Abstract
Two shallow hydrothermal vents were investigated by SCUBA diving to evaluate their influence on the structure and diversity of a sponge community living close to the vent outflow, in the equatorial Pacific Ocean just off the coast of North Sulawesi, Indonesia (1°40.361ʹN, 125°8.112ʹE). No sponges identified were vent-obligate species, since they are found in the surrounding coral reefs too. The sponges were strongly attracted by the vent, concentrating in an area of a few meters around it, where they reached covering values up to 70% in the deeper vent and up to 42% in the shallower one. The high silica concentration, 8.5 mg L−1Si (deep vent) and 5 mg L−1Si (shallow vent), in hot spring water (90°C) was the putative environmental factor driving the sponge settlement and growth. These organisms take advantage of the increased silica availability that, facilitating skeleton formation, probably promotes sponge growth. This hypothesis is in agreement with the evidence that the spicules of the sponge specimens living around the hot springs have a thickness about double that of conspecific specimens present on the coral reefs at least 300 m away.
Introduction
Hydrothermal vents are peculiar habitats that offer a unique opportunity to investigate the effects of extreme environmental conditions on biodiversity (Amils et al. Citation2007). Despite the convenience of studying shallow-water structures, due to their easier accessibility, deep hydrothermal vents have always received more interest from the scientific community because they host peculiar, sometimes endemic faunas characterized by unusual physiological adaptations (Tarasov et al. Citation2005).
Several studies have shown that shallow vents are, in general, colonized by communities that are subsets of the surrounding biota, where no vent-obligate species are recognized (Fricke et al. Citation1989; Pansini et al. Citation2000; Cardigos et al. Citation2005). However, there is evidence that biodiversity is proportionally higher at the sites closest to the hydrothermal vents and that vent proximity is more important than depth and distance from the shore (Morri et al. Citation1999; Pansini et al. Citation2000; Bianchi et al. Citation2011). These evidences are in contrast with the opinion that the total number of macrobenthic species reduces close to the vents (Fricke et al. Citation1989; Tarasov & Zhirmunsky Citation1989; Kamenev et al. Citation1993; Thiermann et al. Citation1997).
Studies on communities associated with shallow-water hydrothermal vents date back to the beginning of the 20th century (Hinze Citation1903), but an exhaustive global inventory of these outflows is still lacking and information on these structures is fragmentary when compared with that on deep-sea hydrothermal springs (Tarasov et al. Citation2005). Shallow-water hydrothermal vents are recurrent in tectonically active areas of the West Pacific (Ferguson & Lambert Citation1972; Tarasov et al. Citation1986, Citation1988, Citation1990, Citation1991; Sarano et al. Citation1989; Sorokin Citation1991; Tarasov Citation1991; Hashimoto et al. Citation1993; Kamenev et al. Citation1993; Pichler & Dix Citation1996), the coasts of California (Vidal et al. Citation1978; Stein Citation1984), Iceland (Fricke et al. Citation1989; Olafsson et al. Citation1991) and the Azores (Cardigos et al. Citation2005). Even in the Mediterranean Sea, these habitats have been extensively studied (Bavestrello et al. Citation1995; Dando et al. Citation1995; Morri et al. Citation1999; Pansini et al. Citation2000; Bianchi et al. Citation2011).
The influence on biodiversity of shallow-water hydrothermalism is variable according to the different examined groups (Bianchi et al. Citation2011). Sponges are among the most important components of these sessile communities (Fricke et al. Citation1989; Benedetti Cecchi et al. Citation1998; Tarasov et al. Citation1999), and a possible attractive effect due to the spring activity is still debated. Some authors have stated that in both the western and eastern Mediterranean, the proximity of hydrothermal vents had no influence on sponge distribution (Laborel Citation1960; Bavestrello et al. Citation1995). More detailed studies (Pansini et al. Citation2000) suggest a warm-water affinity of the sponge fauna associated with the vent sites. Moreover, Geodia cydonium in the vent community of Milos Island (Aegean Sea) might take advantage of increased silica availability around the outflows. In the same area closeness to vents seemed to have negligible effects on sponge cover, despite a primary effect on species diversity (Pansini et al. Citation2000).
The abundant development of demosponges all over Matupi Harbor (Papua New Guinea), an area characterized by diffuse hydrothermalism, results in the accumulation of large amounts of sponge spicules in the sediment (Tarasov et al. Citation1999). Also in this area, it was suggested that sponge growth might have been stimulated by volcanic emissions enriched in silicon (Tarasov et al. Citation1999).
In the Grotta Azzurra at Capo Palinuro (South Tyrrhenian Sea), the unusual large size of the specimens of G. cydonium living in the cave was related to the occurrence of warm sulfur-rich water springs (Morri et al. Citation1994) and to the abundant presence of sulfur-oxidizing bacteria associated with these emissions (Southward & Southward Citation1993; Morri et al. Citation1994).
In this study, we investigate two shallow hydrothermal springs, located on a sandy bottom at 20 and 25 m depth, in the equatorial Pacific Ocean, just off the coast of the extreme tip of North Sulawesi (Indonesia). The aim of this work is to evaluate the influences of vent outflow on the structure and diversity of the sponge-dominated benthic community that thrives in this area.
Materials and methods
The studied hydrothermal vents are located in a popular dive site known as “Yellow coco” (1°40.361ʹN, 125°8.112ʹE), along the coast of North Sulawesi facing Bangka Island (). The North Sulawesi Peninsula is characterized by the presence of 129 volcanoes, responsible for gas emissions from the sea bottom in several coastal zones (Manini et al. Citation2008; Zeppilli & Danovaro Citation2009).
In the studied site, the underwater seascape consists in a coral reef ending on a sandy ground at about 18 m depth. The two outflows arise from the sandy bottom at 20 (HV20) and 25 m (HV25) depth, 150 and 200 m off the coast, respectively.
Sampling was carried out in October 2014 and all field activities were performed by SCUBA diving.
Sampling was carried out along four orthogonal line transects 10 m long, starting from the hot springs. In each transect photographic sampling, water sampling and temperature measurements were conducted at increasing distances (0, 1, 3, 5 and 10 m) from the vent.
Photographic sampling involved the use of square frames with 50-cm sides (Bianchi et al. Citation2004). A total of 20 images for each vent were collected and analyzed to evaluate the sponge covering.
Water sampling was performed always close to the bottom in order to measure the concentration of dissolved silica. The collected water was filtered using 0.45 μm pore size cellulose acetate filters (ø 47 mm) in order to eliminate any particulate matter, and frozen to await further laboratory analysis. The silicate concentrations were determined in 40 water samples (20 for each vent) following Hansen and Grasshoff (Citation1983), using a Jasco V530 spectrophotometer.
In both of the studied areas, temperatures inside the sediment were measured by SCUBA divers by means of a glass thermometer.
In addition, sub-samples of the sponges found in the square frames were collected to allow their identification. Sponge specimens were fixed in alcohol 70%. The spicule complement of each sponge specimen was analyzed according to Rützler (Citation1978). From 30 measurements for each spicule type, size range and mean were calculated. Whenever possible, skeletal architecture was examined under light microscopy. We followed the classification given by Hooper and van Soest (Citation2002), and the updated nomenclature reported in the World Porifera Database (Van Soest et al. Citation2016).
In Tedania (Tedania) dirhaphis Hentschel, 1912 and Xestospongia muta (Schmidt, 1870) (two of the most common species living close to the vents), the width of 500 spicules per type (100 spicules per five specimens) was measured and compared with that of five specimens living on the coral reef at least 300 m far away. Differences in the calculated averages (± standard error, SE) were tested by the Student’s t-test.
Results
Both of the studied vents were characterized by a continuous outflow of hot water springing from the sandy bottom, easily detectable thanks to the opaque cloud caused by fresh water mixing with salt water (). Visual observations indicated that HV25 had a flow rate approximately double that of HV20.
Figure 2. Main components of the benthic communities around the vents: (A) sponge specimens visible through the cloud caused by warm fresh water mixing with the surrounding marine water; (B) a large horny sponge surrounded by small-branched sponges and crinoids; (C) general view of the community characterized by large sponges and colonial ascidians. Vagile fauna is represented by crinoids; (D) Cinachyrella nuda; (E) a large colonial ascidian close to the water spring; (F) a sponge partially overgrowing a colonial hydroid; (G) dense coverage of branched sponges and hydrozoans.
te: Tedania (Tedania) dirhaphis; mg: Mycale (Mycale) grandis; ir: Ircinia sp.; ha: Haliclona (Reniera) sp.; cl: Cladocroce cf. burapha; dy: Dysidea sp.; ox: Oxycorynia sp.; xm: Xestospongia muta; di: Didemnum sp.; ph: plumularid hydrozoans.
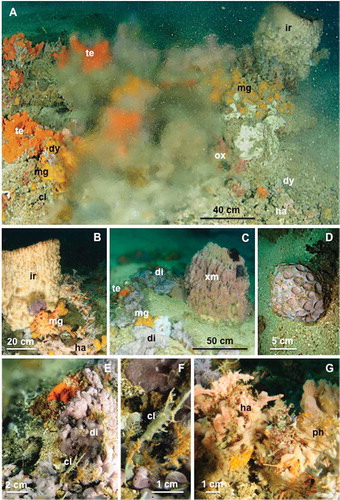
At both vents, the temperature of the water flowing through the sediments is about 85–90°C. At HV20 the average temperature, 1 m from the center of the outflow, is around 82°C, while at HV25 it decreases, at the same distance, to 47°C. At a distance of 3 m from the source, the average temperature in both vents decreased to 32–34°C and remained quite constant for the entire length of the transects (). One meter above both vents, the water temperature was about 40°C.
Figure 3. Trends of the average (± standard error, SE) water temperature, silica concentration in the water and percent sponge covering obtained along four 10-m-long transects starting from both vents (HV20 and HV25).
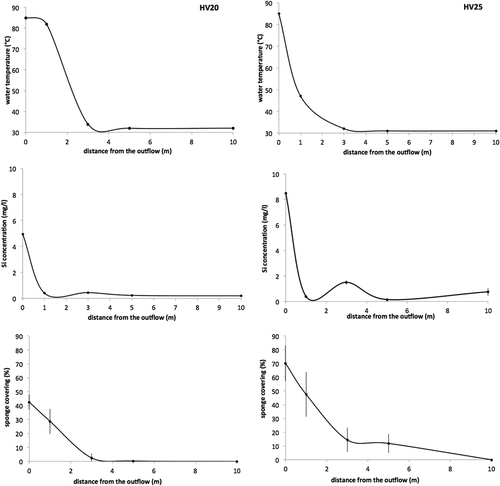
The silicate concentration evaluated at the vent outflow, immediately over the sandy bottom, reached high values ranging from about 5 mg L−1Si at HV20 to 8.5 mg L−1Si at HV25. In both sites at 1 m from the vents, silicate concentrations dropped to 1–0.08 mg L−1Si. At the HV25 site the silicate concentration increases again (2 mg L−1Si) along the transect at a distance of 3 m from the vent, thus suggesting the presence of a secondary, smaller outflow ().
A visual survey of the bottom communities clearly showed a concentration of benthic macrofauna in proximity to the outflows (). The vent community was mainly composed of sponges, with several large specimens belonging to Haliclona (Reniera) sp., Cladocroce cf. burapha Putchakam, de Weerdt, Sonchaeng & van Soest, 2004, Xestospongia muta (Schmidt, 1870), Mycale (Mycale) grandis (Gray, 1867), Tedania (Tedania) dirhaphis Hentschel, 1912, Cinachyrella nuda (Hentschel, 1912), Dysidea sp. and Ircinia sp. Cladocroce cf. burapha grew also as an epibiont on colonial hydrozoans (). The second most representative taxa of benthic organisms in terms of abundance were solitary and colonial ascidians belonging to the genera Didemnum, Rhopalaea, Oxycorynia and Clavelina.Sertularellid and plumularid hydrozoans were widespread as epibionts of sponges and ascidians. At HV25, whip gorgonians of the genus Juncella were detected along the transect. Comatulid crinoids composed the vagile fauna.
The quantitative study of the sponge covering showed in both sites the highest values in an area within 3–5 m of the vents. Around the outflow of HV20 the maximal covering reached 42%, while at HV25 the maximal covering reached values of 70% ().
In order to assess the influence of the water flow on the silica deposition, the average spicule width was measured in two specimens of Xestospongia muta (oxeas) and Tedania (Tedania) dirhaphis (styles and tylotes), respectively, which lived both close to the vents and at a distance of about 300 m from the outflows. In all cases, the thickness of the spicules coming from specimens living close to the vents was about double when compared with that of specimens living far from the water sources (p < 0.01; ).
Discussion
The studied community and particularly the sponge assemblage were formed by species commonly living on the neighboring coral reef, without any obligate species. This situation confirms the data already recorded around other shallow-water vents in different locations: Kolbeinsey, North Iceland (Fricke et al. Citation1989), Aegean Sea (Pansini et al. Citation2000) and the Azores (Cardigos et al. Citation2005).
Nevertheless, our data suggest a strong attractiveness of hydrothermal vents for sponges, allowing them to bypass their usual requirement of a hard substratum for settling. In fact, the species recorded during this study generally settle on coral rocks and not on sand. The vents are indeed “islands” of hard-bottom communities scattered on a wide extension of sandy bottoms. The role played by the hot springs in the sponge concentration is clarified by the quantitative transects showing how the maximal sponge abundance is centered close to the vent, with a rapid and steady reduction as the distance from it increases. This evidence confirms previous observations coming from Indonesian reefs (Tarasov et al. Citation1999), and the assumption of Grassle (Citation1986) that members of phyla lacking a blood vascular system are able to live close to the hot springs.
The ecological factors inducing sponges to settle close to the vents can be of different nature. In some situations, it was hypothesized that sponges are able to feed on the abundant sulfur-oxidizing bacteria recorded as associated with warm sulfur-rich water springs (Southward & Southward Citation1993; Morri et al. Citation1994). Almost all of the studies in the literature on shallow-water hydrothermal vents reveal high chemoautotrophic production (Tarasov Citation2006), which locally enhances trophic conditions (Southward Citation1989). However, it is not yet known to what extent the spring-associated sponges are able to use the high chemoautotrophic production of the vent bacteria (Fricke et al. Citation1989), although it is highly probable that they benefit from this enhanced food supply. Unfortunately, we have no data about the chemosynthetic production of the Yellow coco vents, notwithstanding the organoleptic characteristic of the vent outflows and the lack of colored bacterial mats, which leads us to consider the sulfur emission negligible.
Given the awesome concentrations of silicates in the outflows, up to 10 times higher than in the surrounding water, we can assume that silica is one the main attractive factors promoting the settlement and growth of sponges in an area of a few meters around the vent. Past evidence emphasized the existing link between the high concentrations of silica in the vent fluids and the increased species richness of sessile epibenthic assemblages (Bianchi et al. Citation2011). Here we demonstrate that the sponge abundance also is remarkably enhanced by silicon-enriched emissions. The effect of high silica concentration on the vent sponge community is in agreement with the record of thicker spicules. It has been already stated that silica concentration drives spicule width, as demonstrated by both laboratory (Elvin Citation1971; Pè Citation1973) and field (Stone Citation1970; Bavestrello et al. Citation1993) studies. Moreover, other studies (Maldonado et al. Citation1999; Cárdenas & Rapp Citation2013) have demonstrated that variations in silica concentration are able to modulate the production of the different spicule kinds.
Shallow hydrothermal vents were considered unstable in time and space (Desbruyeres et al. Citation2000), due to the presence of strong currents and rapid fluid dilution at the vent site (Cardigos et al. Citation2005). This is particularly true for a benthic community that draws nourishment from the outflow. In the Yellow coco vents, it is likely that is not the food supply but the silicon enrichment that mainly drives the community structure. This is highlighted by the presence of large specimens of Xestospongia muta, a sponge with a very slow growth rate, presumably with an age of tens of years (McMurray et al. Citation2008).
The restricted amount of data on the presence of sponges in the biotic communities of hydrothermal vents (Fricke et al. Citation1989; Tarasov et al. Citation1999; Pansini et al. Citation2000) does not help in understanding how sponges can withstand the high temperature of sediment where they settle. However, damage resistance and desiccation reduction, both improved by a toughened outer layer, have been observed in the sponge Cinachyrella voeltzkowii (Barnes Citation1999). Even though, at present, we have no evidence of the structural organization allowing sponges to withstand the excessive heat, it seems acceptable to hypothesize that, as occurs in C. voeltzkowii, the strengthening of the peripheral coating, at the cell/substratum interface, can act as a successful barrier.
Acknowledgements
We are grateful for the support of the “Coral eye” research outpost in Bangka Island. Thanks to Marco Segre Reinach and Clara Serra for the platform opportunity and to all the Coral eye volunteers and Indonesian dive guides who participated in the fieldwork. The spicule dissolution study was carried out under the research license of the SIR, Italian Ministry of Education, University and Research.
Additional information
Funding
References
- Amils RA, Blix M, Danson C, Ebel C, Ellis-Evans F, Gaill H, Hinghofer-Szalkay KU, Hinrichs F, Loreto D, Prieur F, Ranjbaran K, Valentine E, Vestergaard, Walter N. 2007. Investigating life in extreme environments - A European perspective. In: Walter N, editor. Strasbourg: European Science Foundation Position Paper. pp. 13–22.
- Barnes DKA. 1999. High diversity of tropical intertidal zone sponges in temperature salinity and current extremes. African Journal of Ecology 37:424–434. DOI:10.1046/j.1365-2028.1999.00197.x.
- Bavestrello G, Bonito M, Sarà M. 1993. Influence of depth on the size of sponge spicules. Scientia Marina 57:415–420.
- Bavestrello G, Cattaneo-Vietti R, Cerrano C, Pansini M. 1995. Considerazioni sull’ecologia e distribuzione dei Poriferi alle Isole Eolie. In: Faranda FM, editor. Caratterizzazione Ambientale Marina del Sistema Eolie e dei Bacini Limitrofi di Cefalù e Gioia (EOCUMM 94). Genova: Data Report CoNISMa. pp. 245–250.
- Benedetti Cecchi L, Airoldi L, Abbiati M, Cinelli F. 1998. Spatial variability in the distribution of sponges and cnidarians in a sublittoral marine cave with sulphur-water springs. Journal of the Marine Biological Association of the United Kingdom 78:43–58. DOI:10.1017/S0025315400039953.
- Bianchi CN, Dando PR, Morri C. 2011. Increased diversity of sessile epibenthos at subtidal hydrothermal vents: Seven hypotheses based on observations at Milos Island Aegean Sea. Advances in Oceanography and Limnology 2:1–31. DOI:10.1080/19475721.2011.565804.
- Bianchi CN, Pronzato R, Cattaneo-Vietti R, Benedetti Cecchi L, Morri C, Pansini M, Chemello R, Milazzo R, Fraschetti S, Terlizzi A, Peirano S, Salvati S, Benzoni F, Calcinai B, Cerrano C, Bavestrello G. 2004. Hard bottoms. Biologia Marina Mediterranea 11:185–215.
- Cárdenas P, Rapp HT. 2013. Disrupted spiculogenesis in deep‐water Geodiidae (Porifera Demospongiae) growing in shallow waters. Invertebrate Biology 132:173–194. DOI:10.1111/ivb.2013.132.issue-3.
- Cardigos F, Colaço A, Dando PR, Àvila SP, Sarradin PM, Tempera F, Conceição P, Pascoal A, Serrão Santos R. 2005. Shallow water hydrothermal vent field fluids and communities of the D João de Castro Seamount (Azores). Chemical Geology 224:153–168. DOI:10.1016/j.chemgeo.2005.07.019.
- Dando PR, Hughes JA, Leathy Y, Niven SJ, Smith C. 1995. Gas venting rates from submarine hydrothermal areas around the Island of Milos Hellenic Volcanic Arc. Continental Shelf Researchers 15:913–929. DOI:10.1016/0278-4343(95)80002-U.
- Desbruyeres D, Almeida A, Biscoito M, Comtet T, Khripounoff A, Le Bris N, Sarradin PM, Segonzac M. 2000. A review of the distribution of hydrothermal vent communities along the northern Mid-Atlantic Ridge: Dispersal vs environmental controls. Hydrobiologia 440:201–216. DOI:10.1023/A:1004175211848.
- Elvin D. 1971. Growth rates of the siliceous spicules of the fresh-water sponge Ephydatia muelleri (Lieberkun). Transaction American Microscopical Society 90:219–224. DOI:10.2307/3225028.
- Ferguson J, Lambert IB. 1972. Volcanic exhalations and metal enrichments at Matupi harbor New Britain TPNG. Economic Geology 67:25–37. DOI:10.2113/gsecongeo.67.1.25.
- Fricke H, Giere O, Stetter K, Alfredson GA, Kristjansson JK, Stoffers P, Svavarsson J. 1989. Hydrothermal vent communities at the shallow subpolar Mid-Atlantic Ridge. Marine Biology 102:425–429. DOI:10.1007/BF00428495.
- Grassle JE. 1986. The ecology of deep-sea hydrothermal vent communities. Advances in Marine Biology 23:301–362.
- Hansen HP, Grasshoff K. 1983. Automated chemical analysis. In: Grasshoff K, Ehrhardt M, Kremling K, editors. Methods of seawater analysis. 2nd ed. Weinheim: Verlag Chemie. pp. 347–379.
- Hashimoto J, Miura T, Fujikura K, Ossaka J. 1993. Discovery of vestimentiferan tube-worms in the euphotic zone. Zoological Sciences 10:1063–1067.
- Hinze G. 1903. Thiophysa volutans ein neues Schwefelbakterium. Berichte der Deutschen Botanischen Gesellschaft 21:309–316.
- Hooper JNA, van Soest RWM. 2002. Systema Porifera: A guide to the classification of sponges. New York: Kluwer Academic/Plenum Publishers.
- Kamenev GM, Fadeev VI, Selin NI, Tarasov VG, Malakhov VV. 1993. Composition and distribution of macro and meiobenthos around sublittoral hydrothermal vents in the Bay of Plenty New Zealand. New Zealand. Journal of Marine and Freshwater Researchers 27:407–418. DOI:10.1080/00288330.1993.9516582.
- Laborel J. 1960. Contribution à l’etude directe des peuplements benthiques sciaphiles sur substrat rocheux en Mediterranee. Recueil des Travaux de la Station Marine d’Endoume 20:117–173.
- Maldonado M, Carmon CM, Uriz MJ, Cruzado A. 1999. Decline in Mesozoic reef-building sponges explained by silicon limitation. Nature 401:785–788. DOI:10.1038/44560.
- Manini E, Luna GM, Corinaldesi C, Zeppilli D, Bortoluzzi G, Caramanna G, Raffa F, Danovaro R. 2008. Prokaryote diversity and virus abundance in shallow hydrothermal vents of the mediterranean sea (Panarea Island) and the Pacific Ocean (north Sulawesi-Indonesia). Microbial Ecology 55:626–639. DOI:10.1007/s00248-007-9306-2.
- McMurray SE, Blum JE, Pawlik JR. 2008. Redwood of the reef: Growth and age of the giant barrel sponge Xestospongia muta in the Florida keys. Marine Biology 155:159–171. DOI:10.1007/s00227-008-1014-z.
- Morri C, Bianchi CN, Cocito S, Peirano A, De Biasi AM, Aliani S, Pansini M, Boyer M, Ferdeghini F, Pestarino M, Dando P. 1999. Biodiversity of marine sessile epifauna at an Aegean island subject to hydrothermal activity: Milos Eastern Mediterranean Sea. Marine Biology 135:729–739. DOI:10.1007/s002270050674.
- Morri C, Cinelli F, Bianchi CN. 1994. Sessile epifauna gigantism in a submarine cave with sulphur springs. Cave Diving 6:4–9.
- Olafsson J, Thors K, Cann J. 1991. A sudden cruise off Iceland. RIDGE Events 2:35–38.
- Pansini M, Morri C, Bianchi CN. 2000. The sponge community of a subtidal area with hydrothermal vents: Milos Island Aegean Sea. Estuarine Coastal Marine Sciences 51:627–635. DOI:10.1006/ecss.2000.0674.
- Pè J. 1973. Etude quantitative de la régulation du squelette chez une éponge d’eau douche. Archives de Biologie 84:147–173.
- Pichler T, Dix G. 1996. Hydrothermal venting within a coral reef ecosystem Ambitle Island Papua New Guinea. Economic Geology 24:435–438. DOI:10.1130/0091-7613(1996)024<0435:HVWACR>2.3.CO;2.
- Rützler K. 1978. Sponges in coral reefs. In: Stoddart DR, Johannes RE, editors. Monographs on oceanographic methodology 5. Paris: UNESCO. pp. 299–313.
- Sarano FR, Murphy C, Houghton BF, Hedenquist JW. 1989. Preliminary observations of submarine geothermal activity in the vicinity of White Island Volcano Taupo Volcanic Zone New Zealand. Journal of the Royal Society of New Zealand 19:449–459. DOI:10.1080/03036758.1989.10421847.
- Sorokin DY. 1991. Oxidation of reduced compounds of sulfur in volcanically active areas of the Bay of Plenty (New Zealand) and Matupi Harbor (The New Britain Island Papua New Guinea). Izvestiya RAN Biology 3:376–387.
- Southward AJ. 1989. Animal communities fuelled by chemosynthesis: Life at hydrothermal vents cold seeps and in reducing sediments. Journal of Zoology 217:705–709. DOI:10.1111/jzo.1989.217.issue-4.
- Southward AJ, Southward E. 1993. Evolution of symbiosis between chemosynthetic bacteria and marine invertebrates. Marine Biological Association of the United Kingdom Annual Report 1992:13–15.
- Stein JL. 1984. Subtidal gastropods consume sulphur-oxidizing bacteria: Evidence from coastal hydrothermal vents. Science 223:696–698. DOI:10.1126/science.223.4637.696.
- Stone AR. 1970. Seasonal variations of spicule size in Hymeniacidon perleve. Journal of the Marine Biological Association of the United Kingdom 50:343–348. DOI:10.1017/S0025315400004562.
- Tarasov, VG. (Ed) 1991. Shallow-water Gasohydrothermal Vents and Ecosystem of Kraternaya Bight (Ushishir Volcano, Kurile Islands), Vol. I, Functional Characteristics. USSR Academy of Sciences, Far East Branch, Vladivostok (in Russian).
- Tarasov VG. 2006. Effects of shallow-water hydrothermal venting on biological communities of coastal marine ecosystems of the western Pacific. Advances in Marine Biology 50:267–421.
- Tarasov VG, Gebruk AV, Mironov AN, Moskalev LI. 2005. Deep-sea and shallow-water hydrothermal vent communities: Two different phenomena? Chemical Geology 224:5–39. DOI:10.1016/j.chemgeo.2005.07.021.
- Tarasov VG, Gebruk AV, Shulkin VM, Kamenev GM, Fadeev VI, Kosmynin VN, Malakhov VV, Starynin DA, Obzhirov AI. 1999. Effect of shallow-water hydrothermal venting on the biota of Matupi Harbour (Rabaul Caldera New Britain Island Papua New Guinea). Continental Shelf Researches 19:79–116. DOI:10.1016/S0278-4343(98)00073-9.
- Tarasov VG, Kondrashev SV, Lastivka TV. 1991. Oxygen metabolism of the diatom and bacterial mats of Kraternaya Bight. In: Tarasov VG, editor. Shallow-water vents and ecosystem of the Kraternaya Bight (Ushishir Volcano Kuriles). Vol. 1. Vladivostok: DVO RAN Press. pp. 4–19.
- Tarasov VG, Propp MV, Propp LN, Blinov SV, Kamenev GM. 1986. Shallow-water hydrothermal vents and a unique ecosystem of Kraternaya Caldera (Kurile Islands). Soviet Journal of Marine Biology 2:72–74.
- Tarasov VG, Propp MV, Propp LN, Zhirmunsky AV, Namsaraev BB, Gorlenko VM, Starynin DA. 1990. Shallow-water gashydrothermal vents of Ushishir Volcano and the ecosystem of Kraternaya Bight (the Kurile Island). Marine Ecology 11:1–23. DOI:10.1111/j.1439-0485.1990.tb00225.x.
- Tarasov VG, Propp MV, Zhirmunsky AV, Kostina EE. 1988. Coastal volcanism and specific ecosystems in the north-western Pacific. In: Il’ichev VI, editor. Pacific annual 88. Vladivostok: Russian Academy of Sciences Far East Branch. pp. 115–120.
- Tarasov VG, Zhirmunsky AV. 1989. Investigation of the ecosystem of Kraternaya Bight (Kurile Islands). Biologiya Morya 3:4–12.
- Thiermann F, Akoumianaki I, Hughes JA, Giere O. 1997. Benthic fauna of a shallow-water gaseohydrothermal vent area in the Aegean Sea (Greece). Marine Biology 128:149–159. DOI:10.1007/s002270050078.
- Van Soest RWM, Boury-Esnault N, Hooper JNA, Rützler K, de Voogd NJ, Alvarez B, de Glasby Hajdu E, Pisera AB, Manconi R, Schoenberg C, Janussen D, Tabachnick KR, Klautau M, Picton B, Kelly M, Vacelet J, Dohrmann M, Díaz MC, Cárdenas P 2016. World Porifera database [Online]. Available: http://wwwmarinespeciesorg/porifera. Accessed Jun 2016 5.
- Vidal VM, Vidal FM, Isaacs JD. 1978. Coastal submarine hydrothermal activity off northern Baja California. Journal Geophysical Researchers 83:1757–1774. DOI:10.1029/JB083iB04p01757.
- Zeppilli D, Danovaro R. 2009. Meiofaunal diversity and assemblage structure in a shallow-water hydrothermal vent in the Pacific Ocean. Aquatic Biology 5:75–84. DOI:10.3354/ab00140.