Abstract
Temporal changes in adult sex ratio of animal populations might be due to differences in movements, survival or detection probabilities. We used data from an intensive capture–mark–recapture study of 720 lizards at the islet of Aire (Balearic Islands, Spain) to investigate the demographic mechanisms underlying the spring uneven sex ratio. We simultaneously estimated survival (f), the proportion of transient animals (p) and the probability of recapture (p) of lizards at the study plot. We then estimated population size using open population models for individually based data and compared these with the observed values. Results indicated that males had a higher probability of recapture than females, but this was not sufficient to generate the observed male-biased sex ratio. The proportion of transient males decreased at the end of spring in parallel with the end of the blooming period of the dead horse arum, Helicodiceros muscivorus, a short-lasting food and thermoregulation resource for lizards during spring. Changes in the proportion of transients suggested that sex-dependent movements, most likely linked to a monopolising behaviour of this plant resource, were responsible for the observed difference in the number of males and females. Our results reveal how the interplay of behavioural and ecological factors explains short-term changes in population dynamics and shapes the movement patterns within the island.
Introduction
The sex ratio of reptile and amphibian populations frequently appears skewed (Hailey Citation1990; Madsen & Shine Citation1993; Arntzen Citation2002; Dreiss et al. Citation2010; Loman & Madsen Citation2010; Okada et al. Citation2011). Uneven sex ratio in vertebrates is often explained by a differential mortality between sexes (see for example Tavecchia et al. Citation2008). However, the observed sex ratio might be due to a temporal dynamic, for example when individuals of a particular sex have an advantage competing for a resource limited in time or space (Pérez-Mellado et al. Citation2007). Uneven sex ratio might also arise by a sampling bias if one sex is easier to observe or capture than the other (Rose Citation1981).
Here we investigate the mechanisms responsible for the observed adult sex ratio of the Balearic lizard, Podarcis lilfordi (Günther, 1874), an endemic lacertid inhabiting coastal islets of Menorca, Mallorca and Cabrera Archipelago (Balearic Islands, Spain, Pérez-Mellado Citation1998). Podarcis lilfordi is an omnivorous species (Pérez-Mellado & Corti Citation1993) that includes several plant species in its diet, promoting the emergence of multiple plant–lizard interactions (Sáez & Traveset Citation1995; Pérez-Mellado & Traveset Citation1999). This is the case for the complex interaction described between the dead horse arum Dracunculus muscivorus (Araceae) and the Balearic lizard at Aire, a coastal islet of Menorca (Pérez-Mellado et al. Citation2000, Citation2006, Citation2007). The dead horse arum is a Tyrrhenian endemic of the Western Mediterranean region (Corsica, Sardinia and Balearic Islands, Roselló & Sáez Citation1997), which is pollinated by flies (Diptera) attracted by the peculiar scent of rotten meat and heat produced by the plant (Bogner & Nicolson Citation1991). The blooming period of the plants is concentrated in the early spring, and lasts only from April to the beginning of May. Blooming arums are intensively visited by lizards, as excellent perching sites for thermoregulation, for ambush foraging of flying pollinators, and for the capture of trapped flies inside the plant’s floral chambers. During the blooming period, adult individuals actively defend inflorescences (Pérez-Mellado et al. Citation2007). In P. lilfordi, males are larger than females and, generally, more successful monopolising the flowers that are employed as a resource site for capturing flies and as a warm substrate for thermoregulation (Pérez-Mellado et al. Citation2007). As a consequence, in areas with higher plant density, during early spring, the lizard population is skewed toward males (Pérez-Mellado et al. Citation2007) and male lizards occupy areas of higher plant densities (Pérez-Mellado et al. Citation2013). Then, the sex ratio changes toward parity over the spring, when the flowering season of the dead horse arum ends (Pérez-Mellado et al. Citation2013).
It is not clear if this variability of observed sex ratios reflects a change in the recapture probability between groups (males and females) and over time (hypothesis A), an increased mortality of males (hypothesis B) or an increased movement of female lizards throughout the study area (hypothesis C). These three hypotheses, which are not mutually exclusive, generate contrasting predictions for the patterns of survival and recapture probability between groups and/or across time. If changes reflect a difference in recapture probability, we would expect males to have a higher recapture probability than females and this difference to decrease over time (hypothesis A). If the increased proportion of females after blooming ends is due to a higher mortality of males, we would expect local survival of males to be lower than survival of females, or to decrease over time (hypothesis B). If the proportion of females increases due to new female lizards coming into the study area, we would expect no survival differences between the two groups (hypothesis C). This hypothesis would indicate that a temporal change in the sex ratio was due to a despotic distribution across sexes mediated by the short temporal availability of a food resource, the dead horse arum.
To test which demographic mechanism is most likely to be responsible for the skewed sex ratio of lizards, a capture–mark–recapture study was conducted throughout the entire spring of 2004 in the area with the highest recorded density of dead horse arums (Pérez-Mellado et al. Citation2007, Citation2013).
Material and methods
Short-term survival and recapture processes
We delimited a study plot of 50 × 50 m in the centre of Aire Island, in the area of highest density of D. muscivorus (Pérez-Mellado et al. Citation2007). Lizards were captured within this area in 28 capture–mark–recapture sessions (k = 28) unequally distributed over the period from 13 April to 15 July 2004 (). During the study period, we collected 720 encounter histories of adults (364 males and 356 females). Animals were caught by noosing and individually marked using a mixed method of natural marks (total or partial loss of toes was recorded in around 60% of individuals Pérez-Mellado Citation1998; Pérez-Mellado et al. Citation2007), toe clipping (40%), and photographic identification of all individuals based on the scale arrangement of the anterior ventral region (Perera & Pérez-Mellado Citation2004a). We restricted the analysis to adult lizards, because the number of marked juveniles was too low. For the analyses, we assumed no detectable effect of toe clipping or natural toe loss. Survival and recapture probabilities can be simultaneously estimated using a capture–recapture model (Burnham et al. Citation1987). We first used all available data (k = 28) to investigate survival and recapture processes. However, the interval length between capture–recapture occasions was mainly 1 day, with the exception of two longer periods during May and between June and July. Capture–recapture models are available for unequal intervals, but daily survival is expected to be very close to 1 and might create numerical problems with a full time-dependent model. A partial solution is to investigate the temporal variation as a trend over time, using linear constraints on survival, in the form:
where T indexes the recapture occasions, α is the average value of survival and b is the slope of the linear relationship. If local survival were decreasing over time as site fidelity diminished, we would have expected b < 0. In addition, if site fidelity were decreasing more rapidly in males than females, we would have expected bmales ≪ bfemales. Using the software program U_CARE (Choquet et al. Citation2002), we assessed the adequacy of a model assuming that all parameters were time dependent and different in males and females. The goodness-of-fit test indicated the need to include additional parameters to account for the presence of transient animals and the heterogeneity of recapture probability (see results). This general model was then simplified by eliminating non-relevant effects using the adjusted Akaike Information Criterion (AICc; Burnham & Anderson Citation1998). Estimates of survival and recapture probabilities were derived by maximum likelihood from the retained model, i.e. the one with the lowest AICc value. When the selection procedure indicated that several models were equally plausible (Delta Akaike Information Criterion, DAIC within two points), the estimates were derived by model averaging techniques (Burnham & Anderson Citation1998). We used the software MARK v. 5.1 for model fitting and parameter estimates (White & Burnham Citation1999).
Table I. Intervals between the capture–recapture occasions retained for the two analyses. The number refers to the interval in days between the current and the previous occasion. K = number of occasions retained; – = first occasion considered.
Population size and sex ratio
Capture–recapture data can be used to estimate population size and its variance. However, in the presence of survival or recapture heterogeneity, capture–recapture models would deliver biased estimates of population sizes (Kendall Citation1999). We used results from the above analyses to detect a period of time during which individuals can be assumed to be homogeneous in their capture probability (). This second sub-set was then used to estimate the population size, its variance and recruitment probability using the procedure POPAN in MARK v. 5.1 for open populations (Schwarz & Anderson Citation2001). This analytical approach estimates survival, recapture and a super-population size denoted by N, a cumulated value made for all possible available animals (Schwarz & Anderson Citation2001). Together with these parameters, the procedure estimates the probability, bi, which represents the probability that an animal from this hypothetical super-population would enter the population between occasions i and i + 1 (Schwarz & Anderson Citation2001). These probabilities sum to 1, and the population size at a given time i is estimated as a derived parameter as Nbi. The standard errors of these derived parameters are calculated by delta-methods (Morgan Citation2000). We began model selection from a simple model that extends the Cormack–Jolly–Seber model (CJS) to multiple groups in which recapture and survival varied over time in males and females. We modelled three main parameters: the probability of recapture, noted “p”, the probability of local daily survival of a newly marked lizard (only for males), noted “f´”, and the probability of local daily survival of an already marked lizard, noted “f”. Following Tavecchia et al. (Citation2008), the effects considered in each model were noted as follows: “m” denotes the trap-dependent effect, “t” denotes the time and “s” denotes the sex. The statistical interaction between effects was noted as “*”, while the symbol “+” was used for a parallel variation over time, i.e. the absence of an interaction term.
Results
Proportions of captured lizards
The proportion of females among captured lizards increased gradually with time (). In April, captured lizards were mainly males (G test, G = 15.68, p < 0.01), while in June and July the proportion of males and females was similar (June: G = 5.24, p > 0.05; July: G = 2.95, p > 0.05). We wanted to confirm whether these observational findings were also supported once the probabilities of recapture of males and females were taken into account.
Survival and recapture probabilities
The goodness-of-fit (GOF) test of the CJS model confirmed an important presence of transient animals, i.e. individuals seen only at marking, among males (Z = 3.25, p = 0.001) but a less important presence among females (Z = 1.66, p = 0.05). The transient effect can also be interpreted as a negative effect of marking. However, in this case, it is not clear why it should be present in males but not in females. The apparent transient effect was accounted for by including extra parameters for the newly marked individuals (Pradel et al. Citation1997; Tavecchia et al. Citation2008). Moreover, the GOF test revealed that some lizards had higher probability of being recaptured than expected by chance. This was true for males and females (males: Z = −12.00, p = 0.000, females: Z = −7.84, p = 0.000).
We thus built a new starting model assuming extra parameters to account for transients in males and for trap-heterogeneity in both groups. The remaining extra-binomial deviance was corrected using a scale parameter ĉ, calculated as the GOF chi-square statistic over its degree of freedom (c2142 = 256, p = 0.00, ĉ = 1.80). The starting model, which was denoted f’(t)f(t*s)p(t*s*m), assumed an effect of time, sex and their statistical interactions for all parameters. In addition, it includes a trap-dependent effect for both sexes. A simpler model without the interaction terms in the probability of recaptures was clearly preferred (), but all other effects were retained.
Table II. Modes and their estimates of daily survival in male and female lizards, f, and recapture probability, p. Model notation: f’ = newly marked male survival; s = sex; t = time; m = trap-dependent effect; * = interactions between effects; + = additive relationship between effects; t = trend over time; np = number of parameters. In model notation, ‘s’ to distinguish it from the daily survival denoted ‘f’ in the previous analysis. QAICc: Quasi-likelihood adjusted Akaike Information Criterion; DQAICc: Delta Quasi-likelihood adjusted Akaike Information Criterion; QDeviance: Quasi-likelihood Deviance.
As expected for the capture protocol, the recapture probability varied over time, ranging from 0.04 to 0.72 in “trap-happy” animals and from 0.01 to 0.37 for the other animals (). The recapture probability of males (average value: 0.23) was about 30% higher than that of females (average value: 0.07). Several survival probabilities were estimated as 1.0, a consequence of the short time interval considered (A); a model with a constant value of this parameter for both sexes was preferred (). Model selection revealed a significant sex difference in survival (c21 = 9.255, p = 0.002). Males had a higher local daily survival probability than females (mean ± SE = 0.978 ± 0.0019 for males and 0.964 ± 0.0029 for females, estimated from model f(s)p(t + s + m)). Models with a linear constraint as in Equation 1 had a lower AIC value. In particular, the AIC value of the model f(T)p(t + s + m), assuming a trend over time, was lower than the one assuming a constant time effect (DAIC = 38.95). The value of b was negative and significantly different from 0 (b = −0.089, 95%CI = −0.095, −0.082; c21 = 33.47, p = 0.001). Finally, the AIC values indicated that male site fidelity abated faster than females (bmales ≪ bfemales; bmales = −0.10, bfemales = −0.05).
Figure 2. A, A model assuming a linear constraint on survival as in Equation1 indicates that local daily survival decreased over time (solid line). Bars are the 95% confidence interval. B, Proportion of transient males, i.e. animals seen only at marking. Solid dots are from model f’(t) f(t*s) p(t + s + m) while the solid line indicates the expected values from the model f’(T) f(T*s) p(t + s + m).
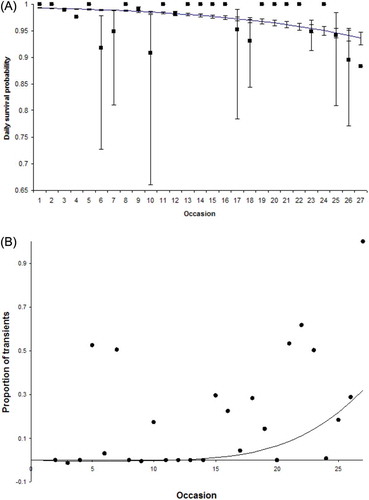
An interesting result is that the b parameter for newly marked males is significantly higher than for already marked males, indicating that the proportion of transient animals increased with time (bnewly marked males = −0.24, balready marked males = −0.097; c21 = 7.022, p = 0.03, B). The average survival estimated on a daily basis suggested monthly local survival of 0.51 and 0.33 for males and females, respectively.
Sex ratio and population size
The presence of transient males and of trap-happy animals prevents the estimation of population size using conventional capture–recapture methods (Kendall Citation1999). We thus constrained the analysis to a period in which this heterogeneity can be assumed to be minimal. According to the GOF, transient animals were present only among males from early June onwards. We thus restricted the estimate of population size to the month of April (13–27 April), analysing the encounter histories of 173 males and 105 females. As expected, the GOF test this time indicated homogeneity of survival (males: Z: −1.803, p = 0.964; females: Z: 0.998, p = 0.159), but there was still some heterogeneity in the recapture probability of males (males: Z: −3.1851, p = 0.001; females: Z: −1.177, p = 0.239). The distribution of gap lengths between two consecutive recaptures, i.e. the number of consecutive capture failures between two recapture events, indicates that some animals were absent or were not captured on seven or more consecutive occasions (). This type of encounter history is not expected under the hypothesis that all individuals have the same recapture probability, and contributed largely to the significance of the GOF test, behaving as outliers.
Figure 3. Frequency of gaps, i.e. number of consecutive zeros between two recapture events, in April capture–recapture data.
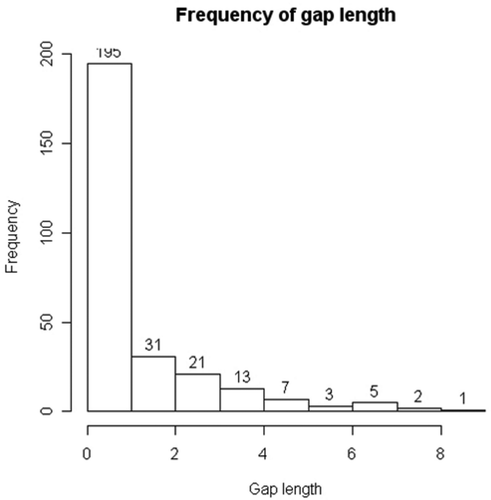
We next eliminated eight individuals (out of 278) with more than six consecutive capture failures between two capture events (50% of total time). Eliminating individuals that were absent on many consecutive occasions might artificially bias survival downward. However, in our case, survival was already very high due to the short time interval, and its value did not change substantially after eliminating these individuals (results not shown). The remaining data showed no significant heterogeneity across individuals in survival (males: Z = −1.84, p = 0.07; females: Z = 1.0796, p = 0.14) or recapture (males: Z = −1.88, p = 0.06; females: Z = −1.05, p = 0.29). Data were then suitable for calculation of the male and female numbers, their variance and the recruitment probability into the area during April. Model selection was not fully conclusive, as two models (models f(sex) p(t*sex)b(t + sex) and f(sex) p(t + sex) b(t + sex)) had very similar AICc values (). Because these two models had by far the highest model likelihood, the proportion of females in the study area was calculated as the averaged value of the derived estimates from these two models (). Final estimates indicated that the proportion of females to males increased during April from 0.23 to 0.40 ().
Table III. Models of population size and recruitment, b, during the month of April Model notation: f’ = newly marked male survival; s = sex; t = time; m = trap-dependent effect; * = interactions between effects; + = additive relationship between effects; t = trend over time; np = number of parameters. In model notation, ‘s’ to distinguish it from the daily survival denoted ‘f’ in the previous analysis (see more details in the text).
Figure 4. The proportion of females in the study area during the month of April, estimated by averaging the derived estimates from model f(s) p(t + s) b(t + s) and f(s) p(t*s) b(t + s). The number of individuals estimated to be present at a given time is estimated as derived parameters (see text for details).
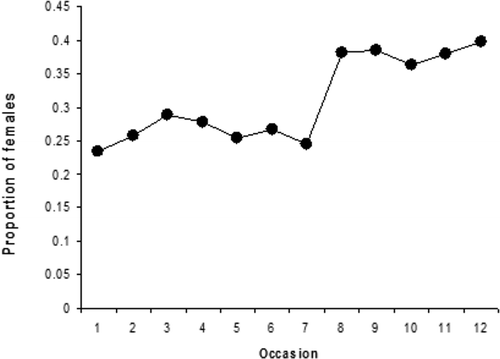
Discussion
Adult sex ratio and short-term dynamics
Our results rejected the hypothesis that the skewed sex ratio was due to a difference in recapture probability (hypothesis A). While we showed that males had about 30% more chance to be recaptured than females, this difference was constant over time and cannot explain an increase in the observed number of females over the spring. This result does not depend on the data set considered, as the interaction term between sex and time effects was never retained. This is not surprising, as data sets were not independent. On the other hand, it shows that this result was robust no matter how we considered the encounter histories.
The second hypothesis (hypothesis B), that males had a higher mortality than females and that this difference increased over time, was only partially verified. Contrary to the predictions, males had a lower mortality than females, but the proportion of transient lizards increased during the spring months with the decrease in blooming of H. muscivorus.
The second analysis favoured the hypothesis that the proportion of females increased due to new females coming into the study area (hypothesis C). Hypotheses A, B and C were not mutually exclusive; we conclude that the observed pattern of sex ratio is the result of a combination of increasingly lower site fidelity of males and a higher immigration into the study area of new females. Note, however, that female local survival also decreased during the study, which suggests that the monopolisation of dead horse arum is decreasing over the spring months. In the early summer (June–July), the population reached an “equilibrium” which we believe to be the state without the additional resource of flowering arum. Hence, the results suggested that at equilibrium, females are more mobile than males. During June, lizards intensively exploit ripening fruits of H. muscivorus, but in this case, we did not observe a higher consumption by males or females (Pérez-Mellado et al. Citation2006, Citation2007; Pérez-Mellado unpubl. data). The initial hypothesis that local survival decreases from April to July is supported for both sexes (). Moreover, the interaction between male status and the b parameters of Equation 1 from the first analysis indicated that the proportion of site-faithful males diminished over the study. This result again supports the hypothesis that males defend blooming plants during the dead horse arum flowering peak (Pérez-Mellado et al. Citation2007). This agrees with the hypothesis that movement patterns, and not differential mortality or catchability, are responsible for the observed changes in the sex ratio, and that during horse arum blooming males behave despotically, excluding females from the temporal resource. Nevertheless, site tenacity seems to abate early in June (), while the flowering peak ends at the beginning of May.
The capture–recapture framework and heterogeneity among individuals
The use of a short interval (1 day) pushed survival estimates to the upper boundary. When estimates reach upper limits, confidence intervals and the number of estimable parameters in the model cannot be easily calculated. Also, the long gaps after occasions 12 and 23 made it difficult to estimate a clear trend over time. Despite this, several robust results emerged from all analyses. Goodness-of-fit tests indicated the presence of transient males, i.e. animals seen only at marking. This might indicate that marking is harmful. However, this should apply only to males and should have a positive trend over time. If a negative effect of toe clipping cannot be excluded, it is unlikely that marking is harmful only for males (see above). Toe-clipping was extensively used as a marking technique in several lizard populations without deleterious effects (Dodd Citation1993; Langkilde & Shine Citation2006). In addition, natural toe-clipping is extremely common in several populations of the Balearic lizard, particularly in males, without any detectable effect (Pérez-Mellado unpubl. data). Finally, in our analysis, only a fraction of the animals were toe-clipped because nearly 60% had natural marks (natural toe loss).
Assuming that the apparent transient effect in males is not due to the marking method, it indicates that a sub-group of male lizards is behaving as “resident males” while another group is behaving as “transient”. The presence of heterogeneity in survival (transient effect) and recapture (trap-happiness) must be expected, especially in large data sets. However, this type of heterogeneity cannot easily be incorporated in capture–recapture models for estimating population size in open populations. One possibility would be to estimate population size within homogeneous groups, e.g. resident males and females, as we showed here, but boundary estimates and small sample size might complicate the task.
Another interesting result of our analyses is that males have a higher survival probability than females. This was clearly indicated by both analyses and cannot be due to the interval chosen. A difference in survival might be a consequence of the larger average body size of males, but could also be attributable to their enhanced site fidelity because models do not distinguish between survival and permanent emigration. Therefore, the higher survival probability of males can be linked to their priority in the use of blooming dead horse arums, an important resource for thermoregulation and an excellent foraging perch (Pérez-Mellado et al. Citation2007; Pérez-Mellado unpubl. data).
Temporal resources and population dynamics
As young lizards are rarely observed on dead horse arums (Pérez-Mellado et al. Citation2007), it appears that plants have more impact on the structure and dynamics of consumers than vice versa (Crawley Citation1993). Dead horse arum on Aire Island seems to strongly influence the spatial distribution of lizards and sex ratios in the areas of higher plant density. We showed that the sex ratios and movements of lizards are clearly linked with the presence of the dead horse arum during its blooming period. Our results confirm that males are prone to stay close to blooming plants, occupying the study plot area in a significantly higher proportion than females. Then, after the flowering period has ended, males tend to move to other areas of the island, while females come to the study plot, equilibrating the sex ratio. The analysis of space use by males and females and the construction of individual home ranges confirm these findings and indicate a clear establishment of males’ home ranges in areas with higher dead horse arum densities (Pérez-Mellado et al. Citation2013).
Taking into account that the breeding season and copulations take place during the whole spring and summer in P. lilfordi (Castilla & Bauwens Citation2000; Perera & Pérez-Mellado Citation2004b), and that we were unable to detect any other change in available resources at the study plot during spring and summer, the most parsimonious explanation for our results is that site fidelity of adult males decreases as a consequence of the end of the flowering of dead horse arums. Hence, our study is an example of how an ephemeral resource can influence the short-term spatial distribution and population structure of a lizard species. Differences in plant densities among areas of Aire Island (Pérez-Mellado et al. Citation2007) are apparently enough to promote significant movements of adult lizards and small-scale differences in the sex and age structure of the population.
Acknowledgements
This work was granted with a financial aid from the Institut Menorquí d’Estudis (Mahón, Menorca Island) and with the grants REN2003-08432-CO2-02 from the Ministry of Education and Science during fieldwork, and CGL2009-12926-C02-02, BFU2009-09359 and CGL2012-39850-CO2-02 from the Spanish Ministry of Science and Innovation during the preparation of the manuscript. The ringers from “Piccole Isole” project provided logistic support, especially the coordinator, Raúl Escandell. Joaquín Ensesa and Cala Torret Diving Center also provided important logistical support. Carlos Herrero, Nùria Riera, Isabel Catalán, Anna Perera and Toni Escandell helped during fieldwork. The capture and handling of lizards was done with an official permit of scientific capture (ref. 29/2004) from the Servei de Protecció d’Espècies de la Conselleria de Medi Ambient del Govern de les Illes Balears (issued 5 March 2004) and complies with the current laws of Spain. Three reviewers made very useful comments to a first draft of the manuscript.
References
- Arntzen JW. 2002. Seasonal variation in sex ratio and asynchronous presence at ponds of male and female triturus newts. Journal of Herpetology 36:30–35. doi:10.1670/0022-1511(2002)036[0030:SVISRA]2.0.CO;2.
- Bogner J, Nicolson DH. 1991. A revised classification of Araceae with dichotomous keys. Willdenowia 21:35–50.
- Burnham KP, Anderson DR. 1998. Model selection and inference. A practical information-theoretic approach. New York: Springer.
- Burnham KP, Anderson DR, White GC, Brownie C, Pollock KH. 1987. Design and analysis methods for fish survival experiments based on release-recapture. Monograph 5. Bethesda: American Fisheries Society.
- Castilla AM, Bauwens D. 2000. Reproductive characteristics of the island Lacertid lizard Podarcis lilfordi. Journal of Herpetology 34:390–396. doi:10.2307/1565362.
- Choquet R, Reboulet AM, Lebreton J, Gimenez O, Pradel R. n.d. 2002. U_Care 2.2: User’s Manual http://ftp.cefe.cnrs.fr/biom/Soft-CR/. Paris: CEFE-CNRS. 1.0 ( Multistate SURvival Generalized Estimation).
- Crawley MJ. 1993. On the consequences of herbivory - comment. Evolutionary Ecology 7:124–125. doi:10.1007/BF01237739.
- Dodd CK. 1993. The effects of toe-clipping on sprint performance of the lizard Cnemidophorus sexlineatus. Journal of Herpetology 27:209–213. doi:10.2307/1564938.
- Dreiss AN, Cote J, Richard M, Federici P, Clobert J. 2010. Age- and sex-specific response to population density and sex ratio. Behavioral Ecology 21:356–364. doi:10.1093/beheco/arp198.
- Hailey A. 1990. Adult survival and recruitment and the explanation of an uneven sex ratio in a tortoise population. Canadian Journal of Zoology 68:547–555. doi:10.1139/z90-080.
- Kendall WL. 1999. Robustness of closed capture–recapture methods to violations of the closure assumption. Ecology 80:2517–2525.
- Langkilde T, Shine R. 2006. How much stress do researchers inflict on their study animals? A case study using the scincid lizard, Eulamprus heatwolei. Journal of Experimental Biology 209:1035–1043. doi:10.1242/jeb.02112.
- Loman J, Madsen T. 2010. Sex ratio of breeding Common toads (Bufo bufo)- influence of survival and skipped breeding. Amphibia-Reptilia 31:509–524. doi:10.1163/017353710X524705.
- Madsen T, Shine R. 1993. Temporal variability in sexual selection acting on reproductive tactics and body size in male snakes. The American Naturalist 141:167–171. doi:10.1086/an.1993.141.issue-1.
- Morgan BJT. 2000. Applied stochastic modelling. London: Arnold.
- Okada Y, Yabe T, Oda S. 2011. Interpopulation variation in sex ratio of the Japanese Pond Turtle Mauremys japonica (Reptilia: Geoemydidae). Current Herpetology 30:53–61. doi:10.5358/hsj.30.53.
- Perera A, Pérez-Mellado V. 2004a. Photographic identification as a noninvasive marking technique for lacertid lizards. Herpetological Review 35:349–350.
- Perera A, Pérez-Mellado V. 2004b. Ausencia de plasticidad fenotípica en las estrategias reproductoras de la Lagartija balear, Podarcis lilfordi (Squamata, Lacertidae). Revista de Menorca 86:159–171.
- Pérez-Mellado V. 1998. Podarcis lilfordi (Günther, 1874). In: Salvador A, editor. Reptiles. Fauna Ibérica, vol. 10. Madrid: Museo Nacional de Ciencias Naturales, CSIC, pp. 272–282.
- Pérez-Mellado V, Cortázar G, López-Vicente M, Perera A, Sillero N. 2000. Interactions between the Balearic lizard, Podarcis lilfordi and the plant Dracunculus muscivorus. Amphibia-Reptilia 21:223–226.
- Pérez-Mellado V, Corti C. 1993. Dietary adaptations and herbivory in lacertid lizards of the genus Podarcis from western Mediterranean islands (Reptilia: Sauria). Bonner Zoologische Beitrage 44:193–220.
- Pérez-Mellado V, García Díez T, Hernández Estévez JA, Herrero Ayuso C, Riera N, Catalán I. 2013. El uso del espacio en la lagartija balear Podarcis lilfordi. Factores causales en la isla del Aire. Revista de Menorca 92:189–218.
- Pérez-Mellado V, Riera N, Hernández-Estévez JA, Piccolo V, Potter C. 2007. A complex case of interaction between lizards and plants. The dead horse arum (Dracunculus muscivorus) and the Balearic lizard (Podarcis lilfordi). In: Corti C, Lo Cascio P, Baiggini M, editors. Mainland and insular lacertid lizards: a Mediterranean perspective. Firenze: Firenze University Press, pp. 133–160.
- Pérez-Mellado V, Riera N, Piccolo V, Potter C. 2006. Mutualismo en ecosistemas insulares: La interacción de dos endemismos mediterráneos, la Rapa mosquera, Dracunculus muscivorus y la lagartija balear, Podarcis lilfordi. Revista de Menorca 87:45–77.
- Pérez-Mellado V, Traveset A. 1999. Relationships between plants and Mediterranean lizards. Natura Croatica 8:275–285.
- Pradel R, Hines JE, Lebreton J, Nichols JD. 1997. Capture-recapture survival models taking account of transients. Biometrics 53:60–72. doi:10.2307/2533097.
- Rose B. 1981. Factors affecting activity in Sceloporus virgatus. Ecology 62:706–716. doi:10.2307/1937739.
- Roselló JA, Sáez L. 1997. Notes on some Balearic Araceae. Acta Botanica Barcinonensia 44:169–174.
- Sáez E, Traveset A. 1995. Fruit and nectar feeding by Podarcis lilfordi (Lacertidae) on Cabrera Archipelago (Balearic Islands). Herpetological Review 26:121–123.
- Schwarz CJ, Anderson AN. 2001. Jolly-Seber models in MARK. In: MARK. A gentle introduction. Online. Available: http://www.phidot.org/software/mark/docs/book/pdf/chap13.pdf. Accessed March 2009 5.
- Tavecchia G, Mínguez E, De León A, Louzao M, Oró D. 2008. Living close, doing differently: small-scale asynchrony in demography of two species of seabirds. Ecology 89:77–85. doi:10.1890/06-0326.1.
- White GC, Burnham KP. 1999. Program MARK: Survival estimation from populations of marked animals. Bird Study 46 (sup001):S120–S139. doi:10.1080/00063659909477239.