Abstract
The development of the saccular sensory epithelium in the inner ear of the silver carp, Hypophthalmichthys molitrix, was studied from an early stage (2 days after fertilization), when the otic vesicle or otocyst first formed, to the post-larval stage (7 days after hatching), when the development of the inner ear approximates that of the juvenile stage. Light microscopy revealed: (1) the otic vesicle, the primordia of the saccular otolith and the stato-acoustic ganglion were observed by 2 days after fertilization; (2) the saccular macula overlain by a small round otolith is established at 3 days after fertilization, while the utricular macula with its otolith is first observed at 4 days after fertilization; (3) the saccular macula began to differentiate at 3 days after hatching and became well differentiated at 7 days after hatching. At 7 days after hatching, transmission electron microscopy (TEM) revealed: (1) the apical surface of each hair cell is covered with a ciliary bundle formed of a long kinocilium and a short bundle of stereocilia. The supporting cells are provided with microridges on their apical surface and seemed to be covered with small and large vesicles, suggesting that they have a secretory function beside the supporting one; (2) secretory materials such as multivesicular bodies, electron dense granules, empty vesicles and cytoplasmic extrusions are observed scattering over the saccular sensory epithelium, which probably contribute to the formation of the otolith and/or otolithic membrane. Using scanning electron microscopy (SEM), two types of hair bundles are distinguished: the first type consisted of numerous short stereocilia and a kinocilium; the second type has a small number of short stereocilia with a very long kinocilium. Most of these results are discussed with special regard to the environmental factors affecting the early development of the inner ear in teleost fishes.
Introduction
The structure of the adult fish labyrinth (inner ear) is well known, and it has been described for many different species of teleosts (for a review see Platt Citation1983). However, there are few studies on the development of the sensory regions in the labyrinth (Becerra & Anadon Citation1993; Salem & Omura Citation1998a).
The inner ear of teleost fishes is composed of the upper labyrinth (pars superior) containing three semicircular canals and one otolithic organ (i.e., the utriculus), being involved in determination of angular acceleration and responses to positional changes (Kawamura Citation1984; Noro et al. Citation2007); and the lower labyrinth (pars inferior) which includes the two otolithic end organs, the sacculus and lagena, which is usually involved in the auditory sense (Coombs & Popper Citation1982; Fay & Edds-Walton Citation2008). It was known that each of the end organs contains a sensory epithelium or macula overlain by a calcareous otolith, which connected via an otolithic membrane to the ciliary bundles of the hair cells in the macula. As reported by Dunkelberger et al. (Citation1980) and Fay and Edds-Walton (Citation2008), the otolithic membrane consists of two layers, a thick gelatinous layer which is adjacent to the otolith and a thin fibrous subcupular meshwork layer which adjacent to the sensory epithelium. It has been considered that the otolithic membrane which lies between the otolith and the sensory macular region is an apparatus for mechanoreception of gravitational forces (Saitoh & Yamada Citation1989).
A number of investigators have demonstrated that there is considerable inter-specific variation in the gross morphology of the vertebrate ear (Fay & Popper Citation1980; Noro et al. Citation2007; Liang & Burgess Citation2009), a fact that is especially evident in the auditory portions of the inner ear in the teleost fishes, the sacculus and lagena (Platt & Popper Citation1984; Campana & Neilson Citation1985; Salem & Omura Citation1998a,b; Song et al. Citation2006). Inter-specific differences in the sacculus and lagena are seen in a number of structural features that include the shape and size of the otoliths and the area of the sensory epithelium that lies directly in contact with the otolith. Ultrastructural variations include the lengths of the cilia extending from the hair cells on different regions of the sensory epithelium and also the orientations of these cilia on the sensory cells at different regions of the epithelium (Sokolowski & Popper Citation1987; Hertwig & Schneider Citation1999).
The saccular macula appears to be the major receptor of sound in most fishes (Lombarte & Fortuño Citation1992; Popper & Fay Citation2011). According to previous studies, most structural variations in the fish inner ear occur in the inferior division, particularly in the saccular macula (Popper et al. Citation1993; Ramcharitar et al. Citation2004). These structural variations are: the structure and position of the saccular macula, the morphology of the saccular otolith and the otolithic membrane (Salem & Omura Citation1998a; Lovell et al. Citation2006; Liang & Burgess Citation2009).
According to their position in the inner ear, three different types of otoliths can be distinguished, namely the utricular otolith (lapillus), saccular otolith (sagitta) and lagenar otolith (asteriscus). Otoliths can cover the entire macula or only a part of it (Buran et al. Citation2005; Schulz-Mirbach et al. Citation2010); in the latter case, the remaining macula area is covered by the otolithic membrane only. Still, the interrelationships between otolith morphology, otolithic membrane structure and structure of the sensory epithelium are not yet fully understood (Popper et al. Citation2003).
It has been agreed that the teleost otolith is composed of calcium carbonate (Morales-Nin Citation1987) and other secretory materials deposited on a small amount of an organic matrix (Degens et al. Citation1969). These secretory materials were believed to be secreted by the macular epithelial cells of the otolithic organs (Dale Citation1976; Dunkelberger et al. Citation1980).
It was reported that the sensory epithelium in each macula consists of sensory hair cells, supporting cells, transitional cells and/or precursor cells (Salem & Omura Citation1998a,b; Hertwig & Schneider Citation1999; Ladich & Popper Citation2001). Each hair cell possesses a hair bundle protruding from the apical surface. The bundle consists of one kinocilium and numerous stereocilia or stereovilli (Neugebauer & Thurm Citation1984; Salem & Omura Citation1998a; Deng et al. Citation2013). It has been reported that detection of sound and head acceleration in vertebrates depends on the transduction of mechanical force into electrical signals in inner ear hair cells (Hudspeth Citation1983). It is a common observation that hair bundles differ in length and size in different regions of the sensory epithelium (Popper Citation1981; Bang et al. Citation2001; Schulz-Mirbach et al. Citation2011). Also, there are considerable variations in the number and lengths of the stereocilia borne by sensory cells in the inner ear, and in the length of the kinocilium (Buran et al. Citation2005). It has been proposed that this might have a functional significance (Platt & Popper Citation1984; Tanimoto et al. Citation2011).
Most previous studies on different species of teleosts were concentrated on the structure of the sacculus in the adult fish (e.g., Platt Citation1983; Ramcharitar et al. Citation2004; Lovell et al. Citation2006; Deng et al. Citation2013). However, there are few studies on the development of the saccular macula in fish inner ear (e.g., Becerra & Anadon Citation1993; Salem & Omura Citation1998a). These studies have demonstrated the addition of new sensory cells over a long period in fish. It is known that the sensory epithelial cells of each otolithic organ are associated with branches of the eighth auditory nerve. The role of innervation in the differentiation of the sensory cells in vertebrate ear is controversial. Some authors claim that the influence of the nervous system is necessary for cellular differentiation (Chandler Citation1984; Fritzsch et al. Citation2002). However, others believe that sensory cells can differentiate without this influence (Raymond Citation1987; Becerra & Anadon Citation1993).
The present study was conducted to characterize the development and structure of one of the most important auditory parts of the inner ear, the saccular macula, in an attempt to understand the cellular contribution of the saccular sensory epithelium to otolith growth in the silver carp, Hypophthalmichthys molitrix, using light and electron microscopy (transmission and scanning). Neither TEM (transmission electron microscopy) nor SEM (scanning electron microscopy) data on the saccular macula are currently available on this species.
Materials and methods
Fertilized eggs of the silver carp, Hypophthalmichthys molitrix, were obtained from Abbasa Fisheries Experimental Station (Sharkia Province, Egypt). Whole bodies of five different ages of the silver carp were selected: 2 and 3 days after fertilization, hatching day (the fourth day after fertilization), and 3 and 7 days after hatching. The specimens were immersed in fixative (2% paraformaldehyde – 1% glutaraldehyde in 0.1 M phosphate buffer, pH 7.3) and left for 2–4 hours. After post-fixation in 1% osmium tetroxide in 0.1 M phosphate buffer at pH 7.3, all specimens were dehydrated in graded series of ethanol and embedded in epoxy resin (Robenson et al. Citation1987). Three individuals from each stage were selected for the study. For light microscopy observations, serial semithin sections (1–3 µm) at the inner ear region in all of the selected ages were cut (on a Porter-Blum MT-1 ultramicrotome) with glass knives and stained with 1% toluidine blue.
For TEM observations, ultra-thin sections from three specimens at 7 days after hatching were cut (at the inner ear region), stained with uranyl acetate and lead citrate, and examined under a JEOL 100 CX TEM at 80 kV.
For SEM, three adult specimens of silver carp were captured and euthanized. The crania were opened dorsally, the brain removed and, through a small opening in the inner ear, a small amount of 2% glutaraldehyde in phosphate buffer was placed for 10 min. The inner ear was dissected, and the otoliths and the otolithic membrane were removed. The sacculus was gently cut, rinsed with 0.1 M phosphate buffer containing a dilute solution of magnesium chloride (MgCl2), and fixed in 2% glutaraldehyde + 2% tannic acid in the same buffer on ice. After 15–30 min on ice the tissues were washed with the buffer, postfixed with 0.5% osmium tetroxide for 10 min, washed and incubated for 20 min in 2% tannic acid. The tissues were then dehydrated through ascending ethanol series, critical point dried using carbon dioxide, mounted on stubs and coated with gold in a JEOL fine coat Ionsputter, and examined with a JEOL JSM-35 scanning electron microscope. The SEM technique proceeded according to the method of Neugebauer and Thurm (Citation1984).
Results
Light microscopy observations
Two days after fertilization
The otic vesicle, which has an oval shape, is present on either side of the posterior portion of the head at the level of the developing hind brain (). Numerous heavily stained spherules with various sizes and shapes, representing the primordia or otoconia of the saccular otolith, are observed scattering in the lumen of the otic vesicle (). The dorso-lateral walls of the otic vesicle are thin, while the ventro-medial wall is thick representing establishment of macular sensory area (). The stato-acoustic ganglion (VIIIth nerve ganglion) which contains the neuronal precursors of the macular epithelium is also clearly seen at this stage. This ganglion appears as a single ventro-medial mass beneath the medial floor of the otic vesicle ().
Three days after fertilization
The otic vesicle is rapidly enlarged by expanding its lumen and forms a pear-shaped structure (). The saccular otolith appears over a thickened region in the ventro-medial wall of the vesicle, representing the establishment of the saccular macula that lies in an oblique position (). The utriculus and the semicircular canals are not observed until this stage.
Figure 2. Hypophthalmichthys molitrix, 3 days after fertilization. Light microscopy micrograph of a transverse section across the developing inner ear, showing first appearance of the saccular otolith (so) on an accumulation of polygonal cells, representing the saccular macula (sm). Note the stato-acoustic ganglion (sg) behind the saccular macula. br, brain. 350×.
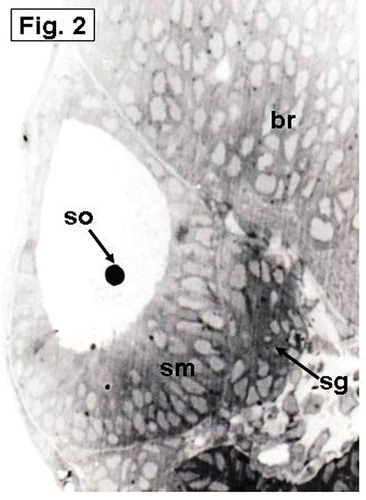
Four days after fertilization (hatching day)
The saccular macula, which is not yet differentiated, is more invaginated medially. It becomes a flattened region of arranged columnar cells and overlain by a small rounded otolith (). At this day, an aggregation of cells taking horizontal position and overlain by a small rounded otolith, representing establishment of the utricular macula, is also seen ().
Three days after hatching
The stage of 3 days after hatching represented an advanced step in the development of the saccular macula and showed major differences compared with the previous stage, manifested mainly in the beginning of differentiation of the macular epithelial cells (). The sensory epithelium of the saccular macula, which is the thickest region in the saccular wall, is vertically oriented and essentially restricted to the medial wall. The hair cells of the sensory epithelium, which gradually started to differentiate, are concentrated in the middle part of the epithelium (). The saccular otolith elongates and covers the middle part of the saccular macula ().
Figure 5. Hypophthalmichthys molitrix, 3 days after hatching. Light microscopy micrograph of a transverse section across the developing inner ear, showing the beginning of differentiation of the saccular macular epithelium (sm) that positioned in a vertical position and restricted to the medial wall. The saccular macula (sm) overlain by an elongated saccular otolith (so) which covers the middle part of the macula. br, brain; asc, anterior semicircular canal; hsc, horizontal semicircular canal. 200×.
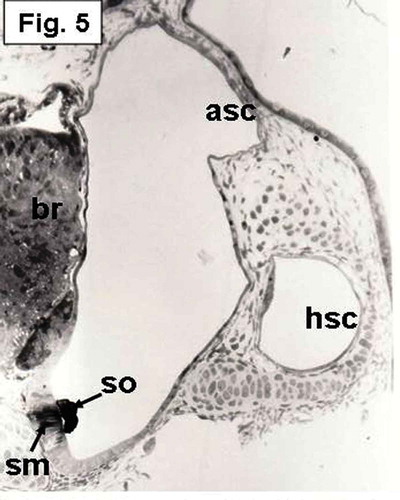
Seven days after hatching
At this time, the saccular sensory epithelium is well developed and differentiated. It invaginates deeply toward the ventro-medial region (). The saccular otolith increases in size and covers most of the saccular macula (). The saccular macula consists of lightly stained hair cells and dark-stained supporting cells (). Each hair cell exhibits a large, oval or elongate nucleus. At the periphery of the macula, the epithelium remains thickened; this epithelium is called transitional epithelium ().
Figure 6. Hypophthalmichthys molitrix, 7 days after hatching. Light microscopy micrograph of a transverse section through the developing inner ear showing the saccular macula (sm) which is more invaginated toward the ventro-medial wall. The saccular otolith (so) increased in size and covered most of the saccular epithelial cells. br, brain. 250×.
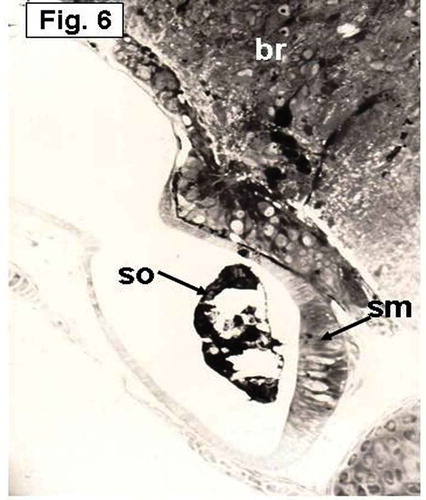
Figure 7. Hypophthalmichthys molitrix, 7 days after hatching. Light microscopy micrograph of a transverse section through the developing inner ear showing differentiation of the saccular macula into transitional epithelium (te) and sensory epithelium that differentiate into hair cells (arrows) and supporting cells (sc). so, saccular otolith. 300×.
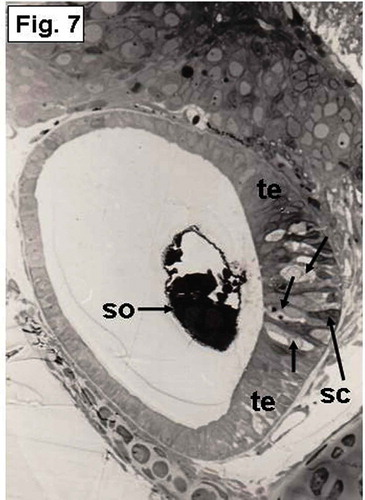
Transmission electron microscopy observations
Because the stage of 7 days after hatching represented an advanced step in the saccular sensory epithelium differentiation, it was important to examine it under TEM. Two types of cells are clearly observed in the saccular sensory epithelium: hair (sensory) cells and supporting cells (–). The hair cells are basically columnar but are slightly narrowed apically, possibly due to the expansion of the adjacent supporting cells at this level. Projecting from the luminal surface of each hair cell is a bundle of sensory hairs, which is planted in a cuticular plate (). The cytoplasm of each hair cell contains an abundance of elongated and oval mitochondria ().
Figure 8. Hypophthalmichthys molitrix, 7 days after hatching. TEM micrograph of the saccular sensory epithelium, showing supporting cells (sc) and a hair cell (hc) which possesses a hair bundle (hb) that planted in a cuticular plate (c). Secretory materials, like cytoplasmic extrusion (ce) and empty vesicles (arrows), seemed to be librated from supporting cells (sc). Microridges (mr) can be seen on the apical surface of a supporting cell. j, junctional complex; st, stereocilia of the hair bundle. 8300×.
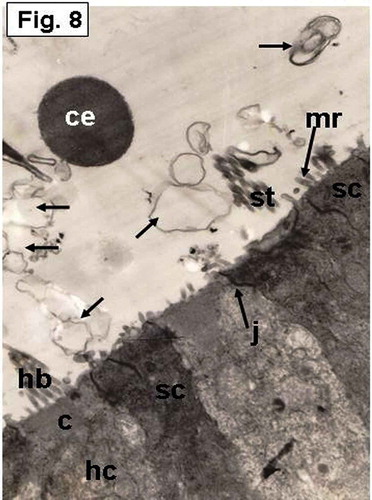
Figure 9. Hypophthalmichthys molitrix, 7 days after hatching. TEM micrograph of the saccular sensory epithelium, showing the otolith (ot) covering hair cells (hc) and supporting cells (sc). Secretory materials like numerous vesicles (arrows), cytoplasmic extrusion (ce) and electron dense granules (eg) can be seen between the otolith and the sensory epithelium. hb, hair bundle. 6300×.
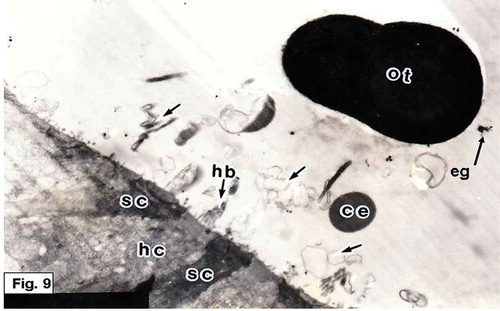
Figure 10. Hypophthalmichthys molitrix, 7 days after hatching. TEM micrograph of the saccular sensory epithelium, showing cytoplasmic extrusion (ce) projecting from supporting cell (sc) and still in contact with its apical surface. Secretory materials, as vesicular bodies (vb) containing small spherules, seemed to be librated from supporting cell. Note the microridges (mr) of the supporting cell. 17,000×.
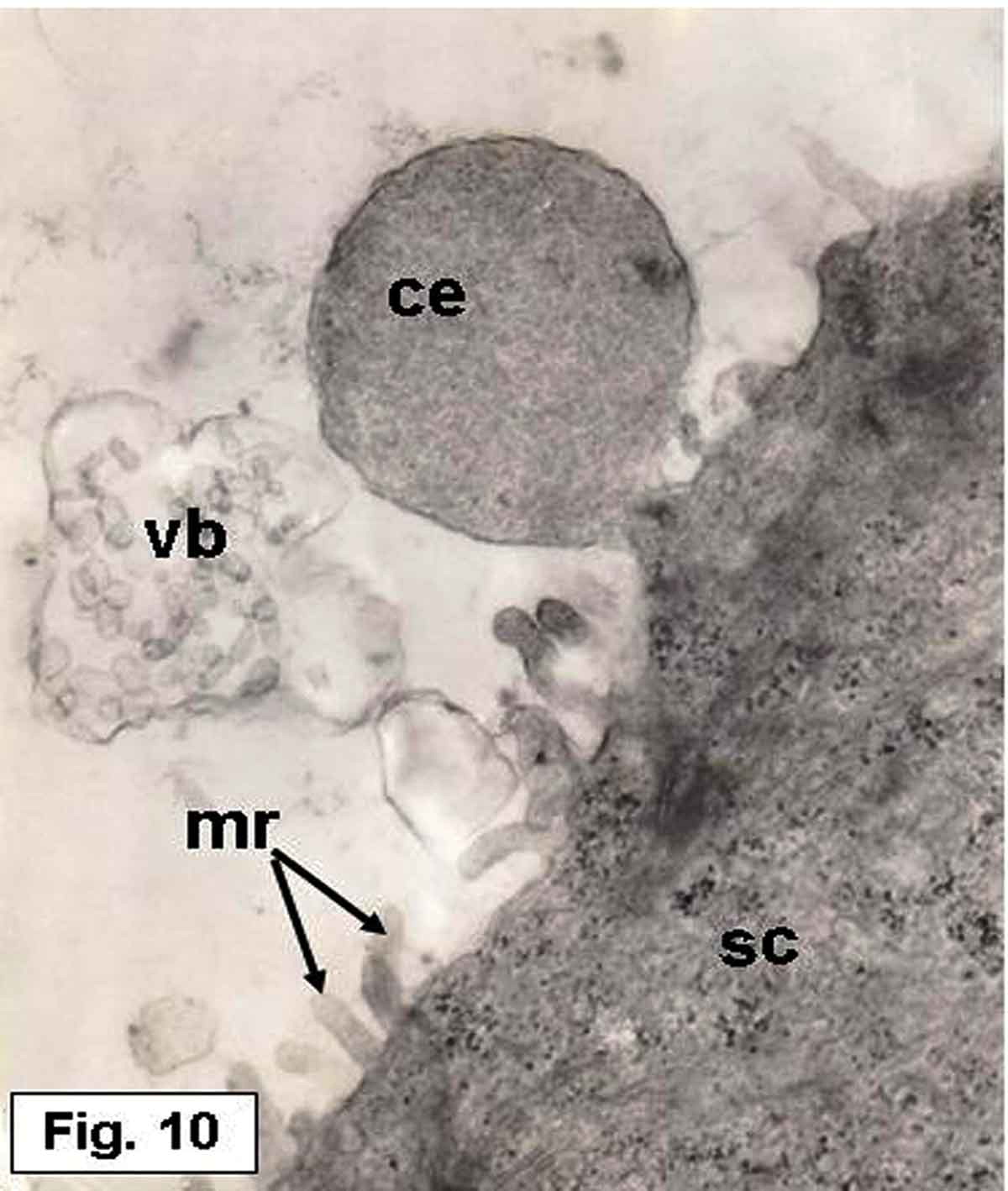
Figure 11. Hypophthalmichthys molitrix, 7 days after hatching. TEM micrograph of the saccular sensory epithelium, showing an accumulation of various kinds of secretory materials over the saccular sensory epithelium; empty vesicles (ev), electron dense granules (arrows). Note the stereocilia (st) of a hair bundle projecting from the cuticular plate (asterisk) of the hair cell (hc). The supporting cells (sc) provided with microridges (mr) on their apical surfaces. 8500×.
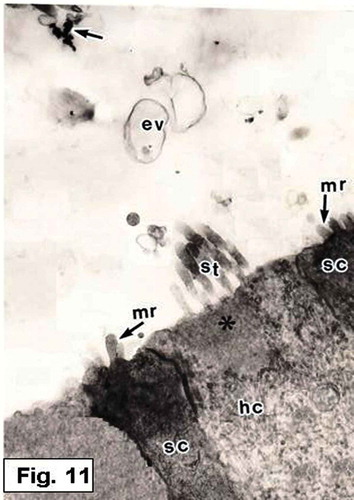
Figure 12. Hypophthalmichthys molitrix, 7 days after hatching. TEM micrograph of a sensory hair cell (hc), showing the stereocilia (st) which is planted in a cuticular plate (arrow). Secretory materials, as vesicular bodies (vb) containing numerous spherules and other vesicles (asteriske), are also seen. 15,000×.
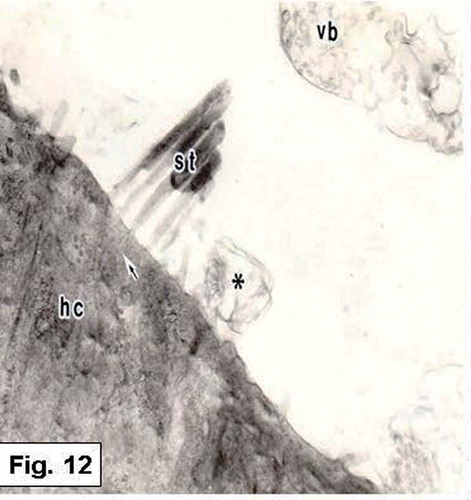
Figure 13. Hypophthalmichthys molitrix, 7 days after hatching. TEM micrograph of a sensory hair cell, showing numerous mitochondria (arrows) in the supranuclear part of the hair cell (hc). c, cuticular plate; hb, hair bundle. 16,000×.
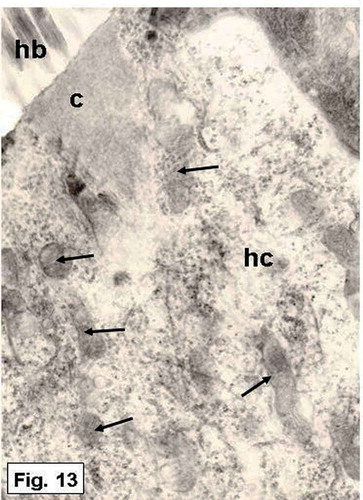
The hair cells are embraced by supporting cells, which expand at their apical parts ( and ). Some supporting cells are provided with microridges on their apical surface (, and ). The supporting cells are joined with each other or with the adjacent hair cells by junctional complexes ( and ). The cytoplasm of the supporting cells is more electron opaque than that of the hair cells. Some secretory materials, such as small and large vesicles, cytoplasmic extrusions and vesicular bodies, appeared to be liberated from the supporting cells (, and ). These secretions are restricted to the sensory region. Electron-dense granules are also observed scattering in the saccular lumen ( and ).
Scanning electron microscopy observations
Two types of hair bundles are distinguished in the saccular sensory epithelium. The first type is mostly observed in the central region and consists of numerous short stereocilia that grade in height, and a kinocilium which is generally twice as long as the longest stereocilium (). The second type of the hair bundles occurrs at the peripheral regions and consists of a small number of short stereocilia and an extremely long kinocilium ().
Discussion
Although the overall pattern of development is similar among the vertebrates, the initial development of the otic vesicle of teleost fishes begins at different times depending on the species and/or environmental parameters such as water temperature (Becerra & Anadon Citation1993). In the present study, the otic vesicle is formed around 2 days after fertilization in embryos kept at 28–30°C, whereas the vesicle was formed at 7 days after fertilization in Opsanus tau embryos (kept at 18–20°C; Tracy Citation1959) and at 15 hours after fertilization in zebrafish embryos (kept at 28°C; Waterman & Bell Citation1984). These results indicate that water temperature seems to influence rate of the development; high temperature accelerates the time of hatching which in turn hastens the differentiation of the sensory regions.
Some studies have been reported that the inner ear in fish begins with the formation of the saccular epithelium followed by the formation of the utricular epithelium and the semicircular canals (Salem & Omura Citation1998a,b; Salem & Zaghloul Citation2001). The development of the inner ear in Hypophthalmichthys molitrix follows this general sequence, with the first formation of the saccular epithelium at 3 days after fertilization and the utricular macula at 4 days after fertilization. A similar result was found in Krypropterus bicirrhis (Jenkins Citation1977). This pattern of development is also the same in frogs (Villy Citation1890) and lizards (Landmann Citation1972). The early appearance of the sacculus before the utriculus and semicircular canals in some fishes and in the present study, suggests that the auditory sense is probably established earlier than the sense of equilibrium. However, in other teleost species, such as Paralichthys olivaceus (Kawamura & Ishida Citation1985) and Epinephelus fuscoguttatus (Salem & Al-Jahdali Citation2006), the equilibrium sense is established earlier than the auditory sense, where the utricular macula appeared prior to the saccular macula. In contrast, other studies have observed that the saccular and utricular maculi appear at the same time (e.g., Sokolowski & Popper Citation1987; Becerra & Anadon Citation1993; Whitfield et al. Citation2002). These developmental differences probably depend on the behavioral and habitual requirements of the fish.
The statoacoustic ganglion, the saccular macula and the saccular otolith were formed in the silver carp before the appearance of the utricular macula and its otolith. The significance of the appearance of these structures at an early stage (before the formation of the utricular macula) may be important for the establishment of the auditory mechanism. Therefore, it can be said that the inner ear of the silver carp probably becomes functional for the auditory or hearing sense at early embryonic stage, before the equilibrium one. Similar cases have been recorded in the ayu Plecoglossus altivelis (Salem & Omura Citation1998b) and in Fundulus heteroclitus (Radtke & Dean Citation1982). In contrast, Kawamura and Ishida (Citation1985) in Paralichthys olivaceus and Becerra and Anadon (Citation1993) in Salmo trutta fario reported that the equilibrium sense was established before the auditory sense. Consequently, it is possible to assume that the auditory sense in some fishes is established earlier than the equilibrium sense and vice versa in other fishes: probably, this phenomenon depends on the fish habitat.
In Hypophthalmichthys molitrix of the present study, numerous heavily stained spherules or particles of various sizes and shapes, representing the primordia or otoconia of the saccular otolith, were observed in the lumen of the otic vesicle at 2 days after fertilization. Similar results were recorded in the embryos of Fundulus heteroclitus (Radtke & Dean Citation1982), Opsanus tau (Sokolowski & Popper Citation1987), Oreochromis niloticus (Zhang & Runham Citation1992) and Plecoglossus altivelis (Salem & Omura Citation1998a). These primordia or otoconia are either separated or fused during the embryonic development of different fish species (Brothers Citation1984). It has been suggested that the types of fusion or separation, and shape and size, in addition to the number of the primordia are taxonomically useful characteristics (Brothers Citation1984).
The saccular otolith develops at different times in the inner ear of different teleost species. The saccular otolith in the silver carp embryos appeared at 3 days after fertilization. In comparison, in killifish (Radtke & Dean Citation1982) and zebrafish (Waterman & Bell Citation1984), the saccular otolith appeared at 48 and 72 hours after fertilization, respectively, while in Oncorhynchus mykiss (Salem & Omura Citation1998a) the saccular otolith appeared at 12 days after fertilization, and it appeared 30 days after fertilization in Epinephelus fuscoguttatus (Salem & Al-Jahdali Citation2006). This disparity in the appearance time of the saccular otolith is presumed to be a species-specific feature.
In the silver carp, the stato-acoustic ganglion (VIIIth nerve ganglion) appeared before the differentiation of the saccular sensory epithelium. This result was similar to that found in the brown trout Salmo trutta fario (Beccera & Anadon 1993) and zebrafish Danio rario (Haddon & Lewis Citation1996). It is possible to suggest that the stato-acoustic ganglion is necessary to start differentiation of the saccular sensory cells. Further experimental applications are necessary to show whether the stato-acoustic ganglion (nerve fibers) is required for sensory cell differentiation in fish inner ear.
It has been shown that the otoliths attach to the sensory epithelium by the otolithic membrane (Dunkelberger et al. Citation1980). Although the latter in the bony fishes has been treated as a relatively familiar structure throughout the literature, data concerning its fine structural features are still limited. This is probably due to the discreteness of its organic phase as well as the technical difficulties involved in preparing sections of the mineralized otolith.
In vertebrates other than fishes, a few studies have suggested that the otolithic membrane may participate in otolith formation. Marco et al. (Citation1971) indicated that in the guinea pig, the central parts of the inner ear otoliths and the two zones of the otolithic membrane were similar in morphology and that otolithic membrane material penetrated through fissures in the otolith. These findings led the authors to believe that otoliths were generated within the otolithic membrane.
The present observations showed various kinds of secretory materials over the saccular sensory epithelium. These secretions, which seemed to be produced by the sensory epithelial cells (hair cells and/or supporting cells) of the saccular macula, include cytoplasmic extrusions, vesicular dilations and multivesicular bodies containing numerous spherules and numerous electron dense granules.
Multivesicular bodies or vesicular dilations containing numerous spherules or small vesicles were observed within the subcupular meshwork zone, suggesting that they have an important role in the formation of the otolithic membrane and/or the otolith matrix. This result agrees with that found in Oreochromis niloticus (Zhang Citation1992). It was reported by Dale (Citation1976) that the subcupular meshwork of the otolithic membrane of Gradus morhua seemed to consist of the same sort of vesicles or spherules. Saitoh and Yamada (Citation1989) also suggested that these vesicles in the sacculus of Oreochromis niloticus could be incorporated into the subcupular meshwork, which is responsible for otolith protein matrix formation (Dunkelberger et al. Citation1980; Liang & Burgess Citation2009). Similar structures were also observed and considered to contribute to the organic matrix of otoconia in frogs (Harada Citation1972) and rats (Salamat et al. Citation1980).
In the silver carp, numerous electron-dense granules are found over the saccular macula and also scattered in the otolithic membrane region. The chemical nature of these granules remains unknown. Dunkelberger et al. (Citation1980) and Saitoh and Yamada (Citation1989) presumed that such granules contain the precursor materials of the organic matrix of the otolith, and also suggested that these materials are incorporated into the subcupular meshwork, which later becomes an organic constituent of the otolith matrix. An abundance of mitochondria, particularly at the apical regions of the hair cells, is observed in the saccular sensory epithelial cells of the silver carp. Such an abundance of mitochondria was believed to be responsible for supplying calcium for otolith growth (Mugiya Citation1974).
In the saccular sensory epithelium of the silver carp, it was found that the peripheral hair bundles have very long kinocilia with short stereocilia, while the central ones possessed moderately long kinocilia and short stereocilia. The variations between the peripheral and central regions suggest that their hair bundles respond to different types of stimuli (Platt & Popper Citation1984). Dale (Citation1976), in a comprehensive study of the cod inner ear, similarly suggested that long kinocilia and short stereocilia are sensitive primarily to large and low-frequency displacements. Short kinocilia and stereocilia, in contrast, were presumed to be pressure sensitive, responding to the high frequencies of auditory stimuli (Lu & Popper Citation2001). From another point of view, Saunders and Dear (Citation1983) mentioned that the bundles with long stereocilia may be tuned to low frequencies, while bundles with shorter stereocilia may be tuned to higher frequencies. However, Platt and Popper (Citation1984) and Ramcharitar et al. (Citation2004) proposed that the differences in the length of the ciliary bundles may be related to the nature of the stimulus applied to the ear fluid and tissues.
Acknowledgements
The author is very grateful to Prof Dr Al-Ahmady S Al-Zahaby and Prof Dr Momtaz H Ismail, Department of Zoology, Faculty of Science, Zagazig University, for reading the manuscript and also for their valuable suggestions.
References
- Bang PI, Sewell WF, Malicki JJ. 2001. Morphology and cell type heterogeneities of the inner ear epithelia in adult and juvenile zebrafish (Danio rerio). The Journal of Comparative Neurology 438:173–190. doi:10.1002/(ISSN)1096-9861.
- Becerra M, Anadón R. 1993. Development of the inner ear of the brown trout (Salmo trutta fario): II. Cytodifferentiation and innervation of sensory cells. Journal of Morphology 216:241–257. doi:10.1002/jmor.v216:3.
- Brothers EB. 1984. Otolith studies on early life history of fishes. In: La Jolla CA, editor. Ontogeny and systematic of fishes. Special publication No. 1. American Society of Ichthyologists and Herpetologists. pp. 50–57.
- Buran BN, Deng X, Popper AN. 2005. Structural variation in the inner ears of four deep-sea elopomorph fishes. Journal of Morphology 265:215–225. doi:10.1002/(ISSN)1097-4687.
- Campana SE, Neilson JD. 1985. Microstructure of fish otoliths. Canadian Journal of Fisheries and Aquatic Sciences 42:1014–1032. doi:10.1139/f85-127.
- Chandler JP. 1984. Light and electron microscopic studies of the basilar papilla in the duck, Anas platyrhynchos. II. Embryonic development. The Journal of Comparative Neurology 222:523–542. doi:10.1002/(ISSN)1096-9861.
- Coombs S, Popper AN. 1982. Structure and function of the auditory system in the clown knife fish, Notopterus chitala. Journal of Experimental Biology 97:225–239.
- Dale T. 1976. The labyrinthine mechanoreceptor organs of the cod Gadus morhua (Teleostei: Gadidae). Norwegian Journal of Zoology 24:85–128.
- Degens ET, Deuser WC, Haedrich RL. 1969. Molecular structure and composition of fish otoliths. Marine Biology 2:105–113. doi:10.1007/BF00347005.
- Deng X, Wagner HJ, Popper AN. 2013. Interspecific variations of inner ear structure in the deep-sea fish family melamphaidae. The Anatomical Record 296:1064–1082. doi:10.1002/ar.v296.7.
- Dunkelberger DG, Dean JM, Watabe N. 1980. The ultrastructure of the otolithic membrane and otolith in the juvenile mummichog, Fundulus heteroclitus. Journal of Morphology 163:367–377. doi:10.1002/(ISSN)1097-4687.
- Fay RR, Edds-Walton PL. 2008. Structures and functions of the auditory nervous system of fishes. In: Webb JF, Fay RR, Popper AN, editors. Fish bioacoustic. New York: Springer. pp. 49–97.
- Fay RR, Popper AN. 1980. Structure and function in teleost auditory system. In: Popper AN, Fay RR, editors. Comparative studies of hearing in vertebrates. New York: Springer-Verlag. pp. 1–42.
- Fritzsch B, Beisel KW, Jones K, Fariñas I, Maklad A, Lee J, Reichardt LF. 2002. Development and evolution of inner ear sensory epithelia and their innervation. Journal of Neurobiology 53:143–156. doi:10.1002/neu.v53:2.
- Haddon CM, Lewis JM. 1996. Early ear development in the embryo of the Zebrafish, Danio rerio. The Journal of Comparative Neurology 365:113–128. doi:10.1002/(ISSN)1096-9861.
- Harada Y. 1972. Surface view of the frog vestibular organ with the scanning electron microscope. Acta Oto-Laryngologica (Stokh.) 73:316–322. doi:10.3109/00016487209138947.
- Hertwig I, Schneider H. 1999. Comparative light and electron microscopic study of the auditory organs of two species of fishes (Pisces): Hyphessobrycon simulans (Ostariophysi) and Poecilia reticulata (Acanthopterygii). European Journal of Morphology 37:17–28. doi:10.1076/ejom.37.1.0017.
- Hudspeth AJ. 1983. Mechano-electrical transduction by hair cells in the acousticolateralis sensory system. Annual Review of Neuroscience 6:187–215. doi:10.1146/annurev.ne.06.030183.001155.
- Jenkins DB. 1977. A light microscopic study of the saccule and lagena in certain catfishes. American Journal of Anatomy 150:605–629. doi:10.1002/(ISSN)1553-0795.
- Kawamura G. 1984. The sense organs and behavior of milkfish fry in relation to collection techniques. In: Juario JV, Ferraris RP, Beitez LV, editors. Advance in milkfish biology and culture. Manilla: Island Publication House. pp. 69–85.
- Kawamura G, Ishida K. 1985. Changes in sense organ morphology and behaviour with growth in the flounder Paralichthys olivaceus. Bulletin of the Japanese Society of Scientific Fisheries 51:155–165. doi:10.2331/suisan.51.155.
- Ladich F, Popper AN. 2001. Comparison of the inner ear ultrastructure between teleost fishes using different channels for communication. Hearing Research 154:62–72. doi:10.1016/S0378-5955(01)00217-9.
- Landmann L. 1972. Bau und ontogenese des vestibularen system bei Lacerta sicula. Bericht Über Die Verhandlungen Der Naturforschenden Gesellschaft in Basel 82:1–53.
- Liang J, Burgess SM. 2009. Gross and fine dissection of the inner ear sensory epithelia in adult zebrafish (Danio rerio). Journal of Visualized Experiments 27(pii):1211.
- Lombarte A, Fortuño JM. 1992. Differences in morphological features of the sacculus of the inner ear of two hakes (Merluccius capensis and M. paradoxus, gadiformes) inhabits from different depth of sea. Journal of Morphology 214:97–107. doi:10.1002/jmor.v214:1.
- Lovell JM, Findlay MM, Harper GM, Moate RM. 2006. The inner ear ultrastructure from the paddlefish (Polyodon spathula) using transmission electron microscopy. Journal of Microscopy 222:36–41. doi:10.1111/jmi.2006.222.issue-1.
- Lu Z, Popper AN. 2001. Neural response directionality correlates of hair cell orientation in a teleost fish. Journal of Comparative Physiology A: Sensory, Neural, and Behavioral Physiology 187:453–465. doi:10.1007/s003590100218.
- Marco J, Sánchez-Fernández JM, Rivera-Pomar JM. 1971. Ultrastructure of the otoliths and otolithic membrane of the macula utriculi in the guinea pig. Acta Oto-Laryngologica (Stokh.) 71:1–8. doi:10.3109/00016487109125326.
- Morales-Nin B. 1987. Ultrastructure of the organic and inorganic constituents of the otoliths of the sea bass. In: Summerfelt RC, Hall GE, editors. The age and growth of fish. Ames, IA: Iowa State University Press. pp. 331–343.
- Mugiya Y. 1974. Calcium-45 behavior at the level of the otolithic organs of rainbow trout. Bulletin of The Japanese Society of Scientific Fisheries 40:457–463. doi:10.2331/suisan.40.457.
- Neugebauer D-C, Thurm U. 1984. Intra-and extracellular membrane connections in stereovilli from fish inner ear. An electron microscopic study. Verhandlungen der Deutschen Zoologischen Gesellschaft 77:300–313.
- Noro S, Yamamoto N, Ishikawa Y, Ito H, Ijiri K. 2007. Studies on the morphology of the inner ear and semicircular canal end organ projections of ha. Fish Biology Journal Medaka 11:31–41.
- Platt C. 1983. The peripheral vestibular system of fishes. In: Northcutt RG, Davis RE, editors. Fish neurobiology. Ann Arbor: University of Michigan Press. pp. 89–123.
- Platt CT, Popper AN. 1984. Variations in lengths of ciliary bundles on hair cells along the macula of the sacculus in two species of teleost fishes. Scanning Electron Microscopy IV:1915–1924.
- Popper AN. 1981. Comparative scanning electron microscopic investigations of the sensory epithelia in the teleost sacculus and lagena. The Journal of Comparative Neurology 200:357–374. doi:10.1002/(ISSN)1096-9861.
- Popper AN, Fay RR. 2011. Rethinking sound detection by fishes. Hearing Research 273:25–36. doi:10.1016/j.heares.2009.12.023.
- Popper AN, Fay RR, Platt C, Sand O. 2003. Sound detection mechanisms and capabilities of teleost fishes. In: Collin SP, Marshall NJ, editors. Sensory processing in aquatic environments. New York: Springer. pp. 3–38.
- Popper AN, Saidel WM, Chang JS. 1993. Two types of sensory hair cell in the saccule of a teleost fish. Hearing Research 64:211–216. doi:10.1016/0378-5955(93)90008-O.
- Radtke RL, Dean JM. 1982. Increment formation in the otoliths of embryos, larvae, and juveniles of the mummichog, Fundulus heteroclitus. Fisheries Bulletin 80:201–215.
- Ramcharitar JU, Deng X, Ketten D, Popper AN. 2004. Form and function in the unique inner ear of a teleost: The silver perch (Bairdiella chrysoura). The Journal of Comparative Neurology 475:531–539. doi:10.1002/(ISSN)1096-9861.
- Raymond J. 1987. In vitro differentiation of mouse embryo statoacoustic ganglion and sensory epithelium. Hearing Research 28:45–56. doi:10.1016/0378-5955(87)90152-3.
- Robenson DG, Ehlers U, Kerkin R, Hermenn B, Meyer F, Schurman FW. 1987. Methods of preparation for electron microscopy. Germany: Springer.
- Saitoh S, Yamada J. 1989. Ultrastructure of the saccular epithelium and the otolithic membrane in relation to otolith growth in tilapia, Oreochromis niloticus (Teleostei: Cichlidae). Transactions of the American Microscopical Society 108:223–238. doi:10.2307/3226341.
- Salamat MS, Ross MD, Peacor DR. 1980. Otoconial formation in the fetal rat. Annals of Otology, Rhinology & Laryngology 89:229–238. doi:10.1177/000348948008900308.
- Salem MA, Al-Jahdali MO. 2006. Development, structure and function of the sense organs in the brownmarbled grouper Epinephelus fuscoguttatus (Teleostei: Serranidae). Journal of the Egyptian Society of Biotechnoloy and Environmental Sciences 8:95–138.
- Salem MA, Omura Y. 1998a. Embryonic development of the inner ear and otolith of the rainbow trout Oncorhynchus mykiss. Archives of Histology and Cytology 61:179–187. doi:10.1679/aohc.61.179.
- Salem MA, Omura Y. 1998b. Light and electron microscopic studies on the development of the inner ear and otolith of the ayu Plecoglossus altivelis. Fisheries Science 64:259–264.
- Salem MA, Zaghloul MS. 2001. Structure and development of the saccular sensory epithelium in relation to otolith growth in the perch Perca fluviatilis (Teleostei). Egyptian Journal of Biology 3:1–12.
- Saunders JC, Dear SP. 1983. Comparative morphology of stereocilia. In: Fay RR, Gourevitch G, editors. Hearing and other senses. Groton: Amphora Press, Conn. pp. 175–198.
- Schulz-Mirbach T, Ladich F, Riesch R, Plath M. 2010. Otolith morphology and hearing abilities in cave and surface-dwelling ecotypes of the Atlantic molly, Poecilia mexicana (Teleostei: Poeciliidae). Hearing Research 267:137–148. doi:10.1016/j.heares.2010.04.001.
- Schulz-Mirbach T, Martin H, Plath M. 2011. Inner ear morphology in the Atlantic molly, Poecilia mexicana- first detailed microanatomical study of the inner ear of a cyprinodontiform species. PLoS One 6:102–115. doi:10.1371/journal.pone.0027734.
- Sokolowski BHA, Popper AN. 1987. Gross and ultrastructural development of the saccule of the toadfish, Opsanus tau. Journal of Morphology 194:323–348. doi:10.1002/jmor.1051940311.
- Song J, Mathieu A, Soper RF, Popper AN. 2006. Structure of the inner ear of bluefin tuna Thunnus thynnus. Journal of Fish Biology 68:1767–1781. doi:10.1111/jfb.2006.68.issue-6.
- Tanimoto M, Ota Y, Inoue M, Oda Y. 2011. Origin of inner ear hair cells: Morphological and functional differentiation from ciliary cells into hair cells in zebrafish inner ear. Journal of Neuroscience 31:3784–3794. doi:10.1523/JNEUROSCI.5554-10.2011.
- Tracy HC. 1959. Stages in the development of the anatomy of motility of the toadfish (Opsanus tau). The Journal of Comparative Neurology 111:27–81. doi:10.1002/(ISSN)1096-9861.
- Villy F. 1890. The development of the ear and accessory organs in the common frog. Quarterly Journal of Microscopical Science 30:523–550.
- Waterman RE, Bell DH. 1984. Epithelial fusion during early semicircular canal formation in the embryonic zebrafish, Brachydanio rerio. The Anatomical Record 210:101–114. doi:10.1002/(ISSN)1097-0185.
- Whitfield TT, Riley BB, Chiang MY, Phillips B. 2002. Development of the zebrafish inner ear. Developmental Dynamics 223:427–458. doi:10.1002/dvdy.10073.
- Zhang Z. 1992. Relationship of saccular ultrastructure to otolith growth in the teleost Oreochromis niloticus. Journal of Morphology 212:99–107. doi:10.1002/(ISSN)1097-4687.
- Zhang Z, Runham NW. 1992. Initial development of Oreochromis niloticus (Teleostei: Cichlidae) otolith. Journal of Zoology 227:465–478. doi:10.1111/jzo.1992.227.issue-3.