakes Kerkini (41ο 12′ N, 23ο 9′ E) and Mikri Prespa (40ο 44′ N, 21ο 4′ E), both designated as Wetlands of International Importance under the Ramsar Convention. Lake Kerkini is a seasonally flooded semi-artificial lake with a surface varying from 55 to 75 km2. Flooded and riverine forests of willow Salix alba x fragilis hybrids, common alder Alnus glutinosa, oriental plane Platanus orientalis, and tamarisk Tamarix parviflora occur at the northeast part of the lake, where great cormorants, 2400 pairs in 2000 (Liordos & Goutner Citation2008a) mainly nested on willows, over water, in association with 12 waterbird species (Nazirides & Papageorgiou Citation1996). Lake Mikri Prespa is part of the Prespa watershed, along with Lake Megali Prespa and the surrounding forested mountain slopes. It is situated at an altitude of 853.5 m a.s.l. extending over 47.35 km2 of which 43.5 km2 belong to Greece. Great cormorants (220 pairs in 2001; Liordos & Goutner Citation2008a) nested on Vidronissi Island, the smaller of the two occurring in the lake, on a stand of ancient juniper Juniperus foetidissima trees.
The Ricklefs & White (Citation1975) method was used to reduce disturbance at the colony. The Lake Kerkini colony was visited on 4 and 15 May 2000, while Lake Mikri Prespa on 28 April and 9 May 2001. Four body measurements of 39 nestlings from 18 broods (0.75% of total) and 30 nestlings from 11 broods (5% of total) were taken at the Lake Kerkini and Lake Mikri Prespa colonies respectively. In the first visit fresh body mass, bill length, bill + head length, and tarsus length of each nestling were measured. Body mass was measured using Pesola spring balances of 100, 500, 1000, 2500, and 5000 g to the nearest 1, 5, 10, 25, and 25 g respectively. Bill length (the upper mandible of the bill, from tip to first feathers), bill + head length together (from the tip of the bill to the back of the skull), and tarsus length (from middle of midtarsal joint to distal end of tarsometatarsus, with foot closed towards tail) were measured with digital calipers to the nearest 0.01 mm. All measurements were taken by the same person (Vasilios Liordos) to eliminate variation among investigators. All visits were made at the same time of day, from 09:00 to 12:00 am, to avoid diurnal variations in body mass. During the first visit at Lake Kerkini two “wet” nestlings were found, that could be assigned to 1-day-old status (i.e., day = 0, Cramp & Simmons Citation1977; Johnsgard Citation1993). The newly-hatched nestlings weighed 33 and 35 g respectively, and measured 9.6 and 11.6 mm bill length, 30.7 and 31.1 mm bill + head length, 12.4 and 12.4 mm tarsus length. After measurements, nestlings were individually marked with plastic colour rings placed on the tarsus, and returned to their nests. On the second visit, 11 days later, the measurements of the same nestlings were repeated. Body measurements of 11 adults collected from the Axios and Evros Deltas, northern Greece (Liordos & Goutner Citation2007, 2008b), were also used for comparison.
Data analysis followed Ricklefs & White (Citation1975), using the S–PLUS® 6.2 statistical package (Insightful Corp.) for curve-fitting. The equation best describing the initial-final measurements plot was first estimated. Then, the values of each growth variable at ages incremented by the time interval between successive nest visits (11 days) were estimated, by using as starting values the average growth of the two “wet” nestlings from Lake Kerkini (34 g for body mass, 10.6 mm for bill length, 30.9 mm for bill + head length, and 12.4 mm for tarsus length). The starting values were calculated from only two nestlings and for one of the studied colonies, but were used for both colonies as they fell within their range of measurements. Re-calculated growth data points that fell within the original data range were only retained. Growth parameters were then derived iteratively by fitting logistic growth curves to the growth values re-calculated from the above equation (Ricklefs Citation1967, 1968, 1973) using the nls command in S-PLUS® 6.2 (Crawley Citation2002). Between-sites comparisons of growth parameters were made using two-tailed t tests (Motulsky & Christopoulos Citation2004). The t10–90 index, the time interval (in days) needed for growth from 10% to 90% of the equation's asymptotic value was also calculated according to Ricklefs (Citation1967).
Results
The final to initial measurements plots were best described by the logarithmic equation, for all growth variables in both colonies:
where M1 and M2 are the initial and final measurement respectively, a and b the logarithmic equation constants. The estimated values of the equation constants are given in .
Table I. Parameter values of the logarithmic equation, M2 = a + b · ln(M1), which best described the final (M2)-initial (M1) measurements plots, for each growth variable of nestling great cormorants, using measurements taken at the Lake Kerkini and Lake Mikri Prespa breeding colonies, at 11-day time intervals
Growth data at successive 11-day intervals were then calculated using Equationequation (1)(1). Growth values for five age points (up to the approximate age of 44 days) fell within the original measurements for all growth variables and were used for the estimation of growth models. Logistic growth models were then fitted to the re-calculated growth patterns for all variables at both Lake Kerkini and Lake Mikri Prespa colonies (, ):
Figure 2. Postnatal growth of four body components of great cormorant nestlings from Lake Kerkini and Lake Mikri Prespa. Composite logistic growth curves were fitted to 5-point data sets, re-calculated from the logarithmic equation, which fitted well to the actual growth data of initial-final measurements of 39 and 30 nestlings from Lakes Kerkini and Mikri Prespa respectively. Approximate age at 11-day intervals is given, by using the average values of two newly-hatched nestlings found at Lake Kerkini as starting points. Data points that fell within the range of actual measurements were only used.
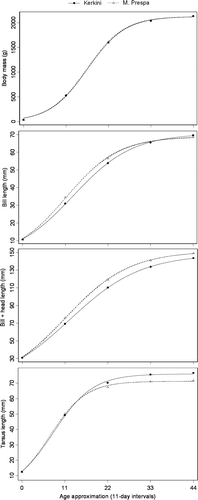
Table II. Values of the logistic equation parameters for body mass (1), bill length (2), bill + head length (3), and tarsus length (4) of nestling great cormorants, re-calculated from the logarithmic equation which fitted well to the actual growth data of initial-final measurements taken during the growth period at the Lake Kerkini and Lake Mikri Prespa breeding colonies. A is the asymptotic value of growth variables, K is the logistic growth constant (day−1), and T is the age (days) at which the inflection point occurs. Parameters are given with their standard errors (SE) and compared with two-tailed t tests. Significant differences are given in italics. R2 values of the regressions and the t10–90 time (days) required to complete logistic growth from 10 to 90% of the asymptote are also given
where GV is the growth variable, A its asymptotic value, t the nestling's age in days, K the logistic growth rate constant in day−1, which is proportional to the overall growth rate (Ricklefs Citation1968), and T the inflection point in days, which occurs when 50% of the total logistic growth has been accomplished.
The intercolony comparison of derived logistic growth parameters revealed variable patterns. Growth parameters (A, K, T) did not significantly differ between Lakes Kerkini and Mikri Prespa for body mass (). The t10–90 index was also similar in both colonies. The asymptotic value (A) and growth rate (K) were also similar for bill length in both colonies, although the inflection point (T) occurred significantly later and the t10–90 time interval needed more time to be accomplished at Lake Kerkini than Lake Mikri Prespa. In contrast, asymptotic value (A) was significantly longer at Lake Mikri Prespa than Lake Kerkini for bill + head length, whereas the other parameters (K, T, t10–90) followed the same trends as bill length. Opposing to bill + head's, asymptotic tarsus length (A) was significantly longer at Lake Kerkini than Lake Mikri Prespa, with other growth parameters (K, T, t10–90) being similar in both colonies.
Goutner et al. (Citation1997) studied the growth of great cormorant nestlings in 1994 in the Axios Delta, northern Greece, also using the same method and time interval between visits (11 days), their findings being therefore directly comparable with the present study. Pairwise comparisons showed that asymptotic values (A) were not statistically different between the Axios and the two lacustrine colonies for body mass and bill length (2105 g and 68.8 mm respectively for the Axios Delta nestlings; t4 <2.322, p> 0.081), whereas the Axios Delta nestlings had shorter bill + length and tarsus (141.5 and 67.7 mm respectively) than either the Kerkini or Mikri Prespa ones (t4 > 3.923, p < 0.017). On the other hand, growth rates (K 0.203, 0.132, 0.126, 0.222 day−1 for body mass, bill, bill + head, tarsus respectively in the Axios Delta) were not significantly different in the Axios Delta than either of the other colonies for all the growth variables (t4 < 1.861, p > 0.136). In contrast, the inflection point (T 18.6, 15.7, 13.8, 10.0 days for body mass, bill, bill + head, tarsus respectively in the Axios Delta) occurred significantly later in the Axios Delta than either of the other colonies for all the growth variables (t4 > 4.594, p < 0.010).
The mean body mass of 11 adult birds was 2529 ± 311 g (min. 1940 g, max. 3000 g), mean bill length 70.64 ± 3.28 mm (66.00−74.72 mm), mean bill + head length 152.43 ± 2.74 mm (149.00–155.00 mm), and mean tarsus length 71.66 ± 1.85 mm (68.69–74.48 mm). Adult body mass was about 460 g higher than asymptotic nestling body mass. Adult values of the bill, bill + head and tarsus were similar to asymptotic values of nestling growth.
Discussion
Great cormorants exhibit sexual dimorphism in size, with males generally being larger and heavier than females (Koffijberg & van Eerden Citation1995; Liordos & Goutner Citation2008b), so sex has a large effect on nestling growth patterns (Shmueli et al. Citation2003). However, the sex of the nestlings studied in this paper was not known. Other proximate and ultimate factors that could affect nestling growth include food supply, weather, hatching order, parental quality, hatching date and brood size, nest predation, subspeciation and hybridisation. Given these potential sources of bias, the method proposed by Ricklefs & White (Citation1975) was applied on growth data of nestling great cormorants. The study of avian growth is labour-intensive and time-consuming because many nestlings must be measured several times during the growing season. This involves many visits to the colony that can stress both nestlings and parents and, if combined with other factors (bad weather, nest predation), can cause nest abandonment and breeding failure (Zach & Mayoh Citation1986). Great cormorants nest high in trees (4–6 m) in both colonies and nestlings tend to leave the nest when scared, risking falling off the tree and dying. In addition, they nest in a mixed colony with 12 waterbird species at Lake Kerkini, including the also sensitive pygmy cormorant Phalacrocorax pygmeus and Eurasian spoonbill Platalea leucorodia, which are at a similar risk. To avoid these shortcomings, the method of Ricklefs & White (Citation1975), which requires only two visits to the breeding colony was used to describe the patterns of postnatal growth of the great cormorant. Adding to this, another significant advantage of this method is that the constructed composite growth curves can be used as biological indicators of the environment by revealing spatio-temporal variation in ecological conditions that influence nestling growth (Ricklefs & White Citation1975). The conditions that animals face during their growth period can affect survival and later reproductive success (Lindström Citation1999) and therefore the comparison of avian growth models is useful for revealing intraspecific variation in the inherent growth pattern (Ricklefs Citation1973).
Ricklefs & White (Citation1975) used measurements from 25 nestlings to describe their method. In this study their methodology was followed by taking measurements of 39 and 30 nestlings from Lakes Kerkini and Mikri Prespa respectively. The measurements were taken when nestlings of different age/developmental stages were available in the colonies so that a large part of the growth period could be studied. In fact, subsequent analysis allowed for the description of approximately the 44 first days of nestling age, the greatest part of the fledging period (c. 50 days, Cramp & Simmons Citation1977). In addition, the average growth values of two newly-hatched chicks, which fell within the range of measurements of both colonies, were taken as starting points for the calculation of the 11-day intervals, thus allowing for the comparison between the subsequently re-calculated growth patterns, while at the same time representing a reasonable approximation of nestling age. The logistic growth model fitted well with the growth pattern re-calculated from the logarithmic equation (R2 between 0.96 and 0.99). The growth parameter estimates can be useful indicators of the growth patterns, if the logarithmic function has a good fit for the initial-final measurements relationship. Indeed, the logarithmic equation fitted well to the actual growth data of initial-final measurements, with R2 ranging from 0.87 (tarsus length) to 0.97 (body mass). The comparison of the calculated nestling growth models between the studied Kerkini and Mikri Prespa and also the Axios (Goutner et al. Citation1997) colonies, revealed several differences and similarities, with the most important being: a) logistic growth rates did not significantly differ between all three colonies for all growth variables; b) asymptotic length was significantly different for bill + head and tarsus between all three colonies; and c) the inflection point occurred significantly later in the Axios than at both the Kerkini and Mikri Prespa colonies for all the growth variables.
During their period of growth, nestlings may encounter unfavourable environmental conditions that cause phenotypic changes from the normal ontogenetic development given by their genotype. Such phenotypic changes, arising from variation in food availability or other environmental conditions, are known as developmental plasticity (Schew & Ricklefs Citation1998; Moe et al. Citation2004). In a comparative study of altricial birds, Saether (Citation1994) showed that nestling growth significantly correlated with the provisioning rate of the parents (after adjusting for body size). Food availability seems to be the most important environmental factor for postnatal growth (Martin Citation1987) and also most other environmental causes of growth variation seem somehow related to food supply (Gebhardt-Henrich & Richner Citation1998). On the other hand, Moe et al. (Citation2004) showed that overall structural growth was very well conserved even during food restriction in nestling European shags Phalacrocorax aristotelis and they argued that this could have been shaped by sibling competition. Structural size is therefore expected to vary less between years and areas (with varying food availability) than body mass. The high increase of fish prey abundance and availability in the great cormorant's fishing grounds has been identified as the major factor responsible for the bird's high rate of population increase in Greece during the last 20 years (Liordos & Goutner Citation2008a). Therefore, food supply could not be considered as a restricting factor of growth in the studied colonies, especially of structural variables such as bill + head and tarsus length. Adverse weather conditions have been found to affect the foraging success of the parents in the European shag, thus exposing nestlings to variable food provisioning during early development (Velando et al. Citation1999). Adverse weather also increases the need for brooding at the expense of foraging (Beintema & Visser Citation1989). During the course of this study, and also when studying the reproductive performance of great cormorants at the Kerkini, Mikri Prespa and Axios colonies in 2001 and 2002, neither extreme weather events nor differences in climatic conditions between the colonies were reported (pers. obs.; NOA Citation2011).
When the possibility that the observed differences in growth were caused by differences in environmental conditions can be excluded, other factors such as hatching order, brood size, sex-specific growth, subspeciation and hybridisation should be examined. Léger & McNeil (Citation1987) reported that nestling double-crested cormorants Phalacrocorax auritus were fed the same amount of food and their growth rates and final weights did not seem to vary as a function of hatching order or brood size. Kalmbach & Becker (Citation2005) found that the growth rates of nestling neotropic cormorants Phalacrocorax brasilianus did not vary with hatching position, irrespective of brood size, except in four-chick broods where last-hatched chicks grew slower and showed a higher pre-fledging mortality. The effects of these factors on nestling growth are exacerbated during periods of low food availability, when parents cannot provide enough food for all chicks (Gebhardt-Henrich & Richner Citation1998), which did not seem to be the case in the studied colonies. Velando et al. (Citation2000) found that the wing, tarsus and body mass asymptotes were larger in male European shags, but females had a higher growth rate. Asymptotic head length was also larger in males, contrasting to the similar asymptotes in bill length between the sexes. As referred to earlier, male great cormorants are also generally larger and heavier than females (Liordos & Goutner Citation2008b) and the observed differences in the asymptotic bill + head and tarsus lengths between the colonies could be partly explained by possible differences in the sex ratio of each sample. Subspecific differentiation also occurs, with the continental P. c. sinensis being smaller than the Atlantic coast P. c. carbo (Newson et al. Citation2004). The two subspecies of the great cormorant have been found to coexist and hybridise in England (Kirby et al. Citation1995; Goostrey et al. Citation1997; Winney et al. Citation2001). The smaller P. c. sinensis is regularly occurring in Greece, however the incidence of P. c. carbo and their potential hybridisation have not been established. All in all, avian growth is a complex phenomenon determined by many factors and by their interactions and present findings do not allow for drawing additional conclusions on how they affected the growth of nestling great cormorants. Further investigations are therefore required to resolve these issues, through research integrating the study of physiological, ecological and genetic aspects of growth.
In a study of nestling great cormorants in the Barents Sea, Belopol'skii (1957 in Ricklefs Citation1973) calculated a Gompertz growth rate of 0.078 day−1 for body mass. This corresponds to a logistic growth rate of 0.115 day−1 (Starck & Ricklefs Citation1998b), lower than the Greek populations' values. Higher energetic demands for thermoregulation not met by food provisioning rates in the cold arctic Barents Sea, compared to the temperate Mediterranean colonies, may explain the differences in nestling growth.
Structural size variables displayed different growth patterns. Tarsus grew faster and completed growth earlier than bill at both Lakes Kerkini and Mikri Prespa (this study), and in the Axios Delta (Goutner et al. Citation1997) for the great cormorant and in New England for the double-crested cormorant (Dunn Citation1975). Great cormorant nestlings beg for food by waving their bill and throat, then inserting their head into their parent's mouth to eat (Cramp & Simmons Citation1977; Olver & Kuyper Citation1978; Johnsgard Citation1993). Nestlings therefore may not benefit from an early bill growth, but a fast growing tarsus may confer competitive advantage in the struggle to reach parental mouth before siblings. The lower growth rates of bill + head than bill, also observed in all the areas, may be probably due to the fact that the skull is growing slower than the bill, thus affecting the composite bill + head growth (Ricklefs Citation1973; Ricklefs et al. Citation1998).
Asymptotic lengths of the bill, bill + head and tarsus were similar to the average adult lengths. On the other hand, asymptotic body mass of nestlings was only 84% of adult body mass. The same trend has been observed for other members of the family Phalacrocoracidae, such as the double-crested cormorant (Dunn Citation1975; Léger & McNeil Citation1987) and the European shag (Østnes et al. Citation2001). This contrasts other altricial and semialtricial birds, which normally achieve higher body mass than adults before fledging (Ricklefs Citation1973).
Growth rates of Greek great cormorant nestlings are similar to those of the double-crested cormorant and higher than those of the European shag. Growth rates of body mass have been found 0.191 day−1 (Léger & McNeil Citation1987), 0.196 day−1 (Palmers 1962 in Ricklefs Citation1968, 1973), 0.208 day−1 (Dunn Citation1975), 0.235 day−1 (Cleary Citation1977) for the double-crested cormorant, and 0.172 day−1 (Østnes et al. Citation2001), 0.147--0.190 day−1 (Starck & Ricklefs Citation1998b) for the European shag. Members of the family Phalacrocoracidae usually have higher growth rates when compared to other altricial seabirds of comparable size. Members of the family Fregatidae (A = 854--1455 g) have logistic growth rates from 0.039--0.051 day−1, and of the family Sulidae (A = 800--4080 g) from 0.052--0.138 day−1 (Starck & Ricklefs Citation1998b).
This study presented the postnatal growth of the great cormorant in two Greek colonies. The method proposed by Ricklefs & White (Citation1975) for constructing average growth curves from only two visits was used. This method has considerable advantages such as: it can be applied fast and with little effort, the disturbance to birds is kept at a minimum, and spatial and temporal comparisons of growth patterns can be made. Results revealed similarities in nestling growth rates between the colonies for all the growth variables, as well as significant variations, especially in the asymptotic values of structural variables such as bill + head and tarsus length. The need for further examination of the effects of proximate and ultimate factors on nestling growth has also been highlighted. In doing so, this method could be useful for the monitoring of the influence of spatial and temporal variation of ecological conditions on nestling growth.
Acknowledgements
We are very grateful to P. Chatzigiannidis, P. Menounos, T. Nazirides, and P. Vafeidis for their assistance during fieldwork. We also thank two anonymous reviewers whose useful comments and suggestions helped greatly improve the manuscript.
References
- Baccetti , N and Cherubini , G , eds. 1997 . “ Proceedings IV European Conference on Cormorants, Bologna, Italy ” . In Supplemento alle Ricerche di Biologia della Selvaggina Vol. XXVI , 1 – 594 .
- Barati , A. 2009 . Diet and growth of chicks of the great cormorant, Phalacrocorax carbo, at Ramsar, northern Iran . Zoology in the Middle East , 46 : 29 – 36 .
- Beintema , AG and Visser , GH. 1989 . The effects of weather on time budgets and development of chicks of meadow birds . Ardea , 77 : 181 – 192 .
- Cleary , L. 1977 . “ Succès de reproduction du Cormoran a aigrettes, Phalacrocorax auritus, a. sur 3 îles du St-Laurent, en 1975 et 1976 ” . In Thèse de maîtrise , Québec, Canada : Université Laval .
- Cowx , IG , ed. 2003 . Interactions between fish and birds: Implications for management , Oxford : Fishing News Books, Blackwell Science Ltd .
- Cramp , S and Simmons , KEL , eds. 1977 . “ Handbook of the Birds of Europe, the Middle East and North Africa ” . In The Birds of the Western Palearctic , Vol. 1 , Oxford : Oxford University Press .
- Crawley , MJ. 2002 . Statistical computing: An introduction to data analysis using S-Plus , Chichester : John Wiley & Sons Ltd .
- Debout , G , Røv , N and Sellers , RM. 1995 . Status and population development of Cormorants Phalacrocorax carbo carbo breeding on the Atlantic coast of Europe . Ardea , 83 : 47 – 59 .
- Dunn , EH. 1975 . Growth, body components and energy content of nestling double-crested cormorants . Condor , 77 : 431 – 438 .
- Gebhardt-Henrich , S and Richner , H. 1998 . “ Causes of growth variation and its consequences for fitness ” . In Avian growth and development: evolution within the altricial-precocial spectrum , Edited by: Starck , JM and Ricklefs , RE . 324 – 339 . Oxford : Oxford University Press .
- Goostrey , A , Carss , DN , Noble , LR and Piertney , SB. 1997 . Population introgression and differentiation in the great cormorant Phalacrocorax carbo in Europe . Molecular Ecology , 7 : 329 – 338 .
- Goutner , V , Papakostas , G and Economidis , PS. 1997 . Diet and growth of great cormorant (Phalacrocorax carbo) nestlings in a Mediterranean estuarine environment (Axios Delta, Greece) . Israel Journal of Zoology , 43 : 133 – 148 .
- Handrinos , G and Akriotis , T. 1997 . The Birds of Greece , London : Christopher Helm (Publishers) .
- Johnsgard , PA. 1993 . Cormorants, Darters, and Pelicans of the world , Washington : Smithsonian Institution Press .
- Kalmbach , E and Becker , PH. 2005 . Growth and survival of neotropic cormorant (Phalacrocorax brasilianus) chicks in relation to hatching order and brood size . Journal of Ornithology , 146 : 91 – 98 .
- Keller , TM , Carss , DN , Helbig , AJ and Flade , M . 2003 . “ Cormorants: Ecology and Management ” . In Proceedings of the 5th International Conference on Cormorants Edited by: Keller , TM , Carss , DN , Helbig , AJ and Flade , M . 1 – 402 . Vogelwelt 124 (Supplement)
- Kirby , JS , Gilburn , AS and Sellers , RM. 1995 . Status, distribution and habitat use by Cormorants Phalacrocorax carbo wintering in Britain . Ardea , 83 : 93 – 102 .
- Kirby , JS , Holmes , JS and Sellers , RM. 1996 . Cormorants Phalacrocorax carbo as fish predators: an appraisal of their conservation and management in Great Britain . Biological Conservation , 75 : 191 – 199 .
- Koffijberg , K and van Eerden , MR. 1995 . Sexual dimorphism in the cormorant Phalacrocorax carbo sinensis: possible implications for differences in structural size . Ardea , 83 : 37 – 46 .
- Léger , C and McNeil , R. 1987 . Brood size and chick position as factors influencing feeding frequency, growth, and survival of nestling Double-crested Cormorants, Phalacrocorax auritus . Canadian Field Naturalist , 101 : 351 – 361 .
- Lindström , J. 1999 . Early development and fitness in birds and mammals . TREE , 14 : 343 – 348 .
- Liordos , V and Goutner , V. 2007 . Spatial patterns of winter diet of the great cormorant in coastal wetlands of Greece . Waterbirds , 30 : 103 – 111 .
- Liordos , V and Goutner , V. 2008a . Reproductive performance of the great cormorant (Phalacrocorax carbo sinensis) in three Greek colonies . Journal of Natural History , 42 : 317 – 331 .
- Liordos , V and Goutner , V. 2008b . Sex determination of great cormorants (Phalacrocorax carbo sinensis) using morphometric measurements . Waterbirds , 31 : 203 – 210 .
- Martin , TE. 1987 . Food as a limit on breeding birds: A life-history perspective . Annual Review of Ecology and Systematics , 18 : 453 – 487 .
- Moe , B , Brunvoll , S , Mork , D , Brobakk , TE and Bech , C. 2004 . Developmental plasticity of physiology and morphology in diet-restricted European shag nestlings (Phalacrocorax aristotelis) . Journal of Experimental Biology , 207 : 4067 – 4076 .
- Motulsky , H and Christopoulos , A. 2004 . Fitting models to biological data using linear and nonlinear regression , Oxford : Oxford University Press .
- Nazirides , T and Papageorgiou , N. 1996 . “ The breeding biology of pygmy cormorant (Phalacrocorax pygmeus), a vulnerable bird species, at Lake Kerkini, northern Greece ” . In Colonial Waterbirds 19 (Special Publication 1) 219 – 223 .
- Newson , SE , Hughes , B , Russell , IC , Ekins , GR and Sellers , RM. 2004 . Subspecific differentiation and distribution of great cormorants Phalacrocorax carbo in Europe . Ardea , 92 : 3 – 10 .
- NOA . 2011 . Meteodata search . National Observatory of Athens. Available: penteli.meteo.gr/meteosearch. Accessed Nov , 2011 : 20
- Olver , MD and Kuyper , MA. 1978 . Breeding biology of the white-breasted cormorant in Natal . Ostrich , 49 : 25 – 30 .
- Østnes , JE , Jenssen , BM and Bech , C. 2001 . Growth and development of homeothermy in nestling European Shags (Phalacrocorax aristotelis) . Auk , 118 : 983 – 995 .
- Platteeau , M , Koffijberg , K and Dubbeldam , W. 1995 . Growth of cormorant Phalacrocorax carbo sinensis chicks in relation to brood size, age ranking and parental fishing effort . Ardea , 83 : 235 – 245 .
- Ricklefs , RE. 1967 . A graphical method of fitting equations to growth curves . Ecology , 48 : 978 – 983 .
- Ricklefs , RE. 1968 . Patterns of growth in birds . Ibis , 110 : 419 – 451 .
- Ricklefs , RE. 1973 . Patterns of growth in birds . II. Growth rate and mode of development. Ibis , 115 : 177 – 201 .
- Ricklefs , RE , Starck , JM and Konarzewski , M. 1998 . “ Internal constraints on growth in birds ” . In Avian growth and development: evolution within the altricial-precocial spectrum , Edited by: Starck , JM and Ricklefs , RE . 266 – 287 . Oxford : Oxford University Press .
- Ricklefs , RE and White , E. 1975 . A method for constructing nestling growth curves from brief visits to seabird colonies . Bird Banding , 46 : 135 – 140 .
- Røv , N , Lorentsen , SH and Nygård . 2003 . Status and trends in the Great Cormorant Phalacrocorax carbo carbo populations in Norway and the Barents Sea Region 71 – 75 . Vogelwelt 124 (Supplement)
- Saether , BE. 1994 . Food provisioning in relation to reproductive strategy in altricial birds: A comparison of two hypotheses . Evolution , 48 : 1397 – 1406 .
- Schew , WA and Ricklefs , RE. 1998 . “ Developmental plasticity ” . In Avian growth and development: evolution within the altricial-precocial spectrum , Edited by: Starck , JM and Ricklefs , RE . 288 – 304 . Oxford : Oxford University Press .
- Shmueli , M , Arad , Z , Katzir , G and Izhaki , I. 2003 . Developmental rates and morphometrics of the sympatric pygmy cormorant (Phalacrocorax pygmeus) and great cormorant (Phalacrocorax carbo sinensis) . Israel Journal of Zoology , 49 : 159 – 173 .
- Starck , JM and Ricklefs , RE. 1998a . “ Patterns of development: the altricial-precocial spectrum ” . In Avian growth and development: evolution within the altricial-precocial spectrum , Edited by: Starck , JM and Ricklefs , RE . 3 – 30 . Oxford : Oxford University Press .
- Starck , JM and Ricklefs , RE. 1998b . “ Data set of avian growth parameters ” . In Avian growth and development: evolution within the altricial-precocial spectrum , Edited by: Starck , JM and Ricklefs , RE . 381 – 415 . Oxford : Oxford University Press .
- van Dam , C and Asbirk , S. 1997 . “ Cormorants and Human Interests. Proceedings of the Workshop towards an International Conservation and Management Plan for the Great Cormorant (Phalacrocorax carbo) ” . In 3–4 October 1996. Lelystad , The Netherlands : Wageningen .
- van Eerden , MR and Gregersen , J. 1995 . Long-term changes in the northwest European population of Cormorants Phalacrocorax carbo sinensis . Ardea , 83 : 61 – 79 .
- van Eerden , MR , Koffijberg , K and Platteeuw , M. 1995 . Riding on the crest of the wave: possibilities and limitations for a thriving population of migratory Cormorants Phalacrocorax carbo in man-dominated wetlands . Ardea , 83 : 1 – 9 .
- Velando , A , Graves , J and Freire , J. 2000 . Sex-specific growth in the European shag Stictocarbo aristotelis, a sexually dimorphic species . Ardea , 88 : 127 – 136 .
- Velando , A , Ortega-Ruano , JE and Freire , J. 1999 . Chick mortality in European shag Stictocarbo aristotelis related to food limitations during adverse weather events . Ardea , 87 : 51 – 59 .
- Volponi , S and Addis , P. 2003 . Great Cormorants Phalacrocorax carbo sinensis in two key Italian wintering areas 93 – 98 . Vogelwelt 124 (Supplement)
- Winney , BJ , Litton , CD , Parkin , DT and Feare , CJ. 2001 . The subspecific origin of the inland breeding colonies of the Cormorant Phalacrocorax carbo in Britain . Heredity , 86 : 45 – 53 .
- Zach , RY and Mayoh , KR. 1986 . Weight and feather growth of nestling Tree Swallows . Canadian Journal of Zoology , 60 : 1080 – 1090 .