Abstract
The order Coleoptera comprises species that are used as models in evolutionary studies, such as Alticinae, which features giant sex chromosomes. Thus, the aim of the present study was to investigate the differentiation and evolution of the sex chromosome in species of the Omophoita genus (Alticinae) using repetitive DNA (total C0t-1), C-banding and base-specific fluorochromes. Analyses using probes generated through C0t-1 reassociation kinetics showed markers distributed across all the chromosomes, especially the sex chromosomes. In conclusion, repetitive sequences are distributed across the sex chromosomes and autosomes, demonstrating that the heterochromatin of the species is largely composed of repetitive DNA. Cross-hybridisation among the species produced a very similar staining pattern for the probes. Thus, we conclude that the majority of the genome of the species of Omophoita is shared, showing the heterochromatin to be largely composed of repetitive DNA distributed along the sex chromosomes and autosomes.
Introduction
Omophoita belongs to the tribe Oedionychini and it is endemic in Neotropical regions (Riley et al. Citation2002). The species of this tribe have revealed certain singular chromosomal features, such as sex chromosomes of extremely large size and asynapsis during meiosis, 2n = 22, meiotic formulae 10II+X+y and karyotype with a predominance of metacentric chromosomes. The morphological variability of the chromosomes observed always involves the occurrence of metacentric and acrocentric chromosomes and seems to be a characteristic of this tribe (Smith & Virkki Citation1978; Petitpierre et al. Citation1988; Virkki Citation1988, Citation1989; Segarra & Petitpierre Citation1990; Virkki et al. Citation1991; Virkki & Santiago-Blay Citation1993). The metacentric condition has also been registered in most Coleoptera species and has been considered to be an ancestral feature (Smith & Virkki Citation1978). Almeida et al. (Citation2009) described the chromosome morphology of four species of Omphoita, and the differences observed were explained by the occurrence of the pericentric inversion. The loss of homology between the sex chromosomes of the Alticinae species seems to be an ancient event, taking into account the fact that the basal tribe Alticini, as well as the derivative subtribe Oedionychina, do not show synapsis between the sex chromosomes (Virkki Citation1972).
Heterochromatin contains a variety of repetitive DNA sequences normally found in eukaryote genomes, and is an important tool for chromosomal structuring. These sequences comprise tandem repeats, such as satellite DNA (satDNA), including minisatellite and microsatellite DNA, as well as interspersed repetitive elements, such as transposons and retrotransposons (Charlesworth et al. Citation1994).
Studies of constitutive heterochromatin in Coleoptera have demonstrated preferential localisation at the centromeric region, with lower frequency in the interstitial and telomeric regions. The constitutive heterochromatin in the sex chromosomes varies; it may be exclusively localised at the pericentromeric region or throughout the entire chromosome length, which depends on the level of chromosome differentiation in the species (Almeida et al. Citation2000; Rozek et al. Citation2004). Certain families, such as Elateridae, Cantharidae and Tenebrionidae, feature high levels of heterochromatin (Rozek et al. Citation2004; Schneider et al. Citation2006). C-banding data are available for various Coleoptera families; however, only seven Alticinae species have been studied (Virkki Citation1983; Almeida et al. Citation2006, Citation2009). Virkki (Citation1983) studied mitotic and meiotic chromosomes in four Alticinae species (Omphoita annularis Illiger 1807, Omphoita personata Illiger 1807, Omphoita octoguttata Fabricius 1775 and Alagoasa januaria Bechyné 1955) using the C-banding method and observed pericentromeric bands on each complementary chromosome, as well as additional bands that varied in number, position and staining intensity on the sex chromosomes.
Base-specific fluorochromes have been used for certain Coleoptera species to discern the base composition in heterochromatic regions; these may be detected by C-banding and may be heterochromatin associated or coincident with nucleolus organiser regions (NOR) (Ennis Citation1974; Juan et al. Citation1991; Plohl et al. Citation1993; Maffei et al. Citation2001; Moura et al. Citation2003; Vitturi et al. Citation2003; Bione et al. Citation2005a, b; Almeida et al. Citation2006; Schneider et al. Citation2007; Goll et al. Citation2013). Knowledge of chromatin composition may facilitate certain inferences about the functions of particular chromosomal regions. GC-rich DNA sequences are related to synapsis and genetic recombination during meiosis, as well as “housekeeping genes” such as NORs; such sequences are more susceptible to breaks. Highly repetitive AT sequences are linked to the structural organisation of the genetic material, as well as to replication and transcription initiation events (Sumner Citation2003). In Coleoptera, base-specific fluorochromes, especially chromomycin A3 (CMA3) and 4′-6-diamidino-2-phenylindole (DAPI), have only been used for families such as Chrysomelidae (Almeida et al. Citation2006), Coccinellidae (Maffei et al. Citation2001), Elateridae (Schneider et al. Citation2007), Geotrupidae (Vitturi et al. Citation1999; Colomba et al. Citation2004), Lucanidae (Colomba et al. Citation2000a), Scarabaeidae (Colomba et al. Citation1996, Citation2006, Citation2000b; Moura et al. Citation2003; Vitturi et al. Citation2003; Bione et al. Citation2005a, b) and Tenebrionidae (Juan et al. Citation1991; Plohl et al. Citation1993; Goll et al. Citation2013). Currently, only one study has used fluorochromes in Alticinae (Almeida et al. Citation2006).
Repetitive DNA has been studied in certain Coleoptera species to discern the localisation of ribosomal (Galián et al. Citation1995; De la Rúa et al. Citation1996; Proença et al. Citation2002a, b; Bione et al. Citation2005a, b; Cabral-de-Mello et al. Citation2010, Citation2011a; Oliveira et al. Citation2012; Goll et al. Citation2013) and histone genes (Cabral-de-Mello et al. Citation2010, Citation2011a), the base composition and localisation of satDNA monomers, and to study the evolution of such sequences (Juan et al. Citation1993a, b; Ugarković et al. Citation1996; Pons et al. Citation1997; Lorite et al. Citation2001, Citation2002; Pons et al. Citation2002; Pons Citation2004; Mravinac & Plohl Citation2007; Wang et al. Citation2008). Satellite DNA sequences have been studied in few Chrysomelidae species: Chrysolina americana Linnaeus 1758, Xanthogaleruca luteola Müller 1766 (Lorite et al. Citation2001, Citation2002) and Chrysolina carnifex Fabricius 1792 (Palomeque et al. Citation2005). Currently, no studies have investigated satDNA or repetitive DNA in the Alticinae species.
Repetitive DNA has been identified as a possible agent for chromosomal rearrangement in multiple species. Examples of such DNA include satDNA, for which amplification and movement in chromosomes has been well-documented in certain organisms (Hamilton et al. Citation1990; Modi Citation1993), as well as transposable elements (TEs) (Cáceres et al. Citation1999), which play a role in karyotype evolution (Hamilton et al. Citation1992; Wichman et al. Citation1992; Yang et al. Citation1997; Garagna et al. Citation2001; Marchal et al. Citation2004). Thus, because repetitive sequences are mobile, they are interesting cytogenetic markers for studying the evolution of chromosomal rearrangements in many organisms. Genome amplification through repetitive DNA sequences, such as satDNA and TEs, could be related to the evolution of giant sex chromosomes in Alticinae, especially in the subtribe Oedionychina. Thus, the aim of the present study was to investigate the differentiation and evolution of the sex chromosomes in species of the genus Omophoita (Alticinae) using repetitive DNA (total C0t-1), C-banding and base-specific fluorochromes.
Materials and methods
Cytologic preparations
Omophoita octoguttata, Omophoita personata and Omophoita sexnotata Harold 1876 samples were collected from natural populations in Itaiacoca, Paraná State, Brazil (25°07′10′′S and 49°56′24′′W), and identified by Carlos Campaner of the Museu de Zoologia, Universidade de São Paulo, Brazil.
Adult gonads were used for the cytological preparations. The gonads were dissected in physiological saline for insects, hypotonised and fixed in Carnoy I as described in Almeida et al. (Citation2000). The chromosomes were stained with fluorochromes, according to Schweizer et al. (Citation1983). The fluorochrome 4’-6-diamidino-2-phenylindole (DAPI) was used to verify AT-rich regions, and the fluorochrome chromomycin A3 (CMA3) was used to identify GC-rich regions, both combined with distamycin A (DA) counterstain. The slides were then submitted to C-banding in accordance with Férnandez et al. (Citation2002). Subsequently, the slides were hybridised to the total C0t-1 fraction.
DNA extraction and isolation for repetitive DNA
Murray and Thompson’s (Citation1980) protocol was used for DNA extraction, with modifications. DNA was extracted from some corporal regions, such as the head, pronotum and femur by maceration in liquid nitrogen.
Repetitive DNA, isolated through reassociation kinetics, was based on the total DNA C0t-1 method (DNA enriched with moderately and highly repetitive sequences), following Zwick et al. (Citation1997). Briefly, genomic DNA was diluted to a final concentration of 300 ng/µL in 0.3 mol/L sodium chloride (NaCl). The sample was autoclaved (121°C, 1.034 × 105 Pa) for 5 min to generate fragments between 100 and 1000 bp. The DNA was then denatured at 95°C for 10 min and re-annealed at 65°C. Subsequently, the DNA was digested with nuclease S1 (Promega) at 37°C for 8 min. The DNA fragments were used as total C0t-1 probes for fluorescent in situ hybridisation (FISH) for the three species.
Fluorescent in situ hybridisation (FISH)
FISH was performed with Bio-Nick translation and Dig-Nick translation (Roche). Streptavidin-Alexa Fluor 488 (Molecular Probes) was used for biotin signal detection and amplification, and anti-digoxigenin (Roche) was used to detect digoxigenin.
Hybridisation was performed in accordance with Pinkel et al. (Citation1986) and slightly modified, as described by Almeida et al. (Citation2010). The hybridisation conditions were highly stringent (2.5 ng/µL of probe, 50% deionised formamide, 10% dextran sulphate and 2X saline-sodium citrate buffer (2XSSC) at 37°C overnight). Following hybridisation, the slides were washed in 15% formamide/0.2XSSC at 42°C for 20 min, 0.1XSSC at 60°C for 15 min and 4XSSC/0.05% Tween at room temperature for 10 min, which consisted of two 5-min washes.
The chromosomes were stained with DAPI (0.2 mg/ml). The cells were photographed under an Olympus BX41 epifluorescence microscope. The images were acquired using an Olympus DP-71, 12-megapixel camera.
Results
Karyotypes, C-banding and fluorochrome staining
The conventional meiotic analysis was consistent with previously described standards for Omophoita octoguttata, Omophoita personata and Omophoita sexnotata (Almeida et al. Citation2009). The species analysed possess 2n = 10II + X + Y, with giant sex chromosomes and asynaptic but regular segregation.
Additionally, the present study confirmed the C-banding pattern in the centromeric region of most autosomes described for O. sexnotata and Omophoita magniguttis Bechyné 1955 (Almeida et al. Citation2009). Meiotic cells of the three species analysed were submitted to C-banding and showed similar constitutive heterochromatin distribution patterns with blocks in the pericentromeric region of most autosomes (A, A and A). However, O. octoguttata exhibited the largest amount of heterochromatin in the pericentromeric region.
Figure 1. Meiotic cells from Omophoita octoguttata submitted to C-banding (A) and staining with the fluorochromes CMA3/DA/DAPI (B–H). A, metaphase I, arrow heads = centromeric positive staining, insert = sex chromosomes of another metaphase I and arrows show heterochromatin. B, DAPI positive = arrows. C, CMA3 positive = arrow heads. D, overlay of images B and C of the autosomes; arrows and arrow heads = positive staining. E, idiograms of the autosomes observed in D; arrow = AT-rich short arms and arrow head = GC-rich short arms. F, DAPI positive = arrows. G, CMA3 positive = arrow heads. H, overlay of images F and G of the anaphase II; arrows = AT-positive regions; arrow heads = GC-positive regions. Scale bar: 10 µm.
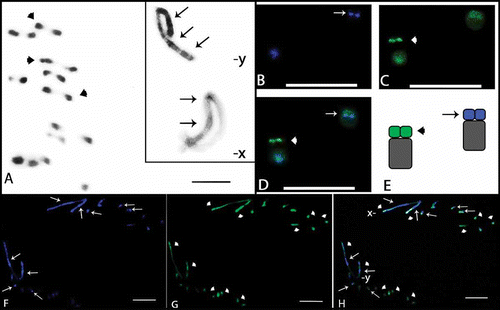
Figure 2. Meiotic cells from Omophoita personata submitted to C-banding (A) and staining with the fluorochromes CMA3/DA/DAPI (B–D). A, metaphase I; arrows = centromeric heterochromatin. B, DAPI positive = arrows. C, CMA3 positive = arrow heads. D, overlay of images B and C of the metaphase I, arrows = AT-positive regions and arrow heads = GC-positive regions. Insert = sex chromosomes (X and Y) of another cell. Scale bar: 10 µm.
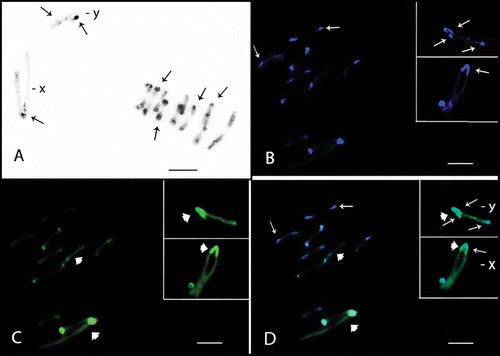
Figure 3. Meiotic cells from Omophoita sexnotata submitted to C-banding (A) and staining with the fluorochromes CMA3/DA/DAPI (B–D). A, metaphase I arrows = centromeric heterochromatin, insert = detailed view of the other Y chromosome. B, DAPI positive = small arrows. C, large arrows = CMA3 positive; and arrow heads = GC-positive short arms D, overlay of images B and C of the metaphase II, small arrows = AT-positive regions and large arrows = GC-positive regions. Insert = X and Y chromosomes; small arrows = AT-positive regions, large arrows = GC-positive regions and arrow heads = GC-positive proximal bands. Scale bar: 10 µm.
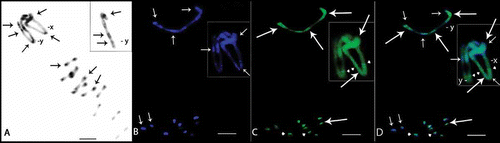
The sex chromosomes exhibited different C-banding patterns for the species analysed. Omophoita octoguttata presented heterochromatin in the centromeric and interstitial regions on one arm of the X chromosome, while the Y chromosome showed interstitial bands on its long arm and a terminal band on its short arm (A insert, ). O. personata presented C-banding in the centromeric region of the X chromosome, while the Y chromosome showed more prominent bands in the terminal regions of one arm and in the interstitial region of the opposite arm (A, ). O. sexnotata exhibited centromeric and terminal heterochromatic bands in the X chromosome, while the Y chromosome showed interstitial bands on its long arm and a terminal band on its short arm (A, ).
Figure 4. Meiotic cells from Omophoita octoguttata (A, D, G), O. personata (B, E, H) and O. sexnotata (C, F, I) submitted to cross-hybridisation using the total C0t-1 product from O. octoguttata in A–C, from O. personata in D–F, and from O. sexnotata in G–I. arrows = punctiform marks, arrow heads = positive marks. Scale bar: 10 µm.
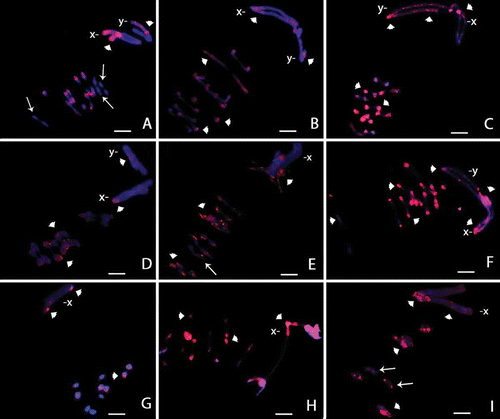
Figure 5. Ideogram of sex chromosomes (X left, Y right) showing the different patterns of marks obtained with C-banding, fluorochromes CMA3/DA/DAPI and C0t-1 probes from Omophoita octoguttata, O. personata and O. sexnotata. (





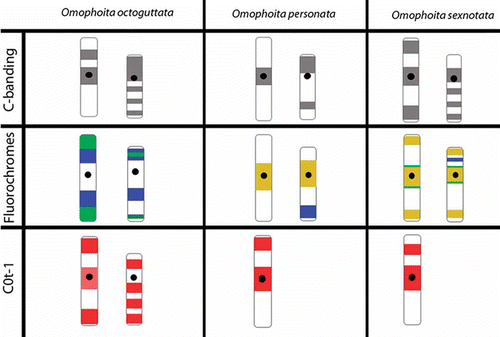
Staining with fluorochromes (CMA3/DA/DAPI) showed small AT-rich blocks (DAPI+) in the centromeric and heterochromatic regions of the autosomes in the three species studied (F, H, B and B). In addition, at least one entirely GC-rich bivalent or GC-rich chromosome was observed in the three species analysed (G, H, C, C and D). Furthermore, O. personata showed one entirely AT-rich bivalent (B and D), O. octoguttata showed one chromosome pair with AT-rich short arms and another pair with GC-rich short arms (B–E), and O. sexnotata showed one chromosome pair with GC-rich short arms (C and D, large arrow).
Concerning sex chromosomes, O. octoguttata showed GC-rich blocks in the terminal regions of the X chromosome as well as interstitial AT-rich blocks; the Y chromosome showed both interstitial and terminal GC- and AT-rich blocks that were intercalated (F–H, ). In O. personata, the X and Y chromosomes showed AT- and GC-rich marks in their pericentromeric regions, while the Y chromosome also had a terminal AT-rich region (B, D inserts, ). In O. sexnotata, the X and Y chromosomes presented AT- and GC-rich blocks in their terminal and pericentromeric regions, in addition to AT interstitial blocks in the Y chromosome (B–D, ). An additional GC-rich proximal band was also found in both sex chromosomes (C and D, arrow heads in the insert, ).
FISH using total C0t-1 probes
Total C0t-1 DNA hybridisation for the three species against their own chromosomes revealed a pericentromeric pattern in most bivalents () that was similar to the heterochromatin distribution pattern. However, there were small differences between the species. In O. octoguttata, three bivalents showed punctiform staining in the pericentromeric region (A) and a differing of the C- banding pattern. In O. personata (E) and O. sexnotata (I), this punctiform pattern was similar in only one or two bivalents, respectively. Additionally, one bivalent was almost entirely stained in O. personata and two bivalents in O. sexnotata. For the sex chromosomes, the X chromosome in O. octoguttata (A, ) showed weak staining in the centromeric region, while terminal regions were strongly stained; staining was also observed throughout one of the Y arms. The X chromosome in O. personata (E, ) and O. sexnotata (I, ) were stained in the pericentromeric and terminal regions; in addition, one arm of this chromosome was also interstitially stained in O. sexnotata. Staining was not evident for the Y chromosome of O. personata and O. sexnotata.
Cross-hybridisation with C0t-1 for DNA showed staining in the pericentromeric region of most autosomes in all species, which was independent of the probe utilised (B–D, F–H). Additional staining along almost an entire bivalent was observed for O. personata (B, H) and O. sexnotata (C, F). Moreover, one almost entirely unstained bivalent or chromosome in O. octoguttata was observed after hybridisation to the total C0t-1 DNA from O. personata and O. sexnotata (D, G).
The X chromosomes for the species displayed a similar staining pattern in the pericentromeric region for each cross-hybridisation (B–D, F–H). However, staining was clearly observed at the ends of the X chromosome arm in O. octoguttata (D, G) and O. sexnotata (C, F).
The Y chromosome for O. personata and O. octoguttata displayed a staining pattern throughout one arm when hybridised to the total C0t-1 DNA for both species (B, D). The Y chromosome of O. sexnotata showed slight staining in the centromeric and terminal regions when hybridised with the total C0t-1 DNA from O. octoguttata (C) and O. personata (F).
Discussion
The extremely large and asynaptic sex chromosomes of Oedionychina could be a derived characteristic (Almeida et al. Citation2009). This is supported if we consider the chromosome system of the Xyp type of the suborder Polyphaga as a basal condition (Smith & Virkki Citation1978), as well as the occurrence of this sex system in the subfamilies Chrysomelinae and Galerucinae, which are more closely related to Alticinae. Some suggestions, such as numerous chromosomal rearrangements, autosomal translocations to the sex chromosomes and changes in the quantity of constitutive heterochromatin, have already been proposed to explain the origin of this special class of chromosomes (White Citation1973; Smith & Virkki Citation1978). However, data about the composition and the process of differentiation of these chromosomes are not available.
The family Chrysomelidae is primarily characterised by a small quantity of heterochromatin (Rozek et al. Citation2004; Schneider et al. Citation2006). The few studies performed on Alticinae have showed a preferential centromeric localisation, which is a common feature for Coleoptera (Virkki Citation1983; Almeida et al. Citation2006, Citation2009). Thus, the data generated for the three species analysed in the present study are consistent with the pericentromeric heterochromatin patterns in autosomes described for other species in the subfamily.
The additional C-banding stain on the sex chromosomes for the three species is also consistent with data described by Virkki (Citation1983) for O. octoguttata and O. personata, as well as species that are taxonomically related to Alticinae (Almeida et al. Citation2006, Citation2009). Thus, the staining diversity of the heterochromatin distribution pattern in Coleoptera may represent karyotypic differentiation for this group. According to Almeida et al. (Citation2006), interstitial heterochromatin on the sex chromosomes may be related to the origin of the sex determination system and may represent the remaining material from chromosomes that fused during evolution.
In relation to heterochromatin composition, the DNA with highly repetitive AT sequences in the centromeric region (C-banding+) was common to the three species analysed. These data are in agreement with those described in the literature regarding other related species (Almeida et al. Citation2006), as well as certain Tenebrionidae and Scarabaeidae (Juan et al. Citation1991; Plohl et al. Citation1993; Moura et al. Citation2003; Colomba et al. Citation2006). Additionally, Cabral-de-Mello et al. (Citation2011b) described GC-rich pericentromeric heterochromatin in the genus Dichotomius (Scarabaeidae), and the authors proposed heterochromatin compartmentalisation in species from this genus. Moreover, the chromosomes that are entirely GC-rich and common to the three species, as well as the AT-rich chromosomes in O. personata, have not been described for other Chrysomelidae species. In addition, the GC-rich blocks on the short arms of the chromosomes in O. octoguttata and O. sexnotata indicated karyotype differentiation among the species in the genus Omophoita, and the distinct repetitive DNA compositions observed in such chromosomes are probably related to their evolutionary process. In this way, the repetitive DNA compositions obtained in this study could also explain the chromosomal morphology diversification described in the literature for Omophoita species (Virkki Citation1983; Almeida et al. Citation2009).
The total C0t-1 method generated a more precise and complementary heterochromatin distribution pattern for the species analysed. According to Cabral-de-Mello et al. (Citation2011b), studies based exclusively on C-banding may lead to erroneous descriptions of homogeneity among karyotypes. Thus, according to the authors, the total C0t-1 DNA method supports a thorough genomic analysis. In this sense, the presence of DAPI-positive heterochromatin fluorochrome, and its correspondence with the C0t-1 fraction in the pericentromeric region, suggest the occurrence of highly repetitive DNA in AT sequences in this chromosomal region in the three species. However, the weak hybridisation for the total C0t-1 probes in certain chromosomes suggests that the heterochromatin is composed of a low copy number for different repetitive DNA sequences or classes. Such variation in heterochromatin composition was also observed by Scarabaeidae (Cabral-de-Mello et al. Citation2011b).
For the sex chromosomes, differences in localisation, composition and hybridisation of the total C0t-1 indicate heterochromatin differentiation among such chromosomes of the three Omophoita species. The variation in composition and hybridisation for certain chromosomes from these species indicates heterochromatin differentiation within the Omophoita species.
Cross-hybridisation among the species produced a similar staining pattern for the probes in the pericentromeric region. Thus, we conclude that much of the genome is shared among the Omophoita species, which showed conserved regions and repetitive sequences distributed along the sex chromosomes and autosomes. Additionally, the heterochromatin for these species is largely composed of shared repetitive DNA. Different types of repetitive DNA may be directly linked to heterochromatinisation and the evolution of the giant sex chromosomes in the genus Omophoita and subtribe Oedionychina, as well as the process of autosomal differentiation. This could also be related to the process of the autosome differentiation. Therefore, more refined techniques will help to elucidate the processes underlying chromosomal differentiation among related species with some karyotypic uniformity. Consistent with Cabral-de-Mello et al. (Citation2011b), the total C0t-1 DNA method is an optimal tool for comparative studies on genomes, karyotype diversity and genome evolution in insects because this group has no available libraries, chromosomal probes or sequenced genomes.
Acknowledgements
This study was supported by the Fundação Araucária de Apoio ao Desenvolvimento Científico e Tecnológico do Estado do Paraná, the Coordenação de Aperfeiçoamento de Pessoal de Nível Superior-CAPES and the Conselho Nacional de Desenvolvimento Científico e Tecnológico-CNPq. The authors are grateful to the expert Carlos Campaner for his assistance in the identification of the species.
References
- Almeida MC, Campaner C, Cella DM. 2006. Karyotype characterization, constitutive heterochromatin and nucleolus organizer regions of Paranaita opima (Coleoptera, Chrysomelidae, Alticinae). Genetics and Molecular Biology 29:475–481.
- Almeida MC, Campaner C, Cella DM. 2009. Cytogenetics of four Omophoita species (Coleoptera, Chrysomelidae, Alticinae): A comparative analysis using mitotic and meiotic cells submitted to the standard staining and C-banding technique. Micron 40:586–596.
- Almeida MC, Goll LG, Artoni RF, Nogaroto V, Matiello RR, Vicari MR. 2010. Physical mapping of 18S rDNA cistron in species of the Omophoita genus (Coleoptera, Alticinae) using fluorescent in situ hybridization. Micron 41:729–734. doi:10.1016/j.micron.2010.06.008.
- Almeida MC, Zacaro AA, Cella DM. 2000. Cytogenetic analysis of Epicauta atomaria (Meloidae) and Palembus dermestoides (Tenebrionidae) with Xyp sex determination system using standard staining, C-bands, NOR and synaptonemal complex microspreading techniques. Hereditas 133:147–157.
- Bione E, Camparoto ML, Simões ZLP. 2005a. A study of the constitutive heterochromatin and nucleolus organizer regions of Isocopris inhiata and Diabrostis mimas (Coleoptera: Scarabaeidae, Scarabaeinae) using C-banding, AgNO3 staining and FISH techniques. Genetics and Molecular Biology 28:111–116.
- Bione E, Moura RC, Carvalho R, Souza MJ. 2005b. Karyotype, C-and fluorescence banding pattern, NOR location and FISH study of five Scarabaeidae (Coleoptera) species. Genetics and Molecular Biology 28:376–381.
- Cabral-de-Mello DC, Moura RC, Martins C. 2010. Chromosomal mapping of repetitive DNAs in the beetle Dichotomius geminatus provides the first evidence for an association of 5S rRNA and histone H3 genes in insects, and repetitive DNA similarity between the B chromosome and A complement. Heredity 104:393–400. doi: 10.1007/s10709-011-9551-7.
- Cabral-de-Mello DC, Moura RC, Martins C. 2011a. Cytogenetic mapping of rRNAs and Histone H3 genes in 14 species of Dichotomius (Coleoptera, Scarabaeidae, Scarabaeinae) beetles. Cytogenetic and Genome Research 134:127–135. doi:10.1159/000326803.
- Cabral-de-Mello DC, Moura RC, Melo AS, Martins C. 2011b. Evolutionary dynamics of heterochromatin in the genome of Dichotomius beetles based on chromosomal analysis. Genetica 139:315–325. doi:10.1007/s10709-011-9551–7.
- Cáceres M, Ranz JM, Barbadilla A, Long M, Ruiz A. 1999. Generation of a widespread Drosophila inversion by a transposable element. Science 285:415–418.
- Charlesworth B, Snlegowski P, Stephan W. 1994. The evolutionary dynamics of repetitive DNA in eukaryotes. Nature 371:215–220.
- Colomba MS, Monteresino E, Vitturi R, Zunino M. 1996. Characterization of mitotic chromosomes of the scarab beetles Glyphoderus sterquilinus (Westwood) and Bubas bison (L.) (Coleoptera: Scarabaeidae) using conventional and banding techniques. Biologisches Zentralblatt 115:58–70.
- Colomba M, Vitturi R, Libertini A, Gregorini A, Zunino M. 2006. Heterochromatin of the scarab beetle, Bubas bison (Coleoptera: Scarabaeidae) II: Evidence for AT-rich compartmentalization and high amount of rDNA copies. Micron 37:47–51.
- Colomba M, Vitturi R, Volpe N, lannino A, Zunino M. 2004. Karyotype banding and rDNA FISH in the scarab beetle Anoplotrupes stercorosus (Coleoptera Scarabaeoidea: Geotrupidae). Description and comparative analysis. Micron 35:717–720.
- Colomba MS, Vitturi R, Zunino M. 2000a. Karyotype analysis, banding, and fluorescent in situ hybridization in the Scarab beetle Gymnopleurus sturni McLeady (Coleoptera, Scarabaeoidea, Scarabaeidae). Journal of Heredity 31:260–264.
- Colomba MS, Vitturi R, Zunino M. 2000b. Chromosome analysis and rDNA FISH in the stag beetle Dorcus parallelipipedus L. (Coleoptera, Scarabaeoidea, Lucanidae). Hereditas 133:249–253.
- De la Rúa P, Serrano J, Hewitt GM, Galián J. 1996. Physical mapping of rDNA genes in the ground beetle Carabus and related genera (Carabidae: Coleoptera). Journal of Zoological Systematics and Evolutionary Research 34:95–101.
- Ennis TJ. 1974. Chromosome structure in Chilocorus (Coleoptera, Coccinelidae). I. Fluorescent and Giemsa banding patterns. Canadian Journal of Genetics and Cytology 16:651–661.
- Fernández R, Barragán MJL, Bullejos M, Marchal JA, Díaz de la Guardia R, Sánchez A. 2002. New C-band protocol by heat denaturation in the presence of formamide. Hereditas 137:145–148.
- Galían J, Serrano J, De la Rúa P, Petitpierre E, Juan C. 1995. Localization and activity of rDNA genes in tiger beetles (Coleoptera: Cicindelidae). Heredity 74:524–530.
- Garagna S, Marziliano N, Zuccotti M, Searle JB, Capanna E, Redi CA. 2001. Pericentromeric organization at the fusion point of mouse Robertsonian translocation chromossomes. Proceedings of the National Academy of Sciences of the United States of America 98:171–175.
- Goll LG, Artoni RF, Vicari MR, Nogaroto V, Petitpierre E, Almeida MC. 2013. Cytogenetic analysis of Lagria villosa (Coleoptera, Tenebrionidae): Emphasis on the mechanism of association of the Xy p sex chromosomes. Cytogenetics and Genome Research 139:29–35. doi: 10.1159/000341674.
- Hamilton MJ, Honeycutt RL, Baker RJ. 1990. Intragenomic movement, sequence amplification and the conccerted evolution in satellite DNA in harvest mice, Reithrodontomys: Evidence from in situ hybridization. Chromosoma 99:321–329.
- Hamilton MJ, Hong G, Wichman HA. 1992. Intragenomic movement and concerted evolution of satellite DNA in Peromyscus: Evidence from in situ hybridization. Cytogenetics and Cell Genetics 60:40–44.
- Juan C, Gonsalvez J, Mezzanotte R, Petitpierre E. 1991. Cytological and biochemical characterization of the in situ endonuclease digestion of fixed Tenebrio molitor chromosomes. Chromosoma 100:432–438.
- Juan C, Pons J, Petitpierre E. 1993a. Localization of tandemly repeated DNA sequences in beetle chromosomes by fluorescent in situ hybridization. Chromosome Research 1:167–174.
- Juan C, Vazquez P, Rubio JM, Petitpierre E, Hewitt GM. 1993b. Presence of highly repetitive DNA sequences in Tribolium flour-beetles. Heredity 70:1–8.
- Lorite P, Carrillo JA, Garnerí I, Petitpierre E, Palomeque T. 2002. Satellite DNA in the elm leaf beetle, Xanthogaleruca luteola (Coleoptera, Chrysomelidae): Characterization, interpopulation analysis, and chromosome location. Cytogenetics and Genome Research 98:302–307.
- Lorite P, Palomeque T, Garnería IM, Petitpierre E. 2001. Characterization and chromosome location of satellite DNA in the leaf beetle Chrysolina americana (Coleoptera, Chrysomelidae). Genetica 110:143–150.
- Maffei EMD, Fragoso DB, Pompolo SG, Serrão JE. 2001. Morphological and cytogenetical studies on the female and male reproductive organs of Eriopis connexa Mulsant (Coleoptera, Polyphaga, Coccinellidae). Netherlands Journal of Zoology 51:483–496.
- Marchal JA, Acosta MJ, Nietzel H, Sperling K, Bullejos M, Díaz de la Guardia R, Sánchez A. 2004. X chromosome painting in Microtus: Origin and evolution of the giant sex chromosomes. Chromosome Research 12:767–776.
- Modi WS. 1993. Comparative analyses of heterochromatin in Microtus: Sequence heterogeneity and localized expansion and contraction of satellite DNA arrays. Cytogenetics and Cell Genetics 62:142–148.
- Moura RC, Souza MJ, Mello NF, Lira-Neto AC. 2003. Karyotypic characterization of representatives fron Melolonthinae (Coleoptera: Scarabaeidae): Karyotypic analysis, banding and fluorescent in situ hybridization (FISH). Hereditas 138:200–206.
- Mravinac B, Plohl M. 2007. Satelite DNA junctions identify the potential origin of new repetitive elements in the beetle Tribolium madens. Gene 394:45–52.
- Murray MG, Thompson WF. 1980. Rapid isolation of high molecular weigth plant DNA. Nucleic acids research 19:4321–4325.
- Oliveira SG, Cabral-de-Mello DC, Arcanjo AP, Xavier C, Souza MJ, Martins C, Moura RC. 2012. Heterochromatin, sex chromosomes and rRNA gene clusters in Coprophanaeus beetles (Coleoptera, Scarabaeidae). Cytogenetics and Genome Research 138:46–55. doi: 10.1159/000339648.
- Palomeque T, López MM, Carrilo JA, Lorite P. 2005. Characterization and evolutionary dynamics of a complex family of satellite DNA in the leaf beetle Chrysolina carnifex (Coleoptera, Chrysomelidae). Chromosome Research 13:795–807.
- Petitpierre E, Segarra C, Yadav JS, Virkki N. 1988. Chromosome numbers and meioformulae of Chrysomelidae. In: Jolivet E, Petitpierre E, Hsiao TH, editors. Biology of Chrysomelidae. Dordrecht: Kluwer Academic Publishers. 161–186.
- Pinkel D, Straume T, Gray JW. 1986. Cytogenetic analysis using quantitative, high–sensitivity, fluorescence hybridization. Proceedings of the National Academy of Sciences of the United States of America 83:2934–2938.
- Plohl M, Lucijanic-Justic V, Ugarkovic D, Petitpierre E, Juan C. 1993. Satellite DNA and heterochromatin of the flour beetle Tribolium confusum. Genome 36:467–475.
- Pons J. 2004. Evolution of diploid chromosome number, sex-determining systems, and heterochromatin in Western Mediterranean and Canarian species of the genus Pimelia (Coleoptera: Tenebrionidae). Journal of Zoological Systematics and Evolutionary Research 42:81–85.
- Pons J, Bruvo B, Juan C, Petitpierre E, Plol M, Ugarkovic D. 1997. Conservation of satellite DNA in species of the genus Pimelia (Tenebrionidae, Coleoptera). Gene 205:183–190.
- Pons J, Petitpierre E, Juan C. 2002. Evolutionary dynamics of satellite DNA family PIM357 of the genus Pimelia (Tenebrionidae, Coleoptera). Molecular Biology and Evolution 19:1329–1340.
- Proença SJR, Serrano ARM, Collares-Pereira MJ. 2002a. An unusual karyotype with low chromosome number in Megacephalini, a basal group of tiger beetles (Coleoptera, Cicindelidae): Cytogenetic characterisation by C-banding and location of rDNA genes. Hereditas 137:202–207.
- Proença SJR, Serrano ARM, Collares-Pereira MJ. 2002b. Cytogenetic variability in genus Odontocheila (Coleoptera, Cicindelidae): Karyotypes, C-banding, NORs and localization of ribosomal genes of O. confuse and O. nodicornis. Genetica 114:237–245.
- Riley EG, Clark SM, Flowers RW, Gilbert AJ. 2002. Family 124. Chrysomelidae. In: Arnett RH, Thomas MC, Skelley PE, Frank JH, editors. American Beetles. Polyphaga: Scarabaeoidea through Curculionoidea. vol. 2. London: CRC Press. 617–691.
- Rozek M, Lachowska D, Petitpierre E, Holecova M. 2004. C-bands on chromosomes of 32 beetle species (Coleoptera: Elateridae, Cantharidae, Oedemeridae, Cerambycidae, Chrysomelidae and Curculionidae). Hereditas 140:1–10.
- Schneider MC, Almeida MC, Rosa SP, Costa C, Cella DM. 2006. Evolutionary chromosomal differentiation among four species of Conoderus Eschscholtz, 1829 (Coleoptera, Elateridae, Agrypninae, Conoderini) detected by standard staining, C-banding, silver nitrate impregnation, and CMA3/DA/DAPI staining. Genetica 128:333–346.
- Schneider MC, Rosa SP, Almeida MC, Costa C, Cella DM. 2007. Chromosomal similarities and differences among four Neotropical Elateridae (Conoderini and Pyrophorini) and other related species, with comments on the NOR patterns in Coleoptera. Journal of Zoological Systematics and Evolutionary Research 45:308–316. doi:10.1111/j.1439/0469.2006.00398.x.
- Schweizer D, Mendelak M, White MJD, Contreras N. 1983. Cytogenetics of the parthenogenetic grasshopper Warramaba virgo and its bisexual relatives. X. Pattern of fluorescent banding. Chromosoma 88:227–236.
- Segarra C, Petitpierre E. 1990. Chromosomal survey in three genera of Alticinae (Coleoptera, Chrysomelidae). Cytobios 64:169–174.
- Smith SG, Virkki N. 1978. Coleoptera. In: John B, editor. Animal Cytogenetic. Berlin, Stuttgart: Gebru der Borntraeger. pp. 366 .
- Sumner AT. 2003. Chromosomes: Organization and function. 1st ed. London: Blackwell Publishing company.
- Ugarković D, Durajlija S, Plohl M. 1996. Evolution of Tribolium madens (Insecta, Coleoptera) satellite DNA through DNA inversion and insertion. Journal of Molecular Evolution 42:350–358.
- Virkki N. 1972. Contraction stage and formation of the distance sex bivalent in Oedionychina (Coleoptera, Alticidae). Hereditas 71:259–288.
- Virkki N. 1983. Banding of Oedionychina (Coleoptera: Alticinae) chromosomes C- and Ag-bands. Journal of Agriculture of the University of Puerto Rico 67:221–255.
- Virkki N. 1988. The sex chromosomes of Disonychina (Coleoptera, Alticinae): Xy + nX systems. Cytobios 53:43–55.
- Virkki N. 1989. Proximal vs. distal collochores in coleopteran chromosomes. Hereditas 110:101–107.
- Virkki N, Mazzella C, Denton A. 1991. Silver staining of the coleopteran Xyp sex bivalent. Cytobios 67:45–63.
- Virkki N, Santiago-Blay JA. 1993. Trends of karyotype evolution in Neotropical Oedionychina (Coleoptera: Chrysomelidae: Alticinae). Hereditas 119:263–283.
- Vitturi R, Colomba MS, Barbieri R, Zunino M. 1999. Ribosomal DNA location in the scarab beetle Thorectes intermedius (Costa) (Coleoptera: Geotrupidae) using banding and fluorescent in situ hybridization. Chromosome Research 7:255–260.
- Vitturi R, Colomba M, Volpe N, Lannino A, Zunino M. 2003. Evidence for male X0 sex-chromosome system in Pentodon bidens punctatum (Coleoptera Scarabaeoidae: Scarabaeidae) with X-linked 18S-28S clusters. Genes & Genetic Systems 78:427–432.
- Wang S, Lorenzen MD, Beeman RW, Brown SJ. 2008. Analysis of repetitive DNA distribution patterns in the Tribolium castaneum genome. Genome Biology 9:1–14. doi:10.1186/gb-2008-9-3–r61.
- White MJD. 1973. Animal cytology and evolution. London: Willian Clowes & Sons.
- Wichman HA, Van den Bussche RA, Hamilton MJ, Baker RJ. 1992. Transposable elements and the evolution of genome organization in mammals. Genetica 86:287–293.
- Yang F, O‘brien PCM, Wienberg J, Ferguson-Smith MA. 1997. A reappraisal of the tandem fusion theory of karyotype evolution in the Indian muntjac using chromo-some painting. Chromosome Research 5:109–117.
- Zwick MS, Hanson RE, Mcknight TD, Nurul-Islam-Faridi M, Stelly DM. 1997. A rapid procedure for the isolation of C0t–1 DNA from plants. Genome 40:138–142.