Abstract
The morphological study of the vertebral column of Carangoides caeruleopinnatus (Rüppell, 1830) enabled the division of this bony structure into four morphologically distinct regions, and the drawing of characteristic-looking vertebral profiles. This regionalization is more complicated than the classical division in truncal and caudal parts only. These morphological descriptive parameters express a morphotype that may be linked with the carangiform mode of swimming of the onion trevally.
Introduction
Ward and Brainerd (Citation2007) suggested that vertebrates show variations in the degree of regionalization of their vertebral column. Kubo and Asano (Citation1987, Citation1990) and Desse et al. (Citation1989) stated that such differences in the morphology of vertebrae of different regions of the vertebral column can be revealed by biometrical studies. In general, and in the actinopterygian fishes, there are two well-defined regions in the vertebral column, the pre-anal abdominal region and post-anal caudal region (Grande & Bemis Citation1998), but within these two regions, diversity in vertebral form is present (Ford Citation1937; Pietsch Citation1978; Grande & Bemis Citation1998; Bemis & Forey Citation2001; Ward & Brainerd Citation2007). The abdominal region may include, from anterior to posterior, occipital vertebrae that are incorporated into the skull through ontogeny, anterior vertebrae that are highly modified (e.g. Weberian apparatus in Ostariphysi, and fused vertebrae in Syngnathoidei), and vertebrae that generally bear abdominal ribs. The caudal region includes vertebrae that bear haemal spines, and ural vertebrae that bear hypurals. This regional pattern of vertebral structure is probably linked to, and could thus express, the locomotory functions of the vertebral column (Ramzu et al. Citation1992).
The vertebral column plays a very important mechanical role in fish locomotion (Laerm Citation1976; Lindsey Citation1978; Weihs Citation1989). During the developmental stages, this structure is subjected to different types of biological strains, which seem to be expressed by local and specific morphological peculiarities (Kubo & Asano Citation1987, Citation1990; Desse et al. Citation1989). It is noteworthy to study the morphology of the vertebral column and to make links between this morphology and locomotion. This is due to the strong anatomical and functional relationship with the trunco-caudal musculature (Le Danois Citation1958; Lindsey Citation1978; Vronskii & Nikolaitchouk Citation1989).
The aim of the present work is to study the biometry of the vertebral column of the onion trevally, C. caeruleopinnatus, collected from the Sea of Oman, as there is no such information is available in the literature for this species.
Materials and methods
Fishes of C. caeruleopinnatus (52 specimens) ranging in total length (TL) from 202 to 313 mm were collected during an ichthyological survey from the vicinity of Muscat City, Sea of Oman on 23 February 2011. The method of James (Citation2008) was used to prepare dry vertebral column. The fish specimens were boiled to 110°C to strip the flesh off the bone, and running water was used to brush the bones after boiling. After drying, the vertebrae were separated and numbered, then measured with a digital 1/100 caliper (Ted Pella, Inc., Redding, USA). The dry vertebrae were deposited in the ichthyological collection of the Marine Science and Fisheries Centre, Ministry of Agriculture and Fisheries, Oman, catalogue no. 1328.
Three measurements of the vertebral centrum were selected (). The first is vertebral length (VL), the distance along the left mid-ventral line. It is considered among the factors controlling the degree of the body’s flexion (Ramzu et al. Citation1992). The other two measurements were vertebral height (VH), the maximum vertical distance of the anterior side of the vertebra, and vertebral width (VW), the maximum horizontal length across the anterior surface of the vertebra (). To avoid individual variation and to facilitate future comparisons with other samples – even other species – each vertebral measurement was converted into a vertebral index Vi (Ramzu & Meunier Citation1999):
Figure 1. Hypothetical vertebra showing the three measurements of the vertebral centrum. VL, central length; VH, central height; VW, central width.
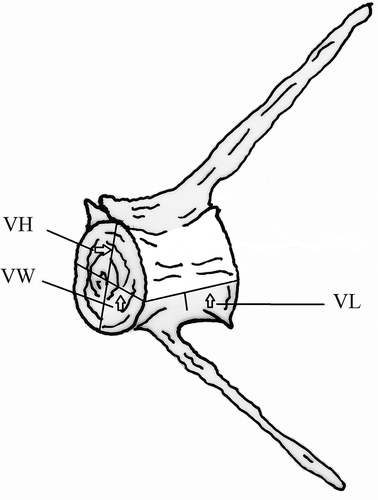
The numbers of precaudal and caudal vertebrae were counted and the mean value was calculated for each vertebra, then species means were calculated for abdominal vertebral number (AVN) and caudal vertebral number (CVN). The thoracic vertebrae were defined as those that were cranial to vertebrae with separated haemal arches. The caudal region was defined as the region from the first fused haemal arch posterior to the last centrum including the ural centrum. The mean vertebral aspect ratio (AR = centrum height/centrum width) for each region was calculated for each individual. The means were then calculated for abdominal aspect ratio (AAR) and caudal aspect ratio (CAR). Osteological terminology mainly follows Ramzu and Meunier (Citation1999) and Nowroozi et al. (Citation2012).
Results
There are 24 vertebrae in the vertebral column of C. caeruleopinnatus in all the specimens examined of this species. Each vertebra, in general appearance, looks like a cylinder, with each vertebra comprising an amphicoelous (hourglass shape) centrum, whose chordal cavities were connected by a thin hole. Outside these chordal cavities, no bony deposit masks this structure ().
It is possible to divide the vertebral column of the species in question into two main regions: an anterior region made of precaudal vertebrae without haemal spine, and a posterior region with caudal vertebrae where the two haemal arches are joined and prolonged by a haemal spine. The anterior region is divided into two regions, the postcranial (V1–V3) and the middle region (V4–V10). Similarly, the posterior region is divided into the caudal region (V11–V19) and the ural region (V20–V24). Vertebra no. 10 marks the border between the abdominal and caudal vertebrae. The 10 anterior vertebrae, or precaudal vertebrae (V1–V10), define the abdominal or truncal region, delimited by the presence of the abdominal cavity. The next set of vertebrae, the caudal vertebrae (V11–V24), belong to the tail; their haemal arches are fused from the 11th vertebra and prolonged by a haemal spine. This latter spine showed variation in both its length and its shape. It is long and directed downward in vertebrae V11–14 and directed backward in V15–V20. It reaches its maximum length at the vertebrae V14–V15, after which it starts to decrease in length and reaches its minimum length at vertebra number 23. Starting from V19–V24, the haemal arch and spine get smaller and directed backward. The haemal arch and spine of V21–V23 run parallel to the long axis of the centrum. In the five most posterior vertebrae (V26–V30), the haemal spine is short, lies parallel to the axis of the vertebral column and is directed posteriorly. The anterior surface of the haemal arch is smooth and a concavity is present on its anterior side. There was no intraspecific variation in the shape of the vertebrae.
The vertebral column of C. caeruleopinnatus showed characteristic regionalization (; ). The anteriormost three vertebrae appear different from the remaining precaudal vertebrae. The first vertebra is small and fits into the anterior part of the second vertebra. There are no anterior zygapophyses in the first vertebra as this vertebra supports the skull; instead, it has two facets for the skull to rest on. The lateral processes of vertebrae 1–3 are well developed and lie parallel to the axis of the vertebral column. The development of the lateral paraphysis decreases posteriorly. Six vertebrae (V5–V10) following the first three can be considered transitional vertebrae that form a connection between the abovementioned first three vertebrae and the trunk, because they increase or decrease regularly in their biometrical parameters (). The middle region (V4–V10) appears to be made up of one morphological entity. Here, the length of the trunk vertebrae increases until a maximum is attained around the 19th vertebra. V1–V10 bear ribs that extend on the sides of the fish body. The ural region includes vertebrae V20–V24. It corresponds to the caudal peduncle and is characterized by a fall in the vertebral length, height and width.
Table I. Mean values (M; mm) of length (LV), height (LH) and anterior width (LW) for the successive vertebrae of the vertebral column of Carangoides caeruleopinnatus (SD = standard deviation).
Figure 3. Vertebral profiles of Carangoides caeruleopinnatus. VL, central length; VH, central height; VW, central width.
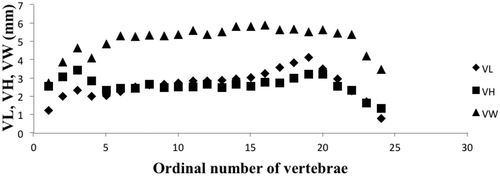
The profiles corresponding to the three parameters measured on all of the vertebrae were the same in of all the specimens studied, indicating a similar trend. The morphometric analysis shows that the vertebral axis of the onion trevally, C. caeruleopinnatus, has complex divisions. Four regions can be characterized along the vertebral axis, according to the changes of the three parameters measured from one vertebra to another.
The vertebral profile given by the variation of the vertebral length along the axis shows one maximum at V19 and two minima at V1 and V24. The value of vertebral length increases steadily from V1–V3, then decreases at V4. A trend of increasing starts at V5 and ends at V19. A sharp decrease occurs beyond V19 before reaching the lowest value at V24.
The vertebral profile revealed by the vertebral height shows two maxima at V3 and V20, and two minima at V5 and V24. There is a significant increase between V1 and V3 followed by a clear decrease between V3 and V5, and then the height value shows a slight trend of increasing between V5 and V20. A dramatic decrease is present beyond V20 before reaching a minimum value at V24.
There are two maxima, one at V16 and the other at V22, and three minima at V1, V4 and V24. The width profile shows a sharp increase between V1 and V3, and the value decreases at V4. A slight increase is evident between V5 and V22, and then a dramatic decrease occurs beyond V23.
There are 10 abdominal and 14 caudal vertebrae in the vertebral column of C. caeruleopinnatus. The vertebral aspect ratio for the abdominal and caudal regions is 0.47 and 0.52, respectively.
Discussion
The analysis of the variation of the length, height and width of vertebrae along the vertebral column of C. caeruleopinnatus shows that its structure is more complex than a simple division into two areas, precaudal and caudal. This biometric study suggests the division of the vertebral column into four regions: (1) a postcranial region, (2) a middle region, (3) a caudal region and (4) a ural region. Regions 1 and 4 are characterized by strong variation in vertebral parameters; in regions 2 and 3, these variations are more regular and characterized by a cline on both side of the maximum value of the length, height and width of vertebrae.
The post-cranial region, immediately at the back of the head, ensures the articulation with the skull. The first three vertebrae, V1–V3, form a morphological set with mainly specific parameters of vertebral length, height and width. However, these three vertebrae do not show completely different morphological characteristics compared to the other vertebrae (except for the first one). For C. caeruleopinnatus, the first vertebra has an anterior ventral concavity, which is articulated with the basioccipital. This first post-cephalic vertebra is designed to articulate with the posterior region of the skull, forming, with the next vertebra, a link between the two main elements of the axial skeleton, which is a function that requires some morphological specificity (Videler Citation1993). The four to six vertebrae beyond V1–V3 could be considered transitional vertebrae because they show an increase in the vertebral biometric parameters (Ramzu & Meunier Citation1999).
The middle regions include the limit between the precaudal and caudal regions (V4–V10) that corresponds to the haemal arch closing. It is therefore composed of truncal vertebrae and forms a morphological unit. In this region, the increase is regular until a maximum value before decreasing progressively.
The ural region starts with the 20th vertebra. It corresponds to the tail and is characterized by a decrease in the three values of the analyzed parameters. The ural vertebra has a different anatomy as it lacks real haemal arches, but it has hypural elements that support the lepidotrichia of the caudal fin.
As in other teleost fishes (Ramzu et al. Citation1992), the substitution of classical anatomical precaudal and caudal regions by more than two regions is probably linked to the mechanical constraints of swimming. This is the case for the species in question, C. caeruleopinnatus. Moreover, the anterior–posterior development of the three morphological parameters studied with the sudden variations of the postcephalic and ural regions on the one hand, and the maximum of the middle-caudal region on the other, favors this hypothesis (Ramzu et al. Citation1992; Ramzu Citation1994).
In the carangiform mode of swimming that the onion trevally follows (Breder Citation1926; Lindsey Citation1978; Webb Citation1978), the vast majority of movement is concentrated in the very rear of the body and tail, and fishes are shown to swim with rapidly oscillating tails. The maximum length of the vertebra (VL) occurs around the vertebrae V14–V16; this may be due to the structural response of these vertebrae to the local presence of maximal mechanical constraints.
Regarding the fourth region of the vertebral column, its specific parametrical variation might express the major role performed by the caudal vertebrae in the motor process of swimming. The caudal skeleton responds to the alternate contractions of the intrinsic muscles on the lateral sides of this region; thus, a torsion of the caudal peduncle is created, when the muscles slightly increase or decrease the surface area of the caudal fin at different phases of one beat (Bainbridge Citation1963), thereby creating a support on water.
The first haemal process of this species agrees in its morphology with those of the members of other genera of the family Carangidae described by Starks (Citation1911), being single pointed and much longer and straighter. In having this shape, it differs from those of the members of the genera Seriola and Naucrates (Starks Citation1911). Among the other characters of the vertebral column of C. caeruleopinnatus that appear similar to those of the other carangid species studied by Starks (Citation1911) are that the zygapophysis is small and the first two vertebrae bear epipleurals.
Suzuki (Citation1962) put a key to the genera of the family Carangidae. According to this key, the genus Carangoides can be separated from the remaining genera in having a haemal spine without a disc-like enlargement on anterior margin. This character is shown in the vertebral column of C. caeruleopinnatus.
The vertebral column of C. caeruleopinnatus differs from that of Parastromateus niger described by Hilton et al. (Citation2010), in having the neural spines of the first two vertebrae directed upward (they incline anteriorly in P. niger), while the anteriormost haemal spine is straight and directed downward (slightly curved anteriorly in P. niger), but it shared the following characters: the neural spines of the remaining vertebrae are elongate, nearly vertical and posteriorly inclined in the caudal region; haemal spines are well developed with the posterior haemal spines directed posteriorly; the first two vertebrae have rudimentary parapophyses which get longer in the remaining vertebrae beginning at the third vertebra; skeletal support of the caudal fin, which is similar to the other caranginid members (Hollister Citation1941; Monod Citation1968; Fujita Citation1990); fusion of the urostylar centrum and uroneural; presence of three hypural elements; and presence of two epurals. In terms of the number of epurals in this species, there are two explanations for the disappearance of the second epural: first, it ossifies and fuses to ep1 (epural 1) as in, for example, Selene vomer (Hilton et al. Citation2010) or, second, it atrophies and eventually disappears as in Caranx crysos (Hilton & Johnson Citation2007). The presence of two epurals in C. caeruleopinnatus is a case similar to that found in both P. niger (Hilton et al. Citation2010) and Caranx crysos (Hilton & Johnson Citation2007).
The morphometric analysis of the vertebral column has revealed no significant difference in the variation of the vertebral length, height and width studied taken on each vertebra of C. caeruleopinnatus. Therefore, the characterization of one vertebra along the vertebral column would be sufficient if it was based on only one of these two parameters (Desse et al. Citation1989). Furthermore, the morphology of the vertebrae of males and females is similar. This result backs that of Kacem et al. (Citation1998) on the morphology of the skeleton of Salmo salar.
The regionalization in the vertebral column of the species in question could be developed through the difference in length of vertebrae in different regions of the vertebral column, which in turn is due to different mechanisms that regulate the growth of the vertebrae in each region (Fjelldal et al. Citation2005). The closeness in the value of the aspect ratio of both abdominal and caudal regions obtained in this study may indicate that changes in the vertebral length in abdominal and caudal regions are closely linked (Ward & Brainerd Citation2007).
Acknowledgements
I would like to thank the Ministry of Fisheries Wealth, the Agriculture and Fisheries Development Fund and the Marine Science and Fisheries Centre for providing the appropriate financial support.
References
- Bainbridge R. 1963. Caudal fin and body movement in the propulsion of some fish. Journal of Experimental Biology 40:23–56.
- Bemis WE, Forey PL. 2001. Occipital structure and the posterior limits of the skull in actinopterygians. In: Ahlberg P, editor. Major events in vertebrate evolution. London: Taylor & Francis. pp. 350–369.
- Breder CM. 1926. The locomotion of fishes. Zoologica 4:159–256.
- Desse J, Desse-Bersrt N, Rochteau M. 1989. Les profils rachidiens globaux. Reconstitution de lataille des poisons et appréciation du nombre minimal d’individus à partir des pieces rachidiennes. Review of Paléobiology 8:89–94.
- Fjelldal PG, Nordgarden U, Berg A, Grotmol S, Totland GK, Wargelius A, Hansen T. 2005. Vertebrae of the trunk and tail display different growth rates in response to photoperiod in Atlantic salmon, Salmo salar L., post-smolts. Aquaculture 250:516–524. doi:10.1016/j.aquaculture.2005.04.056.
- Ford E. 1937. Vertebral variation in teleostean fishes. Journal of the Marine Biological Association of the United Kingdom 22:1–60. doi:10.1017/S0025315400011863.
- Fujita K. 1990. The Caudal Skeleton of Teleostean Fishes. Tokyo: Tokai University Press.
- Grande L, Bemis WE. 1998. A comprehensive phylogenetic study of amiid fishes (Amiidae) based on comparative skeletal anatomy. An empirical search for interconnected patterns of natural history. Journal of Vertebrate Paleontology 18:1–696. doi:10.1080/02724634.1998.10011114.
- Hilton EJ, Johnson GD. 2007. When two equals three: Developmental osteology and homology of the caudal skeleton in carangid fishes (Perciformes: Carangidae). Evolution & Development 9:178–189. doi:10.1111/j.1525-142X.2007.00148.x.
- Hilton EJ, Johnson GD, Smith-Vaniz WF. 2010. Osteology and systematics of Parastromateus niger (Perciformes: Carangidae), with comments on the carangid dorsal gill-arch skeleton. Copeia 2010:312–333. doi:10.1643/CI-09-118.
- Hollister G. 1941. Caudal skeleton of Bermuda shallow water fishes. V. Order Percomorphi: Carangidae. Zoologica 26:31–45.
- James PSBR. 2008. Osteo-taxonomic distinction of fishes of the family Leiognathidae. Indian Journal of Fisheries 55:305–310.
- Kacem A, Meunier FJ, Bagliniere JL. 1998. A quantitative study of morphological changes in the skeleton of Salmo salar during its anadromous migration. Journal of Fish Biology 53:1096–1109.
- Kubo Y, Asano H. 1987. Growth type of vertebral centra and the hard tissue observed by microradiography of the rainbow trout. Nippon Suisan Gakkaishi 53:1367–1372. doi:10.2331/suisan.53.1367.
- Kubo Y, Asano H. 1990. Relative growth pattern and hard tissue of vertebral centra by microradiography of Bluefin tuna, bigeye tuna and skipjack. Nippon Suisan Gakkaishi 56:1021–1027. doi:10.2331/suisan.56.1021.
- Laerm J. 1976. The development, function, and design of amphicoelous vertebrae in teleost fishes. Zoological Journal of the Linnean Society 58:237–254. doi:10.1111/j.1096-3642.1976.tb00830.x.
- Le Danois Y. 1958. Système musculaire. In: Grassé PP, editor. Traité de Zoologie. Vol. 13. Paris: Masson. pp. 783–817.
- Lindsey CC. 1978. Form, function and locomotory habits in fish. In: Hoar WS, Randall DJ, editors. Fish physiology. New York: Academic Press. pp. 1–100.
- Monod T. 1968. Le complex urophore des poisons téléostéens. Mémoires de l’institut Fondamental d’Afrique Noire 81:1–705.
- Nowroozi BN, Harper CJ, De Kegelb B, Adriaens D, Brainerd EL. 2012. Regional variation in morphology of vertebral centra and intervertebral joints in striped bass, Morone saxatilis. Journal of Morphology 273:441–452. doi:10.1002/jmor.11034.
- Pietsch TW. 1978. Evolutionary Relationships of the Sea Moths (Teleostei: Pegasidae) with a Classification of Gasterosteiform Families. Copeia 1978:517–529. doi:10.2307/1443620.
- Ramzu M. 1994. Étude de la régionalization de la colonne vertebrale en function des modes de nage chez les téléostéens, Morphologie, histologie et croissance. Thèse de doctorat. Paris, University of Paris.
- Ramzu M, Meunier F J. 1999. Descripteurs morphologiques de la zonation de la colonne vertébrale chez la truite are-en-ciel Oncorhynchus mykiss (Walbaum, 1792) (Teleostei, Salmonidae). Annals des Sciences Naturalles 3:87–97.
- Ramzu M, Meunier FJ, Schovaer D. 1992. Morphological and histological characteristics of the vertebral axis zonation in the trout (Salmo trutta L.) (Teleostei, Salmonidae): possible functional implications. Oceanis 18:85–91.
- Starks E C. 1911. The osteology and relationships of the fishes belonging to the family Carangidae. Leland Stanford Jr. University Publications, University Series 5:27–49.
- Suzuki K. 1962. Anatomical and taxonomical studies on the carangid fishes of Japan. Report of Faculty of Fisheries Prefectural University of Mie 4:43–232.
- Videler JJ. 1993. Fish swimming. London: Chapman & Hall. 260 pp.
- Vronskii AA, Nikolaitchouk LA. 1989. Morphologie fonctionnelle de la musculature locomotrice du corps des poissons. Kiev: La Pensée Scientifique. 184 pp. [In Russian]
- Ward AB, Brainerd EL. 2007. Evolution of axial patterning in elongate fishes. Biological Journal of the Linnean Society 90:97–116. doi:10.1111/j.1095-8312.2007.00714.x.
- Webb PW. 1978. Hydrodynamique et énergétique de la propulsion des poisons. Bulletin de l’Office des Recherches sur les Pêcheries du Canada 190:1–160.
- Weihs D. 1989. Design features and mechanics of axial locomotion in fish. American Zoologist 24:107–120.