Abstract
Ovarian hormones, especially estrogen, may facilitate drug use vulnerability in females. Oxytocin is of special relevance to females and an important neuroendocrine factor regulating drug use. Mandarin vole (Microtus mandarinus) is a monogamous rodent, with complex nonsexual social behavior. Here, we examined whether ovariectomy produces effects on cocaine-induced aggressive behaviors, estrogen receptor alpha (ERα) and oxytocin in virgin female mandarin voles. The data indicate that repeated cocaine exposure increased aggressive behavior and reduced levels of oxytocin expression in the paraventricular nucleus in ovariectomized and sham-operated females. In addition, cocaine reduced the levels of ERα expression in the medial nuclei of the amygdale in sham-operated females. In contrast, cocaine decreased ERα expression in the medial preoptic area and increased serum estradiol levels in ovariectomized females. These results suggest that ovariectomy influences the effects of cocaine on central levels of ERα and oxytocin expression and serum estrogen levels. The estrogen system acts in concert with oxytocin to regulate cocaine-induced aggressive behavior in virgin females.
Introduction
Cocaine is a commonly abused drug and affects many different neurotransmitter systems, which in turn affect a number of behaviors or hormones (e.g. prolactin, estrogen and progesterone) that may be involved in cocaine-induced alterations in behavior (Johns et al. Citation1998a; Nelson et al. Citation1998). Gonadal hormones have been postulated to be important determinants of cocaine effects by influencing neuronal activity and plasticity of the brain (Chin et al. Citation2002). Circulating levels of ovarian hormones, especially estrogen, are a key biological substrate mediating female responses to drugs (Becker & Hu Citation2008; Segarra et al. Citation2010; Anker & Carroll Citation2011). For example, estradiol exacerbates the cocaine-induced locomotor response and affects the expression of cocaine-induced conditioned place preference (CPP) in female rats (Segarra et al. Citation2010; Twining et al. Citation2013; Bobzean et al. Citation2014). Effects of ovarian hormone on cocaine-induced behavioral sensitization have been widely researched in female rats (Rattus norvegicus Berkenhout, 1769) (Roberts et al. Citation1987; Sircar & Kim Citation1999; Quiñones-Jenab et al. Citation2000; Perrotti et al. Citation2001). However, previous work has paid less attention to other behavioral parameters. As social interaction encompasses different behavioral components with different functions and neurochemical circuits (Varlinskaya & Spear Citation2008), the effects of ovarian hormone on cocaine-induced social behavior and aggressive behavior requires further investigation.
Estrogen has been proven to modulate social behavior and aggressive behavior through estrogen receptor alpha (ERα) (Trainor et al. Citation2008; Zhai et al. Citation2008; Murakami et al. Citation2011; Wu et al. Citation2011). Decreased and eliminated ERα is associated with decreased aggression (Ogawa et al. Citation1998; Cushing & Wynne-Edwards Citation2006; Trainor et al. Citation2006b). Furthermore, neurobiological data suggest that estrogen may facilitate drug taking by interacting with reward- and stress-related systems (Anker & Carroll Citation2011). For instance, estradiol replacement enhances cocaine-stimulated locomotion through ERα in female mice (Van Swearingen et al. Citation2013). The neuropeptide oxytocin (OT) is of special relevance to females because it is regulated by estrogen (Nomura et al. Citation2002; Campbell Citation2008). OT also plays a significant role in regulating social behavior and aggression (Razzoli et al. Citation2003; Keverne & Curley Citation2004; Johns et al. Citation2010). Many effects of the OT system, especially those associated with social behavior, are estrogen-dependent. This estrogen-dependent effect requires ERα binding (Young et al. Citation1998a). Additionally, a growing body of evidence indicates OT is involved in modulating acute and long-term drug effects and inhibiting addiction-relevant behavior such as reducing self-administration, CPP, hyperactivity, stereotyped behavior and reinstatement (Sarnyai & Kovács Citation1994; McGregor & Bowen Citation2012; Hicks et al. Citation2014; Zanos et al. Citation2014).
The expression of social behavior, as well as their hormonal control, may vary in a pattern that is associated with social organization or the mating system of a species (Razzoli et al. Citation2003). Furthermore, ERα and OT may be critical regulating factors in determining monogamous social behavior (Young et al. Citation1998b; Cushing & Wynne-Edwards Citation2006).The mandarin vole (Microtus mandarinus Milne-Edwards, 1871) is a socially monogamous rodent with complex nonsexual social behavior (Smorkatcheva Citation1999; Tai et al. Citation2001). This rodent has an extended family group occupying a single burrow system, and shows a number of traits associated with a cooperative breeding system such as the delayed dispersal of juveniles and the presence of multiple successive litters within a nest (Smorkatcheva Citation1999; Tai & Wang Citation2001). In the lab, mandarin vole families have higher levels of social interaction via biparental, alloparental and affiliative behavior and stable pair-bonds (Wang et al. Citation2005; Song et al. Citation2010; Wu et al. Citation2011, Citation2013). The behavioral patterns of adult–adolescent interactions may be determined by adolescent development and prior familiarity (Wang & Tai Citation2012). Male and female dominant voles engage in higher levels of approaching, investigating, self-grooming and exploring behavior than subordinates do (Qiao et al. Citation2014). Unfamiliar female dyads display significantly more aggressive behavior and less affiliation than male dyads do (Zhai et al. Citation2008; Wu et al. Citation2011). Additionally, they display reinforcing effects in response to cocaine (Wang et al. Citation2012a, Citation2012b). This species is therefore an interesting model for studying the effects of drugs on social behavior and aggression. To determine whether cocaine-induced alterations of aggressive behavior are modulated by ovarian hormones and OT, the aggressive behavior and the levels of ERα and OT were explored in ovariectomized and intact female mandarin voles.
Materials and methods
Experimental animals
Mandarin voles used in this study were laboratory-reared animals that originated from a wild population in Henan, China. Animals were reared in conventional plastic cages (length × width × height: 46 cm × 22 cm × 16 cm). Cotton wool and wood shavings were provided for nesting material and changed weekly. The colony room was illuminated on a 14 h:10 h light:dark cycle (lights on at 20:00) and the temperature was maintained at 23 ± 2°C. Lighting was maintained at 200 lux. Water, carrots and standard rabbit chow (Xian Jiaotong University Laboratory Animal Center, Xian, China) were freely available. Immediately after weaning at the age of 21 days, female pups were separated from parents and housed with peers. At 70–80 days of age, healthy virgin females were used as subjects. Animal care followed the guidelines of the National Institute of Health and was approved by the Shaanxi Normal University Institutional Animal Care and Use Committee.
Ovariectomy
Since the behavioral effects of cocaine vary across the estrous stage for adult female rats (Sell et al. Citation2000; Kosten et al. Citation2005), only diestrous females were used in our study. Although sexually inexperienced female mandarin voles require male cues for natural estrous induction, we assessed the stage of the estrus cycle of virgin females by examining vaginal cytology (Byers et al. Citation2012). The stage of the estrous cycle was determined based on the presence or absence of leukocytes, cornified epithelial cells, and nucleated epithelial cells (Felicio et al. Citation1984; Byers et al. Citation2012). Specifically, when the female is in estrus, mostly cornified epithelial cells are present. Vaginal smears show primarily polymorphonuclear leukocytes and a few epithelial cells during diestrus. Twenty adult female voles were anesthetized with isofiourane (2 mL/kg, i.p.) and the bilateral ovaries were removed using a ventral approach. Another 16 females were sham-ovariectomized and experienced the same incisions as the ovariectomized females and sutured in an identical way. A one-week recovery period was provided to ensure the wound healed.
Drug treatments
Cocaine hydrochloride (Northwest Pharmaceutical, Sinopharm, China) was used in this experiment diluted in physiological saline (0.9% NaCl) (4.5 mg/mL), which was also used as vehicle. Cocaine injections were conducted 1 week after the ovariectomy or sham operation. Tested females were assigned to one of four treatment groups: Sham-S and Sham-C (n = 8 per group) were sham-operated female voles that received physiological saline and cocaine injections, respectively; OVX-S and OVX-C (n = 10 per group) were ovariectomized female voles that received physiological saline and cocaine injections, respectively. Sham-operated and ovariectomized females received a daily binge injection subcutaneously of cocaine or saline for 4 days in the dorsal caudal flank region. The daily binge pattern consisted of two injections of an identical dose of cocaine (20 mg/kg) or saline in 12 h at 08:00 and 14:00. Cocaine doses and pattern were chosen based on a previous study on effects of cocaine on CPP and aggressive behavior (Lubin et al. Citation2001; Wang et al. Citation2012b). Tested animals had similar body weights to stimulus animals. Stimulus animals (n = 36) are another set of group-housed females which were sexually naïve individuals of the same sex, and unfamiliar with the tested females.
Social interaction tests
Half an hour after the final injection, social interaction tests were carried out in a neutral plastic cage (44 cm × 22 cm × 16 cm), with 2 cm of wood shavings covering the floor and a removable opaque divider in the middle. In order to identify individuals, the stimulus animal was marked by cutting a bundle of hair on the back. Prior to testing, stimulus and focal animals were placed on opposite sides of the observed cage and acclimated for 10 min, but initially separated by a clapboard in the middle of the cage. Once the clapboard was removed the test commenced and we recorded the total duration of the following behaviors using a video camera for 15 min: “social sniffing behavior” included sniffing the face, body or anogenital area of an individual; “aggressive behavior” included pouncing (jumps or lunges), fighting (tumbling and biting) and chasing; “contact” with another individual included staying together or amicable grooming; “self-grooming” was cephalocaudal progression that begins with rhythmic movements of the paws around the mouth and the face, ears, descending to the ventrum, flank, anogenital area and tail; “exploration” involved the nose being very near to bedding in order to sniff substrate, moving in the cage and rising on the hind legs and sniffing the air or the wall of the cage; and “inactivity” was defined as sitting quietly. We cleaned the cage with alcohol solution after each test.
Tissue collection and immunochemistry
Following the social interaction test, animals were deeply anesthetized (isofiourane, 2 mL/kg, i.p.), and perfused with 0.1 M phosphate buffer solution (PBS, pH 7.4) and 4% paraformaldehyde in 0.1 M PBS. The brain was removed and placed in 4% paraformaldehyde overnight. Prior to dissection, brains were immersed in 30% sucrose until saturated. Coronal sections (40 µm) were cut on a cryostat and consecutive sections collected in two vials containing 0.01 M PBS to enable ERα and OT immunohistochemical staining. ERα (sc-542; Santa Cruz Biotechnologies, USA) and OT (AB911; Upstate, Lake Placid, USA) were rabbit polyclonal types. Floating sections were processed using the primary antibody and Streptavidin/Peroxidase methods (Bioss Company, Beijing, China). We incubated each vial per brain for 10 min with 3% H2O2, and then washed them for 4 × 5 min with 0.01 M PBS. Sections were preincubated for 90 min with normal goat serum (SP-0023), and incubated at 4°C overnight in primary antibody solution (ERα antibody, 1:100; OT antibody, 1: 5000) diluted using antibody diluent (0.01 M PBS containing 20% bovine serum albumin and 1.7% Trition-X-100). The next day, sections were washed 4 × 5 min with 0.01 M PBS and incubated for 60 min in a 37°C water bath with biotinylated goat anti-rabbit antibody (SP-0023), followed by another round of 4 × 5 min 0.01 M PBS. After 60 min of incubation with S-A/HRP and 4 × 10 min washing with 0.01 M PBS, sections were stained with 3, 3'-diaminobenzidine tetrahydrochloride for immunoreactive visualization.
The following brain regions were selected for analysis, taking into account their role in social behavior and aggressive in rodents (Gobrogge et al. Citation2007; Clipperton-Allen et al. Citation2012; Wang et al. Citation2013): the bed nucleus of stria terminalis (BNST), medial nuclei of the amygdala (MeA), medial preoptic area (MPOA), ventromedial nucleus of hypothalamic (VMH), paraventricular nucleus (PVN) and supraoptic nucleus (SON). OT is synthesized primarily in the PVN and SON.
An Olympus microscope was used to count stained nuclei. Slides were randomized and coded for microscopic analysis so that counters were blind to experimental treatment. The number of immunoreactive neurons was quantified by eye with the aid of a reticle placed in one ocular lens. For each brain area investigated, three representative sections from anterior to posterior, anatomically matched between subjects, were chosen and counted to minimize variability. Individual means were obtained by counting positive neurons bilaterally in three sections from each nucleus. Counts were performed separately for each hemisphere and results were averaged between hemispheres. Sections were chosen by correspondence to the reference atlas plate, not by the level or intensity of ERα-IR and OT-IR neuron labeling. Chosen sections were photographed with a Nikon (Tokyo, Japan) camera attached to an Olympus microscope.
Serum estradiol assays
Blood from experimental voles was collected by the heart via a terminal bleed. After collection, samples were immediately centrifuged and separated, and serum was stored at −20°C until assayed. The concentration of estradiol was measured using enzyme-linked immunosorbent assay kit (Shanghai Hushang Biotechnology, Shanghai, China) following standard procedures. The optimal dilutions of serum (1:5) were determined via dilution curves (1:1, 1:5 and 1:10). First, the prepared sample and the standard were incubated for 30 min at room temperature. Second, the prepared sample and the standard were placed in separate plate wells and horseradish peroxidase-conjugate reagent was added and incubated for 60 min at 37°C. Last, after the plate was washed four more times, we added Chromogen solutions A and B. After 15 min of incubation at 37°C, the reaction was stopped using a stop solution. The optical density of the sample was determined at 450 nm using a Metertech microplate reader (BioTek Instruments, Winooski, USA), and we set the blank well as zero. The variation between duplicate measurements was < 5%.
Statistical analyses
Statistical analyses were carried out using SPSS 13.0 (SPSS Inc., Chicago, Illinois, USA). Data were checked for normality using the one-sample Kolmogorov–Smirnov test. Data were compared using two-way analysis of variance (ANOVA) with ovariectomy and drug treatment as factors. Group differences were compared using post-hoc tests. All data are presented as mean ± standard error (SE). In all statistical tests, P values of less than 0.05 were considered significant.
Results
Social interactions
Two-way ANOVA revealed an effect of cocaine on the aggression (F(1, 32) = 6.395, p = 0.017) and no interaction between cocaine and ovariectomy (F(1, 32) = 0.449, p = 0.508). Ovariectomy had a main effect on the social sniffing behavior (F(1, 32) = 5.376, p = 0.027) and there was no interaction between cocaine and ovariectomy (F(1, 32) = 0.452, p = 0.506). This means that cocaine affected aggression in sham-operated and ovariectomized females, and ovariectomy affected social sniffing behavior in cocaine- and saline-treated females (). No effects of ovariectomy and cocaine, or an interaction between these two factors, were found for other behaviors (p > 0.05, data not shown) ().
Figure 1. Duration of social interaction in female mandarin voles. Asterisks (*) indicate levels of significance (P < 0.05). Sham-S and Sham-C: sham-ovariectomized females injected with saline and cocaine, respectively; OVX-S and OVX-C: ovariectomized females injected with saline and cocaine, respectively.
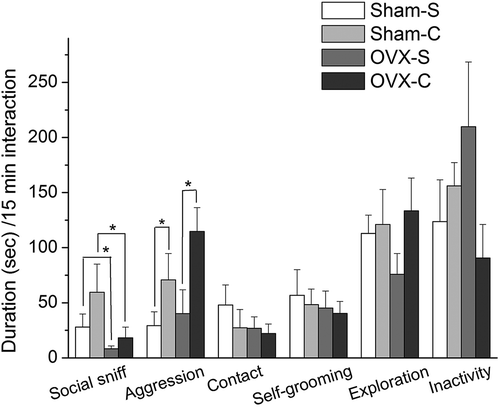
The expression of ERα and OT
Two-way ANOVA showed an effect of cocaine (F(1, 32) = 10.543, p = 0.002) and an interaction between ovariectomy and cocaine (F(1, 32) = 5.871, p = 0.019) on the number of ERα-IR neurons in the MeA. Interaction effects between ovariectomy and cocaine on the number of ERα-IR neurons in the BNST (F(1, 32) = 5.465, p = 0.022) and MPOA (F(1, 32) = 13.315, p = 0.001) were also found. Post-hoc tests showed that under saline treatment, ovariectomized females (OVX-S) had fewer MeA ERα-IR neurons compared to sham-operated females (SHAM-S) (mean difference = −22.706, p = 0.032). In comparison with saline-treated females (SHAM-S), cocaine-treated females (SHAM-C) had fewer MeA ERα-IR neurons in the sham-operated groups (mean difference = −45.039, p < 0.001). In the ovariectomized groups, cocaine-treated females (OVX-C) had fewer MPOA ERα-IR neurons than saline-treated females (OVX-S) (mean difference = −47.704, p < 0.001). Under cocaine treatment, ovariectomized females (OVX-C) have fewer ERα-IR neurons in the BNST (mean difference = −28.669, p = 0.005) and MPOA (mean difference = −42.797, p < 0.001) than saline-treated females (OVX-S). These group differences were not found in the VMH (p > 0.05) ( and ).
Figure 2. The number of ERα-IR neurons (A) and OT-IR neurons (B) in female mandarin voles. Groups not sharing same letters are significantly different. BNST, bed nucleus of stria terminalis; MeA, medial amygdale; MPOA, medial preoptic area; VMH, ventromedial nucleus of hypothalamic; PVN, paraventricular nucleus; SON, supraoptic nucleus. Sham-S and Sham-C: sham-ovariectomized females injected with saline and cocaine, respectively; OVX-S and OVX-C: ovariectomized females injected with saline and cocaine, respectively.
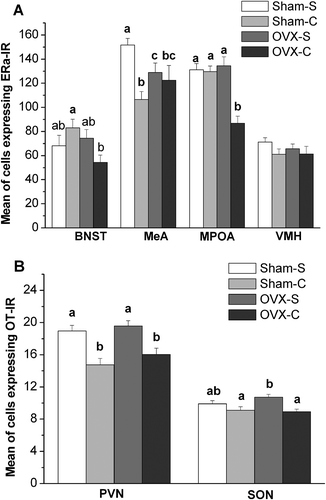
Figure 3. ERα immunopositive staining in female mandarin voles. Sham-S, Sham-C and OVX-S (A, B and C); Sham-C and OVX-C (D and H); Sham-C, OVX-S and OVX-C (E, F and G). MeA, medial amygdale; BNST, bed nucleus of stria terminalis; MPOA, medial preoptic area; Sham-S and Sham-C: sham-ovariectomized females injected with saline and cocaine, respectively; OVX-S and OVX-C: ovariectomized females injected with saline and cocaine, respectively. Scale bar: 200 µm.
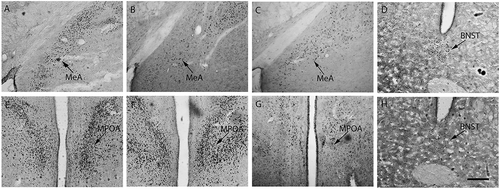
Cocaine significantly affected the number of OT-IR neurons in the PVN (F(1, 32) = 27.172, p < 0.001) and SON (F(1, 32) = 12.141, p = 0.001). There was no marked interaction between ovariectomy and cocaine for the number of OT-IR neurons in the PVN (F(1, 32) = 0.189, p = 0.664) and SON (F(1, 32) = 1.711, p = 0.192). Cocaine-treated females (SHAM-C/OVX-C) had fewer PVN OT-IR neurons compared to saline-treated females (SHAM-S/OVX-S) in the sham-operated (mean difference = –4.201, p < 0.001) and ovariectomized groups (mean difference = −3.554, p = 0.001). Furthermore, cocaine-treated females (OVX-C) had fewer SON OT-IR neurons than saline-treated females (OVX-S) in the ovariectomized groups (mean difference = −1.812, p = 0.001). Under the saline treatment, there was no difference in OT-IR neurons in the PVN (mean difference = −0.640, p = 0.514) and SON (mean difference = −0.812, p = 0.111) between sham-operated (SHAM-S) and ovariectomized females (OVX-S) ( and ).
Figure 4. Oxytocin immunopositive staining in female mandarin voles. Sham-S and Sham-C (A and B); OVX-S and OVX-C (C and D); OVX-S and OVX-C (E and F). PVN, paraventricular nucleus; SON, supraoptic nucleus. Sham-S and Sham-C: sham-ovariectomized females injected with saline and cocaine, respectively; OVX-S and OVX-C: ovariectomized females injected with saline and cocaine, respectively. Scale bar: 200 µm.
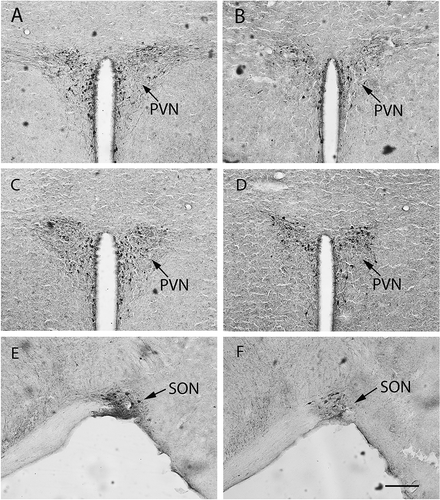
Concentration of serum estradiol
Data from enzyme-linked immunosorbent assay indicate that ovariectomy (F(1, 32) = 88.538, p < 0.001) and cocaine (F(1, 32) = 6.508, p = 0.019) affected serum estradiol levels. Under saline treatment, sham-operated females (SHAM-S) had higher levels of estradiol than ovariectomized females (OVX-S) (mean difference = 98.667, p < 0.001). Cocaine-treated females (OVX-C) had higher levels of estradiol than saline-treated females (OVX-S) in the ovariectomized groups (mean difference = 34.817, p = 0.016). There was no difference in serum estradiol levels between cocaine (SHAM-C) and saline-treated females (SHAM-S) in the sham-operated groups (mean difference = 12.683, p = 0.347) ().
Figure 5. Concentration of serum estradiol in female mandarin voles. Groups not sharing same letters are significantly different. Sham-S and Sham-C: sham-ovariectomized females injected with saline and cocaine, respectively. OVX-S and OVX-C: ovariectomized females injected with saline and cocaine, respectively.
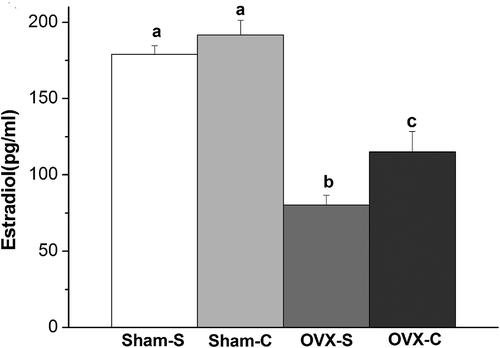
Discussion
The current study found that repeated cocaine administration would produce a similar change in aggressive behavior (toward an unfamiliar female) in ovariectomized females and in intact females. Following repeated cocaine exposure, sham-operated females had different levels of ERα expression in the BNST and MPOA, and serum estradiol, relative to ovariectomized females. Cocaine increased levels of serum estradiol and suppressed the expression of ERα in the MPOA and OT in the PVN and SON in ovariectomized females. Given that ovariectomy significantly reduced peripheral levels of estrogen, these findings demonstrate an interaction between ovarian hormones and cocaine that leads to different levels of neurochemical release.
Effects of ovariectomy on female mandarin voles
We found ovariectomy decreased the social investigation (social sniffing behavior) and the baseline levels of serum estradiol. A previous study found that ovariectomized Japanese macaques (Macaca fuscata Blyth, 1875) exhibited a significant decrease in positive social behavior (Coleman et al. Citation2011). In contrast, systemic estradiol-priming significantly increased time spent in social interaction with a conspecific in rats (Frye et al. Citation2008). Our results suggest that intact females investigated conspecifics more than ovariectomized females do, and these differences seem to depend upon circulating estrogen. Aggressive behavior was not altered following ovariectomy, indicating ovariectomy does not appear to affect female–female aggression at least during the first week following surgery. The effects of ovariectomy on aggression have been widely researched (Takahashi & Lisk Citation1983; DeBold & Miczek Citation1984). Albert et al. (Citation1992) found that circulating levels of ovarian hormones did not influence the level of aggression of a primiparous lactating female rat toward an unfamiliar female conspecific. Our findings are similar to those of a previous study where non-lactating female rats maintained consistent levels of aggressive behavior toward an intruder before and up to 7 weeks following ovariectomy (DeBold & Miczek Citation1984). Lubin et al. (Citation2001) proposed that aggressive behavior in virgin females may be more independent of normally cycling ovarian steroids. Thus, the present results are not surprising. There is evidence that estrogen increases aggression and decreases affiliation through ERa (Ogawa et al. Citation1997; Rissman et al. Citation1999; Scordalakes & Rissman Citation2004), and low levels of ERα in the anterior hypothalamus (AHA), MeA and BNST were associated with the effect of estrogen on aggression in monogamous California mice (Peromyscus californicus Gambel, 1848) (Cushing & Wynne-Edwards Citation2006; Trainor et al. Citation2008). However, another report suggested that the MeA is not involved in mediating changes in individual levels of aggression in female California mice (Davis & Marler Citation2004). Estrogens modulate aggressive behavior by acting on a circuit that includes the lateral septal nucleus (LS), ventral bed nucleus of the stria terminalis (vBNST) and AHA, and the source of estrogens is non-gonadal (Trainor et al. Citation2006a). Here, ovariectomy lowered the levels of MeA ERα expression, but not levels of aggression in saline-treated females. These results indirectly support the proposal by Trainor et al. (Citation2006a) that estrogen acts in a specific subset of a complex network of nuclei to affect aggressive behavior. He et al. (Citation2012) proposed that aggressive behavior and gonadal hormones have a complex relationship, and the effects of gonadal hormones on aggression are species dependent. Thus, the relationship between ovariectomy and aggressive behaviors may be species typical and variable. Female mandarin voles display more aggressive behavior than males do (Zhai et al. Citation2008). The persistent aggression in ovariectomized mandarin voles may be associated with this trait.
Effects of cocaine on female mandarin voles
The majority of studies on cocaine treatment and aggression in female rodents emphasize maternal aggression (Johns et al. Citation2010). Chronic cocaine treatment has been shown to increase maternal aggression during the postpartum period (Johns et al. Citation1994, Citation1998a), while acute treatment has been shown to decrease it (Johns et al. Citation1994, Citation1998b; McMurray et al. Citation2008). Here, we found that repeated cocaine exposure increased aggressive behavior in ovariectomized and sham-operated virgin females. The previous study in female mandarin voles found 24 h of cocaine withdrawal attenuates the aggressive behavior (Wang et al. Citation2012b). Since the aggressive behaviors may also be affected by the patterns of drug administration (single or binge administration), the dosing regimens and the specific temporal window assessed (Estelles et al. Citation2004, Citation2007; Wang et al. Citation2012b), this discrepancy may be due to the fact that the previous study chose a pattern of single administration and temporal window of testing after 24 h, instead of binge administration and a half hour, respectively, as in the present study.
In addition, cocaine decreased the expression of MeA ERα-IR neurons in sham-operated females, while it decreased the expression of MPOA ERα-IR neurons and increased serum estradiol levels in ovariectomized females, indicating these neurochemical variations contribute to altering aggressive behaviors. Unlike in sham-operated counterparts, the MeA ERα-IR neurons were not reduced by cocaine in ovariectomized females, and it is possible that we observed a “floor effect” due to a low level of MeA ERα expression following ovariectomy. Although estrogen is secreted mainly from the follicle and corpus luteum in the ovary, it is also secreted from the adrenals and therefore ovariectomized females have a high level of circulating estradiol following repeated cocaine exposure. Cocaine-induced sensitization of brain activity is dependent on plasma estradiol in female rats (Segarra et al. Citation2010). Our results indicate that the adrenal gland is also a source of cocaine-stimulated estrogen response and this response may contribute to the effects of cocaine on aggression. This was indirectly supported by a previous report showing adrenal hormones are necessary during the initiation phase of sensitization when observed after early withdrawal (1 day; Prasad et al. Citation1996). Estrogen and its mimic have been proven to increase the probability and intensity of aggressive behavior (Moore et al. Citation2004; Wisniewski et al. Citation2005; Patisaul & Polston Citation2008). Several lines of evidence suggest that estrogen may facilitate aggression via ERα and inhibit aggression via ERβ (Nomura et al. Citation2002; Scordalakes & Rissman Citation2004). One possible mechanism for increased aggressive behavior in cocaine-treated female voles is that aggression depends not only on the different roles of ERα and ERβ but also on their relative ratio (Trainor et al. Citation2006b) (although ERβ was not measured here).
The enhancement of aggressive behavior in ovariectomized and sham-operated females was accompanied by the reduction of OT expression in the PVN, indicating that OT plays an important role in modulating cocaine-induced female–female aggression. This result is consistent with previous findings that cocaine exposure may inhibit central OT production (Johns et al. Citation1998a; Sarnyai Citation1999; Wang et al. Citation2014). OT was particularly effective in the modulation of agonistic behavior during an initial female–female encounter. For instance, OT decreased aggression in females, producing low levels of agonistic interactions (Razzoli et al. Citation2003). Neonatal exposure to OT may increase aggression in female mandarin voles (Jia et al. Citation2008), and chronic continuous cocaine-treated females were more aggressive than controls, while OT levels were lower in the MPOA (Johns et al. Citation2010). Since downstream effectors of gonadal hormone action may include OT (Choleris et al. Citation2009), OT may be a putative downstream effector of estrogen action and act more directly to regulate the aggression displayed by females toward females following repeated cocaine exposure. The effects of estrogen on behavior are, in part, likely related to the modulation of OT systems (Michopoulos et al. Citation2011). It is believed that variations of estrogen receptor levels are associated with differences in social interaction through the OT and arginine vasopressin (AVP) systems, by upregulating gene expression for those peptides and their receptors (Murakami et al. Citation2011). It is therefore possible that OT is also involved in the modulation of ovarian hormones in drug use in females.
In summary, cocaine alters aggressive behavior and produced different effects on levels of ERα and OT expression and serum estrogen in ovariectomized and intact female voles. The change of aggressive behavior is partly modulated by OT and estrogen system. This variation in ovarian hormones could be related to differences in neuroendocrine response in cocaine abuse. The present results demonstrate an interactive effect between ovarian hormones and cocaine on neurochemical release in females. Drug abuse amongst women has grown rapidly; therefore, more research on female drug addicts is needed. However, the underlying biological mechanisms mediating cocaine-induced increases in female aggressive behavior are not fully understood (Johns et al. Citation2010). Further experiments are needed to determine the precise neural mechanisms regulated by ovarian hormones responsible for the patterns described here, and drug vulnerability in females.
Additional information
Funding
References
- Albert DJ, Jonik RH, Walsh ML. 1992. Ovariectomy does not attenuate aggression by primiparous lactating female rats. Physiology & Behavior 52:1043–1046. DOI:10.1016/0031-9384(92)90457-D.
- Anker JJ, Carroll ME. 2011. Females are more vulnerable to drug abuse than males: Evidence from preclinical studies and the role of ovarian hormones. Current Topics in Behavioral Neurosciences 8:73–96.
- Becker JB, Hu M. 2008. Sex differences in drug abuse. Frontiers in Neuroendocrinology 29:36–47. DOI:10.1016/j.yfrne.2007.07.003.
- Bobzean SA, Dennis TS, Perrotti LI. 2014. Acute estradiol treatment affects the expression of cocaine-induced conditioned place preference in ovariectomized female rats. Brain Research Bulletin 103:49–53. DOI:10.1016/j.brainresbull.2014.02.002.
- Byers SL, Wiles MV, Dunn SL, Taft RA. 2012. Mouse estrous cycle identification tool and images. Plos One 7:e35538. DOI:10.1371/journal.pone.0035538.
- Campbell A. 2008. Attachment, aggression and affiliation: The role of oxytocin in female social behavior. Biological Psychology 77:1–10. DOI:10.1016/j.biopsycho.2007.09.001.
- Chin J, Sternin O, Wu HB, Burrell S, Lu D, Jenab S, Perrotti LI, Quiñones-Jenab V. 2002. Endogenous gonadal hormones modulate behavioral and neurochemical responses to acute and chronic cocaine administration. Brain Research 945:123–130. DOI:10.1016/S0006-8993(02)02807-X.
- Choleris E, Clipperton-Allen AE, Phan A, Kavaliers M. 2009. Neuroendocrinology of social information processing in rats and mice. Frontiers in Neuroendocrinology 30:442–459. DOI:10.1016/j.yfrne.2009.05.003.
- Clipperton-Allen AE, Lee AW, Reyes A, Devidze N, Phan A, Pfaff DW, Cholerisa E. 2012. Oxytocin, vasopressin and estrogen receptor gene expression in relation to social recognition in female mice. Physiology & Behavior 105:915–924. DOI:10.1016/j.physbeh.2011.10.025.
- Coleman K, Robertson ND, Bethea CL. 2011. Long-term ovariectomy alters social and anxious behaviors in semi-free ranging Japanese macaques. Behavioural Brain Research 225:317–327. DOI:10.1016/j.bbr.2011.07.046.
- Cushing BS, Wynne-Edwards KE. 2006. Estrogen receptor-distribution in male rodents is associated with social organization. Journal of Comparative Neurology 494:595–605.
- Davis ES, Marler CA. 2004. c-fos Changes following an aggressive encounter in female California mice: A synthesis of behavior, hormone changes and neural activity. Neuroscience 127:611–624. DOI:10.1016/j.neuroscience.2004.05.034.
- DeBold JF, Miczek KA. 1984. Aggression persists after ovariectomy in female rats. Hormones and Behavior 18:177–190. DOI:10.1016/0018-506X(84)90041-2.
- Estelles J, Lluch J, Rodríguez-Arias M, Aguilar MA, Miñarro J. 2007. Cocaine exposure during adolescence affects anxiety in adult mice. Brain Research Bulletin 71:393–403. DOI:10.1016/j.brainresbull.2006.10.008.
- Estelles J, Rodrı́guez-Arias M, Aguilar M, Miñarro J. 2004. Social behavioural profile of cocaine in isolated and grouped male mice. Drug and Alcohol Dependence 76:115–123. DOI:10.1016/j.drugalcdep.2004.04.019.
- Felicio LS, Nelson JF, Finch CE. 1984. Longitudinal studies of estrous cyclicity in aging C57BL/6J mice: II. Cessation of cyclicity and the duration of persistent vaginal cornification. Biology of Reproduction 31:446–453. DOI:10.1095/biolreprod31.3.446.
- Frye CA, Paris JJ, Rhodes ME. 2008. Estrogen is necessary for 5alpha- pregnan- 3alpha- ol- 20-one (3alpha, 5alpha-THP) infusion to the ventral tegmental area to facilitate social and sexual, but neither exploratory nor affective behavior of ovariectomized rats. Pharmacology Biochemistry and Behavior 91:261–270. DOI:10.1016/j.pbb.2008.08.018.
- Gobrogge KL, Liu Y, Jia X, Wang Z. 2007. Anterior hypothalamic neural activation and neurochemical associations with aggression in pair-bonded male prairie voles. Physiology & Behavior 502:1109–1122.
- He F, Tai F, Zhang Y, Zhang X. 2012. Effects of castration on aggression and levels of serum sex hormones and their central receptors in mandarin voles (Microtus mandarinus). Journal of Comparative Physiology A 198:347–362. DOI:10.1007/s00359-012-0713-6.
- Hicks C, Cornish JL, Baracz SJ, Suraev A, McGregor IS. 2014. Adolescent pre-treatment with oxytocin protects against adult methamphetamine-seeking behavior in female rats. Addiction Biology. DOI:10.1111/adb.12197.
- Jia R, Tai FD, An SC, Broders H, Ding XL, Kong Q, Zhao L, Zhang H. 2008. Effects of neonatal oxytocin treatment on aggression and neural activities in mandarin voles. Physiology & Behavior 95:56–62. DOI:10.1016/j.physbeh.2008.04.015.
- Johns JM, McMurray MS, Joyner PW, Jarrett TM, Williams SK, Cox ET, Black MA, Middleton CL, Walker CH. 2010. Effects of chronic and intermittent cocaine treatment on dominance, aggression, and oxytocin levels in post-lactational rats. Psychopharmacology (Berl) 211:175–185. DOI:10.1007/s00213-010-1877-9.
- Johns JM, Nelson CJ, Meter KE, Lubin DA, Couch CD, Ayers A, Walker CH. 1998b. Dose-dependent effects of multiple acute cocaine injections on maternal behavior and aggression in sprague-dawley rats. Developmental Neuroscience 20:525–532.
- Johns JM, Noonan LR, Zimmerman LI, Li L, Pedersen CA. 1994. Effects of chronic and acute cocaine treatment on the onset of maternal behavior and aggression in sprague-dawley rats. Behavioral Neuroscience 108:107–112. DOI:10.1037/0735-7044.108.1.107.
- Johns JM, Noonan LR, Zimmerman LI, McMillen BA, Means LW, Walker CH. 1998a. Chronic cocaine treatment alters social/aggressive behavior in Sprague– Dawley rat dams and in their prenatally exposed offspring. In: Harvey JA. Kosofsky BE, editors. Cocaine: Effects on the Developing Brain. Annals of the New York Academy of Sciences 846:399–404. DOI:10.1111/j.1749-6632.1998.tb09765.x.
- Keverne EB, Curley J. 2004. Vasopressin, oxytocin and social behaviour. Current Opinion in Neurobiology 14:777–783. DOI:10.1016/j.conb.2004.10.006.
- Kosten TA, Sanchez H, Jatlow PI, Kehoe P. 2005. Neonatal isolation alters the estrous cycle interactions on the acute behavioral effects of cocaine. Psychoneuroendocrinology 30:753–761. DOI:10.1016/j.psyneuen.2005.03.004.
- Lubin DA, Meter KE, Walker CH, Johns JM. 2001. Effects of chronic cocaine administration on aggressive behavior in virgin rats. Progress in Neuro-Psychopharmacology & Biological Psychiatry 25:1421–1433. DOI:10.1016/S0278-5846(01)00196-8.
- McGregor IS, Bowen MT. 2012. Breaking the loop: Oxytocin as a potential treatment for drug addiction. Hormones and Behavior 61:331–339. DOI:10.1016/j.yhbeh.2011.12.001.
- McMurray MS, Joyner PW, Middleton CW, Jarrett TM, Elliott DL, Black MA, Hofler VE, Walker CH, Johns JM. 2008. Intergenerational effects of cocaine on maternal aggressive behavior and brain oxytocin in rat dams. Stress 11:398–411. DOI:10.1080/10253890701850239.
- Michopoulos V, Checchi M, Sharpe D, Wilson ME. 2011. Estradiol effects on behavior and serum oxytocin are modified by social status and polymorphisms in the serotonin transporter gene in female rhesus monkeys. Hormones and Behavior 59:528–535. DOI:10.1016/j.yhbeh.2011.02.002.
- Moore TO, Karom M, O’Farrell L. 2004. The neurobehavioral effects of phytoestrogens in male Syrian hamsters. Brain Research 1016:102–110. DOI:10.1016/j.brainres.2004.04.073.
- Murakami G, Hunter RG, Fontaine C, Ribeiro A, Pfaff D. 2011. Relationships among estrogen receptor, oxytocin and vasopressin gene expression and social interaction in male mice. European Journal of Neuroscience 34:469–477. DOI:10.1111/ejn.2011.34.issue-3.
- Nelson CJ, Meter KE, Walker CH, Ayers AA, Johns JM. 1998. A dose–response study of chronic cocaine on maternal behavior in rats. Neurotoxicology and Teratology 20:657–660. DOI:10.1016/S0892-0362(98)00016-6.
- Nomura M, Durbak I, Chan J, Smithies O, Gustafsson JA, Korach KS, Pfaff DW, Ogawa S. 2002. Genotype/age interactions on aggressive behavior in gonadally intact estrogen receptor beta knockout (betaERKO) male mice. Hormones and Behavior 41:288–296. DOI:10.1006/hbeh.2002.1773.
- Ogawa S, Lubahn DB, Korach KS, Pfaff DW. 1997. Behavioral effects of estrogen receptor gene disruption in male mice. Proceedings of the National Academy of Science USA 94:1476–1481. DOI:10.1073/pnas.94.4.1476.
- Ogawa S, Washburn TF, Taylor J, Lubahn DB, Korach KS, Pfaff DW. 1998. Modifications of testosterone-dependent behaviors by estrogen receptor-alpha gene disruption in male mice. Endocrinology 139:5058–5069.
- Patisaul HB, Polston EK. 2008. Influence of endocrine active compounds on the developing rodent brain. Brain Research Reviews 57:352–362. DOI:10.1016/j.brainresrev.2007.06.008.
- Perrotti LI, Russo SJ, Lagos F, Quiñones-Jenab V. 2001. Vendor differences in cocaine-induced behavioral activity and hormonal interactions in ovariectomized Fischer rats. Brain Research Bulletin 54:1–5. DOI:10.1016/S0361-9230(00)00330-0.
- Prasad BM, Ulibarri C, Kalivas PW, Sorg BA. 1996. Effect of adrenalectomy on the initiation and expression of cocaine-induced sensitization. Psychopharmacology 125:265–273. DOI:10.1007/BF02247338.
- Qiao XF, Yan YT, Wu RY, Tai FD, Hao P, Cao Y, Wang JL. 2014. Sociality and oxytocin and vasopressin in the brain of male and female dominant and subordinate mandarin voles. Journal of Comparative Physiology A 200:149–159. DOI:10.1007/s00359-013-0870-2.
- Quiñones-Jenab V, Perrotti LI, Mc Monagle J, Ho A, Kreek MJ. 2000. Ovarian hormone replacement affects cocaine-induced behaviors in ovariectomized female rats. Pharmacology Biochemistry and Behavior 67:417–422. DOI:10.1016/S0091-3057(00)00381-6.
- Razzoli M, Cushing BS, Carter CS, Valsecchi P. 2003. Hormonal regulation of agonistic and affiliative behavior in female mongolian gerbils (Meriones unguiculatus). Hormones and Behavior 43:549–553. DOI:10.1016/S0018-506X(03)00064-3.
- Rissman EF, Wersinger SR, Fugger HN, Foster TC. 1999. Sex with knockout models: Behavioural studies of estrogen receptor alpha. Brain Research 835:80–90. DOI:10.1016/S0006-8993(99)01452-3.
- Roberts DC, Dalton JC, Vickers GJ. 1987. Increased self-administration of cocaine following haloperidol: Effect of ovariectomy, estrogen replacement, and estrous cycle. Pharmacology Biochemistry and Behavior 26:37–43. DOI:10.1016/0091-3057(87)90530-2.
- Sarnyai Z. 1999. Oxytocin and neuroadaptation to cocaine. Progress in Brain Research 119:449–466.
- Sarnyai Z, Kovács GL. 1994. Role of oxytocin in the neuroadaptation to drugs of abuse. Psychoneuroendocrinology 19:85–117. DOI:10.1016/0306-4530(94)90062-0.
- Scordalakes EM, Rissman EF. 2004. Aggression and arginine vasopressin immunoreactivity regulation by androgen receptor and estrogen receptor alpha. Genes, Brain and Behavior 3:20–26. DOI:10.1111/gbb.2004.3.issue-1.
- Segarra AC, Agosto-Rivera JL, Febo M, Lugo-Escobar N, Menéndez-Delmestre R, Puig-Ramos A, Torres-Diaz YM. 2010. Estradiol: A key biological substrate mediating the response to cocaine in female rats. Hormones and Behavior 58:33–43. DOI:10.1016/j.yhbeh.2009.12.003.
- Sell SL, Scalzitti JM, Thomas ML, Cunningham KA. 2000. Influence of ovarian hormones and estrous cycle on the behavioral response to cocaine in female rats. Journal of Pharmacology and Experimental Therapeutics 293:879–886.
- Sircar R, Kim D. 1999. Female gonadal hormones differentially modulate cocaine-induced behavioral sensitization in Fischer, Lewis, and Sprague-Dawley rats. Journal of Pharmacology and Experimental Therapeutics 289:54–65.
- Smorkatcheva AV. 1999. The social organization of the mandarine vole, Lasiopodomys mandarinus, during the reproductive period. Zeitschrift fur Säugetierkunde 64:344–355.
- Song ZZ, Tai FD, Yu CJ, Wu RY, Zhang X, Broders H, He FQ, Guo R. 2010. Sexual or paternal experiences alter alloparental behavior and the central expression of ERα and OT in male mandarin voles (Microtus mandarinus). Behavioural Brain Research 214:290–300. DOI:10.1016/j.bbr.2010.05.045.
- Tai F, Wang T. 2001. Social organization of mandarin voles in burrow system. Acta Theriologica Sinica 21:50–56.
- Tai F, Wang T, Zhao Y. 2001. Mate choice and related characteristics of mandarin vole (Microtus mandarinus). Acta Zoologica Sinica 47:266–271.
- Takahashi LK, Lisk RD. 1983. Organization and expression of agonistic and socio-sexual behavior in golden hamsters over the estrous cycle and after ovariectomy. Physiology & Behavior 31:477–482. DOI:10.1016/0031-9384(83)90069-0.
- Trainor BC, Finy MS, Nelson RJ. 2008. Rapid effects of estradiol on male aggression depend on photoperiod in reproductively non-responsive mice. Hormones and Behavior 53:192–199. DOI:10.1016/j.yhbeh.2007.09.016.
- Trainor BC, Greiwe KM, Nelson RJ. 2006a. Individual differences in estrogen receptor α in select brain nuclei are associated with individual differences in aggression. Hormones and Behavior 50:338–345. DOI:10.1016/j.yhbeh.2006.04.002.
- Trainor BC, Kyomen HH, Marler CA. 2006b. Estrogenic encounters: How interactions between aromatase and the environment modulate aggression. Frontiers in Neuroendocrinology 27:170–179. DOI:10.1016/j.yfrne.2005.11.001.
- Twining RC, Tuscher JJ, Doncheck EM, Frick KM, Mueller D. 2013. 17β-estradiol is necessary for extinction of cocaine seeking in female rats. Learning & Memory 20:300–306. DOI:10.1101/lm.030304.113.
- Van Swearingen AE, Sanchez CL, Frisbee SM, Williams A, Walker QD, Korach KS, Kuhn CM. 2013. Estradiol replacement enhances cocaine-stimulated locomotion in female C57BL/6 mice through estrogen receptor alpha. Neuropharmacology 72:236–249. DOI:10.1016/j.neuropharm.2013.04.015.
- Varlinskaya EI, Spear LP. 2008. Social interactions in adolescent and adult Sprague–Dawley rats: Impact of social deprivation and test context familiarity. Behavioural Brain Research 188:398–405. DOI:10.1016/j.bbr.2007.11.024.
- Wang JL, Tai FD. 2012. Effects of different periods of post-weaning separation onfather-son interaction and recognition in mandarin voles. Ethology Ecology & Evolution 24:117–126. DOI:10.1080/03949370.2011.597780.
- Wang JL, Tai FD, An SC. 2005. Recognition memory for mate in lactating mandarin vole. Chinese Journal of Zoology 40:25–29.
- Wang JL, Tai FD, Lai XJ. 2014. Cocaine withdrawal influences paternal behavior and associated central expression of vasopressin, oxytocin and tyrosine hydroxylase in mandarin voles. Neuropeptides 48:29–35. DOI:10.1016/j.npep.2013.10.016.
- Wang JL, Tai FD, Yu P, Wu RY. 2012a. Reinforcing properties of pups versus cocaine for fathers and associated central expression of Fos and tyrosine hydroxylase in mandarin voles (Microtus mandarinus). Behavioural Brain Research 230:149–157. DOI:10.1016/j.bbr.2012.02.005.
- Wang JL, Tai FD, Zhang LX, Zhang P. 2012b. Cocaine-induced rewarding properties, behavioural sensitization and alteration in social behavious in group-housed and post-puberty isolated female mandarin voles. Behavioural Pharmacology 23:693–702. DOI:10.1097/FBP.0b013e328358603c.
- Wang Y, He Z, Zhao C, Li L. 2013. Medial amygdala lesions modify aggressive behavior and immediate early gene expression in oxytocin and vasopressin neurons during intermale exposure. Behavioural Brain Research 245:42–49. DOI:10.1016/j.bbr.2013.02.002.
- Wisniewski AB, Cernetich A, Gearhart JP, Klein SL. 2005. Perinatal exposure to genistein alters reproductive development and aggressive behavior in male mice. Physiology & Behavior 84:327–334. DOI:10.1016/j.physbeh.2004.12.008.
- Wu RY, Song ZZ, Tai FD, An XL, Yu P, Li YN. 2013. The effect of alloparental experience and care on anxiety-like, social and parental behaviour in adult mandarin voles. Animal Behaviour 85:61–69. DOI:10.1016/j.anbehav.2012.10.007.
- Wu RY, Yuan AF, Yuan QW, Guo R, Tai FD, Song ZZ, Yu CJ. 2011. Comparison of sociability, parental care and central estrogen receptor alpha expression between two populations of mandarin voles (Microtus mandarinus). Journal of Comparative Physiology A 197:267–277. DOI:10.1007/s00359-010-0609-2.
- Young LJ, Wang Z, Donaldson R, Rissman EF. 1998a. Estrogen receptor α is essential for induction of oxytocin receptor by estrogen. Neuroreport 9:933–936. DOI:10.1097/00001756-199803300-00031.
- Young LJ, Wang Z, Insel TR. 1998b. Neuroendocrine bases of monogamy. Trends in Neurosciences 21:71–75. DOI:10.1016/S0166-2236(97)01167-3.
- Zanos P, Wright SR, Georgiou P, Yoo JH, Ledent C, Hourani SM, Kitchen I, Winsky-Sommerer R, Bailey A. 2014. Chronic methamphetamine treatment induces oxytocin receptor up-regulation in the amygdala and hypothalamus via an adenosine A2A receptor-independent mechanism. Pharmacology, Biochemistry & Behavior 119:72–79. DOI:10.1016/j.pbb.2013.05.009.
- Zhai P, Xue H, Tai F, Jia R. 2008. Inter-sexual variation in social interactions and distribution of estrogen receptor alpha in the brain of mandarin voles Microtus mandarinus. Acta Zoologica Sinica 54:1020–1028.