Abstract
In this study, we investigated fertilization cytology in Phascolosoma esculenta. The sperm binds to the oocyte envelope in a random position at the point of initial contact. An acrosomal reaction then occurs and the acrosomal filament penetrates the oocyte envelope. The apex of the acrosomal filament contacts with the plasma membrane of the oocyte at the point where the fertilization cone is formed. The fertilization cone then retracts, towing the acrosomal filament back and drawing the sperm into the oocyte. Subsequently, the pores on the oocyte envelope are blocked and filled with fibrin and colloid. After the oocyte is activated by sperm, the first and second meiosis processes start sequentially, followed by the release of the first and second polar bodies. After the second polar body is extruded, the haploid female nucleus rapidly reorganizes to form a female pronucleus. In comparison, the sperm nucleus begins to decondense and dilate after the first polar body is released. The male pronucleus is not formed until the end of the second meiosis. The male and female pronuclei migrate to the centre of the oosperm and are incorporated into the zygote nucleus and then the first cleavage occurs. A two cell embryo is then formed by plasma membrane invagination. The cytological changes of sperm and oocyte nuclei during sperm entry into the oocyte and the fertilization process in P. esculenta are characterized by: (1) the mature oocyte with fertilization ability being in the metaphase of the first meiosis; (2) sperm is drawn into the oocyte by the acrosomal filament and fertilization cone; (3) some abnormal phenomena such as polyspermy, multiple pronuclei and multipolar spindle; and (4) the male pronucleus is formed earlier than the female pronucleus, at which point they will fuse to form a zygote nucleus.
Introduction
The Sipuncula is a group of barrel-shaped marine worms whose bodies are divided into a rostrum and a trunk section (Adrianov et al. Citation2002). They are benthonic organisms containing a coelom but no body segments (Yang & Sun Citation2006; Mu et al. Citation2013). While the majority of species are dioecious, exert sexual reproduction and external fertilization, and in some individual species asexual reproduction, is also observed (Mary Citation1970; Maiorova & Adrianov Citation2007, Citation2010). The earliest observation of fertilization in Golfingia vulgaris (de Blainville, 1827) and Phascolopsis gouldii (De Pourtalès, 1851) was made by Gerould (Citation1907). At that time, with limited observation methods, he mistakenly believed that the eggs of these two species had micropyles through which the sperm was penetrating into the egg. Subsequently, further histological observation and analysis of the fertilization process in Phascolosoma agassizi Keferstein, 1866, Golfingia pugettensis Fisher, 1952 and Themistes pyroides (Chamberlin, 1919) was conducted (Rice Citation1966). These revealed that an acrosomal enzyme is released by the acrosome after the sperm binds to the egg. The acrosomal filament then penetrates through the egg envelope to form a pore on the surface that permits sperm entry. Following penetration, as the ooplasm shrinks, a perivitelline space forms, prior to the respective elimination of the first and second polar bodies. Male and female pronuclei then combine by fusion.
Phascolosoma esculenta (Chen & Yeh, 1958), a species endemic to China, belongs to the phylum of Sipuncula, family Phascolosomatidea (Li Citation1989), and is distributed along the southern coast of Zhejiang Province (Long et al. Citation2014a). It inhabits muddy and sediment beaches along the high tide areas of the intertidal zone and is particularly found in caves. Previously, we discovered that P. esculenta has a special reproduction mechanism. The male germ cell falls into the coelom in the form of a cell mass at which time the development process proceeds to the prophase of the spermatid. Spermiogenesis is then completed in the body cavity (Reunov & Rice Citation1993; Maiorova & Adrianov Citation2007). The female germ cell separates itself from the ovary and enters the coelom to become mature when it develops into the early phase of the primary oocyte (Gu et al. Citation2009). The mature sperm and egg then enter the nephridia where they remain for a short while before they are released into the surrounding environment for fertilization (Zhu et al. Citation2007). Because of the environmental volatility of the intertidal zone and the severe ecological conditions for reproduction, the cytological mechanism of fertilization in P. esculenta is probably both special and complicated (Zhu et al. Citation2008, Citation2012; Chen et al. Citation2009; Long et al. Citation2014b). For instance, sperm needs to be able to enter the egg rapidly and the time required for the integration of the male and female pronuclei to form a zygote nucleus will be short. Thus, under severe conditions the fertilization rate needs to be increased and the fertilization process accelerated. In order to corroborate this and explore the cytological characteristics of the fertilization process in P. esculenta, light and scanning electron microscopy were employed to further analyse a series of cytological changes during the fertilization process. These included sperm binding to and penetration into the egg, the formation and integration of male and female pronuclei, and the formation and extrusion of polar bodies with reference to already-conducted fluorescence microscopy observations. Moreover, the comparison with other aquatic animals’ fertilization characteristics is discussed. Our conclusion will lay a foundation for the further study on the fertilization mechanism of this species.
Materials and methods
Experimental animals
Specimens of P. esculenta used in the experiment were obtained from a cultivation pond in Wugen Town, Wenling City of Zhejiang Province from July to August 2012. The average weight and size of the individuals were 2–3 g and 3–7 cm, respectively. This work was carried out in strict accordance with the requirement “Governing Regulation for the Use of Experimental Animals in Zhejiang Province” (Zhejiang Provincial Government Order No. 263).
Spawning induction
Five hundred sexually mature individuals were selected for the experiment. The animals were washed with filtered seawater and then unfolded on a web. After several hours of shade-drying in a windy place, they were put into a net basket and placed in an indoor sand filtered seawater substrate (at 30–31°C with a salinity of 23‰) to induce spawning through water flow stimulation. The spermiation process was characterized by the milky semen, which was released from the nephridia into the surrounding environment by nephridiopore, and this phenomenon could be observed directly. When spawning and spermiation were detected, the stimulation was stopped. Subsequently, individuals with their nephridia filled with either sperm or eggs were selected for dissection.
Artificial fertilization and sample collection
Spermatozoa in the male and eggs in the female nephridia were obtained by dissection. Semen was diluted at 1:1000 with filtered seawater (30–31°C with a salinity of 23‰), and then 1000 ml of diluted semen was mixed with 50 ml eggs and the mixture then incubated in filtered seawater. The concentration of sperm and egg number was 5 × 106/ml. A sample of an unfertilized egg was taken before fertilization and then samples were taken every 1 min for the first 5 min after fertilization, and every 2 min from 5 min to 15 min after fertilization, and every 5 min from 15 min later to the first cleavage. Samples destined for scanning electron microscope observation were collected 15 min after fertilization while samples used for light microscopy were taken from the very beginning to the first cleavage.
Scanning electron microscopy
Samples were fixed in a mixture of 2% glutaraldehyde and 2.5% paraformaldehyde (pH 7.4) at 4°C for 2 h and then washed twice with phosphate buffer solution (PBS) (pH 7.4). They were then transferred into 1% osmic acid for 2 h followed by dehydration with graded ethanol, vacuum freeze-drying and gilding. Finally, the samples were observed under a scanning electron microscope (Hitachi S-3400N, Japan).
Light microscopy
Samples were fixed in Bouin’s solution at room temperature for 24 h. Later, they were dehydrated in graded ethanol, immersed in xylene, and embedded in paraffin. The sections were cut from the blocks, mounted on slides, and then stained with haematoxylin and eosin. The sections were observed under a light microscope (Nikon YS100, Japan).
Results
Morphology and nuclear phase of the mature egg
The oocyte in the nephridia of P. esculenta is a mature egg capable of being fertilized. Under the scanning electron microscope the egg appears laterally ovate () and round from an apical view () with a long diameter of 140–150 µm and a short diameter of 110–120 µm. The egg surface is densely covered with granular protuberances, resulting in the removal of the jelly coat and the absence of micropyles (). By light microscopy, the nuclear phase is shown to be the metaphase of the first meiosis. The nucleus anchors preferentially at one end of the ootid. The spindle is clear and its axis is parallel to the egg axis, perpendicular to the egg surface. The homologous chromosomes, driven by the spindle’s microtubules, are arranged in the equatorial plate. The vitelline membrane is 7–9 µm thick, the perivitelline space between the egg envelope and the plasma membrane is obvious and the yolk in the ovoplasm scatters evenly.
Figure 1. Scanning electron microscopic analysis. (a) Mature egg, lateral view. (b) Mature egg, apical view. (c) Granular protuberance on the surface of mature egg. (d) Sperm binding to the surface of egg. (e) Sperm shape. (f) Acrosomal filament is produced by acrosomal action. (g) Acrosomal filament agley penetrates into the egg envelope. (h) The head of the sperm about to enter the egg. (i) The front of the sperm head embedded in the egg envelope. (j) Sperm head largely penetrated into the egg envelope. (k) Micropyle on the surface of the egg envelope. (l–m) Micropyle filled with fibrin and colloid. (n) The micropyle is blocked. (o) Two sperm penetrating the egg. (p) Single sperm penetrating into the egg near a micropyle. (q) Several sperm penetrating into one egg. (r) Many micropyles remaining beyond polyspermy. AC, acrosome; N, nucleus, M, mitochondria; F, flagellum; AF, acrosomal filament; PP, micropyles left by polyspermy; S, sperm.
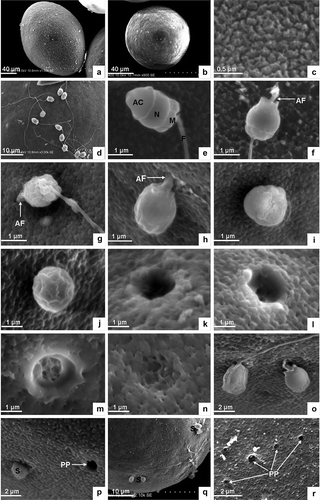
Sperm binding to and penetration into egg
Mature sperm obtained from the nephridia of P. esculenta is capable of movement and fertilization. The sperm head consists of a bell-like acrosome and a drum-shaped nucleus. The midpiece is actually a mitochondrial ring, and the tail a slim flagellum ( and ). On paraffin sections the sperm head appears almost round ().
Figure 2. Light microscopic analysis of sperm penetration into egg and first meiosis. (a) Unfertilized mature egg showing the long axis of spindle positioned perpendicularly to the plasma membrane and with chromosomes arranged and ordered in the equatorial plate. (b) Magnification of Figure 2a. (c) Sperm binding to vitelline membrane. (d) The acrosomal filament penetrates through the egg envelope and contacts the plasma membrane at a site where the ooplasm protrudes to form a fertilization cone. (e) A sperm penetrating into the egg, showing the head of the sperm embedded in the egg envelope and the fertilization cone drawing back. (f) The sperm head entering the fertilization cone after it has penetrated the egg envelope. (g) The sperm in the egg located at the periphery of the ooplasm. (h) Anaphase of the first meiosis showing chromosomes drawn by spindle fibres separating from each other and moving towards the two opposite poles. (i) Anaphase of the first meiosis showing the separated chromosomes arriving at the two opposite poles. (j) At the telophase of the first meiosis, the plasma membrane is lifted up by the chromosomes. (k) At the telophase of the first meiosis, the plasma membrane invaginates and breaks up. (l) The first polar body is extruded, and the sperm nucleus begins to expand. FN, female nucleus; PS, perivitelline space; CH, chromosome; S, sperm; AF, acrosomal filament; PM, plasma membrane; SN, sperm nucleus; PB1, the first polar body.
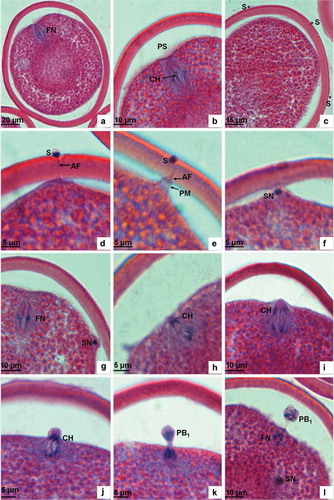
After the mixture of sperm and egg, sperm swim quickly via the waving of their flagella and search for an egg to fertilize. Sperm are able to move for 8 h and fertilize normally within 30 min. Sperm cling to the surface of the egg following contact, and often many sperm cohere simultaneously to the egg surface ( and ). As the sperm happen to make contact with the egg surface they attach themselves at wherever random point of contact initially occurred ().
Acrosomal reaction is induced by egg secretion. The bell-like acrosome disrupts and releases an acrosomal enzyme to dissolve the egg envelope, loosening its structure. Meanwhile, the acrosomal rod in the subacrosomal space assembles to form an acrosomal filament (), which can obliquely penetrate through the egg envelope (). The apex of the acrosomal filament then touches the plasma membrane at a place where the ovoplasm protrudes to form the fertilization cone. The apex of the fertilization cone is connected to the egg envelope (). Subsequently, the fertilization cone draws the acrosomal filament back and the sperm head is gradually pulled into the egg envelope and the flagellum discarded (, ). The pore left on the surface, with its dimension identical to the egg’s short diameter, is then stuffed and blocked by fibres and colloid (, ). Ultimately, most of the granular protuberances on the surface of the egg envelope fall off after sperm penetration. This enables the micropores in the egg envelope to become clearly visible ().
The whole process from the sperm binding to the egg to the sperm penetrating the egg lasts 5–10 min.
The meiosis of oocyte and the formation and release of polar bodies
The oocyte is activated after sperm penetration and the spindle apparatus in the metaphase of the first meiosis is restarted. As a result of the pull force by spindle fibres, the homologous chromosomes, arranged in the equatorial plate, are segregated into two groups and begin moving towards the two respective poles (). They finally arrive in the anaphase of the first meiosis. When it comes to the telophase of the first meiosis, chromosomes that are closer to the plasma membrane will protrude towards the perivitelline space and form the nucleus material for the first polar body ( and ). The plasma membrane then invaginates to form the oval of the first polar body. The polar body is comprised of nucleus material and cytoplasm and is covered by a plasma membrane ( and ).
After the first polar body is extruded, the chromatins of the nucleus remaining in the cytoplasm soon condense to form chromosomes. The spindle then re-appears and chromosomes are arranged on the equatorial plate as pulled by the spindle microtubules. These are the characteristics of the metaphase of the second meiosis (). In the anaphase of the second meiosis, chromosomes are moved towards the two poles by being towed by the spindle fibres ( and ). In the telophase of the second meiosis, the chromosomes closer to the plasma membrane form the second polar body, which is released to the perivitelline space and then locates beneath the first polar body ( and ). At this point, three polar bodies can be found in the perivitelline space () due to the first polar body dividing into two parts ().
Figure 3. Light microscopic analysis of second meiosis. (a) The metaphase of the second meiosis showing the long axis of the spindle positioned perpendicular to the plasma membrane and chromosomes arranged in the equatorial plate. (b) The metaphase of the second meiosis showing the first polar body dividing. (c) The anaphase of the second meiosis showing chromosomes drawn by spindle fibres separating from each other and moving towards the two opposite poles. (d) The anaphase of the second meiosis showing the separated chromosomes moving towards the two opposite poles. (e) The telophase of the second meiosis showing the second polar body extruded. (f) The telophase of the second meiosis showing the first polar body cleaving into two parts. (g) Male and female pronuclei are formed after the second meiosis. (h–i) Male and female pronuclei migrating to the centre of the oocyte. (j) Male and female pronuclei combining. (k) Zygote nucleus. (l) In the prophase of the first cleavage, the spindle appears at the two poles of the nucleus. PB1, the first polar body; FN, female nucleus; CH, chromosome; PB2, the second polar body; FP, female pronucleus; MP, male pronucleus; ZN, zygote nucleus.
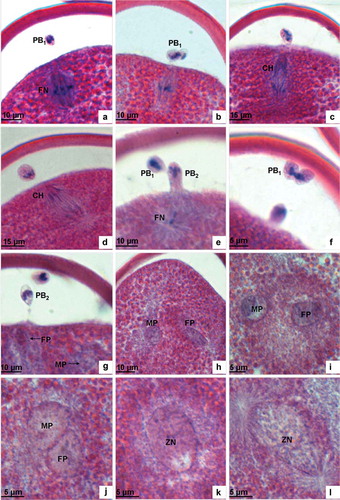
The first and second meiosis each last approximately 10 min.
The formation and integration of male and female pronuclei
The formation of the male pronucleus
Sperm that has just entered the egg is positioned at the verge of the ooplasm. Under the microscope the sperm nucleus appears as a rotund, black, dense dot (). Sperm move inwardly during the two meiosis processes of the egg. When the first polar body is released, the volume of the sperm nucleus expands slightly and the nucleus’ material becomes loose (). As the second meiosis proceeds, the volume of sperm nucleus is further dilated. At an area one-third of a radius’ distance towards the plasma membrane, the orbicular-ovate male pronucleus is not formed until the extrusion of the second polar body (). The male pronucleus has a distinct nuclear envelope with chromatins distributed in the nucleus in the form of tiny aggregates ( and ).
The formation of the female pronucleus
When the second meiosis is complete, the haploid chromosome set rapidly decondenses and expands to form the female pronucleus. At the very beginning, the female pronucleus is located under the plasma membrane (), closer to the polar body where its shape is similar to that of the male pronucleus. The nucleus envelope is clear and chromatin then scatters, dispersing in the form of tiny masses ( and ).
The combination of female and male pronuclei
After their formation, the female and male pronuclei migrate to the centre of the egg, approaching each other simultaneously. When contact is achieved at one end (), the adjacent nucleus membrane disappears, and chromatins blend together to form the zygote nucleus. The newly formed zygote nucleus appears oval with an apparent envelope and homogeneous distribution of small intranuclear chromatin masses ().
The process from sperm penetration into the egg to the integration of female and male pronuclei and the formation of the zygote nucleus lasts between 35 and 40 min.
The first cleavage
In the prophase, the mitotic apparatus spindle appears at each end of the zygote nucleus and chromatins gradually condense to form chromosomes (, ). In the metaphase, chromosomes are rearranged and ordered on the equatorial plate as driven by the spindle microtubules (). In the anaphase, chromosomes are pulled by spindle fibres and separated into two groups moving towards the two poles, respectively (). In the telophase, the two chromosome sets arrive at their respective poles and the ooplasm breaks up. The two groups of chromosomes then migrate to the centre of the now segmented sphere and the two-cell embryo is formed ().
Figure 4. Light microscopic analysis of the first cleavage and polyspermy phenomenon. (a) In the prophase of the first cleavage the nuclear membrane disintegrates and the chromatin condenses to form chromosomes. (b) In the metaphase of the first cleavage chromosomes arrange in the equatorial plate. (c) The anaphase of the first cleavage showing chromosomes drawn by spindle fibres separating from each other and moving towards the two opposite poles. (d) In the telophase of the first cleavage, the separated chromosomes arrive at the two opposite poles. (e) The telophase of the first cleavage showing the cleavage furrow shape. (f) Two-cell embryo. (g) Polyspermy showing multiple male pronuclei. (h) Fusion of several pronuclei. (i–l) Multipolar spindle and multipolar separation of chromosomes. ZN, zygote nucleus; CH, chromosome; CF, cleavage furrow.
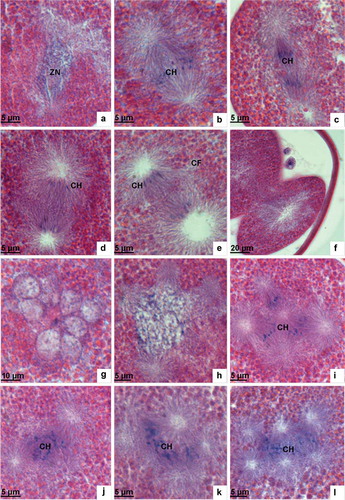
Polyspermy and multipolar separation
In this experiment, polyspermy was also observed where other sperm are observed penetrating the egg beyond the point the first sperm has already entered ( and ). In some eggs, it was found that many sperm were simultaneously penetrating into one egg (), leaving may pores on the egg surface (). The nuclei of multiple sperm which have entered the same egg may decondense and expand to form male pronuclei. These male pronuclei can combine with each other during the process of egg meiosis (). The appearance of a multipolar spindle in abnormal mitosis and multipolar separation of chromosomes was even observed (–l).
Discussion
Sperm binding to and penetration into egg
The pattern of sperm binding and egg penetration is related to the structure of the sperm and egg. Teleost fish sperm have a flagellum but no acrosome and can move in water for a short time. Their egg has a special micropyle through which the sperm is able to gain entry (Liu & Yang Citation2002). In decapod crustaceans the sperm, having an acrosome but no flagellum, meet the egg after extrusion and attach to it via a nuclear plasma process or a nuclear radiate arm. The decapod egg has no micropyle and the sperm penetrates into it by acrosomal reaction (Yang et al. Citation1998). In bivalve molluscs the sperm, having both flagellum and acrosome, is capable of maintaining movement for a long period. Here, therefore, there is provided adequate time for the sperm to search for an egg to fertilize. The bivalve mollusc egg is without a micropyle and the sperm’s entry into it is by acrosomal reaction and by a direct pull force exhibited by the egg (Li et al. Citation2005). Our study has shown that the sperm of P. esculenta has developed both an acrosome and flagellum. This facilitates a considerable period of movement. The P. esculenta egg has no micropyle and the pattern of sperm binding and penetration into the egg is quite different from that of both teleost fish and decapods but considerably similar to that of bivalve molluscs. The site where the sperm docks and penetrates into egg is randomly decided. This indicates that the sperm can cling and enter into the egg at any place on the egg’s surface. This is coincident with most bivalve molluscs.
The acrosomal filament, originating from the acrosome reaction, is the key to sperm penetration into the egg. In the oyster Crassostrea gigas (Thunberg, 1793), the acrosomal enzyme is released after the acrosome reaction. Globular actin in the subacrosomal space then integrates into a bundle of fibrous actin filaments, which can penetrate through the egg envelope to drive the sperm into the egg (Jiang et al. Citation2003). In another bivalve example, the Atlantic surf clam Spisula solidissima, the acrosomal membrane tends to fuse with the plasma membrane to form a series of vesicles after sperm contact. The acrosome then breaks up and releases hydrolyase and its other contents. Meanwhile, the globular actin in the subacrosomal space integrates into a bundle of fibrous actin filaments forming an acrosomal filament, which is used by the sperm to penetrate through vitelline membrane (Chen & Longo Citation1983). In P. agassizi, G. pugettensis and T. pyroides the acrosomal filament also plays a critical role in sperm penetration (Rice Citation1966). In P. esculenta the acrosomal reaction occurs immediately upon sperm and egg contact where the acrosomal enzyme is released to dissolve the egg envelope. Simultaneously, the acrosomal rod, comprised of silky fibres in the subacrosomal space, forms the acrosomal filament, which will penetrate through the egg membrane and drive the sperm into the egg.
The fertilization cone functions as an important assistant for sperm penetration. In S. solidissima, the acrosomal filament, after the penetration through the vitelline membrane, contacts microvilli on the surface of the plasma membrane. The microvilli elongate to encompass the sperm head and expand outwardly to form the fertilization cone and to draw the sperm into the egg (Chen & Longo Citation1983). In C. gigas when the acrosomal filament is penetrating through the egg envelope, the fertilization cone, derived from the egg membrane, protrudes to surround the acrosomal filament tightly and helps the sperm to penetrate into the egg (Jiang et al. Citation2003). In our study, the fertilization cone of P. esculenta is formed after the acrosomal filament has penetrated the egg. The end of the acrosomal filament anchors to the surface of the fertilization cone, which retracts to draw the sperm back into the egg.
We argue that, for this species, the cooperation between the acrosomal filament and the fertilization cone is able to facilitate a rapid penetration of sperm into the egg and improve the fertilization rate.
The formation and integration of male and felame pronuclei
The formation of the male and female pronuclei in P. esculenta is not synchronized. For the male, the sperm nucleus begins to decondense and expand at the end of the first meiosis and the male pronucleus is formed before the second meiosis is complete. In comparison, the female pronucleus is formed soon after the termination of the second meiosis. In Chlamys farreri (Jones et Preston, 1904), while the male pronucleus is formed a little earlier than the female pronucleus, its formation occurs after the completion of the second meiosis (Ren et al. Citation2000). In C. gigas, the male and female pronuclei are formed simultaneously after the extrusion of the second polar body (Ren et al. Citation1999). To date, the biological significance of synchronous and asynchronous formation of male and female pronuclei remains unclear.
Depending on the species, female and male pronuclei combine by either fusion or association. In fusion, the nuclear membranes of male and female pronuclei fuse after their integration. The zygote nucleus is then formed by the mixture of chromatins, which will condense into chromosomes and initiate the first mitosis process. In association, the nuclear membranes of male and female pronuclei do not fuse with each other but form a mosaic structure by digitation. Subsequently, the two sets of nucleus chromatins independently condense into chromosomes. After the nuclear envelopes disappear, the two sets of chromosomes move towards each other, forming zygote chromosome groups. Generally, the integration of male and female pronuclei in an animal whose mature egg is in the metaphase of the primary oocyte is by association (Yang et al. Citation1998). This has been confirmed by studies on the fertilization cytology in bivalve mollusc species, including Pinctada martensii (Dunker, 1872) (Shen et al. Citation1993), C. gigas (Ren et al. Citation1999), Crassostrea rivularis (Gould, 1861) (Shen et al. Citation1995), Tegillarca granosa (Linnaeus, 1758) (Sun et al. Citation2000), Ruditapes philippinarum (Adams & Reeve, 1850) (Bi et al. Citation2004) and Meretrix meretrix (Linnaeus, 1758) (Dong et al. Citation2007) and in crustacean species including Fenneropenaeus chinensis (Osbeck, 1765) (Kang et al. Citation2001) and Eriocheir sinensis H. Milne Edwards, 1853 (Du et al. Citation1992). In these species, sperm penetration uniformly occurs in the metaphase of the first meiosis, and female and male pronuclei combine with each other via association. However, there are exceptions. In C. farreri, although the sperm enters the egg in the metaphase of the first meiosis, male and female pronuclei integrate with each other by fusion instead of association (Ren et al. Citation2000). According to our research, the oocyte of P. esculenta also appears in the metaphase of the first meiosis when the sperm is penetrating the egg. Nevertheless, the pattern of male and female pronuclei combination is not by association but rather by fusion. This is consistent with the cases of three other sipuncula species, namely P. agassizi, G. pugettensis and T. pyroides (Rice Citation1966). Theoretically, the time for male and female pronuclei to form a zygote nucleus is short by fusion but relatively long by association (Liu & Yang Citation2002). We therefore believe that male and female pronuclei of P. esculenta combine with each other via fusion because, to some extent, this pattern shortens the fertilization process.
The process of the migration and integration of male and female pronuclei is probably correlated with microtubular activity in the egg and centriole activity in the sperm nucleus (Longo et al. Citation1993). The dynamic mechanism of the integration of male and female pronuclei needs to be studied from the perspective of cytoskeleton distribution in the egg and the physiological connections between male and female pronuclei.
Development of the polyspermic egg
There are two types of polyspermy, namely physiological polyspermy and pathological polyspermy. In physiological polyspermy, despite many sperm penetrating the same egg, there is only one sperm whose male pronucleus is able to combine with the female pronucleus to form a zygote nucleus. In this case, the fertilization and development processes can be normally conducted and other sperm are either absorbed by the egg and/or the formation of pronuclei in them is halted. As for pathological polyspermy, this is often the cause of early embryonic death (Chen et al. Citation2000). In marine invertebrate animals fertilizing in vitro, monospermy is dominant under normal circumstances while a small proportion of cases of polyspermy is also observed. Polyspermy has been observed in C. gigas (Longo et al. Citation1993), C. rivularis (Shen et al. Citation1995), C. farreri (Yang et al. Citation1999; Ren et al. Citation2000), R. philippinarum (Bi et al. Citation2004) and M. meretrix (Dong et al. Citation2007). In some cases, sperm that have entered into the same egg will all form pronuclei, resulting in the appearance of a multipolar spindle. In a manner analogous to the bivalve molluscs mentioned, polyspermy is also observed in some eggs of P. esculenta where all the sperm nuclei are able to form pronuclei and multipolar spindles. Multiple chromosomes can even be observed. It is generally acknowledged that this type of polyspermy is termed pathological polyspermy, which will give rise to an abnormal embryo or a non-diploid embryo because the egg lacks the mechanism for making an even allocation of chromosomes.
In summary, the current research has corroborated our viewpoint that the rapid sperm entry into eggs and the short time needed for male and female pronuclei combination are of great significance in relation to improving fertilization rate under severe conditions. The fertilization cytology of P. esculenta is characterized by: (1) the egg coming into maturation in the metaphase of the first meiosis; (2) the cooperation of the acrosomal filament and the fertilization cone being the driving force for sperm entry into eggs; (3) the existence of abnormal phenomena such as polyspermy, multiple pronuclei and multipolar spindle; and (4) the male pronucleus is formed earlier than the female pronucleus, and their combination is by fusion.
Acknowledgements
We are indebted to all members in our laboratory for their enlightening discussion. This work was supported by the Science Technology Department of Zhejiang Province under Grant No. 2011C12013 and the National Natural Science Foundation of China under Grant Nos. 31272642 and 41276151. In addition, this work was sponsored by K.C. Wong Magna Fund in Ningbo University.
References
- Adrianov AV, Maiorova AS, Malakhov VV. 2002. Microscopic anatomy and ultrastructure of nephridium in the sipunculan Thysanocardia nigra Ikeda, 1904 from the Sea of Japan. Russian Journal of Marine Biology 28:19–29. doi:10.1023/A:1014425529953.
- Bi K, Bao ZM, Huang XT, Wang J, Fang JG, Liu H. 2004. Cytological observations on fertilization and early embryonic development in Ruditapes philippinarum. Journal of Fisheries of China 28:623–627.
- Chen DY, Longo FJ. 1983. Sperm nuclear dispersion coordinate with meiotic maturation in fertilized Spisula solidissima egg. Developmental Biology 99:217–224. doi:10.1016/0012-1606(83)90270-1.
- Chen DY, Sun QY, Li YG. 2000. Fertilization biology-fertilization mechanism and reproduction engineering. Beijing: Science Press. pp. 22–46.
- Chen H, Lin GW, Ke CH, Wang XC, Chen W. 2009. Comparison of sperm ultrastructural in Sipunculus nudus and Phascolosoma esculenta. Journal of Oceanography in Taiwan Strait 28:467–471. doi:10.3969/J.ISSN.1000-8160.2009.04.004.
- Dong YH, Lin ZH, Chai XL, Lu RM, Xiao GQ. 2007. Cytological observation on fertilization and early embryonic development in the clam Meretrix meretrix. Acta Zoologica Sinica 53:700–709.
- Du NS, Lai W, An Y, Jiang HW. 1992. Studies on the cytology of fertilization in the Chinese mitten crab, Eriocheir sinensis. Science in China. Series B: Chemistry 3:260–267.
- Gerould JH. 1907. The development of Phascolosoma. Zoologische Jahrbücher, Anatomie 23:77–162.
- Gu XY, Zhu JQ, Xu SJ, You ZJ, Wang W. 2009. Oogenesis of Phascolosoma esculenta: Histological study. Oceanologia Et Limnologia Sinica 40:283–288.
- Jiang M, Ru SG, Tao XR, Fan RQ, Wang JF, Wang RC. 2003. Ultrastructure change of the sperm of Crassostrea gigas during egg penetrating. Journal of Ocean University of Qingdao 33:551–556.
- Kang XJ, Wang SA, Du NS, Lai W. 2001. Cytological changes of sperm and egg nuclei during fertilization in Penaeus chinensis. Acta Zoologica Sinica 47:182–189.
- Li FL. 1989. Studies on the genus Phascolosoma (Sipuncula) off the China coasts. Journal of Ocean University of Qingdao 19:78–90.
- Li YR, Que HY, Zhang GF. 2005. Progress in fertilization biology in marine bivalves. Marine Science 29:68–72.
- Liu XZ, Yang WX. 2002. Recent progresses of study on fertilization cytology in teleost. Donghai Marine Science 20:36–41.
- Long LL, Lu MM, Zhu JQ. 2014a. Distribution and structural features of nephridial cilia in the sipunculan Phascolosoma esculenta. Chinese Journal of Zoology 49:253–260.
- Long LL, Sheng Z, Lu MM, Ding LF, Zhu JQ. 2014b. Structural features of musculature in the nephridium of Phascolosoma esculenta. Journal of Biology 31:9–12. doi:10.3969/j.issn.2095-1736.2014.02.009.
- Longo FJ, Mathews L, Hedgecock D. 1993. Morphogenesis of maternal and paternal genomes in fertilized oyster eggs (Crassostrea gigas): Effects of cytochalasin B at different periods during meiotic maturation. Biological Bulletin 185:197–214.
- Maiorova AS, Adrianov AV. 2007. Ultrastructural observations on spermiogenesis in the peanut worm, Themiste pyroides (Chamberlin, 1920) (Sipuncula; Sipunculidea). Invertebrate Reproduction & Development 50:173–180. doi:10.1080/07924259.2007.9652243.
- Maiorova AS, Adrianov AV. 2010. Reproduction and development of common species of peanut worms (Sipuncula) from the Sea of Japan. Russian Journal of Marine Biology 36:1–15. doi:10.1134/S1063074010010013.
- Mary ER. 1970. A sexual reproduction in a sipunculan worm. Science 167:1618–1620. doi:10.1126/science.167.3925.1618.
- Mu DL, Long LL, Lu MM, Ding LF, Zhu JQ. 2013. Morphological features of nephridium in the sipunculan Phascolosoma esculenta under light and electron microscopy. Oceanologia Et Limnologia Sinica 44:996–1002.
- Ren SL, Wang DX, Sheng XZ, Wang RC, Gao DY. 2000. Cytological observation on fertilization of Chlamys farreri. Transactions of Oceanology and Limnology 1:24–29.
- Ren SL, Wang DX, Wang RC, Zhang XL. 1999. Sperm nucleus dispersion and meiotic maturation in fertilized eggs of pacific oyster, Crassostrea gigas (Thunberg). Transactions of Oceanology and Limnology 1:34–39.
- Reunov AA, Rice ME. 1993. Ultrastructural observations on spermatogenesis in Phascolion cryptum (Sipuncula). Transactions of the American Microscopical Society 112:195–207.
- Rice ME. 1966. Reproductive biology and development in Sipuncula. Doctor dissertation, Seattle: University of Washionton, 46–66.
- Shen YP, Liu D, Jiang HB, Ye L, Zhang XY. 1993. Cytological observation of the fertilization in Pinctada fucata. Journal of Wuhan University (Natural Science Edition) 39:115–120.
- Shen YP, Liu T, Jiang HB, Zhang XY, Chen XH, Ye L, Wu B. 1995. A cytological study on fertilization of eggs in Ostrea rivularis gould. Journal of Wuhan University (Natural Science Edition) 41:482–486.
- Sun HL, Fang JG, Wang QY, Zhang JH, Liang XM, Yan JP, Kong J, Yang AG. 2000. Cytological observation on fertilization of Tegillarca granosa with fluorescent microscope. Journal of Fisheries of China 24:104–108.
- Yang AG, Wang QY, Kong J, Liu ZH, Liu P, Li F, Wang RC, Jiang M. 1999. Cytological study on meiosis of fertilized eggs from Chlamys farreri. Journal of Fisheries of China 6:96–98.
- Yang DJ, Sun SC. 2006. Marine invertebrate zoology. Qingdao: China Ocean University Press. pp. 318–322.
- Yang WX, Jiang NC, Lu JP. 1998. A review of fertilization cytology in decapoda crustacean. Donghai Marine Science 16:57–63.
- Zhu JQ, Wang W, Ding LF. 2012. Ultrastructural observation on oogenesis, vitellogenesis and vitelline membrane formation during yolk-synthesizing stage in Phascolosoma esculenta. Oceanologia Et Limnologia Sinica 43:870–876.
- Zhu JQ, Wang W, Xu SJ, Zeng HX. 2007. Spermatogenesis and sperm morphology in Phascolosoma esculenta. Acta Zoologica Sinica 53:733–741.
- Zhu JQ, Wang W, Ying XP, Xu SJ, Zeng HY, Chen HQ. 2008. Cytological changes fertilization and early cleavage in Phascolosoma esculenta. Acta Zoologica Sinica 54:290–298.